Introduction
Marine living resources along the Antarctic margin within the Convention Area are managed by CCAMLR (Commission for the Conservation of Antarctic Marine Living Resources). Conservation Measure 22-06 (CCAMLR 2009a) requires vessels fishing within the Convention Area to monitor their bycatch and notify CCAMLR when evidence for a Vulnerable Marine Ecosystem (VME) is encountered. If certain bycatch thresholds for VME indicator taxa are reached, a one nautical mile radius is declared around the site which then becomes closed to fishing (CM 22-07, CCAMLR 2009b). Providing the fishing industry with information regarding the likely distribution of certain VME taxa would allow them to focus their fishing effort in areas with less likelihood of encountering a VME.
The identification of VMEs along the Antarctic continental shelf and slope is made difficult by the sparse biological data coverage. Nevertheless, where VMEs have been identified, they provide the opportunity to define physical surrogates which may assist in predicting their broader distribution. Physical surrogates have been developed for several areas of the Antarctic shelf and successfully applied to map the distribution of benthic biota (e.g. Teixidó et al. Reference Teixidó, Garrabou and Arntz2002, Barry et al. Reference Barry, Grebmeier, Smith and Dunbar2003, Beaman & Harris Reference Beaman and Harris2005, Thrush et al. Reference Thrush, Dayton, Cattaneo-Vietti, Chiantore, Cummings, Andrew, Hawes, Kim, Kvitek and Schwarz2006, Post et al. in press). The application of physical parameters as surrogates for benthic communities has the advantage that parameters such as seafloor bathymetry and oceanographic properties can be measured relatively easily and consistently across broad areas. Physical parameters are being increasingly incorporated in marine management frameworks in many countries (Greene et al. Reference Greene, Yoklavich, Sullivan and Cailliet1995, Hockey & Branch Reference Hockey and Branch1997, Pickrill & Todd Reference Pickrill and Todd2003, Harris et al. Reference Harris, Heap, Post, Whiteway, Potter and Bradshaw2007).
In this paper we report on the distribution of species rich coral-sponge communities imaged from underwater camera transects during the Aurora Australis leg of the Collaborative East Antarctic Marine Census (CEAMARC) survey on the George V Land continental shelf and slope. This survey, between December 2007 and January 2008, focussed on benthic and demersal sampling as well as on physical and chemical oceanography. Two areas sampled during the survey containing dense coral-sponge communities have since been designated as VMEs by CCAMLR and are now closed to bottom fishing. Analysis of the physical and biological datasets collected during the survey allows us to form several hypotheses to guide our understanding of the physical controls on these coral-sponge communities, and therefore to identify more easily other areas where there is likely to be a risk of encountering such VMEs.
Setting
The George V Shelf has an average depth at the shelf break of 500 m (Fig. 1). The main bathymetric features of the shelf are the George V Basin which reaches depths of 1300 m adjacent to the Mertz Glacier Tongue, and the Adélie and Mertz banks which have average depths of c. 200 m to 250 m. The inner shelf is cut by a complex series of depressions and small glacial basins. Several large submarine canyons cut the continental slope, of which the Cuvier and Jussieu canyons reach the shelf break (Caburlotto et al. Reference Caburlotto, De Santis, Zanolla, Cemerlenghi and Dix2006).
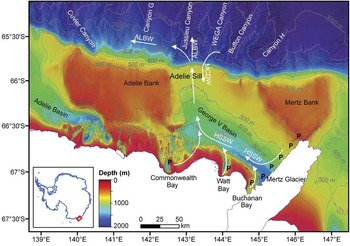
Fig. 1 Bathymetry and simplified bottom circulation over the George V Shelf. The bathymetry grid has a 250 m grid pixel resolution over the shelf (Beaman Reference Beaman2008) and a simplified oceanographic circulation is shown (Rintoul Reference Rintoul1998, Bindoff et al. Reference Bindoff, Rintoul and Massom2000a, Massom et al. Reference Massom, Hill, Lytle, Worby, Paget and Allison2001). HSSW is High Salinity Shelf Water, MCDW is Modified Circumpolar Deep Water and ALBW is Adélie Land Bottom Water. P marks the extent of the Mertz Polynya along the coast and western edge of the Mertz Ice Tongue. The inset map shows the location of the study area highlighted by the red box.
