Introduction
Schistosomiasis is a parasitic disease caused by trematodes of the genus Schistosoma. It is widespread in sub-Saharan Africa; yet, a key epidemiological feature of schistosomiasis is its focal distribution (Colley et al., Reference Colley, Bustinduy, Secor and King2014; Lai et al., Reference Lai, Biedermann, Ekpo, Garba, Mathieu, Midzi, Mwinzi, N'Goran, Raso, Assaré, Sacko, Schur, Talla, Tchuem-Tchuenté, Touré, Winkler, Utzinger and Vounatsou2015). The disease affects more than 250 million people and it is estimated to have caused 1.4 million disability-adjusted life years in 2017 (Hotez et al., Reference Hotez, Alvarado, Basáñez, Bolliger, Bourne, Boussinesq, Brooker, Brown, Buckle, Budke, Carabin, Coffeng, Fèvre, Fürst, Halasa, Jasrasaria, Johns, Keiser, King, Lozano, Murdoch, O'Hanlon, Pion, Pullan, Ramaiah, Roberts, Shepard, Smith, Stolk, Undurraga, Utzinger, Wang, Murray and Naghavi2014; GBD 2017 DALYs and HALE Collaborators, 2018).
In Côte d'Ivoire, urogenital and intestinal schistosomiasis, caused by Schistosoma haematobium and Schistosoma mansoni, respectively, are endemic in humans and crossing of open water sources is a key risk factor for transmission of schistosomiasis (Chammartin et al., Reference Chammartin, Houngbedji, Hürlimann, Yapi, Silué, Soro, Kouamé, N'Goran, Utzinger, Raso and Vounatsou2014; Krauth et al., Reference Krauth, Musard, Traoré, Zinsstag, Achi, N'Goran and Utzinger2015). While S. mansoni is widespread in the western part of the country (Assaré et al., Reference Assaré, Lai, Yapi, Tian-Bi, Ouattara, Yao, Knopp, Vounatsou, Utzinger and N'Goran2015), S. haematobium is mostly present in the central and southern parts of Côte d'Ivoire (Coulibaly et al., Reference Coulibaly, N'Gbesso, N'Guessan, Winkler, Utzinger and N'Goran2013). In the northern part of Côte d'Ivoire, a recent study found a low prevalence among school-aged children for both S. haematobium (1.9%) and S. mansoni (3.5%) (M'Bra et al., Reference M'Bra, Koné, Yapi, Silué, Sy, Vienneau, Soro, Cissé and Utzinger2018). Schistosoma bovis, a parasite of domestic animals, has also been reported in Côte d'Ivoire, but there is a paucity of recent data. In 1997, post-mortem examinations of cattle in the savannah area of Côte d'Ivoire revealed a prevalence of 35% (Achi et al., Reference Achi, Zinsstag, Yéo, Dea and Dorchies2003).
Schistosoma haematobium and S. bovis are phylogenetically closely related and freshwater snails of the genus Bulinus act as intermediate hosts for both species (Cook and Zumla, Reference Cook and Zumla2009). The close phylogenetic association between these two species enables inter-species mating and can result in hybridization between the two species, which might influence disease transmission and alter phenotypic characteristics of parasites in both human and animal (Huyse et al., Reference Huyse, Webster, Geldof, Stothard, Diaw, Polman and Rollinson2009; Boissier et al., Reference Boissier, Grech-Angelini, Webster, Allienne, Huyse, Mas-Coma, Toulza, Barré-Cardi, Rollinson, Kincaid-Smith, Oleaga, Galinier, Foata, Rognon, Berry, Mouahid, Henneron, Moné, Noel and Mitta2016). Of particular concern, hybridization could enhance transmission and expand the distribution of these species. For instance, laboratory hybrids exhibit particularly enhanced life-history traits, including increased virulence, expanded snail host spectrum, maturation and egg production (Leger and Webster, Reference Leger and Webster2017). There is a need to study S. haematobium-bovis hybrids and determine how such hybrids might influence the epidemiology and control of schistosomiasis in terms of virulence, zoonotic potential and resistance to treatment. Previous work focused on Benin, Mali, Niger and Senegal (Leger and Webster, Reference Leger and Webster2017). Recently, S. haematobium-bovis hybrids have been found in Malawi (Webster et al., Reference Webster, Alharbi, Kayuni, Makaula, Halstead, Christiansen, Juziwelo, Stanton, LaCourse, Rollinson, Kalua and Stothard2019) and hybrids have been involved in infection in Corsica, France (Boissier et al., Reference Boissier, Grech-Angelini, Webster, Allienne, Huyse, Mas-Coma, Toulza, Barré-Cardi, Rollinson, Kincaid-Smith, Oleaga, Galinier, Foata, Rognon, Berry, Mouahid, Henneron, Moné, Noel and Mitta2016), which demonstrates the capacity for long-range dispersion. A high prevalence and focal transmission of S. haematobium-bovis hybrids in Bulinus snails have been shown in Côte d'Ivoire (Tian-Bi et al., Reference Tian-Bi, Webster, Konan, Allan, Diakité, Ouattara, Salia, Koné, Kakou, Rabone, Coulibaly, Knopp, Meïté, Utzinger, N'Goran and Rollinson2019), but the occurrence of such hybrids in humans has not yet been investigated.
