Introduction
The Eustachian tube is central to middle-ear health. One of its functions is to equalise negative middle-ear pressure resulting from air absorption. Transient openings achieved during swallowing or other jaw movement can restore middle-ear pressure. However, it is challenging to equalise middle-ear pressure growths when middle-ear pressure is significantly lower than external pressure, as occurs during scuba diving, when descending in an aircraft or during a middle-ear infection. In these instances, the Eustachian tube may not open and middle-ear ventilation might fail.
Trials in rats suggested that topical betahistine can improve Eustachian tube opening, perhaps by decreasing the surface tension of fluid in the lumen and making opening easier.Reference Franz, Shafton and Anderson1 Betahistine is a histamine H3 receptor antagonist used to treat Ménière's disease in humans.Reference Lacour and Sterkers2 Betahistine can pre-junctionally inhibit noradrenaline release from sympathetic post-ganglionic neurons,Reference Silver, Poonwasi, Seyedi, Wilson, Lovenberg and Levi3 favouring vasodilation. Post-ganglionic sympathetic nerve terminals in the vicinity of the Eustachian tube epithelium control blood vessel diameter. It has been hypothesised that inducing vasodilation with betahistine may indirectly alter secretion from the Eustachian tube epithelium and favour easier opening of the tube, as a result of changes in the surface tension of the fluid coating the epithelium.Reference Franz, Shafton and Anderson1
Our goal was to test topical betahistine on Eustachian tube function in humans. Assessment of Eustachian tube function has been difficult.Reference Martino, Di Thaden, Krombach and Westhofen4 Existing tests, such as the Valsalva and Toynbee manoeuvres, or use of the Politzer bag, are only qualitative. While these manoeuvres may help to open the Eustachian tube and ventilate the middle ear, or give some indication of its patency, they are not suited for a quantitative assessment of Eustachian tube function.
More reliable tests of quantitative Eustachian tube function are obtained in a pressure chamber, where the ability of the Eustachian tube to equalise against known pressure gradients can be evaluated using an impedance bridge, as developed by Münker.Reference Münker5 In this approach, a probe with three boreholes is inserted into the ear canal. One borehole delivers a sound (the volume velocity required for tympanometry) and another monitors sound pressure with a microphone. A third borehole is left open to allow pressure in the external ear canal to correspond to the pressure in the chamber (equivalent to the static pressure provided by a clinical tympanometer). This permits continuous recording of the acoustic impedance while the chamber pressure is simultaneously changed.
Since the work of Münker, other workers have used pressure chambers to evaluate Eustachian tube function, and particularly measured hearing levels as a means to assess Eustachian tube function. Kitahara et al.Reference Kitahara, Kodama, Ozawa, Izukura and Inoue6 measured the static and dynamic capacity of the Eustachian tube to restore hearing in normal subjects in a sound proof pressure chamber. Kodama et al.Reference Kodama, Kitahara, Ozawa and Izukura7 evaluated Eustachian tube function in abnormal subjects employing the same technique.
In this study, rather than assessing the hearing level, we employed tympanometry to assess Eustachian tube function in a pressure chamber. We used the pressure chamber to generate both negative and positive middle-ear pressures, and tested the subject's ability to equalise middle-ear pressure both actively (by swallowing with a negative middle-ear pressure) and passively (by recording spontaneous openings of the Eustachian tube when the middle-ear pressure is positive relative to ambient pressure). These experiments were performed initially without betahistine and then repeated after the topical application of betahistine in the nasopharynx.
Materials and methods
Screening
The subjects were aged 18–50 years (average age, 34.7 years), with a male to female ratio of 1:1.6. Inclusion criteria included a history of problems clearing ears or a history of allergic rhinitis. Exclusion criteria included evidence of tympanic scarring, hearing or balance deficit, a history of ear infections, or current upper airways infection.
Twenty-four subjects commenced the trial. One subject withdrew, and the recordings of an additional four subjects were discounted because technical failures reduced the number of usable runs to below 75 per cent of the possible data. These individuals were replaced in the trial by five reserve candidates.
A 10-person commercial hyperbaric chamber (Wesley Centre for Hyperbaric Medicine, Brisbane, Australia) was used. The chamber was ventilated with air. Increases of chamber pressure were at 12–18 kPa per minute, while decreases in chamber pressure were at 5 kPa per minute. The pressure drop was linear and managed manually, and proved highly accurate. The pressure step from +10 kPa to ambient took 2 minutes, plus or minus 3 seconds, with a constant linear decrease of 5 kPa per minute. All pressure changes were confirmed independently within the chamber using a digital data logger (RHTemp 101A; Madgetech, Warner, New Hampshire, USA).
Ear drum stiffness was measured using a custom impedance bridge and software written in LabView 7.0. A small speaker was used to inject a volume-velocity stimulus (400/440 Hz) into the ear canal, with ear canal sound pressure monitored by a small electret microphone. Both of the flexible 1 mm probe tubes going into and out of the ear canal were passed through a standard flexible ear tip, and were of sufficiently high impedance that the ear canal was not loaded acoustically by the speaker and microphone. The ear tip partially filled the external ear canal, but did not seal it against environmental pressure changes, because it included a third high-impedance acoustic pathway to allow pressure equalisation.
Middle-ear pressure was recorded at intervals (see below for details) using an MT-10 portable tympanometer (Interacoustics, Middelfart, Denmark).
The authors assert that all procedures contributing to this work complied with the National Statement on Ethical Conduct in Human Research (2007) of the National Health and Medical Research Council of Australia. The procedures were approved by the UnitingCare Health Human Research Ethics Committee and were in accordance with the Helsinki Declaration of 1975, as revised in 2008.
