Introduction
Relatively large numbers of hybridizations have been carried out in fin-fish species for basic research and aquaculture-oriented studies (Swartz Reference Swartz1981). Various interspecific and intergeneric hybrids, i.e. allo-diploids, have been produced, especially in salmonid species. While some of these hybrids die at the early developmental stages, others are viable and can grow to adult stages (Suzuki and Fukuda, Reference Suzuki and Fukuda1971; Blanc and Chevassus, Reference Blanc and Chevassus1979; Chevassus et al., Reference Chevassus, Guyomard, Chourrout and Quillet1983; Hulata, Reference Hulata2001). Inviability of some hybrids has been partially explained by aneuploidies due to uniparental chromosome elimination during early embryonic stages (Arai Reference Arai1984; Fujiwara et al. Reference Fujiwara, Abe, Yamaha, Yamazaki and Yoshida1997). Even in viable hybrids, sterility can arise during gonadal development or gamete production, and inviable zygotes have often appeared after back-crossing and/or inter-crossing of hybrids (Suzuki and Fukuda, Reference Suzuki and Fukuda1973; Suzuki, Reference Suzuki1974; Chevassus et al., Reference Chevassus, Guyomard, Chourrout and Quillet1983). The induction of triploid hybrids (allo-triploids) has given rise to drastic recovery or increased survival potential in several inviable hybrids (Chevassus et al., Reference Chevassus, Guyomard, Chourrout and Quillet1983; Scheerer and Thorgaard, Reference Scheerer and Thorgaard1983; Arai, Reference Arai1984, Reference Arai1986, Reference Arai1988; Ueda et al., Reference Ueda, Ojima, Sato and Fukuda1984; Parsons et al., Reference Parsons, Busch, Thorgaard and Scheerer1986; Yamano et al., Reference Yamano, Yamaha and Yamazaki1988; Seeb et al., Reference Seeb, Thorgaard and Utter1988; Gray et al., Reference Gray, Evans and Thorgaard1993). These types of viable allo-triploid hybrid strains exhibit sterility and have been commercially farmed to support the vitalization of local economies in Japan (Arai, Reference Arai2000, Reference Arai2001; Arai and Fujimoto, Reference Arai, Fujimoto, Wang, Piferrer, Chen and Shen2019).
In teleost fish, it has been thought that natural hybridization between different species occurs frequently and contributes genetic introgression in the process of speciation (Scribner et al., Reference Scribner, Page and Bartron2001). However, almost all artificial hybrids cannot reproduce their filial generations because of the above-mentioned phenomena such as non-viability and sterility. Therefore, the analysis of hybrid inviability and sterility between species is of great importance in genetic breeding and biological evolution.
In cyprinid species, many hybrids have been produced via interspecies, inter-genus, and even inter-family combinations (Suzuki, Reference Suzuki1956, Reference Suzuki1961, Reference Suzuki1962, Reference Suzuki1968; Ojima, Reference Ojima1973). For inter-subfamilial hybrids that have been produced using species from the subfamilies Acheilognathinae, Gobioninae or Cyprininae, most of these progeny exhibited inviability at the hatching stage (Suzuki, Reference Suzuki1956, Reference Suzuki1961, Reference Suzuki1962, Reference Suzuki1968), while those between species in Oxygastrinae exceptionally developed normally and produced mature eggs (Li et al., Reference Li, Xie, Xiao, Yuan, Zhou, Luo, Zhang, Zhao, Tao and Liu2019). Although inviable cyprinid hybrids have not been cytogenetically studied, Kijima and colleagues (Reference Kijima, Arai and Suzuki1996a,b) reported that inviable interfamilial hybrids between dojo loach females (family Cobitidae) and goldfish, minnow or common carp males (family Cyprinidae), had allo-diploid karyotypes intermediate between the two parental species. Therefore, inviability of these interfamilial hybrids could not be explained by aneuploidies due to chromosome elimination during embryogenesis. These authors also reported that induction of allo-triploidization did not enable the recovery of survival potential and produce viable triploid hybrids, although it did improve the external appearance of the resultant hybrid larvae (Kijima et al., Reference Kijima, Arai and Suzuki1996a,b).
As seen in the inviable hybrids from certain combinations of fish species, artificially induced haploid embryos exhibit severe abnormalities collectively referred to as haploid syndrome. These abnormalities include dwarfism, microcephaly, microphthalmia and oedema, therefore almost all gynogenetic or androgenetic haploids die before hatching or first feeding (Arai, Reference Arai2000, Reference Arai2001; Arai and Fujimoto, Reference Arai, Fujimoto, Wang, Piferrer, Chen and Shen2019). In goldfish, gynogenetically induced haploid embryos die before or around hatching, however haploid cells were able to survive when they were transplanted into diploid blastula embryos and, consequently, viable haploid–diploid chimeras can be produced (Tanaka et al., Reference Tanaka, Yamaha and Arai2004). The appearance of spontaneous haploid–diploid mosaic charr also supports the survival of haploid cells within diploid cell populations under chimeric or mosaic conditions (Yamaki et al., Reference Yamaki, Kawakami, Taniura and Arai1999). If this was also the case for inviable hybrids, cells transplanted from inviable hybrids may survive and function in conspecific hosts under chimeric conditions.