This study molecularly characterized schistosome miracidia collected from schoolchildren in four locations in Côte d'Ivoire to investigate the presence and extent of S. haematobium-bovis hybrids.
Materials and methods
Ethical consideration
Ethical clearance for this study was obtained from the Ministère de la Santé et de l'Hygiène Publique de Côte d'Ivoire (reference no. 003-18/MSHP/CNER-kp). School authorities, teachers, participating children and their parents/guardians were informed about the objectives, procedures, and potential risks and benefits of the study. Written informed consent was obtained from children's parents/guardians, while children provided oral assent.
Study area
The study was carried out in four locations of Côte d'Ivoire: (i) Agboville (5°55′41″N latitude, 4°13′01″W longitude) and (ii) Adzopé (6°06′25″N, 3°51′36″W) in the south-eastern part of the country; (iii) Sikensi (5°40′34″N, 4°34′33″W) in the south-central part; and (iv) Duekoué (6°44′00″N, 7°21′00″W) in the western part. The study was integrated into a cross-sectional survey determining the prevalence of Schistosoma infection among school-aged children (Angora et al., Reference Angora, Boissier, Menan, Rey, Tuo, Touré, Coulibaly, Méité, Raso, N'Goran, Utzinger and Balmer2019). The four locations are well known for their high endemicity of S. haematobium (N'Guessan et al., Reference N'Guessan, Acka, Utzinger and N'Goran2007) and S. mansoni (Raso et al., Reference Raso, Matthys, N'Goran, Tanner, Vounatsou and Utzinger2005). Figure 1 shows the study area.

Fig. 1. Map of the four study locations in Côte d'Ivoire (Adzopé, Agboville, Duekoué and Sikensi) showing the distribution and the proportion of Schistosoma haematobium cox1 or a Schistosoma bovis cox1 genetic profile. The cox1 profile of each miracidium was identified using a rapid diagnostic (RD) multiplex PCR.
Collection of miracidia
From January to April 2018, a total of 1187 children aged 5–14 years from the four locations (Agboville, n = 402; Adzopé, n = 208; Sikensi, n = 205; and Duekoué, n = 372) were invited to provide a mid-day urine sample. Urine samples were transferred to the nearby health centres for parasitological examination. Schistosoma haematobium infection was identified by urine filtration (Mott et al., Reference Mott, Baltes, Bambagha and Baldassini1982). Ten millilitres of vigorously shaken urine was filtered through a Nytrel filter with a 40 µm mesh size and examined under a microscope by experienced laboratory technicians for S. haematobium egg detection. The presence of eggs was recorded, but infection intensities were not determined.
Urine samples from 19 randomly selected infected children were chosen for further analysis in the four locations: Sikensi (n = 6), Agboville (n = 5), Duekoué (n = 5) and Adzopé (n = 3) (Table 1). Under a dissecting microscope, eggs were removed from each urine sample with an elongated Pasteur pipette and placed in a petri dish filled with tap water to facilitate miracidial hatching. Miracidia were collected individually in 3 µL of water using a micropipette and preserved on Whatman-FTA® cards (GE Healthcare Life Sciences; Amersham, UK), as described previously (Webster et al., Reference Webster, Emery, Webster, Gouvras, Garba, Diaw, Seye, Tchuem-Tchuente, Simoonga, Mwanga, Lange, Kariuki, Mohammed, Stothard and Rollinson2012; Boissier et al., Reference Boissier, Grech-Angelini, Webster, Allienne, Huyse, Mas-Coma, Toulza, Barré-Cardi, Rollinson, Kincaid-Smith, Oleaga, Galinier, Foata, Rognon, Berry, Mouahid, Henneron, Moné, Noel and Mitta2016). All samples were transferred to the University of Perpignan in France for molecular analysis.
