Introduction
Bacterial inoculants are the most common additives used to preserve ensiled crops (Kung et al., Reference Kung, Stokes, Lin, Buxton, Muck and Harrison2003), and they are composed mainly of lactic acid bacteria (LAB). Lactic acid bacteria such as Lactobacillus plantarum and L. buchneri have respectively the ability to improve dry matter (DM) recovery during fermentation (Oliveira et al., Reference Oliveira, Weinberg, Ogunade, Cervantes, Arriola, Jiang, Kim, Li, Gonçalves, Vyas and Adesogan2017) and to increase aerobic stability of silages during unloading (Driehuis et al., Reference Driehuis, Oude Elferink and Spoelstra1999; Kleinschmit and Kung, Reference Kleinschmit and Kung2006a). Although bacterial inoculants are used mostly to improve silage quality, some studies reported enhanced feed intake and growth performance of small and large ruminants by feeding inoculated silage with DM content ranging 180–335 g/kg as fed (Keady and Steen, Reference Keady and Steen1995; Nkosi et al., Reference Nkosi, Meeske, Palic, Lang, Leeuw and Groenewald2009; Basso et al., Reference Basso, Adesogan, Lara, Rabelo, Berchielli, Teixeira, Siqueira and Reis2014). This response probably arises from the higher preservation of silage nutrients caused by inoculation (Winters et al., Reference Winters, Fychan and Jones2001; Rabelo et al., Reference Rabelo, Basso, Lara, Jorge, Härter, Mesquita, Silva and Reis2018a), along with a potential probiotic effect of some LAB species (Weinberg and Muck, Reference Weinberg and Muck1996; Weinberg et al., Reference Weinberg, Muck and Weimer2003). However, the mechanisms by which LAB improve livestock production are not completely known, and the results from the literature are largely inconsistent (Addah et al., Reference Addah, Baah, Okine, Owens and McAllister2014; Rabelo et al., Reference Rabelo, Mari, Reis, Da Silva and Santos2016; Muck et al., Reference Muck, Nadeau, McAllister, Contreras-Govea, Santos and Kung2018).
In addition to the LAB, recent studies proposed the utilization of Bacillus subtilis, a bacterium commonly found in the soil, as a silage additive because of its potential to increase the aerobic stability of maize silage (Basso et al., Reference Basso, Lara, Assis, Morelli, Rabelo and Reis2012) and to improve silage digestibility (Lara et al., Reference Lara, Basso, de Assis, Souza, Berchielli and Reis2016). These benefits probably arise from the ability of B. subtilis in producing antifungal compounds (Zuber et al., Reference Zuber, Nakano, Marahiel, Sonenshein, Hoch and Losick1993) and amylase (Van Soest et al., Reference Van Soest, Robertson and Lewis1991). The higher aerobic stability accompanied by enhanced digestibility in maize silages inoculated with B. subtilis alone or combined with L. plantarum (Basso et al., Reference Basso, Lara, Assis, Morelli, Rabelo and Reis2012; Lara et al., Reference Lara, Basso, de Assis, Souza, Berchielli and Reis2016) may result in improved animal performance. However, to the best of our knowledge, only one study has investigated the effect of using B. subtilis as a silage inoculant on ruminant performance, and the resulting feed intake and average daily gain (ADG) of Nellore steers were found to be unaffected (Rabelo et al., Reference Rabelo, Valente, Barbero, Basso and Reis2018b). Thus, further research examining the effects of feeding B. subtilis to livestock through silage is needed to elucidate the mechanisms by which livestock production may be improved. The current authors’ hypothesis is that inoculation of maize silage with L. plantarum combined with either L. buchneri or B. subtilis may result in better silage preservation with lower spoilage during feed-out phase, the way so that lamb production could be improved as well.
Based on this context, the current study was designed to assess the effect of adding LAB and B. subtilis to maize silage and its impact on feed intake, growth performance and carcass and meat traits of finishing feedlot lambs.
Material and methods
Silage production
The flint maize (Zea mays) hybrid ‘Impacto Víptera’ (Syngenta, Matão, SP, Brazil) was harvested at 2/3 of the kernel milk line (hard dough (R5) stage (Nielsen, Reference Nielsen2018); 354 g DM/kg as fed) using a Premium Flex forage harvester (Menta Mit, Cajuru, SP, Brazil) and chopped to a length of 10 mm; kernels were not processed. Whole-crop maize forage was treated with distilled water (5 l/t; control) or inoculated with L. plantarum MA18/5U combined with either L. buchneri CNCM I-4323 (treatment LBLP), or with B. subtilis AT553098 (treatment BSLP). The inoculation dose of each microorganism was 1 × 105 cfu/g of fresh forage. Both L. buchneri and L. plantarum strains were provided by Lallemand Animal Nutrition (Milwaukee, WI, USA), whereas B. subtilis was provided by Fatec Animal Nutrition (São Paulo, SP, Brazil). In order to ensure homogeneous distribution of the inoculants over the crop, while the maize forage was spread and packed, the inoculants were dissolved in distilled water (5 l/t) and sprayed onto fresh forage layer by layer as silos were filled using a sterilized costal pomp (Mohammadzadeh et al., Reference Mohammadzadeh, Khorvash, Ghorbani and Yang2012; Basso et al., Reference Basso, Adesogan, Lara, Rabelo, Berchielli, Teixeira, Siqueira and Reis2014).