Protocol
Up to four subjects were present in the chamber during each trial. The protocol followed is shown in Table 1.
Table 1. Hyperbaric chamber protocol
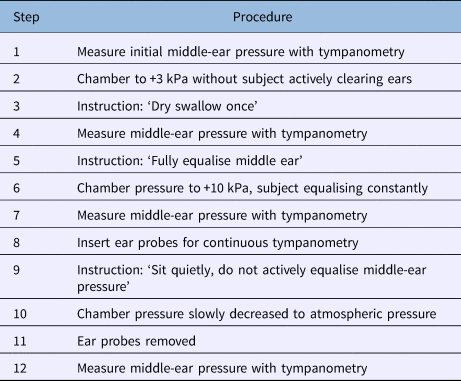
Test of active Eustachian tube function
The chamber pressure was initially increased from ambient to +3 kPa, with the subject instructed not to clear their ears. This resulted in a middle-ear pressure that was negative relative to the chamber pressure. The subject was then instructed to swallow once. Hence, the middle-ear pressure after the dry swallow was a measure of active equalisation against a negative pressure in the middle ear. Swallowing results in a complex interplay of the tensor veli palatini and levator veli palatini muscles that connect to the soft palate and/or the Eustachian tube. The contraction of these muscles acts to decrease the closing force on the Eustachian tube, and favours equalisation of air pressure between the pharynx and the middle ear.
Middle-ear pressure was assessed with a portable tympanometer. Readings were taken in the chamber initially, while at ambient pressure, at +3 kPa prior to the swallow and at +3 kPa after the swallow.
Test of passive middle-ear pressure equalisation
Next, the chamber pressure was increased from +3 kPa to +10 kPa over 35–45 seconds, and the subject was instructed to freely equalise middle-ear pressure to remain comfortable. The intention was to have the middle-ear pressure equal to chamber pressure at +10 kPa. Following this, the chamber pressure was slowly decreased to ambient; this took 2 minutes, plus or minus 3 seconds, with a constant linear decrease of 5 kPa per minute. The subject was instructed not to actively equalise by swallowing or other means. As a consequence, the decrease in chamber pressure, without any attempt of the subject to equalise, resulted in a relative positive pressure appearing in the middle ear. Any subsequent openings of the middle ear were, hence, purely passive, caused by the increasing pressure in the middle ear forcing air out through the Eustachian tube, rather than any activity of the soft palate and levator and tensor veli palatini muscles.
Changes in middle-ear pressure were measured with the portable tympanometer at the following time points: after the increase in chamber pressure to +10 kPa, when the middle-ear pressure was expected to be equal to chamber pressure due to active equalisation by the subject, and when the chamber pressure had returned to ambient.
After the tympanometer reading at +10 kPa, the impedance bridge was fitted into each external auditory canal and used to monitor spontaneous openings of the Eustachian tube. Openings appeared as sudden relaxations in the stiffness of the ear drum. The impedance bridge was removed when the chamber pressure was at ambient, to allow the final tympanometer reading.
The above sequence of pressure changes (ambient to +3 kPa, +3 kPa to + 10 kPa, +10 kPa to ambient) was one cycle (Figure 1). The cycle was repeated seven times for each subject prior to betahistine or vehicle treatment, and seven times after betahistine or vehicle treatment. Each cycle took around 15 minutes, and there was no delay between successive cycles, except after treatment with the drug or vehicle (following cycle seven) – the next cycle was not commenced for 30 minutes. The entire series (control and drug treatment) took approximately 4 hours to complete for each subject. The period available to assess the effect of betahistine (2 hours) was sufficient to evaluate the time course and maintenance of any effect.