Iceberg scours on the George V Shelf have been found to depths of 500 m along the eastern flank of Mertz Bank (Barnes & Lien Reference Barnes and Lien1988). Grounded icebergs on the George V shelf are sourced from the Ross Ice Shelf, the Ninnis Glacier and the Cook Ice Shelf and advected westwards via the west wind drift (Massom Reference Massom2003). While icebergs produced by the Mertz Glacier are relatively small (maximum keel depth of 298 m) and are therefore only likely to ground on the shallowest parts of the banks, those from the Cook Ice Shelf have keel depths of up to 521 m (Dowdeswell & Bamber Reference Dowdeswell and Bamber2007) and ground readily along the margins of the Mertz and Adélie banks.
The occurrence of the Mertz polynya dominates the oceanography on the George V Shelf (Fig. 1). The polynya is sustained by strong and persistent katabatic winds that drain into Buchanan Bay, producing rapid rates of sea ice formation and removal in the near-shore (Massom et al. Reference Massom, Hill, Lytle, Worby, Paget and Allison2001). The northward extension of the Mertz Glacier Tongue, composed of grounded icebergs and fast ice, blocks the westward advection of sea ice into the polynya area. The broader extent of the polynya away from coastal bays is also influenced by the passage of synoptic-scale weather systems.
Summer upwelling of Modified Circumpolar Deep Water (MCDW) onto the shelf via the Adélie Sill (Rintoul Reference Rintoul1998, Bindoff et al. Reference Bindoff, Rintoul and Massom2000a, Williams et al. Reference Williams, Bindoff, Marsland and Rintoul2008; Fig. 1) plays an important role in the formation of Adélie Land Bottom Water (ALBW). MCDW is relatively warm, and therefore provides additional heat which promotes melting of sea ice in the polynya region, enhancing the overall size of the polynya (Rintoul Reference Rintoul1998). Furthermore, MCDW supplies additional salinity to the shelf waters. High Salinity Shelf Waters (HSSW) are formed within the polynya by brine rejection during winter sea ice production (Bindoff et al. Reference Bindoff, Rintoul and Massom2000a, Williams et al. Reference Williams, Bindoff, Marsland and Rintoul2008). Mixing between HSSW and MCDW increases the salinity of the shelf waters to the point where it is dense enough to spill over the Adélie Sill as ALBW. ALBW is found along the continental slope west of the Adélie Sill.
The ice free waters of coastal polynyas support a relatively long growing season, with phytoplankton production starting soon after the spring equinox (Sambrotto et al. Reference Sambrotto, Matsuda, Vaillancourt, Brown, Langdon, Jacobs and Measures2003). These waters therefore have high productivity compared to shelf areas with extensive sea ice cover. A study of summer microplankton on the George V Shelf found that phytoplankton biomass and diatom abundance was highest in the region directly west of Mertz Glacier, within the polynya (Beans et al. Reference Beans, Hecq, Koubbi, Vallet, Wright and Goffart2008). Enhanced productivity within the polynya is also indicated by high nitrate and silicic acid utilization and enhanced chlorophyll a measurements within Commonwealth Bay (Sambrotto et al. Reference Sambrotto, Matsuda, Vaillancourt, Brown, Langdon, Jacobs and Measures2003, Vaillancourt et al. Reference Vaillancourt, Sambrotto, Green and Matsuda2003).
Methods
Physical and biological data were collected from the George V Land continental shelf and slope during December 2007 to January 2008. In this paper we present results from analysis of underwater video and still camera transects, as well as the bathymetric model for the region and the general oceanographic circulation based on 98 CTD casts across the shelf and slope.
Detailed video and still footage was obtained along five transects in four areas of the George V Land continental slope: from east to west these are stations 33, 63, 65, 79 to 88 and 82 to 83. In addition, 22 shelf transects were also analysed. The Australian Antarctic Division (AAD) underwater video was used to image site 65, and the AAD stills cameras were used for stations 33, 79 to 88 (a single transect run between stations 79 and 88) and 82 to 83 (also a single transect run between stations 82 and 83). The Geoscience Australia (GA) underwater video was used for all the shelf transects presented, including the upper slope station 63. The AAD video and stills cameras were mounted on the beam trawl. The video camera consisted of a Sony HDR-HC73 HD 1080i system, with lighting provided by four LED lamps which were set up to provide two wide and two narrow fields of illumination. The still camera consisted of 8 megapixel Canon EOS 20D SLR with two speedlight strobes. The GA underwater video camera consisted of a forward-looking Sony DVCAM Camcorder DSRP DX10 recording to an internal Sony 6 mm DVCAM tape cassette. It was mounted on a metal frame with 2 x 250 watt lights at the front and 2 x 100 watt lights at the rear. A live image feed to the winch control room was used to assist in towing at a consistent height above the seafloor via adjustment of the deck winch.