Table 1. Results of cox1-based rapid diagnostic (RD) PCR analysis of all miracidia collected per patient and of subsequent sequence analysis of a subsample
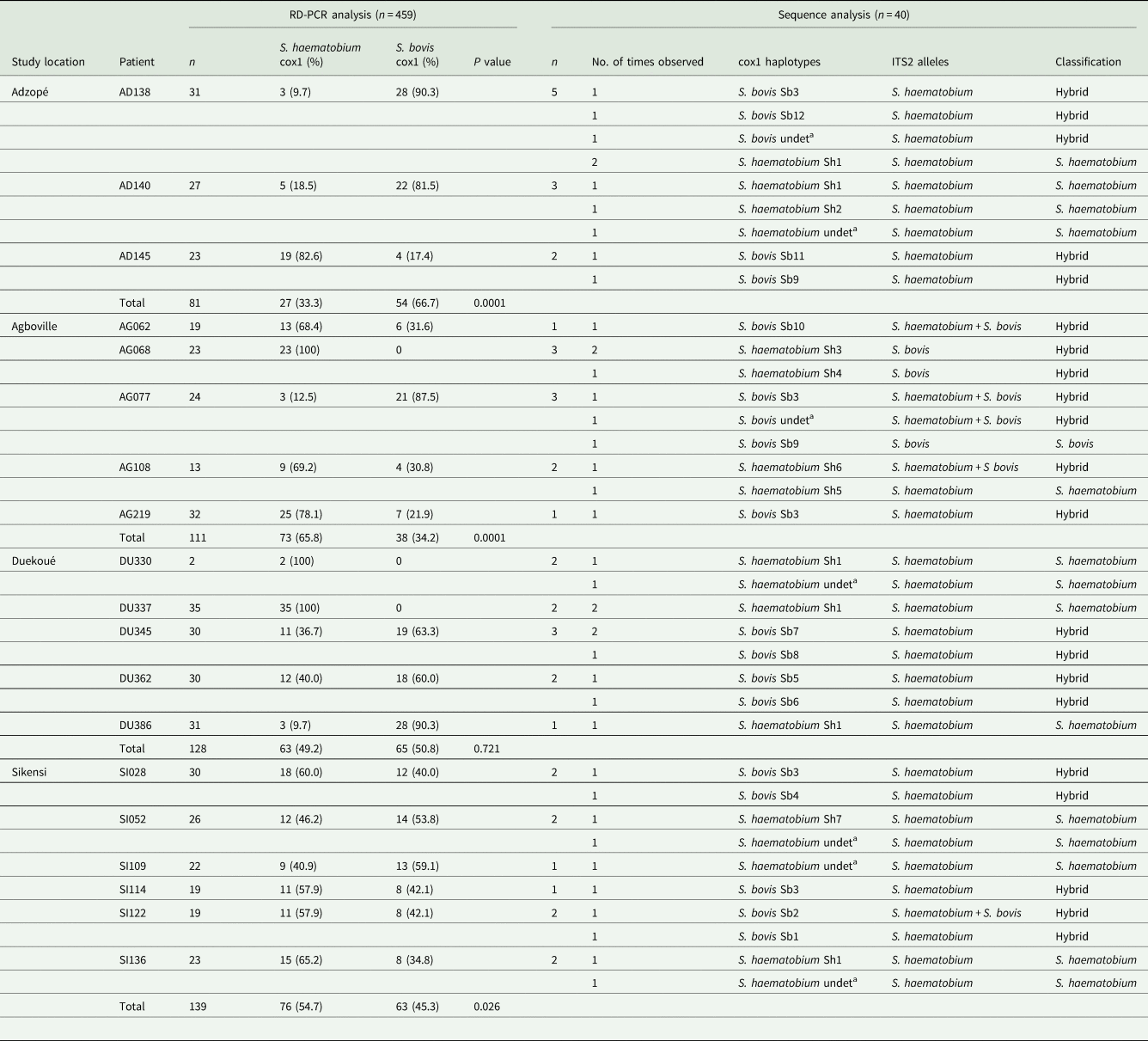
For RD-PCR analysis, the total number of miracidia, the number (and percentage) determined as Schistosoma haematobium cox1 and S. bovis cox1 and the level of significance of differences between parasites per study area using Fisher's exact test are shown. For sequencing, 10 miracidia were randomly selected (five S. haematobium and five S. bovis) per study area, based on the mitochondrial-cox1 RD-PCR results. For sequence analysis, number analysed (n) and the times different combinations of cox1 haplotype and ITS2 alleles found are given together with the resulting species classification.