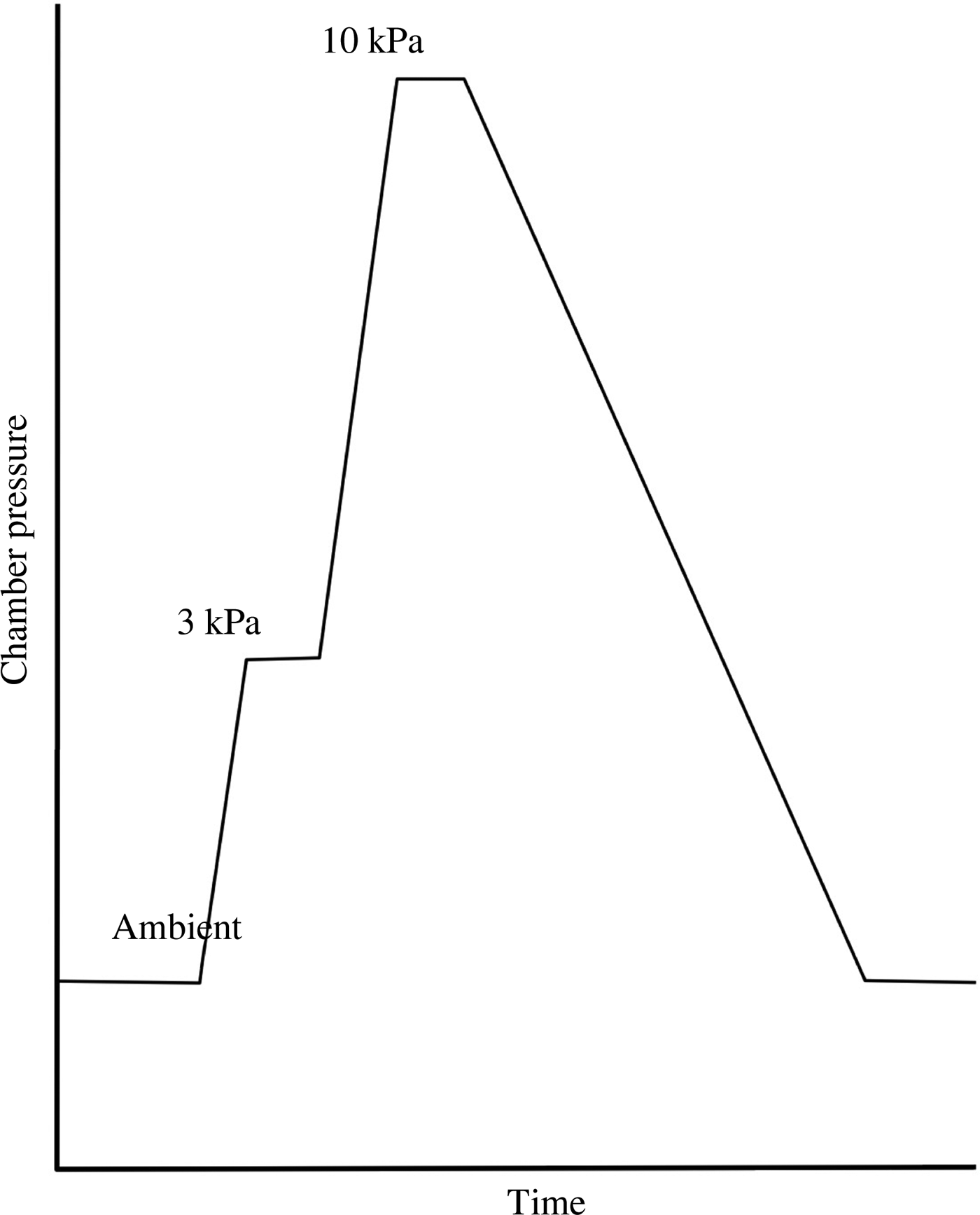
Fig. 1. Pressure change within the chamber for one cycle. Note that pressure increases and decreases were at a constant rate. The time spent at +3 kPa and +10 kPa varied slightly as various parameters were measured.
Drug treatment
After seven control runs, 24 subjects were assigned randomly to one of three equal-sized groups. They were then treated intranasally with an aerosol to expose the medial end of the Eustachian tube to either vehicle (placebo), or with a low dose or high dose of betahistine dihydrochloride. The chamber operators and clinical trials nurses were blind to the doses used. Vehicle constitution was as shown in Table 2.
Table 2. Vehicle for betahistine dihydrochloride
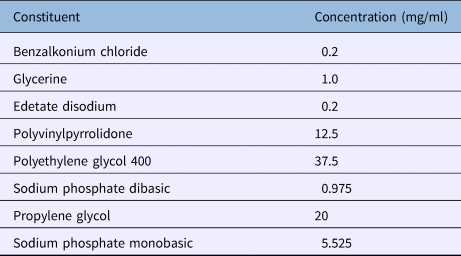
pH adjusted to 5.5
A commercial nasal spray applicator was used to administer 2 × 100 µl doses to each nostril; the applicator was aimed parallel to the floor of the nasal cavity to strike the opening of the Eustachian tube. Application was made with the subject supine. The total dose applied to the nasal cavity was 40 mg (high dose group) or 4 mg (low dose group). Thirty minutes later, the subject was taken through seven further runs (treatment) in the hyperbaric chamber, as previously described.
Following the completion of the trials, the stiffness measurements and tympanometry readings were analysed blind, prior to decoding. The parameters measured (control vs treatment) for each of the three groups (placebo, low dose, high dose) included: the effectiveness of a single swallow in actively ventilating the middle ear at +3 kPa, the total passive openings during the pressure drop from +10 kPa, the total open time of the Eustachian tube during the pressure drop from +10 kPa, and the residual pressure in the middle ear after attempting to equalise at +10 kPa.
In each case, the seven control runs were compared to the seven treatment runs using a paired t-test, with alpha = 0.05. The specific parameters compared were: the mean number of openings across the seven runs, the total time the Eustachian tubes were open over the seven runs, and the difference between the middle-ear pressure after attempting to equalise at 10 kPa and the chamber pressure.
Results
The middle-ear pressure of each subject on entering the unpressurised chamber was recorded, and thus represented the resting middle-ear pressure as a result of prior normal daytime activities. The mean starting middle-ear pressure across both ears of the 24 subjects was −0.17 kPa (standard error of the mean ± 0.32 kPa). The range was from +0.63 to −1.51 kPa, although only two individuals had positive starting middle-ear pressure, each in one ear.
Control runs
Active equalisation
The effect of a voluntary swallow at +3 kPa was assessed. Following the initial +3 kPa pressure step, the majority of subjects had a middle-ear pressure of between −2.5 and −3.0 kPa. However, in each cycle, a proportion of subjects (around 10), either inadvertently or spontaneously, partially or completely, equalised one or both of their middle ears. Of those that maintained a middle-ear pressure of at least −2.5 kPa (mean pressure, −2.78 kPa; standard error of the mean ± 0.23 kPa), a single dry swallow produced a drop in mean pressure by 1.36 kPa (standard error of the mean ± 1.42 kPa).
Passive equalisation
The effect of a pressure decrease from +10 kPa was assessed. During the pressure decrease from +10 kPa to ambient pressure, across all seven control runs for each subject, the behaviour of individual Eustachian tubes in each run fell into one of four patterns (Figure 2).