For this study, 26 still and video camera transects were analysed to determine the dominant benthic communities and, in particular, to note occurrences of the red stylasterid hydrocoral Errina sp. (Fig. 2). The stylasterid hydrocorals are fragile organisms that are very susceptible to fishing gear impact, and have been most commonly observed within the depth range (>550 m) allowed for longline fishing in the CCAMLR Area (Parker & Bowden Reference Parker and Bowden2009). These hydrocorals have therefore been classed as key VME indicator taxa. For each camera transect, density of the benthic communities was also mapped continuously and related to water depth based on the ship’s echosounder. Density was recorded as either sparse or dense, with sparse areas relating to occasional or patchy cover, and dense areas occurring as continuous or nearly continuous cover. The most abundant taxa were also recorded in order of abundance.
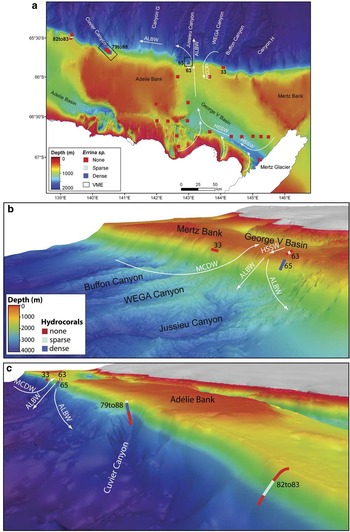
Fig. 2 Occurrence of Errina sp. hydrocoral communities along the George V Land continental slope (coloured squares) with 250 m bathymetry grid and general bottom circulation (based on Rintoul Reference Rintoul1998, Bindoff et al. Reference Bindoff, Rintoul and Massom2000a, Reference Bindoff, Rosenberg and Warner2000b) in a. plan view, b & c. 3D views along the continental slope (15x vertical exaggeration). Black outlines on (a) indicate areas proclaimed as VMEs. The smooth seafloor along the upper slope in (c) reflects the absence of multibeam bathymetry, though there are numerous ship tracks which allow definition of the shelf break.
No USBL (Ultra-Short Baseline) units were deployed on any of the camera systems, so position along the transects was determined based on the ship’s GPS. Based on the estimated position of the trawl the depth accuracy is likely to be within 10 m on the upper slope, though larger offsets will occur with increasing depth and slope. This level of uncertainty does not affect any of the conclusions that we draw regarding the overall bathymetric position and processes at the sites where dense coral-sponge communities occur.
A bathymetric model at 250 m resolution has been produced for the George V shelf and at 500 m resolution on the slope based on multibeam swath bathymetry and singlebeam data (Beaman Reference Beaman2008, Beaman et al. unpublished). The key multibeam dataset along the continental slope was derived from the Italian MOGAM PNRA survey (De Santis et al. Reference De Santis, Brancolini, Accettella, Cova, Caburlotto, Donda, Pelos, Zgur and Presti2007), while smaller areas of swath bathymetry on the shelf were derived from survey NBP0101 on RV Nathaniel B. Palmer.
Oceanographic data was collected from CTD casts during the CEAMARC survey. Bottom temperature, salinity and density data were used to define the summer water masses according to Bindoff et al. (Reference Bindoff, Rosenberg and Warner2000b). Point data for bottom salinity and temperature were interpolated via kriging to a 0.005° grid.
Results
Continental shelf and slope communities
Dense communities of stylasterid hydrocorals (Errina sp.) and demosponges (numerous species) occur at stations 65 and 79 to 88 on the continental slope (Fig. 2, Table I). The other two areas on the slope contain only sparse (station 82 to 83) or no corals (station 33), and station 63, upslope from station 65, also has only sparse hydrocoral occurrence. At all other sites analysed on the continental shelf there are no hydrocoral occurrences (Fig. 2).