Cox1, cytochrome oxidase subunit I gene; ITS, internal transcribed spacer region.
a undet., sequences for which the exact haplotype could not be determined due to sequence quality.
P value < 0.05 was considered significant.
Genomic DNA extraction
Genomic DNA was extracted individually from 503 miracidia. A 2.0 mm disc containing the sample was removed from the FTA card with a Harris-Micro-Punch (VWR; London, UK) and incubated in 50 µL of double-distilled water for 10 min. Water was removed and the disc incubated in 80 µL of 5% Chelex® (Bio-Rad; Hercules, California, USA) solution successively at 65 °C for 30 min and then 99 °C for 8 min. Finally, 60 µL of the supernatant was stored at −20 °C for subsequent molecular analysis.
Mitochondrial cox1 profiling
DNA from each miracidia was analysed using a new rapid diagnostic mitochondrial cox1 rapid diagnostic polymerase chain reaction (RD-PCR) in order to infer mitochondrial species designation. We used species-specific primers to amplify a specific cox1 DNA region (differing in length) for S. bovis (260 bp), S. mansoni (215 bp) and S. haematobium (120 bp). Primers employed were a universal reverse (Shmb.R: 5′-CAA GTA TCA TGA AAY ART ATR TCT AA -3′) and three species-specific forward primers (Sb.F: 5′-GTT TAG GTA GTG TAG TTT GGG CTC AC-3′; Sm.F: 5′-CTT TGA TTC GTT AAC TGG AGT G-3′; and Sh.F: 5′-GGT CTC GTG TAT GAG ATC CTA TAG TTT G-3′). Each PCR was performed in a total reaction volume of 10 µL, comprising 2 µL of the DNA extract, 2 µL of Green GoTaq Flexi Buffer 5X (Promega; Madison, Wisconsin, USA), 0.6 µL of 25 mm MgCl2 (Promega), 0.2 µL of 10 mm dNTP mix (Promega), 1 µL of 10 × primer mix (4 µL of 100 µ m reverse primer, 4 µL of each 100 µ m forward primer and 84 µL of distilled water) and 1 U of GoTaq Hot Start Polymerase (Promega). The reaction conditions included an activation step of 95 °C for 3 min, followed by 45 cycles of 95 °C for 10 s, 52 °C for 30 s and 72 °C for 10 s, and a final extension at 72 °C for 2 min. The cox1-PCR products were visualized for electrophoresis on a 3% agarose gel stained with ethidium bromide (see Supplementary File 1: Fig. S1). The prevalence of schistosomes with each mitochondrial cox1 signature was computed and stratified by study location using Epi Info version 7 (Centers for Disease Control and Prevention; Georgia, Atlanta, USA). Fisher's exact test was used and a P value of 0.05 was considered statistically significant.
Cox1 and internal transcribed spacer 2 (ITS2) analysis
Based on the RD-PCR results, we randomly selected a subsample of 10 miracidia (five S. haematobium cox1 and five S. bovis cox1) per study location. We performed PCR on 40 miracidia and products were sequenced on both mitochondrial cox1 and nuclear ITS2 gene (Table 1), using the following primers: Cox1.R: 5′-TAA TGC ATM GGA AAA AAA CA-3′ and Cox1.F: 5′-TCT TTR GAT CAT AAG CG-3′ for cox1 (Lockyer et al., Reference Lockyer, Olson, Ostergaard, Rollinson, Johnston, Attwood, Southgate, Horak, Snyder, Le, Agatsuma, McManus, Carmichael, Naem and Littlewood2003) and ITS 5.R: 5′-GGA AGT AAA AGT CGT AAC AAG G-3′ and ITS4.F: 5′-TCC TCC GCT TAT TGA TAT GC-3′ for ITS2 (Barber et al., Reference Barber, Mkoji and Loker2000). The PCRs were performed in a final reaction volume of 25 µL, comprising 4 µL of DNA template, 5 µL of 5X Colorless GoTaq® Flexi Buffer (Promega), 1.5 µL of MgCl2 (25 mm), 0.5 µL of dNTP (10 mm), 0.8 µL of each 10 µ m primer and 0.2 µL of Go Taq®G2 Hot Start Polymerase (Promega). The PCR conditions were the same for both markers: 3 min at 95 °C, followed by 45 cycles at 95 °C for 40 s, 48 °C for 40 s and 72 °C for 70 s, followed by a final extension of 2 min at 72 °C. The mitochondrial cox1 and nuclear ITS2 PCR products (4 µL) were visualized on 1.5% agarose gels stained with ethidium bromide to verify band size (expected size 1200 bp) and quality of the amplicons. All successfully amplified PCR products were purified and sequenced with the Cox1.R: 5′-TAA TGC ATM GGA AAA AAA CA-3′ or the ITS4.F: 5′-TCC TCC GCT TAT TGA TAT GC-3′ primers, respectively, on an Applied Biosystems Genetic Analyser at Genoscreen (Lille, France).
