CHD is the most frequent malformation present at birth. Nearly 10% of CHD belong to the group of functionally univentricular hearts. Reference Kaulitz and Hofbeck1
In last decades, the natural fatal course of univentricular hearts has been dramatically improved by surgical univentricular palliation, Reference Driscoll2 which delivers the total systemic venous return to the lungs, by-passing the heart, through a passive filling. Operative mortality of the Fontan procedure has steadily decreased, and it is currently <2% in the best centres, which is no higher than for many biventricular repairs. Reference Fontan and Baudet3 However, early post-operative morbidity is still high and predicting risk factors is still challenging. Reference De Leval4
Even though for many years routine cardiac catheterisation has been considered mandatory in pre-Fontan assessment, recently cardiac MRI has emerged as a useful and alternative non-invasive imaging in selected patients, without adversely affecting the outcome. Reference De Leval and Deanfield5–Reference Ro, Rychik, Cohen, Mahle and Rome7 One of the main indications to perform routine catheterisation before Fontan intervention is the evaluation of end-diastolic ventricular pressure and pulmonary vascular resistance, which represent important variables in patients with functionally single ventricle. Reference Martin, McBrien, Marchak, Atallah, Al Aklabi and Mackie6 Moreover, end-diastolic ventricular pressure is a determinant of central venous pressure in patients with a Fontan circulation. Reference Prakash, Khan, Hardy, Torres, Chen and Gersony8
In this study, we sought to investigate clinical and MRI predictors of high end-diastolic ventricular pressure at pre-Fontan catheterisation. The second aim was to investigate the impact of high end-diastolic ventricular pressure on post-operative Fontan outcome.
Materials and methods
Population study
From our cardiac MRI database, we identified all patients with functionally univentricular heart, potential candidates for Fontan intervention. Patients who underwent pre-Fontan cardiac catheterisation, beside a comprehensive MRI, echocardiographic, and clinical assessment were included in the study. Patients with antegrade pulmonary flow (native pulmonary valve still patent) were also excluded. The medical history, surgical and catheterisation procedures, and clinical data were retrospectively abstracted from hospital records. An adverse post-Fontan outcome was defined as the occurrence of one or more of the following events: prolonged pleural drainage (>15 days), prolonged ICU length of stay (LOS) >10 days, cardiac catheterisation within a 3-month period after the operation Reference Martin, McBrien, Marchak, Atallah, Al Aklabi and Mackie6 and multi-organ failure.
The Institutional Review Board of our hospital approved this study.
Echocardiography
The following echocardiographic parameters were taken into account: distal aortic arch obstruction; atrio-ventricular valve regurgitation categorised as none/trivial, mild/non-significant, moderate, or severe, based on qualitative evaluation. Similarly, systemic ventricular function was qualitatively graded as normal, moderately, or severely depressed.
MRI
All examinations were performed using a Signa/GE CV/I 1,5 T scanner (maximal gradient = 40 mT/m, slew rate = 150 mT/m/second or 3T scanner (Ingenia, Philips Medical Systems, the Netherlands). A comprehensive MRI evaluation was performed following a previously published dedicated protocol. Reference Ait-Ali, De Marchi and Lombardi9 In particular, ECG-gated phase-velocity-contrast free-breathing sequences, perpendicular to ascending aorta, pulmonary arteries, systemic and pulmonary vein (Fig 1a) flow, were also assessed. The examination was performed in deeply sedated patients employing titrated propofol, and sequences were acquired as free breathing.

Figure 1. ( a ) Volume rendering reconstruction posterior view and schematic illustration of systemic and pulmonary flow acquisition. ( b ) Antero-posterior X-Ray angiography of the same patient AO: aorta; IVC: inferior vena cava; LPA: left pulmonary artery; LLPV: left lower pulmonary veins; LUPVs: left upper pulmonary veins; RLPVs: right lower pulmonary veins RPA: right pulmonary artery; RUPVs: right upper pulmonary veins; SVC: superior vena cava.
All MRI studies were off-line analysed using a workstation (Advantage Workstation; GE Healthcare) with a dedicated software (MASS 6.1, Medis, Leiden, The Netherlands). Pulmonary and systemic arterial and venous flows were measured by the analysis of velocity encoded phase contrast images and were indexed to body surface area. Systemic-pulmonary collaterals flow (QAPCs) was calculated as: (left pulmonary veins flow + right pulmonary veins flow) − (right pulmonary artery flow + left pulmonary artery flow). Values were normalised to body surface area.
