1. Introduction
Continental vertebrate faunas from the Jurassic–Cretaceous transition are rare and poorly known (Cifelli et al. Reference Cifelli, Lipka, Schaff and Rowe1999; Clemens et al. Reference Clemens, Goodwin, Hutchinson, Schaff, Wood and Colbert2007; Knoll & Ruiz-Omeñaca, Reference Knoll and Ruiz-Omeñaca2009; J. P. Tennant, unpub. Ph.D. thesis, Imperial College London, Reference Tennant, Mannion, Upchurch, Sutton and Price2016; Tennant et al. Reference Tennant, Mannion and Upchurch2016; Fig. 1). This is especially true for the Gondwanan faunas, particularly the African ones (Sigogneau-Russell et al. Reference Sigogneau-Russell, Evans, Levine, Russell, Lucas, Kirkland and Estep1998; Rauhut & López-Arbarello, Reference Rauhut and López-Arbarello2009). Yet, the Jurassic–Cretaceous transition is a pivotal interval, both from evolutionary and geodynamic perspectives. The Middle Jurassic to Early Cretaceous period saw the emergence or the first diversification of some of the major modern clades of terrestrial vertebrates: modern lissamphibians and dinosaurs diversified 165 million years ago, probably after the Pliensbachian–Toarcian mass extinction event (Marjanović & Laurin, Reference Marjanović and Laurin2007; Allain & Aquesbi, Reference Allain and Aquesbi2008; Allain & Läng, Reference Allain and Läng2009); birds appeared 150 million years ago (Ostrom, Reference Ostrom1976; Zhou, Reference Zhou2004); and the first tribosphenic therian mammal (Tribosphenida), Juramaia, has been dated as early Late Jurassic, about 160 million years ago (Ji et al. Reference Ji, Luo, Yuan, Wible, Zhang and Georgi2002; Luo et al. Reference Luo, Ji, Wible and Yuan2003, Reference Luo, Yuan, Meng and Ji2011), as have the first modern representatives of squamates, including snakes (Evans & Jones, Reference Evans, Jones and Bandyopadhyay2010; Caldwell et al. Reference Caldwell, Nydam, Palci and Apesteguía2015). The angiosperms made their first radiation about 125–130 million years ago (Heimhofer et al. Reference Heimhofer, Hochuli, Burla and Weissert2005; Hochuli et al. Reference Hochuli, Heimhofer and Weissert2006; Berendse & Scheffer, Reference Berendse and Scheffer2009). The Late Jurassic period was also the time of major geodynamic events involving the break-up of Pangaea, with the opening of the Neo-Tethys and Central Atlantic oceans, leading to the separation of Gondwana from Laurasia (Gheerbrant & Rage, Reference Gheerbrant and Rage2006; J. Pouech, unpub. Ph.D. thesis, Claude Bernard – Lyon 1 University, 2008; J. P. Tennant, unpub. Ph.D. thesis, Imperial College London, Reference Tennant, Mannion, Upchurch, Sutton and Price2016; Tennant et al. Reference Tennant, Mannion and Upchurch2016), and eventually to the fragmentation of Gondwana (Li & Powell, Reference Li and Powell2001; Stampfli & Borel, Reference Stampfli and Borel2002; Blakey, Reference Blakey, Fielding, Frank and Isbell2008). The Jurassic and Cretaceous vertebrate faunas evolved as a consequence of these tectonic events and changing geographic position of the continents.

Fig. 1. Continental vertebrate localities known from the Upper Jurassic (Kimmeridgian; bottom) and the Lower Cretaceous (Berriasian; top). Each point represents a locality. Modified from paleobiodb.org (Paleobiology Database Contributors, 2019).