Three stack silos (n = 1) were filled in 2 days with approximately 40 tons each of the respective maize forage (control, LBLP and BSLP). To avoid possible cross-contamination, the control forage was ensiled first, followed by the inoculated forages. Silos were sealed with a 200 µm double-layer silage pit cover (Electro Plastic, São Paulo, SP, Brazil). During ensiling, one composite fresh sample of maize forage (~300 g) was collected from each tractor load (sampled in five different points) while the stack silos were filled, totalling 12 samples per silo.
Silos were stored in the field for 88 days before being used (average temperature 21 ± 1.9 °C). The silage was removed from the silo face using a fork in a feed-out rate of approximately 12.8 cm/day. Both control and inoculated maize silages were used to feed lambs in the current study and to feed Nellore steers in a concomitant study of Rabelo et al. (Reference Rabelo, Valente, Barbero, Basso and Reis2018b). Silage samples (n = 12; ~300 g) were collected weekly during unloading from five locations from the feeding face of each silo (two each from the lower and upper areas and one from the central area) and bulked into a single sample on each sampling week. Both forage and silage samples were stored at −20 °C until further analysis. Beyond the lack of silo replicates, the sampling procedure provided only a descriptive analysis of maize silages (Udén and Robinson, Reference Udén, Robinson, Daniel, Morais, Junges and Nussio2015).
Aerobic stability
An aerobic stability assay was carried out three times during feed-out as follows. Four silage samples (~3 kg) from each farm silo were placed in plastic buckets of 5-l capacity and kept at ambient temperature. The silage temperature was measured every half hour by a datalogger placed in the centre of the mass for 5 days. The ambient temperature was also measured every 30 min by two dataloggers distributed near the buckets. Aerobic stability was defined as the number of hours that the silage temperature remained stable before increasing 2 °C above the ambient temperature (Moran et al., Reference Moran, Weinberg, Ashbell, Hen, Owen and Jones1996; Rabelo et al., Reference Rabelo, Valente, Barbero, Basso and Reis2018b).
Animal performance study
Thirty Dorper × Santa Ines crossbred intact male, weaned lambs (29 ± 3.5 kg initial body weight (BW)) were divided by weight into three groups. The lambs were housed in individual wooden pens (0.5 m2) with slated floors, each fitted of a feed and a water container placed in a well and naturally ventilated covered barn with open sides. Lambs were assigned randomly (n = 10) to one of three diets containing control, LBLP and BSLP silages. Diets were composed of 0.60 the respective maize silage and 0.40 concentrate on a DM basis, and they were balanced to meet the nutrient requirements of growing lambs gaining 200 g/day (NRC, 2007; Table 1). Lambs were fed ad libitum twice daily at 07.00 and 17.00 h and had free access to water. Approximately 0.60 of the diet was offered at 07.00 h and the remaining 0.40 at 17.00 h. Orts were weighed every morning to calculate dry matter intake (DMI). Samples of feed and orts were collected twice a week and stored at −20 °C for later analysis.
Table 1. Ingredient proportions and chemical composition of total mixed rations containing maize silage inoculated with lactic acid bacteria and Bacillus subtilis
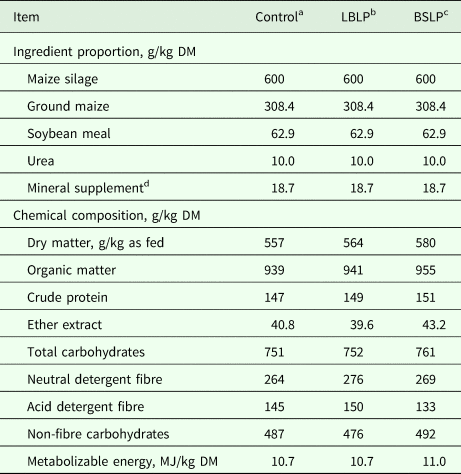
DM, dry matter.
a Untreated maize silage.
b Maize silage treated with Lactobacillus buchneri and Lactobacillus plantarum.
c Maize silage treated with B. subtilis and L. plantarum.
d Mineral supplement was composed of 70 g P/kg, 160 g Ca/kg, 100 g Na/kg, 5 g Mg/kg, 40 g S/kg, 2560 mg Zn/kg, 690 mg Cu/kg, 530 mg Mn/kg, 41 mg Co/kg, 51 mg I/kg, 700 mg F/kg and 13 mg Se/kg, on a DM basis.