Effective cardiac index was calculated as (QAo flow – QAPCs)/BSA. Reference Ait-Ali, De Marchi and Lombardi9 Ventricular volumes, ejection fraction, and mass were measured by manually tracing the endocardial and epicardial border at end ventricular diastole and systole using cine short-axis image. The left and right pulmonary artery diameters and areas were measured from MRA or 2D-TOF. Normative diameters of main pulmonary arteries against body surface area (z-scores) were calculated as well. Reference Gewillig and Brown10
The Nakata index Reference Ait Ali, Cadoni, Rossi, Keilberg, Passino and Festa11 and McGoon index Reference Ait Ali, Cadoni, Rossi, Keilberg, Passino and Festa11 were also, respectively, assessed as follows: left pulmonary artery area + right pulmonary artery area/body surface area; left pulmonary artery diameter + right pulmonary artery diameter/distal descending aorta diameter.
Cardiac catheterisation
All procedures were performed using the Philips Allura H5000C Monoplane with the × Ray tube MRC 200 0508 ROT GS 1001. The anatomy of the pulmonary branches (Fig 1b), aorta, superior and inferior caval vein, and the aortic arch were evaluated by X-ray angiography in appropriate planes. Ventriculography was also performed. The pull-back pressures were recorded: from ventricles to descending aorta, from PAs to superior caval vein, and from atria to inferior caval vein. According to the literature, the mean pulmonary pressure and end-diastolic ventricular pressure were considered “high” if, respectively, >15 mmHg and >10 mmHg. Reference Kaulitz and Hofbeck1,Reference Lehner, Schuh and Herrmann12 Pulmonary vascular resistance and Mayo clinic index were calculated. Pulmonary vascular resistance was calculated by the following formula (mean pulmonary artery pressure − pulmonary capillary wedge pressures)/pulmonary blood flow (estimated by the Fick principle). The Mayo clinic index was calculated Reference Mair, Hagler, Puga, Schaff and Danielson13 adding pulmonary artery resistance to end-diastolic ventricular pressure divided by pulmonary blood flow plus systemic blood flow, respectively, indexed to body surface area and it was considered favourable if <4; the Nakata index Reference Nakata, Imai and Takanashi14 and McGoon index Reference Piehler, Danielson, McGoon, Wallace, Fulton and Mair15 were also assessed as indicated above. According to the literature a Nakata index <250, Reference Knobel, Kellenberger, Kaiser, Albisetti, Bergsträsser and Valsangiacomo Buechel16 a McGoon index <1.8 Reference McGoon, Baird and Davis17 and pulmonary vascular resistance >4 UW*m2 were also considered as risk factors for adverse post-Fontan outcome. Reference Stern18
All procedures were performed under general anaesthesia and endotracheal intubation. Any interventions, as aorto-pulmonary or veno-venous collaterals coil occlusion, or aortic recoarctation balloon angioplasty were recorded, such as intra- and post-procedural complications.
Statistical analysis
Continuous variables were expressed as mean ± standard deviation or median (interquartile [IQ] range 25th–75th percentiles) if skewed. Categorical variables were expressed as absolute frequencies and a percentage. The correlation between continuous variables was tested with Pearson’s correlation r coefficient or Spearman when indicated. Comparisons between two groups were performed by independent samples t test, in the homoscedastic or heteroscedastic form as appropriate. Receiver operating characteristic analysis was performed and area under the curve was calculated. The best cut-off of end-diastolic ventricular pressure associated with post-Fontan complications was determined according to the maximum value of Youden index. For the association between traditional cardiac catheterisation criteria and adverse Fontan outcome, the χ2 test or the Fisher exact test was performed as appropriate. The non-invasive predictors of end-diastolic ventricular pressure were evaluated using univariate logistic regression. Significant variables at the univariate analysis were included in a multivariate logistic regression model with backward stepwise selection. Association was evaluated in term of odds ratio (OR) and 95% confidence intervals (CI). All statistical tests with a two-tailed p < 0.05 were considered statistically significant. All analyses were performed using SPSS (version 21.0).
Results
Patients characteristics
From the 60 patients with comprehensive MRI and cardiac catheterisation pre-Fontan evaluation, 22 patients were excluded, 18 due to the presence of pulmonary antegrade flow, 4 because pre-Fontan cardiac catheterisation was not performed. Therefore, 38 patients (mean age 4.8 ± 2.4 years, 71.1% males) constituted the final study population (Fig 2). The most frequent diagnosis was Hypoplastic Left Heart Syndrome (31.5%), followed by tricuspid atresia (26.3%). Sixteen patients (42.1%) had right morphology single ventricle. Six patients had a persistent left superior vena cava, none of them underwent surgical vessel ligation.