Morocco is a key area for understanding the palaeobiogeographical history of vertebrates because of its geographic position on the African shores of the Tethys (Sigogneau-Russell et al. Reference Sigogneau-Russell, Evans, Levine, Russell, Lucas, Kirkland and Estep1998; Gheerbrant & Rage, Reference Gheerbrant and Rage2006; Haddoumi et al. Reference Haddoumi, Allain, Meslouh, Métais, Monbaron, Pons, Rage, Vullo, Zouhri and Gheerbrant2016), at a crossroad between Laurasia and Gondwana. A remarkably rich and diverse continental microvertebrate fauna, first described in the course of three field campaigns in 1983, 1986 and 1988, occurs in a lenticular calcareous sandstone near the village of Anoual and the Ksar Metlili fort (Sigogneau-Russell et al. Reference Sigogneau-Russell, Evans, Levine, Russell, Lucas, Kirkland and Estep1998) in the High Atlas Mountains, eastern Morocco. This site, known as Ksar Metlili (previously also called the ‘Anoual locality’) belongs to the Ksar Metlili Formation (Haddoumi et al. Reference Haddoumi, Alméras, Bodergat, Charrière, Mangold and Benshili1998; Sigogneau-Russell et al. Reference Sigogneau-Russell, Evans, Levine, Russell, Lucas, Kirkland and Estep1998) and was originally dated as Berriasian (earliest Cretaceous, 140 Ma to 145 Ma) on the basis of calcareous nannofossils (Sigogneau-Russell et al. Reference Sigogneau-Russell, Monbaron and de Kaenel1990). The vertebrate assemblage includes mammals, dinosaurs, pterosaurs, crocodylomorphs, turtles, squamates, rhynchocephalians, lissamphibians, lungfishes, coelacanths, actinopterygians and chondrichthyans (Duffin & Sigogneau-Russell, Reference Duffin and Sigogneau-Russell1993; Sigogneau-Russell et al. Reference Sigogneau-Russell, Evans, Levine, Russell, Lucas, Kirkland and Estep1998). In 2010, an international expedition of French, Swiss and Moroccan researchers discovered new sites with microvertebrate accumulations in the Anoual Formation (Bathonian, Middle Jurassic) near the locality of Guelb el Ahmar (Haddoumi et al. Reference Haddoumi, Allain, Meslouh, Métais, Monbaron, Pons, Rage, Vullo, Zouhri and Gheerbrant2016), and the original Ksar Metlili fossil locality was revisited. About 1.5 tons of sediments were sampled for screen-washing (Figs 2, 3) from several new loci of the Ksar Metlili locality. We describe here this new Ksar Metlili collection as well as that of the 1980s.
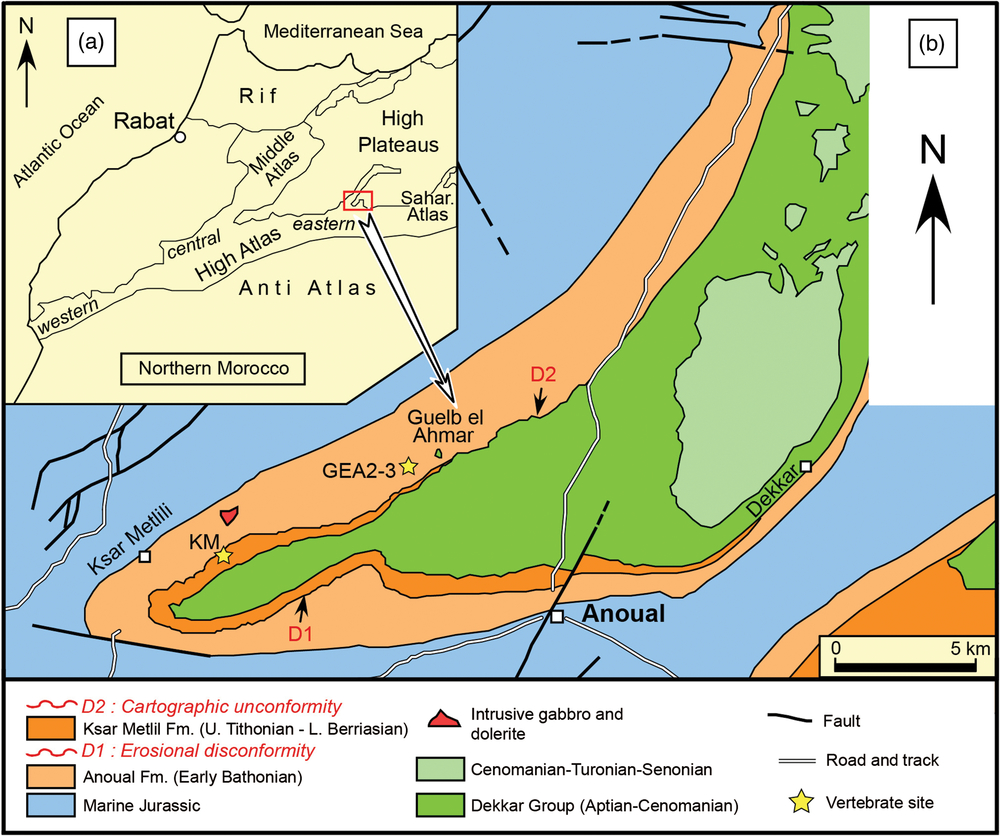
Fig. 2. Geographic and geological settings of the Anoual Syncline. (a) Location of the study area and (b) simplified geological map of the Anoual Syncline indicating the KM and GEA microvertebrate localities. Fm – Formation. Reprinted from Gondwana Research, vol. 29, Haddoumi et al. (Reference Haddoumi, Allain, Meslouh, Métais, Monbaron, Pons, Rage, Vullo, Zouhri and Gheerbrant2016), Guelb el Ahmar (Bathonian, Anoual Syncline, eastern Morocco): first continental flora and fauna including mammals from the Middle Jurassic of Africa, pp. 290–319, Copyright (2016), with permission from Elsevier.