Lambs were adapted to the diets for 21 days and the feeding trial lasted a total of 89 days. During days 1–3 of the adaptation period, lambs were fed only maize silage. Thereafter, there was a gradual increase in concentrate amount during this period in which lambs were fed a forage: concentrate ratio 90:10 on days 4–6, 80:20 on days 7–9, 70:30 on days 10–12, and 60:40 on days 13–21. At the beginning and end of the feeding period, lambs were weighed after a 16 h fast and ADG was calculated by subtracting the initial BW from the final BW and dividing the difference by the time in which lamb remained in the trial. Feed efficiency (gain:feed) was determined by dividing ADG by DMI.
Slaughter, carcass and meat analyses
When the lambs reached 40 ± 2.0 kg BW, they were slaughtered by electro-narcosis anaesthetization (220 V/0.5 A) and subsequent bleeding by cutting their jugular veins and carotid arteries. After evisceration, the carcass was split into two parts and weighed to determine the hot carcass weight (HCW). All carcasses were refrigerated at −4 °C for approximately 24 h and then taken from the cooling chambers and weighed again to determine the cold carcass weight (CCW). Hot (HCY) and cold carcass yield were calculated by dividing the HCW and CCW by the fasted BW and multiplying by 100, respectively. The 12th rib fat thickness (RFT) was measured on the left side of the carcasses over the loin-eye muscle using a calliper. With exception of the determinations performed on hot carcass (i.e., HCW and HCY), all procedures described above for carcass trait measurements were carried out after 24 h post mortem chill on carcasses stored at −4 °C, according to Silva Sobrinho and Osório (Reference Silva Sobrinho, Osório, Silva Sobrinho, Sañudo, Osório, Arribas and Osório2008).
The colour and final pH (after 24 h post mortem chill and at approximately 4 cm deep) were determined in the Longissimus lumborum muscle using a CR-200 Minolta colourimeter (illuminant D65) calibrated to standard white digital and a TESTO 205 pH meter coupled to a penetration electrode, respectively. The meat colour variables luminosity (L*), redness (a*) and yellowness (b*) were determined after the cut meat was exposed to the environment for 5 min (Cañeque and Sañudo, Reference Cañeque and Sañudo2000). The colour was measured at three different points and average values were calculated. The colorimeter was calibrated before analysing the samples against white and black standards.
For the qualitative analysis, the loins were thawed in a biochemical oxygen demand incubator at 10 °C for 12 h. The water holding capacity (WHC) was determined by placing 500 ± 20 mg muscle samples on filter paper between two acrylic plates, which were then placed under 10 kg weight for 5 min. The resulting meat sample was weighed again and the water loss was given by the difference between the initial and final weight (Silva Sobrinho, Reference Silva Sobrinho1999).
The Warner–Bratzler shear force was determined in 3 × 1 cm cooked meat samples by cutting the meat cubes perpendicularly to the muscle fibres using a Texture Analyser fitted with a Warner–Bratzler cutting blade (Ramos and Gomide, Reference Ramos and Gomide2007). Cooking loss was determined by baking the meat samples in a pre-heated industrial furnace at 170 °C, until inner temperature reached 71 °C. The samples were then removed from the oven and weighed again to calculate the percentage of water loss (Ramos and Gomide, Reference Ramos and Gomide2007).
Determination of ruminal fermentation products
Six Dorper × Santa Ines crossbred ruminally cannulated wethers (75 ± 4.5 kg initial BW), each fitted with a silicone 6.4 cm ruminal cannula, were used in a double 3 × 3 Latin Square design. Each animal was housed individually in pens (1.8 m2) equipped with a feed bunk and water trough. Wethers were fed ad libitum twice daily at 07.00 and 17.00 h and had free access to water; the diets used and procedures of feeding were the same as described earlier. Orts were also weighed every morning, and samples of offered feed and orts were collected twice a week and stored at −20 °C for later analyses.
Ruminal measurements were taken over three consecutive 19-day periods without intervals between each period. Each period consisted of 18 days to adapt to the diets and 1 day for rumen fluid collection. A 50 ml sample of ruminal fluid was collected from each wether immediately before feeding (0 h) and at 3, 6, 9, 12 and 24 h post-feeding. Ruminal fluid was squeezed through two layers of cheesecloth and the hydrogen potential (pH) was immediately measured using a pH meter (MA522 model, Marconi Laboratory Equipment, Piracicaba, SP, Brazil). Subsequently, 1 ml of sulphuric acid (H2SO4; 1:1) was added to the ruminal fluid and the solution was stored at −20 °C for the analyses of volatile fatty acids (VFA) and ammonia-N.