Figure 2. CONSORT diagram showing the patients enrolled in the study CC: cardiac catheterization.
Patient characteristics, diagnosis, and surgical data are summarised in Table 1.
Table 1. Population demographic, history, and clinical data

Continuous variables are expressed as mean ± standard deviation or median and 25°;75° IQ.
Pre-Fontan evaluation
At echocardiography, most patients (92.1%) had a normal ventricular function; 10 patients (26.3%) had moderate or severe regurgitation of at least one atrio-ventricular valve. Due to poor acoustic windows, either one or both pulmonary arteries in 27 patients (71%) could not be imaged adequately, as well as the Glenn anastomosis in 13 patients (34.2%).
At MRI study, the median end-diastolic volume was 97 (88; 117) ml/m2 with a mean ejection fraction 58.6 ± 8.7% and mean effective cardiac index 2860.2 ± 632.6 L/minute/m2. Flow was higher in right pulmonary artery than in left pulmonary artery (1049.1 ± 352.5 ml/m2 versus 518.6 ± 326.5 ml/m2, respectively), as well as the areas, respectively, 1.2 ± 0.6 cm2/m2 versus 0.8 ± 0.5 cm2/m2. Moreover, in 22 patients (57.9%), at least one pulmonary branch was hypoplastic (z-score < 2). MRI data are summarised in Table 2.
Table 2. Pre-Fontan imaging findings
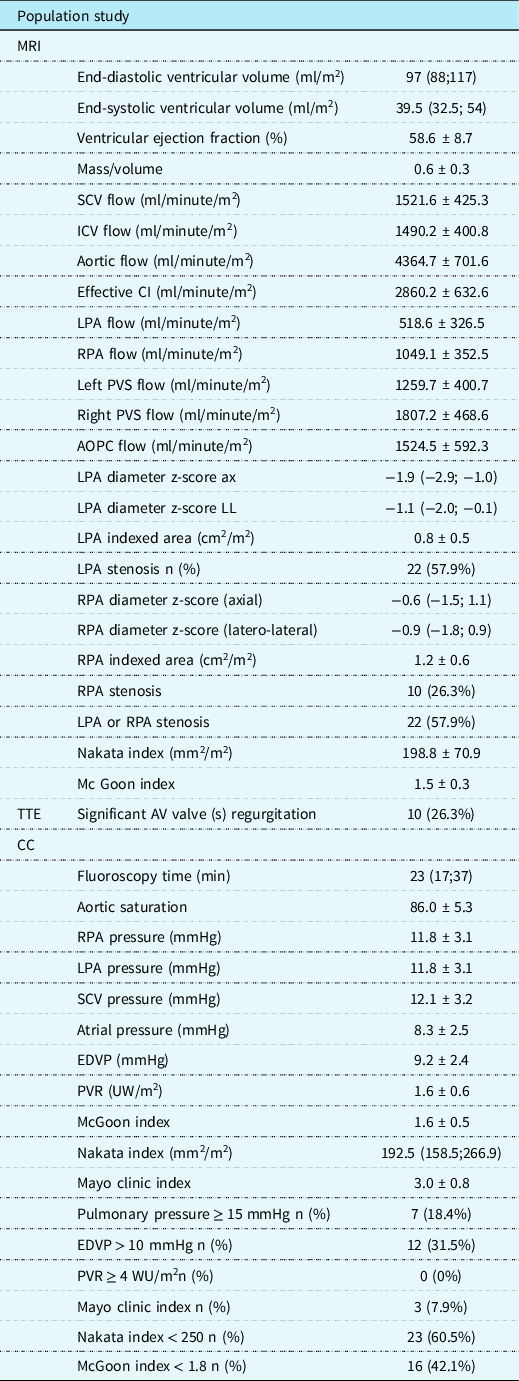
Continuous data are expressed as mean ± SD and or median and IQ.
AOPC = aorto-pulmonary collaterals; ASD = atrial septal defect; AV = atrioventricular; CC = cardiac catheterisation; CI = cardiac index; EDVP = end-diastolic ventricular pressure; ICV = inferior caval vein; LPA = left pulmonary artery; Legend: PV = pulmonary veins; PVR = pulmonary vascular resistance; PVS = pulmonary veins; RPA = right pulmonary artery; SCV = superior caval vein; SV = systemic veins; TTE = transthoracic echocardiography.