Sequences analysis
The partial cox1 and ITS2 sequences were assembled separately and edited using Sequencher version 4.5 (Gene Codes Corporation; Ann Arbor, Michigan, USA). All sequences were aligned using BioEdit version 7.0.9 (Ibis Therapeutic; Carlsbad, California, USA) and compared to sequences deposited in the GenBank Nucleotide Database (https://www.ncbi.nlm.nih.gov/nucleotide/). The nuclear-ITS2 region differs at five polymorphic sites between S. haematobium and S. bovis, and hence, the sequence chromatograms were checked at these mutation points to identify possible heterogeneity, as previously described (Webster et al., Reference Webster, Diaw, Seye, Webster and Rollinson2013). The mitochondrial-cox1 haplotype and nucleotide diversity [±standard deviation (s.d.)] were calculated using DnaSP version 6.0 (Rozas et al., Reference Rozas, Ferrer-Mata, Sánchez-DelBarrio, Guirao-Rico, Librado, Ramos-Onsins and Sánchez-Gracia2017). Phylogenetic trees were constructed separately for S. haematobium and S. bovis cox1 haplotypes using MEGA version 6.0.6 (Penn State University; Philadelphia, Pennsylvania, USA) and employing a maximum likelihood and the HKY + G nucleotide substitution model, which was determined by MEGA version 6.0.6 as the model best describing the data. The support for tree nodes was calculated with 1000 bootstrap iterations. The phylogenies include all the haplotypes identified in this study plus reference haplotypes obtained from GenBank Nucleotide Database. The phylogeny of the S. bovis cox1 data was rooted with an S. haematobium haplotype (JQ397330.1) and the S. haematobium cox1 data with an S. bovis haplotype (AJ519521.1). All cox1 sequences were uploaded onto the GenBank Nucleotide Database (GenBank accession nos. MK757162–MK757168 for S. haematobium and MK757170–MK757181 for S. bovis).
Results
Cox1 rapid diagnostic PCR
Of the 1187 urine samples examined, 166 (14.0%) were found positive for Schistosoma eggs, as described elsewhere (Angora et al., Reference Angora, Boissier, Menan, Rey, Tuo, Touré, Coulibaly, Méité, Raso, N'Goran, Utzinger and Balmer2019). Overall, 503 miracidia were collected from 19 Schistosoma-infected children and stored on Whatman® FTA cards and the cox1 RD-PCR was successful for 459 miracidia. Of these, 239 miracidia (52.1%) gave an S. haematobium cox1 profile and 220 (47.9%) an S. bovis cox1 profile, with no statistically significant difference between the two proportions (P = 0.081). No miracidia gave an S. mansoni cox1 profile. The S. haematobium cox1:S. bovis cox1 ratio varied according to study area (76:63 for Sikensi; 73:38 for Agboville; 63:65 for Duekoué; and 27:54 for Adzopé). The proportion of S. haematobium cox1 was higher than that of S. bovis cox1 in Sikensi (P = 0.026) and Agboville (P < 0.001), whereas S. bovis cox1 was the predominant species found in Adzopé (P < 0.001). In Duekoué, S. haematobium cox1 and S. bovis cox1 were equally distributed (Fig. 1). Table 1 shows for each parasite infra-population, the proportion of S. haematobium cox1 and S. bovis cox1 from the 459 cox1 RD-PCR miracidia.