Table I Summary of slope transects sampled on the George V Land continental shelf.

The mid-slope transect at station 65 crosses depths of approximately 650–950 m. Dense seabed communities occur along the entire transect, dominated by the hydrocoral Errina sp. and a diverse assemblage of demosponges (Table I). Station 63, on the upper slope, contains sparse occurrences of Errina sp. at 430 m. Along transect 79 to 88, the hydrocoral Errina sp. is present in low densities from 440–570 m in a community co-dominated by various demosponge species and soft corals. A high density coral-sponge community occurs at depths of 570–950 m along this transect with highest cover occurring on ridges. No hydrocorals were observed at depths greater than 1025 m on this transect. At station 82 to 83 sparse occurrences of the Errina sp. corals occur at depths of 600–920 m, with a small area of dense cover at 900 m. At no time is Errina sp. the dominant component of the community along transect 82 to 83, with soft corals, bryozoa and at times hexactinellids (glass sponges) the dominant taxa. The seabed communities along transect 33 (670–820 m water depth) contain no hydrocorals, with only a very sparse cover of demosponges present.
The two rich coral-sponge communities are located at the head of the Jussieu and Cuvier canyons (Figs 1 & 2). The shelf edge at the head of these two canyons is indented, as shown most clearly by the contour lines on Fig. 1, indicating that these two canyons cut the continental shelf break (Caburlotto et al. Reference Caburlotto, De Santis, Zanolla, Cemerlenghi and Dix2006). No such indentations are observed at sites 33 and 82 to 83, suggesting that canyons below these sites do not cut the shelf break.
Oceanographic patterns
Analysis of CTD casts indicate that the continental shelf is bathed by relatively warm (>-1.0°C, potential temperature) and highly saline (>34.59 psu) waters, typical of Modified Circumpolar Deep Water (MCDW; Fig. 3) (Bindoff et al. Reference Bindoff, Rintoul and Massom2000a, Reference Bindoff, Rosenberg and Warner2000b). This water mass has been found previously to have significant shelf penetration in the region of the sill at the head of the Jussieu Canyon (143°E; Bindoff et al. Reference Bindoff, Rintoul and Massom2000a) (Fig. 2). Along the slope, salinity measurements are relatively high at stations 65, 82 to 83 and 79 to 88, with much lower salinity at station 33 (Fig. 3). This pattern is consistent with the presence of ALBW along the continental slope at sites west of the sill, as found also in previous studies (Rintoul Reference Rintoul1998, Bindoff et al. Reference Bindoff, Williams and Allison2001).

Fig. 3 Interpolated salinity (based on kriging) across George V Land continental shelf and slope based on summer CTD casts (white dots). High salinity occurs across transects 65, 79 to 88 and 82 to 83, reflecting the influence of ALBW, while transect 33 has relatively low salinity (average salinity values along transects shown in brackets).
Discussion
Underwater video and still footage analysed along four transects on the George V continental slope reveals contrasting benthic communities, particularly in the occurrence of the stylastrid hydrocoral Errina sp. The distribution and abundance of this taxa on the continental slope appears to be related to differences in oceanographic and bathymetric properties. While it is possible that we have not fully captured the distribution of stylastrid hydrocorals with the transects imaged on the George V margin, we use the data available to form some hypotheses about the physical factors which may influence their distribution, providing a framework for broader testing of these associations along the Antarctic continental shelf and slope.
Video and still camera transects at sites 79 to 88 and 65 reveal dense communities dominated by the stylasterid hydrocoral Errina sp. and a diverse assemblage of demosponges in water depths of 570–950 m. The other sites exhibit only sparse (transect 82 to 83 and 63) or no (transect 33) hydrocoral communities. The sparse occurrence of hydrocorals on transect 63 reflects the relatively shallow water depths, reaching a maximum depth of 430 m, which is shallower than the occurrence of dense communities on the other two transects. The sparse occurrence of hydrocoral communities at depths shallower than 570 m on the George V shelf probably reflects their slow recovery to disturbance from iceberg scouring, given probable iceberg keel depths of up to 520 m in this region (Dowdeswell & Bamber Reference Dowdeswell and Bamber2007).