At the pre-Fontan cardiac catheterisation, the mean aortic saturation was 86 ± 5.3%, end-diastolic ventricular pressure was 9.1 ± 2.4 mmHg, pulmonary pressure 11.8 ± 3.1 mmHg, pulmonary vascular resistance 1.6 ± 0.6 UW * m2; 27 patients presented at least one risk factor for Fontan: unfavourable Nakata and McGoon indexes were the most frequent (Table 2), whereas no patient had pulmonary vascular resistance ≥4 UW * m2. In only one patient, occlusion of the femoral artery was recorded. The main haemodynamic results are summarised in Table 2.
Catheter interventions were performed in 11 patients: two aortic re-coarctation balloon angioplasty, five veno-venous collaterals embolisation, two systemic-pulmonary collaterals embolisation, and two left pulmonary artery balloon angioplasties.
Post-operative outcomes
No patient was excluded from Fontan intervention after the evaluation. Four patients are awaiting Fontan at the time of writing. Thirty-four patients underwent Fontan intervention, at a mean age of 5.5 ± 2.3 years, 28 of them (82.3%) with an extra-cardiac conduit. In 20 patients (52.6%), the Fontan was fenestrated (Table 3).
Table 3. Fontan intervention and post-operative outcome

Continuous data are expressed as mean ± SD and or median and IQ.
Median ICU length of stay was 3 days (IQR:2;5); median duration of pleural drainage was 8 days (IQR: 6;12).
At least one complication occurred in eight patients (23.5%): six patients required long (>15 days) thoracic drainage or a new thoracic drainage insertion; in one patient, percutaneous fenestration was performed and one patient had multi-organ failure.
Regarding the traditional cardiac catheterisation risk factors, early post-operative complication was associated with higher end-diastolic ventricular pressure and Mayo clinic indexes scores (respectively p < 0.01 and p = 0.05), whereas the association with the others cardiac catheterisation risk factors was not statistically significant (Table 4).
Table 4. Association between conventional invasive indexes and Fontan outcome

The statistically significant values are expressed with boldface character.
DVP = end-diastolic ventricular pressure.
Moreover, in the patients who experienced a post-Fontan adverse outcome, the end-diastolic ventricular pressure was higher in comparison with the remaining population, respectively, 8.4 ± 2.0 versus 10.8 ± 2.6 mmHg, p < 0.05.
At receiver operating characteristic curve analysis, end-diastolic ventricular pressure ≥ 10.5 mmHg predicts post-op complications with a sensitivity of 75% and specificity of 88%. (AUC: 0.795, 95% CI 0.576;1.000, p < 0.05).
Non-invasive risk factors for higher end-diastolic ventricular pressure
End-diastolic ventricular pressure inversely correlates with MRI McGoon index (r: −0.52, p < 0.01) and SVC indexed flow (r: −0.40 p < 0.05). Moreover, end-diastolic ventricular pressure was higher in patients with systemic right ventricle than in patients with left systemic or two ventricles (10.3 ± 2.6 versus 8.4 ± 2.1 mmHg, p < 0.05) and in patients with at least one pulmonary branch stenosis than in patients with normal pulmonary branches caliper (9.8 ± 2.3 versus 8.2 ± 2.5 mmHg, p < 0.05).
In patients with end-diastolic ventricular pressure ≥ 10.5 mmHg, the MRI McGoon, Nakata index, and indexed superior caval vein were lower in comparison with the remaining population, respectively, 1.32 ± 0.4 versus 1.64 ± 0.24, p = 0.006; 167 ± 48 versus 218 ± 65 mm2/m2, p = 0.03; and 1250 ± 269 versus 1671 ± 426 ml/minute/m2, p = 0.004 (Fig 3).

Figure 3. CMR McGoon Index, CMR Nakata index, SVC indexed flow comparison between EDVP groups (EDVP = end diastolic ventricular pressure; SVC = superior vena cava).