Sample preparation and chemical analyses
To measure the fermentation end products of silages, 25 g of fresh silage was mixed with 225 ml of distilled water. This mixture was blended in a Phillips Walita blender (Walita, Varginha, MG, Brazil) for 1 min at the highest setting and filtered through two layers of cheesecloth. The pH of the filtrate was measured immediately using a pH meter (model MA522, Marconi Laboratory Equipment, Piracicaba, SP, Brazil). Lactic acid was determined by the colorimetric method (Pryce, Reference Pryce1969). The VFA were measured using a gas chromatograph (Shimadzu model GC2014, Shimadzu Corp., Kyoto, Japan) equipped with an HP-INNOWax capillary column (30 m × 0.32 mm; Agilent Technologies, Santa Clara, California, USA) at an initial temperature of 80 °C for 3 min, followed by heating at a rate of 20 °C/min until a final temperature of 240 °C was achieved. Water-soluble carbohydrates (WSC) were measured according to Nelson (Reference Nelson1944). Ammonia-N was measured by distillation (AOAC, 1996, method: 941.04) and expressed as g/kg of total nitrogen (TN).
For microbial analyses, 12 fresh silage samples (25 g) from each silo were collected and then homogenized in 225 ml of autoclaved saline solution (0.85% sodium chloride [NaCl]) for 1 min. An aliquot (1 ml) of this solution was transferred into tubes containing 9 ml of saline solution, and then 1 ml of this mixture was placed onto Petri dishes after serial dilutions from 10−1 to 10−9. Man, Rogosa and Sharpe (MRS) agar was used to count LAB using pour-plates, and potato dextrose agar (PDA) was used to count yeasts and moulds using spread-plates. Both MRS and PDA plates were incubated at 28 °C. Counts of LAB and yeasts were performed after 2 days, and moulds were counted after 5 days. All microbiological data were log10-transformed to ensure normality.
Forage and silage samples were oven dried (55 °C for 72 h) and processed in a knife mill before being ground through a 1 mm screen and analysed for DM (105 °C for 12 h) (AOAC, 1996, method: 930.15) and ash (500 °C for 5 h) (AOAC, 1996, method: 923.03). The organic matter (OM; g/kg) was calculated as 1000 − ash. Ether extract was determined following the procedures described by AOAC (1996, method: 920.39). The TN was measured by rapid combustion using a LECO Analyser (model F528 N, LECO Corp., St. Joseph, MI, USA), and crude protein (CP) was calculated as TN × 6.25. The neutral detergent fibre (NDF) and acid detergent fibre (ADF) were determined in an ANKOM 2000 Fibre Analyser (ANKOM Technologies, Macedon, NY, USA) following the procedures described by Mertens (Reference Mertens2002) and AOAC (1996, method: 973.18), respectively. The NDF was measured using a heat-stable amylase without sodium sulphite, and both NDF and ADF were expressed exclusive of residual ash. Total carbohydrate and non-fibre carbohydrate contents were calculated according to Sniffen et al. (Reference Sniffen, O'Connor, Van Soest, Fox and Russell1992) and Detmann and Valadares Filho (Reference Detmann and Valadares Filho2010), respectively.
Ruminal ammonia-N was determined by distillation with 2N potassium hydroxide according to Fenner (Reference Fenner1965). Aliquots of strained ruminal fluid collected were thawed in a refrigerator overnight and centrifuged (20 000 × g) for 30 min at 4 °C, and the supernatant was analysed for VFA by gas chromatography as described earlier.
Statistical analyses
Statistical analyses of silage data were precluded because there was only one farm-scale silo replicate for each treatment. Feed intake, growth performance and carcass and meat traits were analysed using the MIXED procedure of SAS (v. 9.4 SAS Institute Inc., Cary, NC, USA) in a completely randomized design (n = 10). Diet was considered as a fixed effect and error as a random effect. Ruminal fermentation was carried out under a double 3 × 3 Latin Square design and analysed as repeated measures over time. All variables were analysed using the MIXED procedure of SAS (v. 9.4 SAS Institute Inc.). The covariance matrix that best fit the ruminal fermentation data according to the Akaike information criterion was Variance Components. Diet was considered as a fixed effect and wethers and period as random effects. Differences between diets were determined using the PDIFF option of LSMEANS. Significant differences were declared at P ⩽ 0.05 and trends discussed at 0.05 > P ⩽ 0.10.
Results
Fermentation, microbial profile and chemical composition of the maize silages
Whole-crop maize forage had the following composition prior to ensiling, on a DM basis: 354 g DM/kg fresh weight, 32.9 g/kg ash, 115 g/kg CP and 149 g/kg ADF. After 88 days of ensilage, both LBLP and BSLP silages had lower lactic acid concentrations and higher DM contents and LAB counts compared with that in the control silage (Table 2). Moreover, the LBLP silage had lower WSC content and higher aerobic stability than the others.