Transects 82 to 83 and 33 exhibited only sparse or no hydrocorals, yet encompassed water depths well below the depth of iceberg scouring (400–1500 m and 670–820 m, respectively), and are within the depth range where dense hydrocoral communities occur on other transects. Comparison of additional physical attributes between these sites provides a better understanding of the factors which may control the distribution of Errina sp. dominated communities on the Antarctic margin.
ALBW probably entrains nutrients and organic matter from the productive shelf area of the Mertz polynya, which may thereby sustain higher productivity in bottom communities bathed by ALBW. Evidence for the export of biogenic siliceous sediment off the shelf via ALBW is supported by sedimentological studies linking sediment deposition and bottom water production through the Holocene (Harris et al. Reference Harris, Brancolini, Armand, Busetti, Beaman, Giorgetti, Presti and Trincardi2001). Site 65 sits at the head of the Jussieu Canyon, the main conduit for ALBW exiting the shelf (Bindoff et al. Reference Bindoff, Rintoul and Massom2000a), and continental slope sites to the west also have water properties indicative of ALBW (Rintoul Reference Rintoul1998, Bindoff et al. Reference Bindoff, Rosenberg and Warner2000b). At site 33, to the east, bottom salinity is relatively low, consistent with the presence of Ross Sea Bottom Water on this part of the slope (Bindoff et al. Reference Bindoff, Rintoul and Massom2000a) (Fig. 3). The absence of corals at Site 33 is probably associated with lower food supply due to the absence of ALBW.
The other distinction between the coral and non-coral dominated sites is their location in relation to bottom topography. The two coral dominated transects (65 and 79 to 88) are located on canyons that cut the shelf break while the other two sites (82 to 83 and 33) are located in areas where the canyons do not appear to breach the continental shelf edge. Canyons that cut the shelf break have been shown to be effective conduits for phytoplankton and other detritus produced in productive shelf waters, with the material distributed throughout the canyon system by strong tidal and gravity currents (Vetter & Dayton Reference Vetter and Dayton1999). Increased turbulence within ∼100 m of the canyon bottom resuspends particles and slows deposition, creating relatively high particulate and nutrient content in the water column (Bosley et al. Reference Bosley, Lavelle, Brodeur, Wakefield, Emmett, Baker and Rehmke2004). Submarine canyons are therefore associated with an enhanced food supply able to sustain high densities of filter feeders (e.g. anemones, corals and sponges). The abundance of these taxa within canyons has also been associated with the stronger currents and greater availability of hard substrates available within canyon systems (Hecker et al. Reference Hecker, Logan, Gandarillas and Gibson1983). Hydrocoral communities sampled in the Ross Sea have also been shown to be located in areas of high current flow on the shelf break and slope (Parker & Bowden Reference Parker and Bowden2009).
Conclusions
Dense communities dominated by the hydrocoral Errina sp. and a diverse assemblage of demosponge species have been found at depths ranging from 570–950 m on the George V Land continental slope. We propose that these communities are sustained by three key factors: 1) their location below the depth of maximum iceberg scouring, 2) the presence of Adélie Land Bottom Water rich in organic matter which provides a food supply for the organisms, 3) their location at the head of shelf cutting canyons which promotes current flow and provides hard substrates for the organisms to attach to. These relationships should be tested in other areas, such as the Ross Sea, the western Weddell Sea and Prydz Bay where the seafloor bathymetry and oceanography is well-defined and there are already some observations for the occurrence of stylasterid hydrocorals. Such testing would clarify whether the factors determined in this study are important only in combination, as is implied by this study, or whether any one of these factors can be related to hydrocoral growth independently. Refining the associations between these taxa and physical parameters will allow better prediction of the distribution of similar VMEs around the Antarctic margin, and more accurate assessment of the likelihood of encountering these communities.
Acknowledgements
We would like to thank Captain Ian Moodie, the crew and scientific party aboard Aurora Australis survey V3 who undertook the sampling for the CEAMARC program, with special thanks to voyage leader Dr Graham Hosie (Australian Antarctic Division). An earlier copy of this manuscript was improved by critical review by Drs Matthew McArthur and Scott Nicol (Geoscience Australia). We also thank Dr David Bowden (NIWA) and an anonymous reviewer for their constructive comments. The work is published with the permission of the Chief Executive Officer, Geoscience Australia.