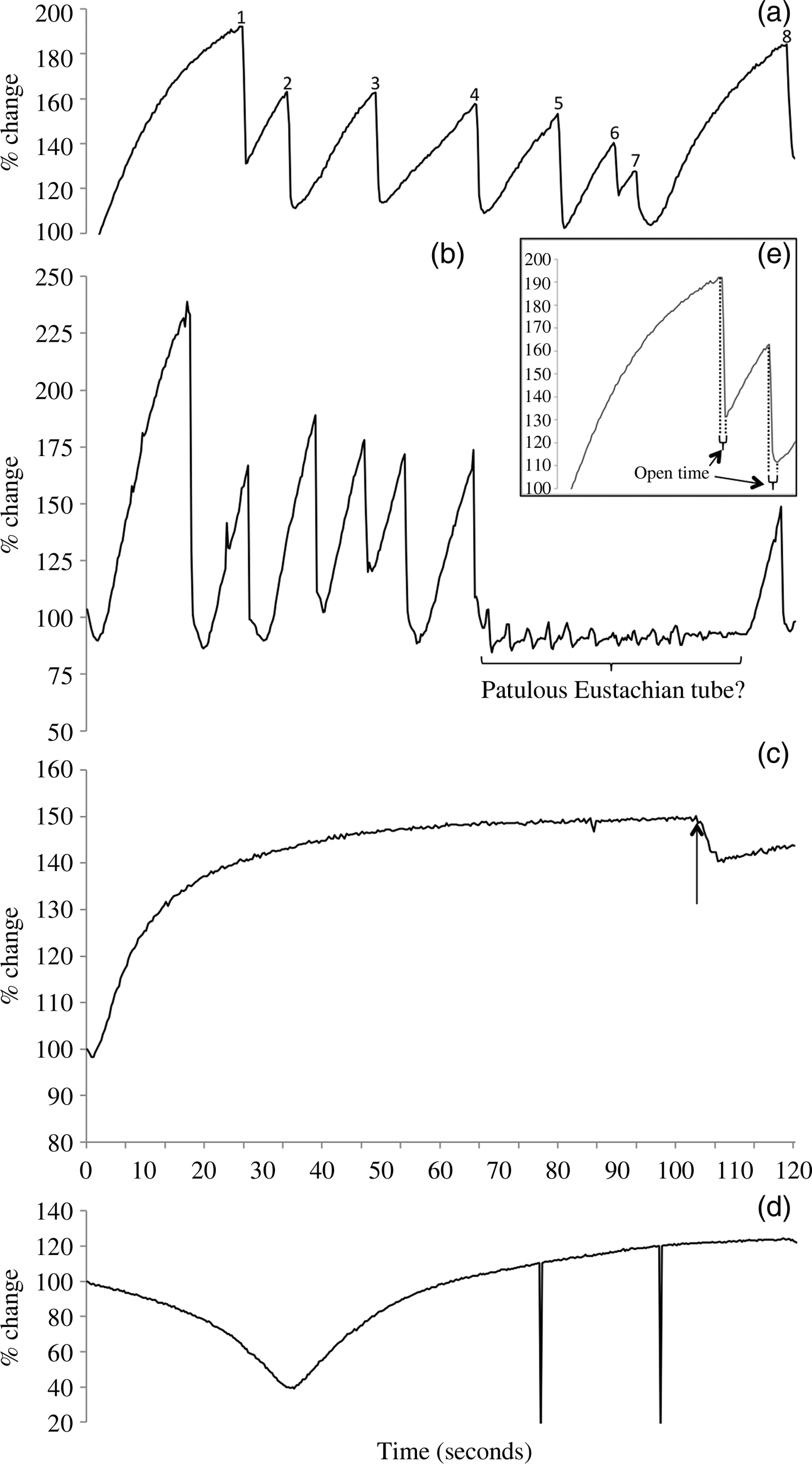
Fig. 2. Ear drum stiffness recordings in the pressure chamber during the +10 kPa pressure reduction, showing representative types of traces. (a) An ‘opening’ pattern, in which the tube spontaneously opens periodically as the external pressure decreases, to give a saw-tooth shape. This trace shows nine openings during the pressure decrease and presumably represents an optimally functioning Eustachian tube. (b) In this example, the Eustachian tube opens very readily and repeatedly, in a saw-tooth pattern. However, over the period marked, and despite the constant pressure decrease, no openings are observed and there are small fluctuations in ear drum stiffness at around 0.3 Hz. This trace is interpreted as pressure fluctuations due to respiratory activity acting through a patulous tube. (c) In this ‘limited opening’ example, the tube fails to open for most of the pressure decrease. It shows only a single, small, prolonged opening at the point arrowed. (e) The inset shows a detail of the trace from part (a) and illustrates the measurement of ‘open time’ for each Eustachian tube opening. (d) In this example of an inverted peak, the middle ear has not equalised after the chamber has increased to +10 kPa and so has a residual negative pressure. As the pressure decreases, the eardrum, which starts tightly drawn in due to the net negative pressure in the middle ear, relaxes as the chamber pressure decreases towards the middle-ear pressure. The nadir in the trace occurs when the chamber pressure equals the middle-ear pressure. The subsequent chamber pressure decrease results in the stiffness increasing again, as the chamber pressure drops below the middle-ear pressure and draws the ear drum outwards. In this example, the Eustachian tube never opened (‘no opening’). The two sharp downward peaks are artifacts caused by subject movement.
In the first (‘opening’) pattern (Figure 2a), the Eustachian tube was seen to open and then close at least three times as the chamber pressure dropped. Each opening caused a sudden decrease in ear drum stiffness, and each closing was followed by a rapid asymptotic increase in stiffness of the ear drum. Openings and closings usually occurred multiple times in each cycle, to give a characteristic saw-tooth pattern to the stiffness trace (rapid equalisation and a drop in stiffness, followed by a subsequent rise in stiffness as the pressure difference increased, until the next tube opening and equalisation).