Fig. 3. Location of the fossiliferous loci from the KM site. (a) Geological section and (b) location of the sampled fossiliferous loci from KM (2010 expedition, photography R. Allain): sites KM-A1, KM-A2, KM-B’, KM-C, KM-D1 and KM-D2. The sites are close to each other, separated by a few metres (up to 10–20 m).
The Anoual Syncline is the only place in Africa that has yielded microvertebrate assemblages of Middle Jurassic and possibly earliest Cretaceous age. From a palaeobiological perspective, it includes the two richest and most diversified faunas known in Africa and Gondwana for this period (Sigogneau-Russell et al. Reference Sigogneau-Russell, Evans, Levine, Russell, Lucas, Kirkland and Estep1998; Haddoumi et al. Reference Haddoumi, Allain, Meslouh, Métais, Monbaron, Pons, Rage, Vullo, Zouhri and Gheerbrant2016). These faunas are currently among the best-known representatives of the Mesozoic African palaeoecosystems. In addition, the Anoual Syncline records one of the few Jurassic–Cretaceous faunal successions in Gondwana (Haddoumi et al. Reference Haddoumi, Allain, Meslouh, Métais, Monbaron, Pons, Rage, Vullo, Zouhri and Gheerbrant2016), if the Cretaceous age of the Ksar Metlili Formation is confirmed (see Section 6.c below). The aim of this paper is to update the faunal list of the Ksar Metlili fossil locality, to examine the diversity and the main taphonomic and palaeoenvironmental characteristics of this fossil assemblage, and to review the biostratigraphical and palaeobiogeographical significance of the fauna.
Abbreviations. CR2P – Centre for Research on Palaeontology, Paris, France; GEA – Guelb el Ahmar locality, Anoual Formation, Bathonian (Middle Jurassic), Anoual Syncline, Morocco; KM – Ksar Metlili fossil locality, Ksar Metlili Formation, ?Berriasian (Lower Cretaceous), Anoual Syncline, Morocco (it corresponds to the fossil locality discovered in 1983 by D. Sigogneau-Russell’s team (old collections, indicated by the prefixes MCM or SA and deposited at the National Museum of Natural History), including the new loci sampled in 2010 by our team (Fig. 3; new collections, indicated by the prefix KM and currently housed at the MNHN); they will be deposited at the Faculty of Sciences Aïn Chock after study, with the prefix FSAC-KM); MNHN – National Museum of Natural History, Paris, France.
2. Geological context
The Ksar Metlili (KM) microvertebrate site, which forms the focus of this study, is situated in the ‘Couches Rouges’ (‘Red Beds’, or ‘Continental Intercalaire’; Kilian, Reference Kilian1931) in the Moroccan eastern High Atlas Mountains, c. 12 km west of Anoual City, near the Ksar Metlili fort (32° 42′ 2″ N, 3° 13′ 3″ W; Fig. 2). These ‘Red Beds’ are widely distributed in the Middle and High Atlas (Haddoumi et al. Reference Haddoumi, Allain, Meslouh, Métais, Monbaron, Pons, Rage, Vullo, Zouhri and Gheerbrant2016).