At univariate regression logistic analysis, systemic ventricle end-diastolic ventricular pressure ≥ 10.5 mmHg was associated with systemic morphologic right ventricle (OR: 20.000, 95% CI: 3.283–128.843, p < 0.01), MRI Nakata index (OR: 0.987, 95% CI: 0.973–1.000, p = 0.05), MRI McGoon index (OR: 0.032, 95% CI: 0.002–0.541, p < 0.05), superior caval vein indexed flow (OR: 0.996, 95% CI: 0.994–0.999, p < 0.05), and SVC/IVC flow ratio (OR: 0.049, 95% CI: 0.003–0.692, p < 0.05). Whereas at multivariate analysis, both systemic right ventricle (OR: 23.312, 95% CI: 2.704–200.979, p < 0.01) and superior caval vein indexed flow (OR: 0.996, 95% CI: 0.993–0.999, p < 0.05) influenced worse end-diastolic ventricular pressure (≥10.5 mmHg) (Table 5).
Table 5. Univariate and multivariate logistic regression analysis for EDVP > 10.5 mmHg

The statistically significant values are expressed with boldface character.
AV = atrioventricular; EDVV = end-diastolic ventricular volume; EF = ejection fraction; ESVV = end-systolic ventricular volume; HLHS = hypoplasic left heart syndrome; ICV = inferior caval vein; LPA = left pulmonary artery; PA = pulmonary artery; RPA = right pulmonary artery; RV = right ventricle; SCV = superior caval vein.
A second model was evaluated replacing the superior caval vein indexed flow by SVC/IVC flow ratio and both systemic right ventricle (OR: 83, 95% CI: 3,407–2021,819, p < 0.01) and SVC/IVC flow ratio (OR: 0.003, 95% CI: 0–0,56, p < 0.05) influenced worse end-diastolic ventricular pressure (≥10.5 mmHg).
Discussion
In our cohort of pre-Fontan patients, end-diastolic ventricular pressure ≥ 10.5 mmHg was associated with post-Fontan complications. Moreover, lower superior caval vein flow, Nakata and McGoon indexes evaluated by MRI and also by right morphology of the systemic ventricle influenced higher end-diastolic ventricular pressure.
Historically, risk stratification and assessment of suitability for the Fontan procedure have been based on several anatomic and haemodynamic variables. Reference Choussat, Fontan, Besse, Anderson and Shinebourne19 However, the emerging understanding of the “unnatural” physiology of the Fontan circuit led to focus particular attention to diastolic ventricular function.
In the absence of a pre-pulmonary pump and also in the context of in-series connection of the systemic and pulmonary vascular bed, as in Fontan circulation, the venous flow from the pulmonary vascular bed is reduced; this results in a decreased or absent preload reserve to the ventricle. In these hearts, preload is the most important determinant for cardiac output, and it is principally determined by passive trans-pulmonary flow, strictly dependent on several factors, particularly on pulmonary artery anatomy, pressure and resistance, and ventricular diastolic function. Reference Gewillig and Brown10
Diastolic dysfunction is usually the result of impaired ventricular relaxation and increased chamber stiffness, which increases cardiac filling pressures, Reference Nagueh, Smiseth and Appleton20 and it is demonstrated on the pressure volume loop, with ventricular pressures that do not decrease to expected normal values after isovolumic relaxation, and a greater-than-normal increase in ventricular pressure at end diastole despite normal or near-normal end-diastolic volumes. Reference Budts, Ravekes, Danford and Kutty21
The association we found between pre-Fontan end-diastolic ventricular pressure and post-operative complications, with a higher end-diastolic ventricular pressure (≥10.5 mmHg) predicting post-operative adverse effects with good sensitivity and specificity, stresses the weight of diastolic function on the early outcome post-Fontan palliation. Our results are in line with previous studies: Garofalo et al Reference Garofalo, Cabreriza and Quinn22 found a correlation between ventricular stiffness and duration of pleural effusions, net perioperative fluid balance, and hospital length of stay after Fontan palliation.
Despite its importance, diastolic function of the palliated single ventricle is poorly understood, and no-invasive risk factors associated with higher end-diastolic ventricular pressure have not yet been clearly identified in pre-Fontan patients. In 2018, Schwartz et al Reference Schwartz, Brock, Nykanen and DeCampli23 studied possible predictors of an elevated end-diastolic ventricular pressure in pre-Fontan patients, and they found that invasive ventricular systolic pressure and decreased ventricular systolic function were positively associated with end-diastolic ventricular pressure. In our knowledge, this is the first study that investigates non-invasive MRI predictors of pre-Fontan end-diastolic ventricular pressure.