Table 2. Fermentation and microbial profile, chemical composition and aerobic stability of maize silages inoculated with lactic acid bacteria and Bacillus subtilis in stack silos during the unloading period (mean ± standard error of the mean)

DM, dry matter; TN, total nitrogen; WSC, water-soluble carbohydrates; LAB, lactic acid bacteria.
a Untreated maize silage.
b Maize silage treated with Lactobacillus buchneri and Lactobacillus plantarum.
c Maize silage treated with B. subtilis and L. plantarum.
Feed intake, growth performance, and carcass and meat traits
Inoculation of maize silage did not affect the DMI of lambs, expressed as kg/day (P = 0.242, overall mean = 1.16 kg/day) and as % BW (P = 0.207, overall mean = 3.31% BW; Table 3). Likewise, the intakes of OM (P = 0.197), CP (P = 0.182) and NDF (P = 0.156) were unaffected by inoculation. Inoculation of maize silage did not produce any effect on the final BW (P = 0.589), nor on ADG (P = 0.221, overall mean = 0.217 kg/day) or feed efficiency (P = 0.298) of lambs. The yellowness (b*) of lamb meat decreased (P = 0.001) by inoculation of maize silage, mainly when lambs were fed the BSLP diet. Other measurements on the carcass and meat traits of lambs were unaffected by inoculation.
Table 3. Feed intake, growth performance, and carcass and meat traits of finishing feedlot lambs fed total mixed rations containing maize silage inoculated with lactic acid bacteria and Bacillus subtilis (n = 10)

BW, body weight; ADG, average daily gain; HCW, hot carcass weight; CCW, cold carcass weight; HCY, hot carcass yield; CCY, cold carcass yield; RFT, rib fat thickness; WHC, water holding capacity; CL, cook losses; WBSF, Warner–Bratzler shear force.
a Untreated maize silage.
b Maize silage treated with Lactobacillus buchneri and Lactobacillus plantarum.
c Maize silage treated with B. subtilis and L. plantarum.
Ruminal fermentation
There was an interaction between diet and the interval by which ruminal fluid was sampled for total VFA concentrations and molar proportions of butyrate, valerate and isovalerate (Table 4). Compared with the control diet, wethers fed the LBLP and BSLP diets had higher (P = 0.023) VFA concentrations at 3 h post-feeding and lower VFA concentrations at 24 h post-feeding. Wethers fed the BSLP diet had increased concentrations of butyrate, valerate and isovalerate at 3, 6 and 12 h post-feeding, respectively. Inoculation of maize silage did not affect ruminal pH (P = 0.823), ammonia-N (P = 0.663), or proportions of acetate (P = 0.102), butyrate (P = 0.084) or isobutyrate (P = 0.589). Regarding the interval at which ruminal fluid was sampled, lower (P < 0.001) ruminal pH was observed between 3 and 12 h post-feeding (5.62–6.10). Ammonia-N had a peak at 3 h post-feeding (P < 0.001, 23.0 mg/dl). The molar proportion of acetate was higher (P < 0.001) at 0 and 24 h post-feeding (71.8 and 70.7 mm/100 mm), while propionate had higher (P = 0.001) values at 3, 6 and 12 h post-feeding. The molar proportion of isobutyrate was higher (P < 0.001) at 0 and 24 h post-feeding (0.90 and 0.92 mm/100 mm, respectively).
Table 4. Ruminal fermentation in cannulated wethers fed total mixed rations containing maize silage inoculated with lactic acid bacteria and Bacillus subtilis (n = 6)

S, silage; T, time; S × T, interaction between silage and time.
a Untreated maize silage.
b Maize silage treated with Lactobacillus buchneri and Lactobacillus plantarum.
c Maize silage treated with B. subtilis and L. plantarum.
Discussion
Bacterial inoculants composed of LAB are the most common silage additives used worldwide to ensure a suitable fermentation process in silo (Kung et al., Reference Kung, Stokes, Lin, Buxton, Muck and Harrison2003). Ruminants fed silage-based diets following LAB inoculation have shown improved growth performance in some cases, but the mechanisms for such responses are not yet entirely clear. Additionally, little is known on the effects of feeding ruminants B. subtilis through silage. Thus, studies examining the utilization of bacterial inoculants with particular emphasis on their effects on livestock production may be of interest to the scientific community and useful for farmers.