2.a. Stratigraphy and age of the Ksar Metlili site
In the Anoual Syncline, Haddoumi et al. (Reference Haddoumi, Alméras, Bodergat, Charrière, Mangold and Benshili1998, Reference Haddoumi, Charrière, Andreu and Mojon2008) distinguished three Middle Jurassic–Cretaceous stratigraphical units in the ‘Red Beds’, which comprise from base to top: the Anoual Formation (lower Bathonian), the Ksar Metlili Formation (?Berriasian or upper Tithonian – lower Berriasian transition) and the Dekkar Group (?Barremian to Cenomanian). The Ksar Metlili Formation is delimited at its base by an erosional disconformity (‘D1’, Fig. 4) and at its top by a cartographic unconformity (the angle of unconformity is slight at the outcrop scale but is of regional scale and so easily recognized on a geological map; ‘D2’, Fig. 4), constituting a lenticular bedded deposit of c. 80 m thickness (Haddoumi et al. Reference Haddoumi, Charrière, Andreu and Mojon2008).

Fig. 4. Stratigraphic log and sequential evolution of the ‘Red Beds’ exposed in the Anoual Syncline, with indication of the stratigraphic position of the KM and GEA localities. Barr – Barremian; Fm – Formation. Modified from Haddoumi et al. (Reference Haddoumi, Charrière, Andreu and Mojon2008, Creative Commons BY-NC-SA 4.0).
The age of the ‘Couches Rouges’ has long been debated because of the absence of reliable chronostratigraphic markers (Monbaron, Reference Monbaron1988; Sigogneau-Russell et al. Reference Sigogneau-Russell, Monbaron and de Kaenel1990; Haddoumi et al. Reference Haddoumi, Allain, Meslouh, Métais, Monbaron, Pons, Rage, Vullo, Zouhri and Gheerbrant2016), but a Middle Jurassic to Early or early Late Cretaceous age is generally assigned to the strata (Sigogneau-Russell et al. Reference Sigogneau-Russell, Monbaron and de Kaenel1990; Zouhri et al. Reference Zouhri, Sigogneau-Russell, Haddoumi and Zouhri2017). However, opinions diverge about the temporal interpretation of this sequence. The ‘Red Beds’ containing the KM microvertebrate lens are a thick pelitic sandstone sequence that lies in stratigraphic conformity on the upper Bajocian – ?lower Bathonian marls and limestones (Pholadomya marls and limestones Formation, Fig. 4; Haddoumi et al. Reference Haddoumi, Allain, Meslouh, Métais, Monbaron, Pons, Rage, Vullo, Zouhri and Gheerbrant2016). A few dozen metres above the microvertebrate-yielding lens, there are some slightly discordant conglomerates, but the same index minerals (micas, potash feldspars and kaolinite) are present below and above the discordance D2. This led Sigogneau-Russell et al. (Reference Sigogneau-Russell, Monbaron and de Kaenel1990) to exclude the presence of a major hiatus and to suggest that the sequence may be continuous from the Bathonian to the Cenomanian. However, given the involved time span (c. 68 Ma), continuous sedimentation (i.e. the absence of an important gap) would imply incredibly low sedimentation rates, which are unlikely in the geodynamic context. Haddoumi et al. (Reference Haddoumi, Charrière, Andreu and Mojon2008) recognized instead a major discontinuity (D1) between the Anoual and Ksar Metlili formations. In the south of the Anoual Syncline it is represented by a hardened surface, often ferruginous, which incises the marine upper member of the Anoual Formation (Fig. 4: D1). The Ksar Metlili Formation directly overlies the continental lower member of the Anoual Formation (Fig. 4) in several sections described by Haddoumi et al. (Reference Haddoumi, Charrière, Andreu and Mojon2008).
The original Berriasian age of the KM microvertebrate site was based on the discovery of calcareous nannofossils resembling the genera Polycostella (Tithonian–lower Berriasian), Micrantholithus (Berriasian–Aptian) and Nannoconus (upper Tithonian–Barremian) in a marly horizon located just below the microvertebrate-yielding lens (Sigogneau-Russell et al. Reference Sigogneau-Russell, Monbaron and de Kaenel1990). The presence the selachian Egertonodus basanus at the KM fossil locality would also support this Berriasian age (Duffin & Sigogneau-Russell, Reference Duffin and Sigogneau-Russell1993). Gyrogonites of the Porocharaceae Porochara maxima have been identified 5 to 7 m above the KM lens (Haddoumi et al. Reference Haddoumi, Charrière, Andreu and Mojon2008; Mojon et al. Reference Mojon, Haddoumi and Charrière2009). This species is restricted to the late Tithonian and early Berriasian period, thus suggesting that the top of the Ksar Metlili Formation was deposited during this interval. However, all those datings (calcareous nannofossils, charophytes and fauna) are based only on the presence of isolated species and not on faunal associations, which would give more reliable information on the Ksar Metlili Formation age, as was acknowledged by Sigogneau-Russell et al. (Reference Sigogneau-Russell, Monbaron and de Kaenel1990).