In viable hybrids, disturbance can also frequently occur during meiosis and the subsequent differentiation of gonads or gametes because of the presence of non-homologous genomes from genetically distant species. The loss of germ cells has also been reported in viable marine red dram hybrids (Yoshikawa et al., Reference Yoshikawa, Xu, Ino, Hayashida, Wang, Yazawa, Yoshizaki and Takeuchi2018). Hybrid fish are therefore often sexually abnormal and can exhibit reproductive performances that range widely, from near-normal fertility to complete sterility or infertility in either or both of the sexes. However, as sterility is a useful characteristic for aquaculture – because sterile fish diminish the risk of genetic contamination of indigenous populations by escaped farmed fishes – sterile hybrids are considered highly useful hosts for transgenesis, genome editing and surrogate reproduction for biological containment (Arai and Fujimoto, Reference Arai, Fujimoto, Wang, Piferrer, Chen and Shen2019). For example, hybrids between female crucian carp (the origin of goldfish) Carassius auratus and male common carp Cyprinus carpio show male sterility due to the death of spermatocytes, which presumably results from a germ cell-autonomous abnormality (Makino et al., Reference Makino, Ojima and Matsui1958; Ojima, Reference Ojima1973). When exotic primordial germ cells (PGCs) were transplanted from goldfish, one parental fish, the germline of the resultant chimera, would produce sperm that is exclusively derived from the transplanted PGCs, because the host PGCs are abnormal (Yamaha et al., Reference Yamaha, Murakami, Hada, Otani, Fujimoto, Tanaka, Sakao, Kimura, Sato and Arai2003).
In inviable hybrids, the process of PGC differentiation into gametes has not been investigated. In normal teleost development, PGCs differentiate from blastomeres that are inherited along with maternal germplasm (Saito et al., Reference Saito, Pšenička, Goto, Adachi, Inoue, Arai and Yamaha2014) and, in various species, PGCs have been visualized by injecting GFP-nos3 3′UTR mRNA to mimic maternal mRNA in the germplasm (Saito et al., Reference Saito, Fujimoto, Maegawa, Inoue, Tanaka, Arai and Yamaha2006; Nagasawa et al., Reference Nagasawa, Femandes, Yoshizaki and Babiak2013; Goto et al., Reference Goto, Saito, Kawakami, Kitauchi, Takagi, Todo, Arai and Yamaha2015). PGCs visualized in this way maintain their migratory potential under in vivo conditions, even after transplantation (Saito et al., Reference Saito, Goto-Kazeto, Arai and Yamaha2008). This technique enables the analysis of PGC differentiation and potential (i.e. cellular viability/inviability) in germline chimeras.
Goldfish (Carassius auratus) is a popular ornamental fish in Japan that has long been a useful experimental model for reproductive biology, developmental biology, endocrinology and other disciplines (Devlin and Nagahama, Reference Devlin and Nagahama2002; Habibi et al., Reference Habibi, Nelson and Allan2012; Tsai et al., Reference Tsai, Chang, Liu, Abe and Ota2013; Urushibata et al., Reference Urushibata, Takahashi, Shimizu, Miyazaki, Fujimoto, Arai and Yamaha2019). In particular, as a model for micromanipulation, embryos of this species have revealed specific insights of several biological processes. For example, graft transplantations have revealed that blastoderm cells are highly pluripotent at the blastula stage (Yamaha et al., Reference Yamaha, Mizuno, Hasebe and Yamazaki1997; Kazama-Wakabayashi et al., Reference Kazama-Wakabayashi, Yamaha and Yamazaki1999). Germline chimeras are also easily induced by graft transplantation (Yamaha et al., Reference Yamaha, Kazama-Wakabayashi, Otani, Fujimoto and Arai2001, Reference Yamaha, Murakami, Hada, Otani, Fujimoto, Tanaka, Sakao, Kimura, Sato and Arai2003), as well as by PGC transplantation (Goto-Kazeto et al., Reference Goto-Kazeto, Saito, Takeda, Fujimoto, Takagi, Arai and Yamaha2012). These data suggest that hybrids between goldfish females and males of other species can be highly advantageous for analyses of hybrid cells, including germline cells. Specifically, developmental potential, such as the viability and fertility of hybrid somatic and germline cells themselves, can be confirmed in vivo by transplantation of hybrid cells into normal goldfish, as intrinsic survival and differentiating potentials of hybrid cells, which gave rise to lethal effect to an individual, could be examined under the chimeric conditions with a viable host individual.
Golden venus chub (Hemigrammocypris rasborella) is a small freshwater cyprinid fish that lives in the swamps and rivers of Japan. Recently, this species has been designated as an endangered species 1B, because its population is in decline (https://www.env.go.jp/press/files/jp/109165.pdf). Previously induced artificial hybrids between Gobioninae female fish – including Biwia zezera, Squalidus japonicus, and Gnathopogon elongatus – and Hemigrammocypris rasborella males were inviable (Suzuki, Reference Suzuki1968). However, hybridization between goldfish and golden venus chub has not yet been reported.
Here, we induced hybrids between female goldfish, Carassius auratus, and male golden venus chub, Hemigrammocypris rasborella (hereafter HR), and analyzed their ploidy and development potential. The resultant hybrid progeny with allo-diploidy were inviable, but spontaneously occurring allo-triploid hybrids were viable. Thereafter, we confirmed whether artificial allo-triploidization increased viability in this hybrid combination, and we examined the cause of inviability by transplanting diploid hybrid cells into a goldfish host. Finally, we observed PGCs’ specification and gonadal development.