In the current study, the number of farm-scale silos used per treatment (n = 1) and sampling procedure carried out during unloading prevented a meaningful statistical analysis of fermentation and chemical composition of maize silage (Udén and Robinson, Reference Udén, Robinson, Daniel, Morais, Junges and Nussio2015). Consequently, only a descriptive analysis regarding silage measurements was provided. However, a discussion about the most obvious silage variables affected by bacterial inoculation is needed to support the responses observed with lambs. The chemical composition of maize silage was typical of those produced under tropical areas (Arriola et al., Reference Arriola, Kim, Staples and Adesogan2011; Lara et al., Reference Lara, Basso, de Assis, Souza, Berchielli and Reis2016; Rabelo et al., Reference Rabelo, Basso, Lara, Jorge, Härter, Mesquita, Silva and Reis2018a). The LBLP silage had lower WSC concentration than the control and BSLP silages, which is in accordance with previous studies (Filya, Reference Filya2003a, Reference Filya2003b; Kleinschmit and Kung, Reference Kleinschmit and Kung2006b). Lowered WSC is expected in silages treated with L. buchneri because this bacterium remains fairly active in latter phases of fermentation (e.g., silages stored for up to a year; Kleinschmit and Kung, Reference Kleinschmit and Kung2006b), with continuous utilization of WSC as substrate for its growth (Pahlow et al., Reference Pahlow, Muck, Driehuis, Oude-Elferink, Spoelstra, Buxton, Muck and Harrison2003). Counts of LAB increased in both inoculated silages as a response of adding bacteria from inoculant at ensiling; however, the concentration of lactic acid was reduced. The lowered lactic acid concentration in the LBLP silage likely arises from the ability of L. buchneri in converting lactic acid into acetic acid and 1,2-propanodiol under anaerobic conditions (Oude Elferink et al., Reference Oude Elferink, Krooneman, Gottschal, Spoelstra, Faber and Driehuis2001). In contrast, the competition between B. subtilis and L. plantarum for substrate (i.e., WSC) may explain the lower concentration of lactic acid in the BSLP silage. Bacillus spp. was found to be very inefficient producer of lactic acid during silage fermentation (McDonald et al., Reference McDonald, Henderson and Heron1991). The current results are in agreement with those of previous studies, in which bacterial inoculation increased LAB counts in maize silage, but this response was not accompanied of higher lactic acid concentration (Driehuis et al., Reference Driehuis, Oude Elferink and Spoelstra1999; Hu et al., Reference Hu, Schmidt, McDonell, Klingerman and Kung2009). Furthermore, both inoculated silages showed higher butyric acid concentration than control silage, which may be indicative of clostridial fermentation. Clostridial activity is known to lead large losses of DM and poor recovery of energy because the inefficient metabolic pathway utilized for butyric acid production (Pahlow et al., Reference Pahlow, Muck, Driehuis, Oude-Elferink, Spoelstra, Buxton, Muck and Harrison2003). In addition, clostridial fermentation may reflect in lower than normal concentrations of lactic acid (Kung et al., Reference Kung, Shaver, Grant and Schmidt2018). Although the reasons for this result remain unclear, a mini-silo experiment assessing maize silage treated with B. subtilis alone or combined with L. plantarum reported higher butyric acid concentration in the inoculated silage (Lara et al., Reference Lara, Basso, de Assis, Souza, Berchielli and Reis2016).
The LBLP silage had increased aerobic stability, which may be related to the reduced WSC concentration in this silage and the production of antimicrobial substances other than acetic acid by L. buchneri, since there was only a slight increase in acetic acid concentration. The L. buchneri was found to produce a bacteriocin, buchnericin LB, which could be responsible for improved aerobic stability (Yildirim, Reference Yildirim2001; Kleinschmit and Kung, Reference Kleinschmit and Kung2006a). Furthermore, in the presence of air yeasts utilize WSC as a substrate to grow, which causes deterioration and heating of silage mass (McDonald et al., Reference McDonald, Henderson and Heron1991). Thus, the lower supply of substrate (i.e., WSC) for yeast growth may also explain the increased aerobic stability in the LBLP silage. Contrary to expectations, inoculation of maize silage with L. plantarum and B. subtilis did not improve aerobic stability. Bacillus subtilis has the ability to produce antifungal compounds (bacteriocins or bacteriocin-like inhibitory substances; Zuber et al., Reference Zuber, Nakano, Marahiel, Sonenshein, Hoch and Losick1993), which has been associated with increased aerobic stability (Basso et al., Reference Basso, Lara, Assis, Morelli, Rabelo and Reis2012; Lara et al., Reference Lara, Basso, de Assis, Souza, Berchielli and Reis2016). However, to date, no antimicrobial compounds yielded by B. subtilis have been detected in maize silage, suggesting that mechanisms other than antimicrobial substances production may be related to improved aerobic stability. The unchanged aerobic stability of the BSLP silage compared with the control is consistent with the results found in a mini-silo experiment investigating different strains and doses of B. subtilis (Rabelo et al., Reference Rabelo, Viche, Lara, Basso, Jorge and Reis2014).