2.b. Sedimentary facies
The sedimentary facies of the Ksar Metlili Formation are similar to those of the continental lower beds of the Anoual Formation (fine-to-medium-grained sandstones, overturned cross-stratifications, ripple marks, root traces; Haddoumi et al. Reference Haddoumi, Charrière, Andreu and Mojon2008). The Ksar Metlili Formation was likely deposited in a river-dominated deltaic plain, close to the sea (Sigogneau-Russell et al. Reference Sigogneau-Russell, Monbaron and de Kaenel1990, Reference Sigogneau-Russell, Evans, Levine, Russell, Lucas, Kirkland and Estep1998; Haddoumi et al. Reference Haddoumi, Charrière, Andreu and Mojon2008). All the vertebrate-bearing beds sampled in the various KM loci show this kind of transitional facies. The KM microvertebrate lens is intercalated between two marine horizons (Sigogneau-Russell et al. Reference Sigogneau-Russell, Monbaron and de Kaenel1990, Reference Sigogneau-Russell, Evans, Levine, Russell, Lucas, Kirkland and Estep1998). There is a mixing of continental (wood, mammals, dinosaurs, etc.), brackish (bivalves) and undisputedly marine (echinoderms, coral, brachiopods, etc.) forms in this horizon, which suggests a near-shore, deltaic depositional environment, probably influenced by eustatic variations (Sigogneau-Russell et al. Reference Sigogneau-Russell, Monbaron and de Kaenel1990).
3. Materials and methods
The fossil microvertebrates reported here were discovered in a 0.2 m thick lenticular calcareous sandstone covering a surface of c. 200 m2; and was first sampled in the 1980s (Sigogneau-Russell et al. Reference Sigogneau-Russell, Evans, Levine, Russell, Lucas, Kirkland and Estep1998; Jones et al. Reference Jones, Evans and Sigogneau-Russell2003; Fig. 4). In 2010 we resampled six fossiliferous loci, separated a few metres apart (KM-A1, KM-A2, KM-B’, KM-C, KM-D1 and KM-D2; Fig. 3), in the same calcareous fossiliferous sandstone bed.
The KM faunal assemblage consists of microvertebrate fossil remains with a size range of 0.5 to 30 mm, but most elements are millimetre sized. It includes the remains of various taxa, which have been disarticulated, fragmented and transported. It is dominated by isolated teeth and fish scales. This taphocoenosis provides a partial picture of the palaeocommunity. However, it has been demonstrated that such microvertebrate sites remain broadly representative of the local palaeobiodiversity (Rogers & Brady, Reference Rogers and Brady2010; Cullen et al. Reference Cullen, Fanti, Capobianco, Ryan and Evans2016). By giving a general overview of it, such sites highlight the link between large-scale environmental and faunal changes, and they are important biochronological and palaeoenvironmental indicators (Rogers & Brady, Reference Rogers and Brady2010; Cullen et al. Reference Cullen, Fanti, Capobianco, Ryan and Evans2016).
3.a. Material
Except for the pterosaur and dinosaur material (Knoll, Reference Knoll2000; Knoll & Ruiz-Omeñaca, Reference Knoll and Ruiz-Omeñaca2009), the original KM collections made by Sigogneau-Russell and colleagues in the 1980s (prefix MCM or SA) are housed at the MNHN. The new material collected in 2010, which comprises 24 541 microvertebrate elements, will be housed, after study, at the Faculty of Sciences Aïn Chock, University Hassan II, Casablanca, Morocco (with the prefix FSAC-KM). Full details of specimen numbers are given in the online Supplementary Material Table S1.
3.b. Specimen preparation
The newly sampled blocks of calcareous sandstone containing the KM microremains were processed by acid attack, using 7 % formic acid saturated with tricalcium phosphate. The lithoclastic and bioclastic sand recovered following this process was reconcentrated using hydrogen peroxide; size fractions were separated from each other using sieves of 5, 2, 1 and 0.5 mm mesh and then sorted under stereomicroscope.