Materials and methods
Fish
Parent goldfish and golden venus chub were kept at the Nanae Fresh-Water Laboratory, Hokkaido University. Parent goldfish were maintained at 10–14°C. Parent HR originated from Hyogo Prefecture and were purchased from a commercial pet shop. Parent HR were kept in laboratory aquaria at 22–25°C and with a 16 h : 8 h, light : dark photoperiod. Collection of goldfish sperm and mature eggs was performed according to Yamaha et al. (Reference Yamaha, Kazama-Wakabayashi, Otani, Fujimoto and Arai2001). HR sperm was collected using a 10 μl crystal tip from anesthetized male fish, and diluted in artificial seminal plasma for goldfish (NaCl 5.61 g, KCl 5.23 g, CaCl2.2H2O 0.33 g, MgCl2.6H2O 0.22 g, NaHCO3 0.2 g/l in distilled water) (Yanagimachi et al., Reference Yanagimachi, Harumi, Matsubara, Yan, Yuan, Hirohashi, Iida, Yamaha, Arai, Matsubara, Andoh and Vines2017).
Artificial fertilization of goldfish eggs with HR sperm was performed as follows: goldfish eggs were inseminated with diluted HR sperm on a polyvinylidene film, fertilized by mixing with a small amount of freshwater, and then scattered into plastic Petri dishes containing urea water (0.2% urea and 0.24% NaCl in tap water) to reduce viscosity of eggs. In control goldfish fertilizations, eggs inseminated with diluted sperm were directly scattered into urea water. Eggs were fertilized in several different dishes in each cross. We used a representative dish for calculation of fertilization and survival rates by counting cleaved and surviving (and dead) eggs. Other dishes were used for samples of flow cytometry (FCM), histology and/or micromanipulation.
Dechorionation and incubation conditions
Dechorionation of fertilized eggs was performed using the method described by Yamaha and Yamazaki (Reference Yamaha and Yamazaki1993). Prior to experimental manipulations, dechorionated embryos were incubated in 1% agar-coated Petri dishes filled with Ringer’s culture solution (128 mM NaCl, 2.8 mM KCl, 1.8 mM CaCl2) with 1.6% albumen.
Thermal treatment of fertilized eggs
To induce allo-triploid progeny, goldfish eggs fertilized with either HR or goldfish sperm were heat-shocked at 40°C for 50–75 s, 5 min after fertilization (Nagoya et al., Reference Nagoya, Kimoto and Onozato1990). Immediately after treatment, Petri dishes with fertilized eggs were washed and filled with urea water at 20°C, and then cultured in an incubator at 10 or 20°C. Hatched fish with a normal appearance were reared by feeding with brine shrimp nauplii and artificial crumble fish feed.
Microinjection
Fertilized and dechorionated eggs were injected with several solutions to label the egg cytoplasm, germplasm or PGCs. PGCs were visualized by injecting artificial mRNA for GFP and a 3′UTR region of nos3, a germplasm specific RNA of zebrafish (Saito et al., Reference Saito, Fujimoto, Maegawa, Inoue, Tanaka, Arai and Yamaha2006). To label donor cells for the blastoderm and blastomere transplantations, 5% FITC (Sigma) and 5% biotin–dextran–lysine (Sigma) in 0.2 M KCl solution were injected for fluorescence and histological detection, respectively. To detect germline cells before or after PGC differentiation, a mixture of GFP-Bucky ball mRNA and GFP-nos3 3′UTR mRNA in 0.2 M KCl solution was injected or co-injected into egg cytoplasm at the 1-cell to 2-cell stage (Saito et al., Reference Saito, Fujimoto, Maegawa, Inoue, Tanaka, Arai and Yamaha2006, Reference Saito, Pšenička, Goto, Adachi, Inoue, Arai and Yamaha2014). The labelled germplasm or cells were observed under a fluorescence microscope (Leica MZ16F) and photographed with an attached digital camera (Leica DFC7000T). For histological detection of labelled cells, serial paraffin sections were made from embryos in which labelled cells were transplanted, as described below.
Cell transplantation
In this study, we performed two types of transplantation. In the first type, various grafts of hybrid blastoderm labelled with FITC and biotin–dextran were transplanted to goldfish blastulae according to Yamaha et al. (Reference Yamaha, Mizuno, Hasebe and Yamazaki1997) and Kazama-Wakabayashi et al. (Reference Kazama-Wakabayashi, Yamaha and Yamazaki1999). Specifically:
-
(i) The entire blastoderm was dissected away from the yolk cells of a hybrid blastula and transplanted onto the animal part of a goldfish blastula that had had the upper part of its blastoderm removed – resultant embryos are referred to as goldfish-hybrid chimeras (Fig. S1 A).
-
(ii) The lower part of the blastoderm was dissected from a hybrid blastula and transplanted into the middle of a goldfish blastula in which the blastoderm had been horizontally bisected – resultant embryos are referred to as sandwich chimeras (Fig. S1 B).
-
(iii) The upper part of the blastoderm from hybrid and goldfish blastulae was removed by glass needle and exchanged – resultant embryos are referred to as goldfish-base chimeras and hybrid-base chimeras, respectively (Fig. S1 C1, C2).