Cox1 and ITS2 sequence analysis
The cox1 and ITS amplicons of a total of 40 miracidia were sequenced. Good quality ITS2 sequences were obtained for all samples while, for cox1, only 33 samples yielded good sequences. Among the 33 cox1 sequences, we identified 15 S. haematobium sequences (Table 1) belonging to seven distinct haplotypes with a very low diversity showing few single nucleotide polymorphisms (Supplementary File 2: Table S2) and 18 S. bovis sequences belonging to 12 distinct haplotypes (Supplementary File 3: Table S3). Haplotype diversity (±s.d.) was 0.922 ± 0.051 and 0.724 ± 0.121 for S. bovis and S. haematobium, respectively. Nucleotide diversity (±s.d.) was 0.0094 ± 0.0013 and 0.0011 ± 0.0003 for S. bovis and S. haematobium, respectively. Among the ITS2 sequences, 31 gave an S. haematobium profile and four an S. bovis profile. Five miracidia gave double chromatogram peaks at the polymorphic positions between S. haematobium and S. bovis, suggesting heterozygosity (Huyse et al., Reference Huyse, Webster, Geldof, Stothard, Diaw, Polman and Rollinson2009; Webster et al., Reference Webster, Diaw, Seye, Webster and Rollinson2013).
Miracidia identified as hybrids and non-hybrids
Discordance in the cox1 and ITS2 profiles showed that S. haematobium-bovis miracidia were present in all the four study locations and were excreted by 12 children. Among these 12 children, nine excreted S. haematobium-bovis hybrids and seven excreted pure S. haematobium. Two children excreted both S. haematobium-bovis hybrids and pure S. haematobium miracidia, while one child excreted both S. haematobium-bovis hybrids and pure S. bovis miracidia (Table 1). From all the schistosome miracidia sequenced, 16 were S. haematobium, one was S. bovis and 23 were hybrids (57.5%). The different hybrid profiles according to the discordance in the cox1 (S. haematobium or S. bovis) and ITS2 (S. haematobium or S. bovis) profiles are presented in Table 1. Most of the hybrid profiles found were S. bovis cox1 × S. haematobium ITS2. Hybrids occurred at similar frequencies in all age classes of the children included in the study.
Cox1 phylogenies
Figures 2 and 3 show phylogenies of all S. haematobium and S. bovis cox1 haplotypes, respectively. Note that S. bovis reference sequences used were obtained from animals. All S. haematobium cox1 haplotypes from Côte d'Ivoire cluster with group 1, as defined by Webster et al. (Reference Webster, Emery, Webster, Gouvras, Garba, Diaw, Seye, Tchuem-Tchuente, Simoonga, Mwanga, Lange, Kariuki, Mohammed, Stothard and Rollinson2012). Cox1 S. bovis haplotypes were split into two clusters separating those from Duekoué (Sb5–8), from those from the three remaining locations (Sb1–4 and Sb9–12).

Fig. 2. Majority rule consensus tree from maximum likelihood analysis of the mitochondrial cox1 sequences for Schistosoma haematobium haplotypes Sh1–Sh7, including data from Genbank. Groups 1 and 2 indicate the major clades defined by Webster et al. (Reference Webster, Emery, Webster, Gouvras, Garba, Diaw, Seye, Tchuem-Tchuente, Simoonga, Mwanga, Lange, Kariuki, Mohammed, Stothard and Rollinson2012). Clade support values for each node are maximum parsimony bootstrap percentages.

Fig. 3. Majority rule consensus tree from maximum likelihood analysis the mitochondrial DNA cox1 sequences for Schistosoma bovis haplotypes Sb1–Sb12 and data from GenBank. There are two clusters of S. bovis haplotypes: Duekoué (Sb5–Sb8) and the three other study sites (Sb1–Sb4 and Sb9–Sb12). Clade support values for each node are maximum parsimony bootstrap percentages. Schistosoma bovis reference sequences are from schistosomes collected from bovines.
Discussion
Hybridization of certain parasites is an emerging public health concern at the interface of infectious disease biology and evolution (King et al., Reference King, Stelkens, Webster, Smith and Brockhurst2015). From a population of 459 miracidia obtained from schoolchildren in Côte d'Ivoire, we have identified 47.9% and 57.5% of S. haematobium-bovis hybrids using the diagnostic mitochondrial cox1 analysis and by sequencing of the cox1 and ITS regions, respectively. The analysis of partial mitochondrial cox1 regions showed seven haplotypes for S. haematobium and 12 for S. bovis, which demonstrates the existence of a mitochondrial introgressive hybridization of S. haematobium cox1 by S. bovis. Similar results have been reported in Corsica (Moné et al., Reference Moné, Holtfreter, Allienne, Mintsa-Nguéma, Ibikounlé, Boissier, Berry, Mitta, Richter and Mouahid2015; Boissier et al., Reference Boissier, Grech-Angelini, Webster, Allienne, Huyse, Mas-Coma, Toulza, Barré-Cardi, Rollinson, Kincaid-Smith, Oleaga, Galinier, Foata, Rognon, Berry, Mouahid, Henneron, Moné, Noel and Mitta2016). Our findings were corroborated by analysis of the nuclear ITS2 region.