The counting of the vertebrate microremains was made using the ‘Count Image Elements’ script specially developed by Auréliane Gailliègue (pers. comm. to ML, April 2017). It is based on the principle of picture segmentation: the elements are pictured on a white background; the script then analyses the picture and groups together the pixels with the same grey level. A grey level threshold value is calculated using the Otsu method (see Sezgin & Sankur, Reference Sezgin and Sankur2004 for details) and defines two pixel categories: the pixels with a grey level above the threshold value (i.e. constituting the fossils) are attributed to one category; those with a grey level below this value (i.e. constituting the background) are attributed to the other. The number of pixel groups in the first category is the number of fossils. Thus, this script allows the counting of elements on the basis of the difference in contrast.
4. Review of the Ksar Metlili fauna
4.a. Faunal list and palaeobiodiversity of the Ksar Metlili fauna
The KM collections made by D. Sigogneau-Russell and colleagues in the 1980s have been presented in numerous publications (Sigogneau-Russell et al. Reference Sigogneau-Russell, Monbaron and Russell1988, Reference Sigogneau-Russell, Monbaron and de Kaenel1990, Reference Sigogneau-Russell, Evans, Levine, Russell, Lucas, Kirkland and Estep1998; Sigogneau-Russell, Reference Sigogneau-Russell1989, Reference Sigogneau-Russell1991a, Reference Sigogneau-Russell1991b, Reference Sigogneau-Russell1991c, Reference Sigogneau-Russell1992, Reference Sigogneau-Russell1995a, Reference Sigogneau-Russell1995b, Reference Sigogneau-Russell1999, Reference Sigogneau-Russell2003; Duffin & Sigogneau-Russell, Reference Duffin and Sigogneau-Russell1993; Sigogneau-Russell & Ensom, Reference Sigogneau-Russell and Ensom1994, Reference Sigogneau-Russell and Ensom1998; Broschinski & Sigogneau-Russell, Reference Broschinski and Sigogneau-Russell1996; Evans & Sigogneau-Russell, Reference Evans and Sigogneau-Russell1997, Reference Evans and Sigogneau-Russell2001; Knoll, Reference Knoll2000; Gardner et al. Reference Gardner, Evans and Sigogneau-Russell2003; Hahn & Hahn, Reference Hahn and Hahn2003; Jones et al. Reference Jones, Evans and Sigogneau-Russell2003; Knoll & Ruiz-Omeñaca, Reference Knoll and Ruiz-Omeñaca2009); these include the microvertebrate fauna from the ‘Séquence B des Couches Rouges’ of the Anoual Syncline (Sigogneau-Russell et al. Reference Sigogneau-Russell, Monbaron and de Kaenel1990), now called the Ksar Metlili Formation (Haddoumi et al. Reference Haddoumi, Charrière, Andreu and Mojon2008). Here we provide an updated taxonomic list of the KM microvertebrate fauna, based both on the old and new collections (Table 1).
Table 1. Faunal list of the microvertebrates from the Ksar Metlili locality, Anoual Syncline, eastern Morocco, with details for each local sampled locus*

* Taxa in grey are new with respect to the old collections and determinations.
The 1980s collections comprise 271 numbered specimens, belonging to at least 39 species, among which 22 were new and described for the first time: one selachian, one albanerpetontid, one caecilian, one anuran, one sphenodontian, one squamate and 16 mammals. The new collections contain 30 species (Tables 2, 3). Considering the entire collection and shared species of both old and new collections, there are at least 47 vertebrate species in the KM fauna.
Table 2. Minimum number of species of the microvertebrate taxa from the Ksar Metlili site

Table 3. Vertebrate relative abundances in the different loci of the Ksar Metlili site (% of total vertebrate microremains collected)

For actinopterygians and crocodylomorphs, only teeth were considered (see Section 6.a). The most abundant taxa are bolded.
4.b. Taxonomic composition of the Ksar Metlili fauna
4.b.1. Chondrichthyes
In the KM fauna, the chondrichthyans are only represented by elasmobranchs. Two Hybodontiformes genera have been identified, Egertonodus basanus and Lonchidion marocensis (Duffin & Sigogneau-Russell, Reference Duffin and Sigogneau-Russell1993; Rees & Underwood, Reference Rees and Underwood2002). The Valanginian–Aptian stratigraphic range of E. basanus (Biddle & Landemaine, Reference Biddle and Landemaine1988; Duffin & Sigogneau-Russell, Reference Duffin and Sigogneau-Russell1993; Frederickson et al. Reference Frederickson, Lipka and Cifelli2018) would tend to confirm a Cretaceous age for the Ksar Metlili deposit, although it does not provide a full corroboration of the previously suggested Berriasian age (Duffin & Sigogneau-Russell, Reference Duffin and Sigogneau-Russell1993). Moreover, E. basanus could be a synonym of E. duffini, described from the middle Bathonian of Great Britain (Rees & Underwood, Reference Rees and Underwood2008), so Egertonodus does not provide any precision on the biostratigraphic dating of the KM fossil locality.