Controls for these micro-surgeries were intact embryos with chorion, and intact dechorionated embryos. After transplantation, controls and chimeric embryos were cultured separately for 1 day in 96-well culture plates filled with Ringer’s culture solution. They were then individually moved to a 96-well culture plate filled with 1.8 mM CaCl2 and 1.8 mM MgCl2 solution and cultured until the intact controls hatched.
In the second type of transplantation, small numbers of blastomeres were transplanted into goldfish blastulae in accordance with Saito et al. (Reference Saito, Goto-Kazeto, Fujimoto, Kawakami, Arai and Yamaha2010). At the blastula stage, 20–30 marginal blastomeres were extracted with a glass needle from hybrid embryos co-injected with GFP-nos3 3′UTR mRNA and GFP-Bucky ball mRNA, and transplanted into the marginal part of a goldfish blastula. At the time of operation, donor cells were checked as to whether or not they included a blastomere expressing GFP-Bucky ball using a fluorescence microscope. Transplanted embryos with a GFP-Bucky ball signal are referred to as blastomere transplanted chimeras (BT chimeras). Post-transplantation, embryos were cultured as described above. Chimeric embryos were observed and photographed using a Leica MZ16F fluorescence stereomicroscope equipped with a digital camera (Leica DFC300FX). Images of the embryos were obtained using filters appropriate for GFP.
Ploidy analysis
The relative DNA content of control goldfish, hybrids, several types of chimeric embryos and larvae, and cells from several organs was measured by FCM. According to the animal genome size database, goldfish have 3.58 pg of DNA per cell, while HR cells have 2.73 pg (http://www.genomesize.com). This analysis was performed in accordance with Tanaka et al. (Reference Tanaka, Yamaha and Arai2004).
Genetic analysis
To detect hybrid cells, recombination activating gene 1 (rag1) was used as a genetic marker. Species-specific primers were designed for the rag1 genes of goldfish (DQ196520) and golden venus chub (HM224045) (Table S1).
DNA samples were extracted from cell suspensions after FCM analysis, embryos, and tissue samples, using the alkaline lysis method. Briefly, embryos or small pieces of tissue were immersed in 180 µl of 0.1 N NaOH solution at 95°C for 10 min and then mixed with 20 µl of Tris–HCl solution (pH 8.0). After gentle mixing and centrifugation at 12,000 g for 10 min, supernatants were used as template DNA for PCR analysis.
PCR reactions contained 5 μl of Quick Taq HS DyeMix (TOYOBO), 0.2 μl of each primer, 1 μl of template DNA (50–100 ng/μl) and 3.2 μl of water, for a total volume of 10 μl. PCR was conducted in a Thermal Cycler (TaKaRa Bio Inc.) and consisted of an initial denaturation step of 120 s at 94°C, followed by 40 cycles of 30 s at 94°C, 30 s at the primer-specific annealing temperature, and 60 s at 68°C. Amplified products were separated by 1.5% agarose gel electrophoresis and stained with ethidium bromide.
Histology
Chimeric fish induced by transplantation of biotin-labelled hybrid blastoderm to goldfish were fixed at hatching stage with Bouin’s fixative for over 3 h. Fixed embryos were embedded in paraffin and 8 µm sections were taken. Deparaffinized sections were treated with peroxidase-conjugated streptavidin and coloured with 3,3-diaminobenzidine (DAB), according to the manufacturer’s protocol (Histo-fine, SAB-PO(M), Nichirei).
Allo-triploid individuals and control goldfish were fixed at 1, 3, 7 and 10 weeks after fertilization to analyze gonadal differentiation. Ploidy was analyzed by sampling small fin pieces before fixation. After fixation, gonadal regions were trimmed from the whole body, embedded in paraffin, and then sectioned at 8 µm thickness. Deparaffinized sections were stained with haematoxylin and eosin.
Statistics
The data for developmental potential of the control goldfish and hybrid between female C. auratus and male H. rasborella are shown as mean ± standard deviation, and were analyzed using Student’s t-test or Welch’s t-test (p < 0.05).
Results
Developmental potential and genomic constitution of hybrids
Hybrid eggs cleaved normally into blastulae and started epiboly. The speed of epiboly in hybrid embryos was slightly slower than in goldfish (Fig. 1). Hybrid embryos began to die during epiboly, because of breakage of the enveloping layer (Table 1). Surviving embryos developed until around hatching stage (day 4), then most died because of malformation of the body, including oedema (Table 1 and Fig. 2). A few fish developed normally and survived until after yolk sac absorption (Fig. 2D). Survival rate of hybrid was significantly lower than that of the control. At the hatching stage, the ploidy status of surviving embryos was analyzed by FCM (Table 1 and Fig. S2). The DNA content of abnormal progeny was intermediate between that of goldfish and HR, indicating allo-diploidy of the hybrid, that is one haploid set of goldfish chromosomes and one haploid set of HR chromosomes. In contrast, the DNA content of normally developing progeny was the sum of the diploid goldfish and haploid HR genomes, indicating allo-triploidy of this type of hybrid. Normal progenies with a DNA content equivalent to the diploid goldfish genome were presumed to be gynogenetically developing autodiploid goldfish.

Figure 1. Development during epiboly of hybrid and goldfish control. (A) Hybrid between female goldfish C. auratus × male golden venus chub H. rasborella. (B) Goldfish control.