The polymorphism analysis of the cox1 gene shows that S. bovis is more polymorphic than S. haematobium. This result is consistent with a recent microsatellite-based population genetic study in Cameroon, which reported higher gene diversity and higher allelic diversity for S. bovis compared to S. haematobium (Djuikwo-Teukeng et al., Reference Djuikwo-Teukeng, Kouam Simo, Allienne, Rey, Njayou Ngapagna, Tchuem-Tchuenté and Boissier2019). Of note, the cited Cameroon study compared the diversity of S. bovis to previous data for S. haematobium obtained from Niger and Zanzibar (Webster et al., Reference Webster, Rabone, Pennance, Emery, Allan, Gouvras, Knopp, Garba, Hamidou, Mohammed, Ame, Rollinson and Webster2015). The low polymorphism of S. haematobium cox1 is in line with previous studies (Webster et al., Reference Webster, Emery, Webster, Gouvras, Garba, Diaw, Seye, Tchuem-Tchuente, Simoonga, Mwanga, Lange, Kariuki, Mohammed, Stothard and Rollinson2012, Reference Webster, Diaw, Seye, Webster and Rollinson2013; Gower et al., Reference Gower, Gouvras, Lamberton, Deol, Shrivastava, Mutombo, Mbuh, Norton, Webster, Stothard, Garba, Lamine, Kariuki, Lange, Mkoji, Kabatereine, Gabrielli, Rudge, Fenwick, Sacko, Dembelé, Lwambo, Tchuem Tchuenté, Rollinson and Webster2013). Results obtained at a regional scale (i.e. in different countries) corroborate our results from a finer spatial scale (i.e. four sites within a single country).
Schistosoma haematobium is known to be weakly structured (Webster et al., Reference Webster, Emery, Webster, Gouvras, Garba, Diaw, Seye, Tchuem-Tchuente, Simoonga, Mwanga, Lange, Kariuki, Mohammed, Stothard and Rollinson2012). It has been shown that two groups can be identified across the parasite's range in sub-Saharan Africa: ‘group 1’ clusters parasites from mainland Africa, while ‘group 2’ clusters parasites exclusively from the Indian Ocean islands and the neighbouring African coastal regions (Webster et al., Reference Webster, Emery, Webster, Gouvras, Garba, Diaw, Seye, Tchuem-Tchuente, Simoonga, Mwanga, Lange, Kariuki, Mohammed, Stothard and Rollinson2012). As expected, S. haematobium haplotypes from our study cluster with ‘group 1’. Our study also shows that for S. bovis, there is heterogeneity in the distribution of haplotypes across the country with the haplotypes from Duekoué in the western part differentiated from those from the southern part of Côte d'Ivoire. Furthermore, the current study shows that S. haematobium-bovis hybrids occurred in schoolchildren from each of the four study locations.
No S. haematobium-bovis hybrids were identified, even though such hybrids have been shown in a migrant boy from Côte d'Ivoire upon examination in France (Le Govic et al., Reference Le Govic, Kincaid-Smith, Allienne, Rey, de Gentile and Boissier2019). Recently, it has been shown that Bulinus snails from the northern and central parts of Côte d'Ivoire were infected with S. bovis, S. haematobium and/or S. haematobium-bovis hybrids (Tian-Bi et al., Reference Tian-Bi, Webster, Konan, Allan, Diakité, Ouattara, Salia, Koné, Kakou, Rabone, Coulibaly, Knopp, Meïté, Utzinger, N'Goran and Rollinson2019). The authors showed that S. bovis was particularly prevalent in Bulinus truncatus, S. haematobium was most prevalent in B. globosus and S. haematobium-bovis hybrids infected the two Bulinus species similarly. Schistosoma bovis-infected Bulinus were predominantly found in the northern part, while S. haematobium and hybrid-infected snails were mainly found in the central part of Côte d'Ivoire. These results show the importance of snail's involvement in the transmission of S. haematobium-bovis hybrids.