In the second pattern (Figure 2b), openings of the Eustachian tube occurred, but in some instances the subsequent increase in ear drum stiffness as the chamber pressure continued to drop was not seen. Instead, stiffness remained generally steady, but with small oscillations at around 3 second intervals. In these cases where ear drum stiffness did not respond promptly to changes in chamber pressure, it appeared that the Eustachian tube was passing a constant small flow of air out of the middle ear that almost exactly balanced the pressure change. The presumed continued leak of air through the Eustachian tube may represent a patulous Eustachian tube, but it is possible that the tube may only remain open while a pressure differential exists and air is flowing. The small oscillations in pressure with a 3 second interval seen at this time are likely to represent fluctuations in middle-ear pressure caused by respiration recorded through the open Eustachian tube. Such ‘patulous’ Eustachian tubes were only seen in two individuals.
In the third pattern (Figure 2c), ear drum stiffness increased asymptotically to a plateau as the chamber pressure decreased. This rise in stiffness is essentially an inverted version of one-half of the standard tympanogram trace where compliance falls as ear canal pressure decreases (negative ear canal pressure). The measured stiffness could be maintained at this maximum value in some subjects, or there could be one or two small openings of the Eustachian tube, often prolonged in duration and limited in their effect. We call this pattern ‘no opening’ or ‘limited opening’, respectively, and it probably represents some degree of Eustachian tube dysfunction. Eustachian tubes that opened only once or twice in the course of a run were grouped with those that did not open at all, because the one or two openings were usually late in the run (i.e. when the pressure differential across the ear was high), and invariably they did not result in much equilibration of pressure.
A fourth pattern was present in a number of recordings of ear drum stiffness (Figure 2d). This was seen as an initial decrease in stiffness as the external pressure dropped; this eventually reached a nadir, at which point stiffness started to increase, as was normally seen in most ears from the outset. This initial fall in stiffness followed by a subsequent rise appeared on the trace as an inverted peak. Sometimes, individual ears that showed an inverted peak also showed a normal pattern of opening and closing of the Eustachian tube in other trials, but this was only ever seen after the nadir was reached.
We interpreted this fourth pattern as resulting from an incomplete equalisation of the middle ear by the subjects concerned, once the chamber pressure was increased from +3 kPa to +10 kPa. The pattern represents an inverted version of a standard tympanogram trace, with the ear canal pressure sweeping from positive to negative. The initial rise in chamber pressure would result in a relative negative pressure in the middle ear at +10 kPa, and so the ear drum would be drawn in and stiffened. As the chamber pressure dropped again, the ear drum would slacken until the middle-ear pressure and the external pressure were equal (the nadir in ear drum stiffness). However, as the chamber pressure continued to drop, the ear drum would bulge outward into the external ear canal, and stiffness would again increase. Spontaneous passive openings of the Eustachian tube could occur during this stage, as for other subjects, because middle-ear pressure was now positive relative to the chamber.
Regarding the fourth pattern, where a negative peak was present in the recording of ear drum stiffness during the depressurisation, the tympanometer reading prior to the decrease in chamber pressure from +10 kPa was always strongly negative or, in many cases, recorded as ‘off scale’. Because the tympanometer could record pressures within the range of −3.6 kPa to +3.6 kPa, this indicated that the starting pressure in the ear was more negative than −3.6 kPa. Furthermore, failure to equalise successfully at +10 kPa in one cycle was a predictor of failure to equalise in subsequent cycles, so that the failure of equalisation during the +10 kPa step readily identified a group of subjects who repeatedly were unable to equalise to chamber pressure, perhaps indicating deficient Eustachian tube function. In contrast, other subjects were consistently able to equalise prior to the +10 kPa test.
The pattern of Eustachian tube opening was determined for each control run for all 24 subjects. Then, each subject was classified according to the dominant pattern seen in the majority of the seven control runs. Ear drum stiffness in the left and right ears of each individual are reported separately.
An initial assessment of the results suggested that the behaviour of each Eustachian tube was largely independent of its contralateral counterpart. Comparing left and right ears in the same individual, we found 7 out of 24 instances where both ears were classified as having different patterns of opening (‘opening’ vs ‘no opening’ or ‘limited opening’ vs ‘patulous’). Given that nearly a third of individuals had Eustachian tubes that differed in behaviour, we analysed the data as 48 individual Eustachian tubes, rather than combining them in some way in each of 24 individual subjects. That is, the behaviour of the two Eustachian tubes of any subject is assumed to be largely independent of each other.
One behaviour of Eustachian tubes that appeared not to be independent was the simultaneous openings that were sometimes observed in the two ears. While subjects were instructed not to swallow during the chamber pressure decrease from +10 kPa, bilateral simultaneous openings are likely to be a response to a common trigger event, such as an involuntary swallow or other jaw movement.
Following our classification system, 35 out of 48 Eustachian tubes (73 per cent) were classified as the ‘opening’ type for the seven control runs. However, 13 out of 48 Eustachian tubes (27 per cent) (representing 9 out of 24 individuals) were classified as ‘no opening’ or ‘limited opening’. Given that our subjects were selected for self-reported problems with middle-ear equalisation, this may represent the detection of an underlying Eustachian tube dysfunction. Eight Eustachian tubes were classified in this way (‘no opening’ or ‘limited opening’) for all seven runs, with the remaining five classified in this way for the majority of the runs. Inverted peaks were associated with 5 of the Eustachian tubes classified as ‘opening’ (14 per cent), and with 11 of those classified as ‘no opening’ or ‘limited opening’ (85 per cent). Unsurprisingly, the three patulous Eustachian tubes were always associated with ‘opening’ tubes. In one case, the patulous tubes were bilateral in the one subject.