Table 1. Fertilization, survival and ploidy status of the control goldfish and hybrid between female C. auratus and male H. rasborella
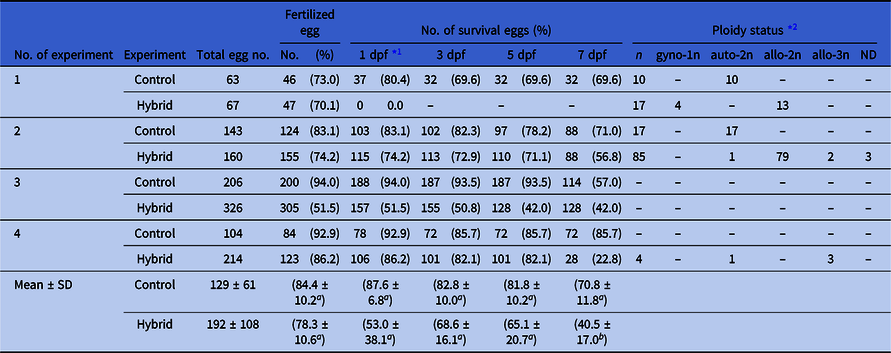
*1 dpf: days post-fertilization.
*2 gyno-1n: gynogenetic haploid goldfish, auto-2n: diploid goldfish, allo-2n: allo-diploid hybrid, allo-3n: allo-triploid. ND: Not detected.
Different superscript letters in each column except for the survival rate at 1 dpf indicate significant differences as determined using Student’s t-test (P < 0.05). There was no significant difference in the survival rate at 1 dpf as determined using Welch’s t-test (P < 0.05).

Figure 2. External appearance of goldfish control and hybrid. (A, B) Goldfish control. (C, D) Hybrid between female goldfish C. auratus and male golden venus chub H. rasborella at 4 days and 4 months post-fertilization, respectively. Hybrid fish (D) were induced by heat-shock treatment at 5 min after fertilization and allo-triploidy was confirmed by flow cytometry.
When genetic analysis was performed using species-specific PCR primers for the rag1 gene, abnormal progenies from the cross-fertilization between goldfish and HR were found to have both the 742-bp HR fragment and the 332-bp goldfish fragment (Fig. 3). These results genetically confirm that the abnormal progeny were hybrid, as they had both the goldfish and HR genome.

Figure 3. Genetic analysis of rag1 gene in hybrid progeny of female goldfish C. auratus and male golden venus chub H. rasborella. Lanes: (1) goldfish female, (2, 3) control goldfish progeny, (4–6) allo-diploid hybrids, (7–9) allo-triploid hybrids, (10) golden venus chub male, (11) negative control. MM indicates molecular size marker.
To induce allo-triploids, heat shock was performed 5 min after fertilization (Tables 2 and S2). These experiments produced externally normal progeny (data not shown). Allo-diploidy and allo-triploidy were identified in individuals sampled at 7 dpf by FCM (Tables 2 and S2). After ploidy determination of sampled individuals, remaining progeny were fed. They survived for over 4 months after hatching (Fig. 2D). In surviving progeny that appeared normal, ploidy status was determined by FCM at 1, 3, 7, and 10 weeks after hatching, at the same time as samples were taken for histological observations of the gonad. Almost all progeny were found to be allo-triploid hybrids, except for one autotriploid (i.e. triploid goldfish), and no allo-diploid hybrids occurred.
Table 2. Fertilization, survival, and ploidy status of control goldfish C. auratus and hybrid between female goldfish and male golden venus chub H. rasborella after heat-shock (HS) treatments at 40°C starting 5 min post-fertilization

*1 dpf: days post-fertilization.
*2 gyno-1n: gynogenetic haploid goldfish, auto-2n: diploid goldfish, allo-2n: allo-diploid hybrid, allo-3n: allo-triploid. ND: Not detected.
Survival of allo-diploid cells under chimeric conditions
To confirm the viability of hybrid cells, blastomere transplantations were performed into goldfish (Fig. S1). These transplantations occurred at the blastula stage and generated: a sandwich chimera, in which the lower part of the hybrid blastoderm was transplanted into the middle of a goldfish blastula; a hybrid-base chimera, in which goldfish blastoderm was transplanted onto the animal part of a hybrid blastula; and a goldfish-base chimera (Fig. 4A), in which hybrid blastoderm was transplanted onto the animal part of a goldfish blastula. The survival rates of these chimeras were drastically increased when compared with allo-diploid hybrids between goldfish and HR, and to both intact and dechorionated controls (Table 3). Moreover, at the hatching stage, some normal progeny appeared within these chimeric experiments. When observed under a fluorescent microscope, hybrid cells were mainly distributed around the anterior part of goldfish-base chimera (Fig. 4 C1), but were found in all parts of the hybrid-base (Fig. 4 D1) and sandwich chimeras (Fig. 4 E1). Histological analysis revealed that hybrid cells were distributed through all three germ layers and the organs containing more hybrid cells than host goldfish cells showed a tendency to be malformed (Fig. 4F, G). In particular, muscle fibres were less differentiated in myomeres that contained many hybrid cells (Fig. 4 G2).

Figure 4. Development of chimeric fish. Chimeric fish in which blastoderm grafts from hybrid female goldfish C. auratus × male golden venus chub H. rasborella were transplanted to goldfish blastula. (A) Immediately after transplantation of the entire hybrid blastoderm onto the upper part of a host goldfish blastula. (B) Control goldfish progeny at 3 dpf. (C) Goldfish-base chimera at 3 dpf. (D) Hybrid-base chimera at 3 dpf. (E) 3-day-old sandwich chimera. (C1, D1, E1) Fluorescent images. (B, C2, D2, E2) Brightfield images. (F, G) Histo-chemical analysis of 3-day-old goldfish-base chimeric embryos; head and trunk region, respectively. Brown cells originated from hybrid blastomeres.