In our study, end-diastolic ventricular pressure showed to be inversely correlated with superior caval vein indexed flow and a lower superior caval vein flow influenced higher end-diastolic ventricular pressure (>10.5 mmHg). Moreover, we demonstrated a significant association between higher end-diastolic ventricular pressure and hypoplasia of pulmonary branches, represented by a lower MRI McGoon index. The pathophysiologic correlation between diastolic dysfunction and pulmonary arteries hypoplasia needs to be investigated. However, it is known that at the time of the Glenn procedure, the single ventricle goes from a volume overloaded and hypertrophied state to a relatively underfilled, volume-deprived condition Reference Budts, Ravekes, Danford and Kutty21 ; also, the insufficient development of the pulmonary vasculature will result with high pulmonary vascular resistance, thus determining a chronic low flow condition, which could lead to chronic underfilling of the ventricle and resultant remodelling.
Another important factor that has largely been investigated is ventricular morphology. Although the intrinsic differences in adaptation between a morphologic single left or single right ventricle after Fontan palliation are not completely elucidated, differing right and left ventricular fibre arrangements could result in clinically significant variations in remodelling. Previous echocardiographic studies suggest that systemic right ventricles have impaired diastolic function compared to systemic left ventricles. Reference Kaneko, Khoo, Smallhorn and Tham24,Reference Tham, Smallhorn and Kaneko25 Moreover, two recent studies found that pre-Fontan end-diastolic ventricular pressure is lower in those with a systemic left ventricle. Reference Seckeler, O’Leary and Anitha Jayakumar26 This is in line with our results showing a higher end-diastolic ventricular pressure in patients with systemic right ventricle than in patients with left systemic or two ventricles although the high variability of the estimate (wide range of the confidence interval) in our population, likely because of the low sample of our population study.
Clinical implications
Cardiac MRI has recently emerged as a useful diagnostic tool and has gained widespread acceptance in the evaluation of pre- and post-operative CHD, allowing for the accurate evaluation of thoracic vascular anatomy, blood flow, ventricular volume and function, and myocardial viability. Reference Brown, Gauvreau and Powell27 However, MRI is not able to measure vascular resistance and pressures and standard for an accurate assessment of end-diastolic ventricular pressure in single ventricle patients remains cardiac catheterisation, although this is an invasive approach associated with morbidity and mortality, Reference Kiesewetter, Sheron and Vettukattill28 increasing the patient’s long-term risk of cancer because of the use of ionising radiation. Reference Ait-Ali, Andreassi and Foffa29
This highlights the importance of identifying non-invasive parameters that could correlate with invasive parameters, particularly with ventricular filling pressures, given their importance in single ventricle pathophysiology.
As already postulated by Prakash et al Reference Prakash, Khan, Hardy, Torres, Chen and Gersony8 and Ait-Ali et al, Reference Ait-Ali, De Marchi and Lombardi9 routine pre-operative invasive evaluation could be avoided in selected “low-risk” subjects, candidates for Fontan, performing a selection based on non-invasive evaluation with MRI.
Therefore, lower superior caval vein flow and McGoon index, both evaluated with MRI, might be considered within a panel of variables identifying a “high-risk” category of patients, who might necessitate further invasive evaluation and who might present worse post-operative outcome.
Further larger multi-centre studies with prospectively collected invasive haemodynamic data and non-invasive MRI parameters are needed to find a useful predictor cut off for McGoon index and superior caval vein or SVC/IVC flow ratio for higher end-diastolic ventricular pressure, therefore improving pre-Fontan selection for cardiac catheterisation.
Limitations
This is not a randomised study and presents all the limits of a retrospective, observational, single-centre study, with a relatively low sample size, characterised by high heterogeneity, due to the epidemiology and the variety of the CHDs referred to univentricular heart palliation. However, the single centre study reduced the bias of inter laboratory MRI variability and inter centre operative outcome. Moreover, we only included patients without antegrade pulmonary flow, thus excluding a moderate number of pre-Fontan patients.
In conclusion, our study confirmed the emerging growing evidence of the importance of ventricular diastolic function in single ventricle patients also in pre-Fontan stage. Moreover, from our data, higher end-diastolic ventricular pressure is associated with reduced svc flow and pulmonary hypoplasia both evaluated non-invasively by cardiac MRI. Further prospective studies are needed to confirm these findings and possibly identify a cut-off value of higher end-diastolic ventricular pressure.
Acknowledgements
None.
Financial support
This research received no specific grant from any funding agency, commercial, or not-for-profit sectors.
Conflicts of interest
None.