The behaviour of the Eustachian tubes during the +10 kPa passive test was correlated with that during the test of active Eustachian tube function at +3 kPa. In the Eustachian tubes classified during the passive test as the ‘opening’ type, one swallow decreased the pressure by a mean of 1.41 kPa (standard error of the mean ± 0.14 kPa) across the seven control runs when testing active opening. The Eustachian tubes classified as ‘no opening’ or ‘limited opening’ during the passive test decreased pressure by only a mean of 0.78 kPa (standard error of the mean ± 0.22 kPa) during a single swallow in the active Eustachian tube function test.
Effect of betahistine treatment
Betahistine did not appear to obviously change the behaviour of the Eustachian tubes (Figure 3). If betahistine made opening of the Eustachian tube easier, then a single swallow at +3 kPa should be more effective in equalising the pressure differential between the middle ear and the pressure chamber. Similarly, if betahistine improved Eustachian tube function, then the tube would be expected to spontaneously open at a lower pressure differential between the middle ear and the chamber, leading to more openings being recorded during the pressure decrease from +10 kPa. Alternatively, the Eustachian tube might remain open for longer on each opening, increasing the amount of air passed.
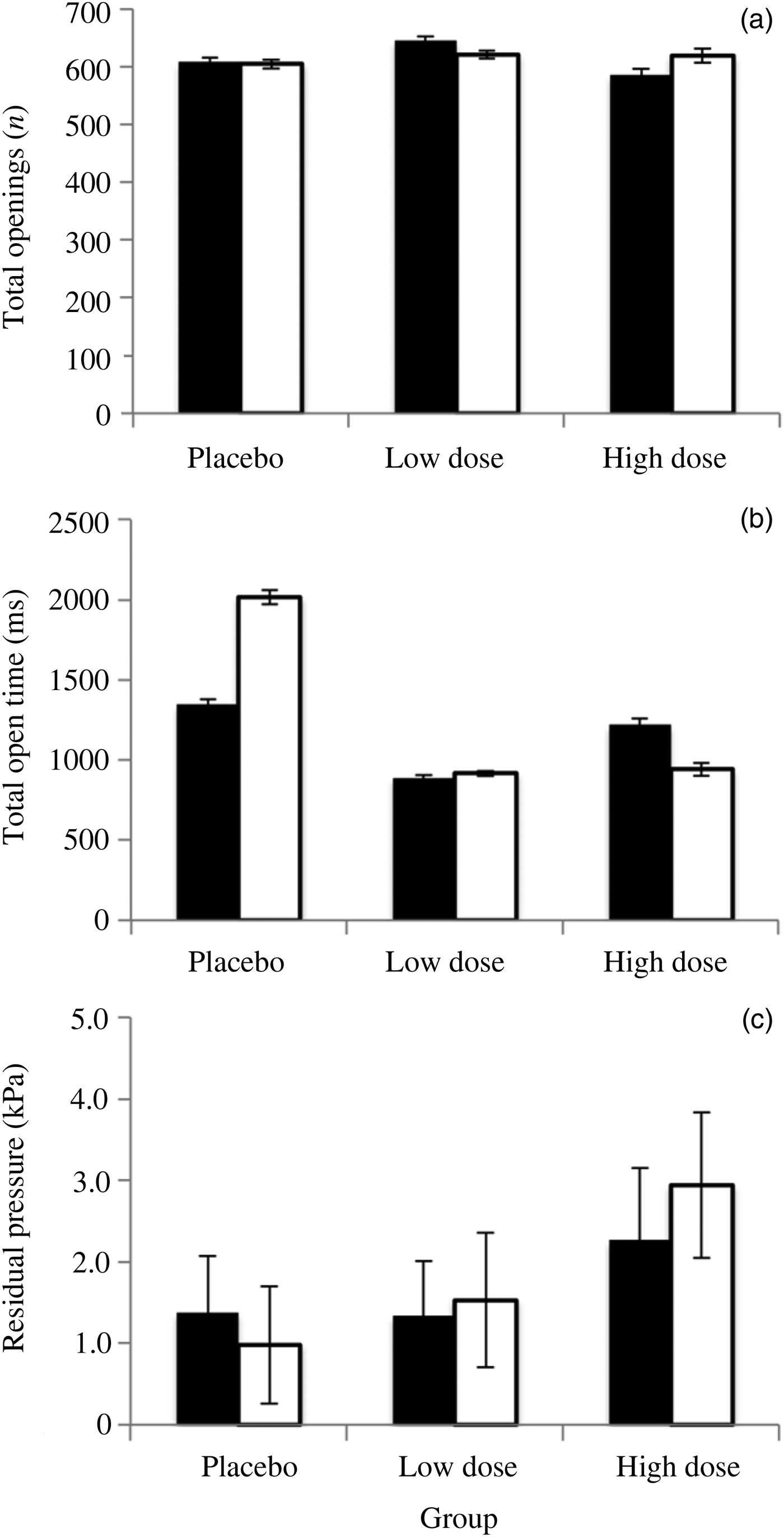
Fig. 3. The results of intranasal treatment of subjects with the vehicle (placebo group), or 4 mg/ml or 40 mg/ml betahistine hydrochloride (low dose and high dose groups respectively), for control runs (black bars) and treatment runs (white bars) during the chamber equalisation to ambient pressure from +10 kPa. Control was compared to treatment with paired t-tests. (a) The total number of Eustachian tube openings did not significantly differ between the control and treatment groups. (b) The total time that the Eustachian tube spent open was not significantly different for any of the treatments. (c) The residual pressure in the middle ear after attempting equalisation at 10 kPa (a measure of how well subjects were equalising after the step from +3 kPa to +10 kPa) did not significantly differ between control and treatment groups. In each case, error bars are standard errors of the mean.