Table 3. Increased survival and occurrence of normal progeny under chimeric conditions in hybrid between female goldfish C. auratus and male golden venus chub H. rasborella

When the entire blastoderm of a hybrid was transplanted onto a goldfish host blastula, five of these goldfish-base chimeric fish survived for over 6 months and one such individual had a tumour on its head (Fig. 5). In these chimeras, the persistence of hybrid cells was confirmed by FCM and by genetic analysis, as described above. When we analyzed the tissue samples from fin, muscle, skin, brain, eye and tumour, hybrid peak was not detected. When PCR analysis was conducted in the tissue samples used for FCM, HR-specific fragment was detected exclusively in the brain and both eyes of the single chimera possessing a tumour (Fig. 6).

Figure 5. External appearance of control goldfish and progeny from chimeric embryos 3 months after operations. (A) Control goldfish. (B1) Experimental fish that developed from a chimeric embryo in which hybrid blastoderm was transplanted to a goldfish blastula. (B2) Higher magnification of white rectangles in (B1).

Figure 6. Genetic analysis of rag1 gene in fish B2 (Fig. 5). (1) Fin of goldfish control. (2) Fin, (3) left eye, (4) right eye, and (5) brain of chimera. (6) Fin of golden venus chub H. rasborella. Both goldfish and H. rasborella specific bands are detected in the samples from the chimeric progeny.
Germ cell differentiation
When an artificial mRNA comprising GFP and the 3′UTR region of zebrafish nos3 (a germplasm specific RNA) was injected into hybrid zygotes immediately post-fertilization, cells with bright GFP fluorescence were observed after epiboly (Fig. 7). These bright GFP cells were detected even in embryos that were seemingly dead during epiboly (Fig. S3). Histologically, PGCs were detected in the genital anlage of newly hatched hybrids.

Figure 7. GFP fluorescence of PGCs in hybrid between female goldfish C. auratus × male golden venus chub H. rasborella. (A) 24 h, (B) 48 h and (C) 72 h after fertilization. GFP-positive cells are located around the presumptive gonadal region, suggesting that hybrid PGCs have the ability to migrate to the genital ridge. Scale bars, 1 mm. Yellow squares indicate lower columns of each picture.
As viable allo-triploid progeny were obtained using heat-shock treatment, germ cell differentiation could be histologically analyzed (Fig. 8). Similar to control goldfish (Fig. 8A), single PGCs were detected in the genital anlage of allo-triploid hybrids at 1 week post-fertilization (wpf) (Fig. 8B). Allo-triploid PGCs were encased in somatic cells and proliferated in the gonad at 7 wpf (Fig. 8D, E), as determined by the similar histological appearance of controls (Fig. 8C). At 10 wpf, a small allo-triploid fish (12 mm in body length) had a similar appearance to that of control fish at 7 wpf (Fig. 8F, G), while allo-triploid progeny over 24 mm long had abnormal gonads with large vacuolar spaces (Fig. 8H). These spaces were similar in size to germ cells in control gonads.

Figure 8. Histological sections of gonads from goldfish and hybrids. (A, C, F) Control autodiploid goldfish. (B, D, E, G, H) Allo-triploid hybrid between C. auratus and H. rasborella. (A, B) 1 week post-fertilization. (C–E) 7 weeks post-fertilization. (F–H) 10 weeks post-fertilization. Yellow arrowheads indicate germ cells; black arrowheads indicate intercellular spaces of a similar size to germ cells. Black arrow indicates the ovarian cavity.
When blastomeres including PGCs were transplanted from allo-diploid hybrids into goldfish blastulae (n = 53), about half of these chimeric embryos survived until 10 dpf (Table S3). GFP-positive cells were detected on the usual migration route of PGCs and in ectopic regions in the chimeric embryos (11.3%) at 1 dpf (Fig. S4 and Table 4). The frequencies of embryos with GFP-positive cells decreased over time, and all GFP-positive cells had disappeared by 10 dpf (Table 4). This indicates that PGCs from allo-diploid hybrids were not able to persist, even under chimeric conditions.
Table 4. Number of PGCs from goldfish × HR embryo in host goldfish × goldfish during development

Discussion
Inviable allo-diploid and viable allo-triploid hybrids
In the present study, allo-diploid hybrid between goldfish eggs fertilized with HR sperm died during embryonic and pre-larva. Survivors were also verified to be either allo-triploid hybrids with two sets of goldfish chromosomes and one set of HR chromosomes, or gynogenetically developed diploid goldfish. Therefore, allo-diploid hybrids with a maternally derived haploid set of goldfish chromosomes and a paternally derived haploid set of HR chromosomes were inviable and died soon after hatching, while allo-triploid hybrids (which sporadically occurred) survived. The occurrence of spontaneous viable allo-triploid hybrids has often been reported in crosses of Ctenopharyngodon idella × Hypophthalmichthys molitrix (Márián and Krasznai, Reference Márián and Krasznai1978), and Oncorynchus mykiss × Salvelinus fontinalis (Capanna et al., Reference Capanna, Cataudella and Volpe1974; Ueda et al., Reference Ueda, Ojima, Sato and Fukuda1984). It is rare that heterospecific fertilization gives rise to the occurrence of spontaneous gynogenesis, however the occurrence of goldfish after hybridization indicates presumable ploidy elevation. This may occur by inhibition of the second polar body release or endomitosis in cleavage, followed by the initiation of spontaneous gynogenesis triggered by heterospecific sperm. Mechanisms underlying such events are presently unknown, but similar cases of spontaneous gynogenesis have been reported in hybrid Oncorhynchus kisutch × S. fontinalis (Uyeno, Reference Uyeno1972), Pleuronectes platessa × Platichtys flesus (Purdom and Lincoln, Reference Purdom, Lincoln and Blaxter1974) and Ctenopharyngodon idella × Cyprinus carpio (Stanley, Reference Stanley1976).