Most of the hybrids in our study showed cox1 sequences from S. bovis and nuclear ITS2 sequences from S. haematobium. This type of hybrid is the most common hybrid reported, including cercariae collected from infected snails in Côte d'Ivoire (Tian-Bi et al., Reference Tian-Bi, Webster, Konan, Allan, Diakité, Ouattara, Salia, Koné, Kakou, Rabone, Coulibaly, Knopp, Meïté, Utzinger, N'Goran and Rollinson2019), miracidia collected from infected patients in Senegal (Huyse et al., Reference Huyse, Webster, Geldof, Stothard, Diaw, Polman and Rollinson2009) and miracidia collected during a recent schistosomiasis outbreak on Corsica (Boissier et al., Reference Boissier, Grech-Angelini, Webster, Allienne, Huyse, Mas-Coma, Toulza, Barré-Cardi, Rollinson, Kincaid-Smith, Oleaga, Galinier, Foata, Rognon, Berry, Mouahid, Henneron, Moné, Noel and Mitta2016).
The current study found that some children excreted both pure S. haematobium and S. haematobium-bovis hybrids, which is in line with observations from Senegal (Huyse et al., Reference Huyse, Webster, Geldof, Stothard, Diaw, Polman and Rollinson2009; Webster et al., Reference Webster, Diaw, Seye, Webster and Rollinson2013). Interestingly, we have also observed a single miracidium with a ‘pure’ S. bovis signature (S. bovis ITS2 and S. bovis cox1) in one child, suggesting that this patient may be infected with S. bovis, which is traditionally considered a parasite of bovines. Such a ‘pure’ S. bovis has been reported in eggs recovered from humans in Corsica (Boissier et al., Reference Boissier, Grech-Angelini, Webster, Allienne, Huyse, Mas-Coma, Toulza, Barré-Cardi, Rollinson, Kincaid-Smith, Oleaga, Galinier, Foata, Rognon, Berry, Mouahid, Henneron, Moné, Noel and Mitta2016). These accounts suggest that zoonotic transmission might occur. However, additional research is needed to confirm this speculation. We assume that the genome of S. bovis is strongly introgressed, and hence, it is plausible that we may have missed signatures of S. haematobium ancestry due to the standard analyses performed (Webster et al., Reference Webster, Diaw, Seye, Webster and Rollinson2013). A broader coverage of the genome would no doubt identify even more hybrids and would allow a clearer distinction between ‘pure’ parasites of each species and different levels of introgression.
Conclusion
Our study has shown that S. haematobium-bovis hybrids are common in Schistosoma egg-positive children in Côte d'Ivoire. Our observations are relevant because hybrid parasites could affect transmission dynamics, treatment efficacy and morbidity, which might jeopardize control of, and progress towards, elimination of schistosomiasis. Our findings are relevant as the presence of hybrids calls into question our present understanding of parasite transmission and host ranges, which in turn may affect the effectiveness of current control strategies. Intensive prospection of domestic and wild animals is warranted to determine whether real zoonotic transmission occurs.
Supplementary material
The supplementary material for this article can be found at https://doi.org/10.1017/S0031182019001549.
Acknowledgements
We are grateful to the district health and village authorities of the four study areas for their support and facilitating the implementation of this study. We also thank the participants (children and their parents/guardians) for their commitment throughout the study.
Author contributions
EKA, HM, OB and JB conceived and designated the study. EKA, OR and JB wrote the first draft of the manuscript. EKA and JFA performed the molecular analyses. EKA and JB performed statistical analysis. OR, AOT, JTC, GR, WY, EKN, JU and OB revised the manuscript. All authors read and approved the final manuscript prior to submission.
Financial Support
This work was financially supported by the Agence National de Recherche (ANR), which supports the Hybrid Swarm project (HySWARM) in Perpignan that is set within the frame of the ‘Laboratoire d'Excellence (LABEX)’ TULIP (ANR-10-LABX-41). Additional financial support was obtained by the Swiss National Science Foundation for EKA, JU and OB (grant no. 31003A_170113). EKA is a recipient of a Swiss Government Excellence Scholarship (ESKAS, grant no. 2017-0746) for which he is deeply grateful.
Conflict of interest
None.
Ethical standards
Ethical clearance for this study was obtained from the Ministère de la Santé et de l'Hygiène Publique de Côte d'Ivoire (reference no. 003-18/MSHP/CNER-kp). Written informed consent was obtained from the children's parents or legal guardians. Oral assent was obtained from children.