The residual pressure in the middle ear after attempting to equalise at 10 kPa did not change after treatment (placebo – t = −2.186, p = 0.06; low dose – t = 0.494, p = 0.63; high dose – t = 1.283, p = 0.23). Similarly, the total number of openings per run of the Eustachian tubes did not vary between the three treatment groups (placebo – t = −0.053, p = 0.96; low dose – t = −0.351, p = 0.73; high dose – t = 0.262, p = 0.80), nor did the cumulative time that the Eustachian tubes were open (placebo – t = 1.797, p = 0.11; low dose – t = 0.235, p = 0.81; high dose – t = −0.80, p = 0.45).
Technical considerations
The major problem encountered during this study was a failure of the ear tips to seal the ear canal and maintain the microphone and speaker tubes in an appropriate relationship to the ear drum. Loss of ear tip position resulted in the loss of the stiffness recording for that run. In nearly all cases, it appeared that the configuration of the external ear canal was incompatible with the range of ear tip sizes available. Failure of long-term maintenance of an ear tip seal could have usefully been included in screening as an exclusion criterion.
A further failing was the lack of confirmation of successful equilibration at +10 kPa. The tympanometer reading at this time often showed that individuals were not fully equilibrated. If the pressure challenge from +10 kPa was delayed until equilibration had been confirmed by tympanometry, then all subjects could have been tested over the same range of pressures. Note that in these subjects, as a consequence of the initial negative pressure in the middle ears, Eustachian tube function was tested over a smaller range of chamber pressures than subjects with fully equalised middle ears. However, these individuals were not excluded from subsequent analysis.
The hyperbaric chamber proved a flexible and reproducible way of testing Eustachian tube performance against known changes in middle-ear or external pressure. While the chamber operator was required to manually regulate the pressure drop to maintain a linear change, this was rapidly mastered by all chamber operators and resulted in a consistent pattern of pressure change.
Discussion
Effect of betahistine on Eustachian tube opening
We have previously shown in a rat model that intranasal betahistine improves Eustachian tube function.Reference Franz, Shafton and Anderson1 In that study, Eustachian tube function was tested in healthy, anaesthetised rats by increasing pressure within the middle ear and inducing them to swallow by stimulating the recurrent laryngeal nerve. Betahistine was applied either within the middle ear or within the nasopharynx to the medial ends of the Eustachian tube. In both cases, the Eustachian tube passed more air for each swallow when treated with betahistine, allowing better equalisation of the middle-ear pressure.
In the current human trial, we used a similar dose to that used previously on rats (200 µl of 4 mg/ml, low dose group), as well as a much higher dose (200 µl of 40 mg/ml, high dose group). However, contrary to the results in rats, betahistine applied to the Eustachian tube in our human subjects failed to result in improved Eustachian tube function. A single voluntary swallow leading to active opening of the Eustachian tube was no more efficient at equalising against a middle-ear pressure of −3 kPa in the presence of betahistine. Similarly, betahistine did not induce more passive openings of the Eustachian tube during periods of positive middle-ear pressure. In fact, the high dose of betahistine appeared to decrease the probability of Eustachian tube opening.
H3 receptors are present in the human nasal epithelium, and when stimulated they lead to sympathetic vasoconstriction.Reference Varty, Gustafson, Laverty and Hey8 H3 receptors are constitutively active, and most antagonists, including betahistine, are inverse agonistsReference Arrang, Morisset and Gbahou9 decreasing constitutive activity. However, betahistine has a complex interaction with the H3 receptor. When tested in either cell lines in vitro or on the mouse brain, it is an inverse agonist at lower doses, but at higher doses it has an agonist action (increasing receptor activity).Reference Gbahou, Davenas, Morisset and Arrang10
The low dose of betahistine used in this study was the same as that used to successfully improve Eustachian tube function in rats.Reference Franz, Shafton and Anderson1 Betahistine has been shown to be broadly similar in effect on recombinant rat and human H3 receptors.Reference Gbahou, Davenas, Morisset and Arrang10 Perhaps the investigation of a range of doses around those used here will reveal one that improves Eustachian tube function. Alternatively, betahistine may not have the ability to modify the surface tension of epithelial secretion into the Eustachian tube in humans, as is proposed in rats.
The high dose of betahistine appeared to decrease the probability of Eustachian tube opening. This may reflect the agonist action of high doses of betahistine on H3 receptors. Alternatively, histamine H1 receptors have been reported in the nasal mucosa of humans;Reference Shelton and Eiser11 as betahistine is a mild H1 agonist,Reference Arrang, Garbarg, Quach, Dam Trung Tuong, Yeramian and Schwartz12 activation of these receptors with a high dose of betahistine may account for the worsening of Eustachian tube function, as reported for the actions of histamine.Reference Downs, Butehorn, Prazma, Rose, Stamat and Pillsbury13–Reference Ebert, Pollock, Dubin, Scharer, Prazma and McQueen15
Measuring Eustachian tube function
Pressure chambers and impedance measurements have been used to examine Eustachian tube function on only a few occasions. In addition to Thomsen,Reference Thomsen16 Maier et al.Reference Maier, Hauser and Munker17 compared subjects with sudden hearing loss to normal subjects, using a pressure chamber and impedance measurements. Maier et al.Reference Maier, Munker and Strutz18 used the same approach to test the effect of anaesthesia on the ear drum, and to later show that Eustachian tube function was adversely affected by radiation therapy for laryngeal carcinoma.Reference Maier, Beck, Hinkelbein and Richter19 Miyazawa et al.Reference Miyazawa, Ueda and Yanagita20 have described the testing of Eustachian tube function over pressure cycles that covered a range of 59 kPa. More recently, Mikolajczak et al.Reference Mikolajczak, Meyer, Felsch, Jumah, Huttenbrink and Grosheva21,Reference Mikolajczak, Meyer, Hahn, Korthauer, Jumah and Huttenbrink22 have used the same system to examine normal Eustachian tube function.