In certain salmonid hybrids, inviable development has been explained by the occurrence of aneuploidies due to the elimination of chromosomes (Arai, Reference Arai1984; Fujiwara et al., Reference Fujiwara, Abe, Yamaha, Yamazaki and Yoshida1997) or by no, weak or delayed expression of paternal genes in inviable hybrid between Oncorhynchus keta female and O. masou male (Arai, Reference Arai1984). In the present study, goldfish × HR hybrids were allo-diploid, and comprised a haploid chromosome set from each parental species and therefore inviability of this hybrid cannot be explained by aneuploidy. This result indicates there were no harmful cytological effects, like chromosome elimination, on the HR genome in the development of resultant hybrids; however, maternal cytoplasm and/or genome may suppress the expression of certain paternal HR genes during early development. As most allo-diploid hybrids die in burst during epiboly, hybrid conditions may affect cytoskeleton of enveloping layer cells in blastoderm. To elucidate probable nucleo-cytoplasmic effects, the analysis of an interspecific androgenetic doubled haploid is required.
In our cross-fertilization experiment, allo-diploid hybrids died in the early stages of embryogenesis, while sporadically occurring allo-triploid hybrids showed better survival and were viable. We were also able to easily induce allo-triploidy by heat shock – which inhibits the release of the second polar body – soon after fertilization of eggs with heterospecific sperm. Increased survival of allo-triploids has been well-studied in salmonid hybrids, including O. mykiss (female) × Salmo trutta (male), O. mykiss × S. fontinalis (Chevassus et al., Reference Chevassus, Guyomard, Chourrout and Quillet1983; Scheerer and Thorgaard, Reference Scheerer and Thorgaard1983), Oncorhynchus keta × S. fontinalis, O. keta × S. leucomaenis (Arai, Reference Arai1984, Reference Arai1986) and Oncorhynchus gorbuscha × S. leucomaenis (Yamano et al. Reference Yamano, Yamaha and Yamazaki1988). This increased viability is presumably caused by doubling of the female genome. Thorgaard (Reference Thorgaard, Hoar, Randall and Donaldson1983) suggested that a decrease in the ratio of paternally derived genes might relate to the increased viability of allo-triploids. However, if the paternal genome positively inhibited the normal development under allo-diploid genomic condition, female genome doubling is not sufficient to recover the developmental potential of hybrids (Yamano et al., Reference Yamano, Yamaha and Yamazaki1988). Here, we conclude that the HR genome is not harmful to development under allo-diploid genomic conditions, but partially suppressed in their expression, because allo-triploid hybrids developed beyond hatching and feeding stages.
Survival of goldfish-hybrid chimera
Doubling of the maternally derived genome gives rise to increased survival in certain combinations of hybrids (Chevassus et al., Reference Chevassus, Guyomard, Chourrout and Quillet1983; Scheerer and Thorgaard, Reference Scheerer and Thorgaard1983; Arai, Reference Arai1984, Reference Arai1986; Yamano et al., Reference Yamano, Yamaha and Yamazaki1988). In addition to analyze the effect of allo-triploidization on the survival of goldfish × HR hybrids, we examined the survival potential of somatic cells from inviable allo-diploid hybrids by experimental induction of interspecific chimera. When allo-diploid hybrid cells were transplanted into host goldfish embryos, hybrid cells could be tracked in 6-month-old individuals under chimeric conditions. Some of the chimeras that included hybrid cells had better survival rates than intact allo-diploid hybrids. Therefore, we propose that insufficiencies in developmental potential of the hybrid cells are mitigated under the chimeric conditions. To our knowledge, this is the first report to show survival recovery of inviable allo-diploid hybrid cells in the chimeric condition.
A similar phenomenon has been reported in haploid–diploid goldfish chimera (Tanaka et al., Reference Tanaka, Yamaha and Arai2004), in which haploid cells were able to survive under chimeric conditions. When haploid blastoderm was transplanted to the animal region of diploid goldfish, the resultant haploid–diploid chimeric goldfish survived to adult size (Tanaka et al., Reference Tanaka, Yamaha and Arai2004). Therefore, the poor viability of haploid blastoderm was recovered by transplantation onto diploid blastoderm and yolk cells. In our study, although a large number of chimeras in which hybrid blastomeres were contained in the lower, middle or upper part of host blastulae had an abnormal appearance, a few developed normally. This suggests that the poor viability of hybrid was recovered under mixed condition with diploid cells. However, histological observation revealed that an increase of hybrid cells often induced higher abnormality in tissue structures. Furthermore, in allo-diploid hybrid–goldfish chimeras, hybrid cell-rich myomeres were generally undifferentiated, compared with those with normal diploid goldfish cells. These results suggest the presence of a community effect, because cell populations comprising more hybrid cells were more abnormal.