Regarding the responses in animal performance, the bacterial inoculation of maize silage did not change the DMI of lambs. Increases in the DMI caused by bacterial inoculation may be primarily accompanied by changes in the fermentation end products and chemical composition of silages (Charmley, Reference Charmley2001; Winters et al., Reference Winters, Fychan and Jones2001; Rabelo et al., Reference Rabelo, Basso, Lara, Jorge, Härter, Mesquita, Silva and Reis2018a). However, such changes were subtle (e.g., ammonia-N, WSC, lactic acid and DM content) or did not occur in the current study, explaining the lack of differences in DMI. Moreover, alterations in the DMI following silage inoculation are difficult to envision because previous studies have reported increased (Nkosi et al., Reference Nkosi, Meeske, Palic, Lang, Leeuw and Groenewald2009; Basso et al., Reference Basso, Adesogan, Lara, Rabelo, Berchielli, Teixeira, Siqueira and Reis2014), unchanged (Arriola et al., Reference Arriola, Kim, Staples and Adesogan2011) and decreased (Mohammadzadeh et al., Reference Mohammadzadeh, Khorvash, Ghorbani and Yang2012) DMI in small and large ruminants. Differences in the type of bacteria, strain and application rate administered, as well as the dietary silage content and its interaction with ruminal microorganisms contribute to understanding of the inconsistencies in literature regarding bacterial inoculation on DMI. Furthermore, enhanced animal performance following silage inoculation appears to occur mostly in silages with low-to-moderate DM content (i.e., 180–335 g/kg as fed) as observed in previous studies (Keady and Steen, Reference Keady and Steen1995; Nkosi et al., Reference Nkosi, Meeske, Palic, Lang, Leeuw and Groenewald2009; Basso et al., Reference Basso, Adesogan, Lara, Rabelo, Berchielli, Teixeira, Siqueira and Reis2014). The explanation for such results is probably due to the improved fermentative process reported in those silages, such as increased lactic acid concentration and lactic:acetic acid ratio and decreased clostridial fermentation, microbial deamination and silage spoilage (McDonald et al., Reference McDonald, Henderson and Heron1991; Muck et al., Reference Kung, Shaver, Grant and Schmidt2018).
To date, little is known about the effect of feeding B. subtilis through silage on DMI. Nevertheless, likely mechanisms by which B. subtilis may increase DMI are as follows: (1) by reducing silage deterioration after the silos are opened, owing to its antifungal property (Zuber et al., Reference Zuber, Nakano, Marahiel, Sonenshein, Hoch and Losick1993; Basso et al., Reference Basso, Lara, Assis, Morelli, Rabelo and Reis2012), (2) by increasing silage digestibility, owing to the production of amylase (Van Soest et al., Reference Van Soest, Robertson and Lewis1991; Zuber et al., Reference Zuber, Nakano, Marahiel, Sonenshein, Hoch and Losick1993; Lara et al., Reference Lara, Basso, de Assis, Souza, Berchielli and Reis2016) and (3) by modifying the bacterial community in the rumen in a positive way to improve ruminal fermentation (Hosoi et al., Reference Hosoi, Ametani, Kiuchi and Kaminogawa2000). As aerobic stability of the BSLP silage was similar to that of the control, and ruminal fermentation was little affected by inoculation, differences in the DMI of lambs fed the BSLP diet are unlikely. Only one study to date has reported that DMI was not altered by feeding Nellore steers with a BSLP diet (Rabelo et al., Reference Rabelo, Valente, Barbero, Basso and Reis2018b).
Despite the unchanged DMI, the total VFA concentrations and molar proportions of butyrate, valerate and isovalerate in the rumen of wethers were affected by an interaction between bacterial inoculation of maize silage and the interval at which ruminal fluid was sampled. In some cases, a potential probiotic effect caused by LAB was used to explain enhanced animal performance when minor or no changes in silage fermentation were detected (Weinberg and Muck, Reference Weinberg and Muck1996). This hypothesis is based on an interaction between LAB added through the bacterial inoculant and bacterial community in the rumen, which may modify ruminal fermentation (Weinberg et al., Reference Weinberg, Muck and Weimer2003). Nevertheless, the changes detected in the current study were unclear and inconsistent over the interval at which ruminal fluid was sampled, and such responses may be of minor importance. These results agree with those of previous studies (Basso et al., Reference Basso, Adesogan, Lara, Rabelo, Berchielli, Teixeira, Siqueira and Reis2014; Addah et al., Reference Addah, Baah, Okine and McAllister2015; Rabelo et al., Reference Rabelo, Valente, Barbero, Basso and Reis2018b), in which few and inconsistent changes on ruminal fermentation end products were found in wethers and bulls fed total mixed rations containing different proportions of maize silage inoculated with LABs and B. subtilis.