Changing the pressure external to the ear drum is the only way to reliably and controllably impose a known pressure challenge on the middle ear and test Eustachian tube function. A hyperbaric chamber, such as one used for hyperbaric oxygen therapy, or a hypobaric chamber, such as is used for experimental aerospace medicine, can easily impose the necessary pressure change. These chambers are capable of imposing large pressure changes exceeding many tens of kilopascals; however, such changes are not necessary to test Eustachian tube function. Pressure changes of 1, 2 or 3 kPa impose a sufficient challenge to test active Eustachian tube function by swallowing. In addition, passive opening properties, as in the middle ear, equilibrated at a positive pressure differential, are challenged by a return to ambient pressure. The ability to operate at these modest pressure differentials means that a full scale hyperbaric or hypobaric chamber is not needed. Instead, such pressure differentials can be generated by more modest means, as has previously been described.Reference Kodama, Kitahara, Ozawa and Izukura7,Reference Ingelstedt and Ortegren23
In such a low pressure chamber, Eustachian tube function can be repeatedly tested and the results quantified by use of a tympanometer and impedance device within the chamber. Both active and passive behaviours of the Eustachian tube can be measured, and it is possible to prove the effectiveness of any intervention designed to alter Eustachian tube function, such as a drug treatment as was used here.
Passive opening can be studied by generating a positive pressure in the middle ear by decreasing hypobaric chamber pressure, or by equalising middle-ear pressure after increasing chamber pressure. The Eustachian tube behaviour can then be examined as the chamber returns to ambient. Among the parameters that can be measured are: the pressure change required to spontaneously open the Eustachian tube, the number of times the Eustachian tube opens, the amount of time the Eustachian tube stays open or the pressure remaining in the middle ear when the chamber pressure reaches ambient. The process is easily repeated to average out variation.
Active swallowing could be tested against either a positive or negative gradient across the ear drum. Useful parameters include the number of swallows or jaw openings required to equalise the ear, or the pressure drop achieved by one or more swallows.
Suitable pressure chamber for this process could be a reinforced and modified auditory booth coupled to a small air pump to shift air (such as is found on a domestic vacuum cleaner). Specific pressure levels could be set by either a rheostat on the motorReference Ingelstedt and Ortegren23 or an adjustable pressure relief valve to balance the chamber pressure. For taking tympanometry and impedance readings, it is best if the chamber is large enough to contain an audiologist as well as the subject. Note that portable tympanometers have a limit to the range of ambient pressures they work over. The range in the tympanometer used in this study was +3.6 kPa to −3.6 kPa.
• The Eustachian tube regulates middle-ear pressure; Eustachian tube dysfunction leads to long-term hearing problems
• This study tested the ability of betahistine to improve Eustachian tube function in human subjects with potential Eustachian tube dysfunction
• The middle-ear pressure was challenged using hyperbaric chamber pressure changes, with and without topical betahistine application
• Middle-ear pressure was measured with tympanometry and Eustachian tube opening was measured with an impedance bridge
• Betahistine treatment did not improve Eustachian tube function, in contrast to our previous animal study
• The hyperbaric chamber, tympanometry and impedance bridge proved ideal for assessing Eustachian tube behaviour
In using such a chamber to test Eustachian tube function, it would be necessary to test both passive and active opening of the Eustachian tubes, as dysfunction could affect one and not the other. For instance, congenital changes in palate formation or of the associated muscles could lead to dysfunction in the active function of a Eustachian tube that responds perfectly well to passive changes in middle-ear pressure. Both ThomsenReference Thomsen16 and MünkerReference Münker5 concluded that tests of Eustachian tube function should rely on positive pressure in the middle ear, not negative pressure. This is certainly the case when testing the passive opening properties of the middle ear, because negative middle-ear pressure will tend to keep the tube closed. However, Eustachian tube performance against negative pressure is the most important clinically, because negative pressure is generated normally in the middle ear by (normal) gas absorption. Negative pressure is most likely to be equalised by swallowing or another jaw movement, and so should be used to test active Eustachian tube function, as was done here.
Conclusion
In contrast to animal studies, betahistine had no positive effect on Eustachian tube function. The combination of a hyperbaric chamber and tympanometry proved ideal for evaluating active and passive Eustachian tube function. This combination promises valuable data in the differential diagnosis of Eustachian tube dysfunctions. Only mild pressure changes are necessary to study Eustachian tube function.
Acknowledgements
We would like to thank Dr Ian Millar, Alfred Hospital, Melbourne, and the staff of the Wesley Centre for Hyperbaric Medicine, Brisbane, particularly Sharon Pert, Dave Saxton and Billy Pert, for their valuable input and assistance. We would also like to acknowledge the volunteers who took part in this study.
Competing interests
This study was sponsored by Otifex Therapeutics, Melbourne, who employed authors BHF, CJW and CRA. It was carried out under a commercial arrangement with Wesley Centre for Hyperbaric Medicine, Brisbane, who employed authors GK and AN.