Here, in PCR analysis, hybrid cells were not detected in any tissues of 6-month-old chimeric individuals, except for brain and both eyes of a single chimeric fish. Cell lineage analysis using fluorescent label tracking showed that donor hybrid cells were mainly distributed around the head region of hatched larvae. Somatic cells are constantly replaced by new cells over time. If hybrid cells have weaker and/or lower proliferative potential than host cells, then they are destined to be lost during development. Alternatively, as the nerve cells of the central nervous system terminally differentiate at an early stage of embryonic development, hybrid cells may persist in such tissues.
PGC differentiation and the reproductive potential of allo-triploid hybrids
PGCs were identified histologically in the gonadal anlage of surviving hybrid larvae at around hatching, and visualized using GFP-nos3 mRNA in hybrids that died at epiboly. In many teleosts, PGCs are destined by maternally supplied cytoplasm that clustered at the ends of early cleavage furrows (zebrafish, Yoon et al., Reference Yoon, Kawakami and Hopkins1997; goldfish, Otani et al., Reference Otani, Maegawa, Inoue, Arai and Yamaha2002; dojo loach, Fujimoto et al., Reference Fujimoto, Kataoka, Sakao, Saito, Yamaha and Arai2006; Atlantic salmon Nagasawa et al., Reference Nagasawa, Femandes, Yoshizaki and Babiak2013; sturgeon, Saito et al., Reference Saito, Pšenička, Goto, Adachi, Inoue, Arai and Yamaha2014; turbot, Lin et al., Reference Lin, Xu, Ma, Xiao, Zhao, Xiao, Chi, Liu and Li2012; barfin flounder, Goto et al., Reference Goto, Saito, Kawakami, Kitauchi, Takagi, Todo, Arai and Yamaha2015). It is therefore reasonable to expect that blastomeres with maternal germplasm would be able to differentiate into PGCs, even in inviable hybrids. However, we observed that hybrid PGCs transplanted into goldfish blastulae disappeared in host embryos by 10 dpf, while somatic cells survived. Usually visualized PGCs with GFP-nos3 mRNA are able to be traced at least 1 month in goldfish. Notably, even in xeno-transplantation, donor PGCs from different species do not always survive and differentiate into gametes in the host species (Saito et al., Reference Saito, Goto-Kazeto, Arai and Yamaha2008, Reference Saito, Pšenička, Goto, Adachi, Inoue, Arai and Yamaha2014). Therefore, whether hybrid PGCs are autonomously inviable remains unclear.
We were able to observe germ cells of allo-triploid hybrids until 7 wpf, after which they disappeared from the gonads. As gonads were abundant with vacuoles at 10 wpf, it is likely that the PGCs had undergone apoptosis. This result suggests that allo-triploid PGCs, with two goldfish genomes and one HR genome, are as inviable as allo-diploid PGCs; however, allo-triploid PGCs survived longer than allo-diploid PGCs, and proliferated in the gonad. Therefore, an increase in maternal genome in hybrid PGCs improved their proliferative potential.
In conclusion, in the present study, allo-diploid hybrids between goldfish females and HR males were inviable, however the cells from these inviable hybrid embryos could survive in chimeric environment together with autodiploid cells of maternal species. The elevation of the maternal genome by allo-triploidization could also recover subsequent differentiation. Many factors and their interaction, such as that between two non-homologous genomes and that between maternal cytoplasm and paternal genome, should play roles in embryonic development of allo-diploid, allo-triploid and interspecific chimeras. Even for autodiploid development, it is obscure whether maternal and paternal genomes have different epigenetic programmes (Labbé et al., Reference Labbé, Robles and Herraez2017). It is very difficult to analyze these interactions precisely. To analyze possible incompatibility between maternal and paternal genomes, homogeneous gametes should be required in both species to produce isogenic hybrids. Therefore, to analyze interactions between maternal cytoplasm and paternal genetic materials, interspecific androgenetic diploid (cybrids) should be analyzed. Neither allo-diploid nor allo-triploid PGCs could differentiate into functional gametes, suggesting that additional HR genomes did not bring any harmonious interaction to maternal genome for further differentiation. To reveal this point, the allo-tetraploid (amphidiploid) condition should be analyzed in the near future. Chromosome set manipulation and chimeric induction are powerful techniques to answer such questions. Genomic combination involved in gamete differentiation of hybrids should give insights into genetic breeding and biological evolution.
Supplementary material
To view supplementary material for this article, please visit https://doi.org/10.1017/S0967199420000349
Acknowledgements
We would like to express our gratitude to the members of the Nanae Fresh-Water Laboratory, Field Science Center for Northern Biosphere and the Laboratory of Aquaculture Genetics and Genomics, Faculty and Graduate School of Fisheries Sciences, Hokkaido University for assistance with obtaining experimental fish, conducting the experiments and discussing the results.
Financial support
This study was supported in part by Grant-in-Aid for Scientific Research from Japan Society for the Promotion of Science to KA (KAKENHI 15H02457).
Conflicts of interest
None.
Ethical standards
This study was carried out in accordance with the Guide for the Care and Use of Laboratory Animals in Hokkaido University and Field Science Center for Northern Biosphere, Hokkaido University (approval ID: 22-1).