As observed for DMI, the feed efficiency and ADG of lambs was not altered by silage inoculation. Increased ADG normally arises from increased feed intake or improved feed efficiency, along with increased dietary digestibility (McAllister et al., Reference McAllister, Selinger, McMahon, Bae, Lysyk, Oosting and Cheng1995). Thus, the lack of differences in feed intake and feed efficiency explains why lambs had similar ADG in the current study. It is worth noting that, in the literature, the enhanced animal performance following bacterial inoculation is reported less frequently than improvements on silage fermentation (Weinberg and Muck, Reference Weinberg and Muck1996). For instance, a review of studies assessing the inoculation of various crop silages under tropical conditions reported that only seven of 39 studies investigating the ADG of small and large ruminants detected an increase due to silage inoculation (Rabelo et al., Reference Rabelo, Mari, Reis, Da Silva and Santos2016). The unaltered growth performance of lambs fed silage-based diets differing in the microorganisms used in the inoculation is supported by a previous study with Nellore steers fed the same silages (Rabelo et al., Reference Rabelo, Valente, Barbero, Basso and Reis2018b).
The inoculation of maize silage decreased yellowness for both lambs fed the LBLP and BSLP diets. However, the cause for such results is unknown as changes in yellowness are often related with pre-slaughter glycogen and marbling contents, as well as with the dietary forage: concentrate ratio and β-carotene consumption (Mancini and Hunt, Reference Mancini and Hunt2005). The WHC also tended to decrease in meat of lambs fed the inoculated diets, mainly for LBLP diet. Ground maize and soybean meal are rich in tocopherols that display antioxidant properties (Chow and Draper, Reference Chow and Draper1974), and the higher intake of these compounds can increase WHC of meat (Cheng and Sun, Reference Cheng and Sun2008). Despite the lack of statistical differences in DMI, lambs fed the LBLP diet consumed 0.16 kg DM/day less than lambs fed the control diet; thus, likely lambs fed the LBLP diet consumed lower amounts of tocopherols, resulting in lower values of WHC. With the exception of meat colour and tendency observed for WHC, other carcass and meat traits of lambs were unaffected by silage inoculation; this result can be attributed to the lack of differences in DMI and ADG, as well as the inconsistent responses in ruminal fermentation. Furthermore, lambs were slaughtered after approximately 54 days of feedlot (not considering the adaptation period), and it is worth mentioning that short-term feeding has little or no effect on the meat quality of ruminants (Arnold et al., Reference Arnold, Scheller, Arp, Williams, Buege and Schaefer1992; Cerdeño et al., Reference Cerdeño, Vieira, Serrano, Lavína and Mantecón2006). Although the number of studies assessing the effect of bacterial inoculation on animal performance has increased in recent years, few studies have examined the effect of bacterial inoculants on carcass and meat traits. However, the majority of those studies have found no effect of silage inoculation on meat quality (Fugita et al., Reference Fugita, Prado, Jobim, Zawadzki, Valero, Pires, Prado and Françozo2012; Addah et al., Reference Addah, Baah, Okine, Owens and McAllister2014), which supports the results of the current study.
To the best of our knowledge, the present study and the research conducted by Rabelo et al. (Reference Rabelo, Valente, Barbero, Basso and Reis2018b) are the first to assess the effect of using B. subtilis as a silage inoculant on livestock production. It is necessary to highlight that both studies reported consistent results (i.e., little or no effect) of inoculation of B. subtilis combined with L. plantarum on the feed intake, ruminal fermentation and growth performance of small and large ruminants. Moreover, during the ensiling of maize silage, each bacterium (i.e., B. subtilis and L. plantarum) was applied at a rate of 1 × 105 cfu/g of fresh forage and silage inclusion in the diet ranged from 40% to 60%. Thus, reports on the effects of using B. subtilis as a silage inoculant to improve livestock are still limited, although this technique represents a novel approach for silage production and feeding. In this regard, further studies should focus on determining whether the metabolic responses to B. subtilis-treated silage are dose-dependent and strain-specific, and identifying the effects this inoculant may have on animal performance using silage-based diets.
Conclusions
In the current study, lambs were fed maize silages yielded on a farm-scale silo differing in the type of bacterial inoculant used; the use of diets based on maize silage inoculated with L. plantarum combined with either L. buchneri or B. subtilis did not display relevant effect on growth performance of lambs. The lack of differences in growth performance of lambs likely arises from the little influence of bacterial inoculants on silage composition.
Financial support
The authors thank the São Paulo Research Foundation (FAPESP grants #2012/25463-0 and #2012/00412-4) for providing financial support to carry out the current study.
Conflict of interest
None.
Ethical standards
The animal care and handling procedures used in the current study were in accordance with the Brazilian College of Animal Experimentation (COBEA) guidelines and were approved by the Ethics, Bioethics, and Animal Welfare Committee (CEBEA) of São Paulo State University, Jaboticabal, SP, Brazil (protocol no. 8.002/16). Pre-slaughter handling was in accordance with good animal welfare practices, and slaughtering procedures followed the Sanitary and Industrial Inspection Regulation for Animal Origin Products (Brasil, 1997).