4.b.2. Actinopterygii
Ginglymode actinopterygian remains are predominant in the KM limestones. Because of the incompleteness of the majority of the scales, it is difficult to make the distinction between Lepisosteiformes and Semionotiformes, and we thus assign the material to Ginglymodi indet. Pycnodontiformes, Osteoglossiformes and, tentatively, Cypriniformes are also identified (Duffin & Sigogneau-Russell, Reference Duffin and Sigogneau-Russell1993; Sigogneau-Russell et al. Reference Sigogneau-Russell, Evans, Levine, Russell, Lucas, Kirkland and Estep1998). Some spine-shaped bones in the new material are reminiscent of pectoral or dorsal fin spines of Siluriformes (see Argyriou et al. Reference Argyriou, Cook, Muftah, Pavlakis, Boaz and Murray2015; Kovalchuk & Ferraris, Reference Kovalchuk and Ferraris2016; Vallone et al. Reference Vallone, Vezzosi and Cione2017 for comparison material) or Polypteriformes (see Daget et al. Reference Daget, Gayet, Meunier and Sire2001 and Gayet et al. Reference Gayet, Meunier and Werner2002 for comparison). These are elongated and flat bones with one end larger than the other. This larger, proximal end bears a bulge with four processes that suggest articular facets, two on the dorsal face and two on the ventral face (Fig. 5). We tentatively assign them to ?Siluriformes or ?Polypteriformes. At present, the oldest fossil record of Siluriformes varies from 72 Ma (Gayet & Meunier, Reference Gayet and Meunier2003) to 88.6 Ma; Patterson, Reference Patterson and Benton1993; Gayet & Otero, Reference Gayet and Otero1999). The earliest fossil occurrence of polypteriforms is Cenomanian (Gayet et al. Reference Gayet, Meunier and Werner2002). Thus, if one of these attributions is confirmed, then we shift back the earliest fossil occurrence of one of these groups by 45 million years at least. Thin-sections of these bones are currently under histological study and could confirm or modify this assignment, as some features in the bone microstructure could be reminiscent of what can be seen in the Holostei.

Fig. 5. Possible fin spine of ?Siluriformes or ?Polypteriformes indet. (KM-A1-40). (a) Dorsal view. (b) Ventral view. (c) Close-up on proximal end, dorsal view. (d) Close-up on proximal end, ventral view. af – articular facet (?).
4.b.3. Sarcopterygii
The 2010 material includes lungfish dental plates similar to the material referred to Ptychoceratodontidae in the old collections (Duffin & Sigogneau-Russell, Reference Duffin and Sigogneau-Russell1993; Sigogneau-Russell et al. Reference Sigogneau-Russell, Evans, Levine, Russell, Lucas, Kirkland and Estep1998), and so probably belongs to the same family. It also includes an actinistian postparietal shield: its ornamentation, made of subcircular grooves and of antero-posteriorly oriented subparallel gutters (Fig. 6a), is typical of the Mawsoniidae (G. Clément, pers. comm. ML, March 2017). The notch observed in the lateral view of the external edge is probably the vestige of the otic canal (Fig. 6b). Thus, we refer this bone to cf. Mawsoniidae.

Fig. 6. Left postparietal shield of cf. Mawsoniidae indet. (KM-A2-11). (a) Dorsal view. (b) Left lateral view. oc – otic canal.
4.b.4. Lissamphibia
4.b.4.a. Albanerpetontidae (Fig. 7a, b)
The presence of an albanerpetontid, Anoualerpeton unicus, both in old and new collections, has to be emphasized. New remains of this species (Fig. 7a, b) increase the available material for this only known Gondwanan representative of albanerpetontids (Sigogneau-Russell et al. Reference Sigogneau-Russell, Evans, Levine, Russell, Lucas, Kirkland and Estep1998; Gardner et al. Reference Gardner, Evans and Sigogneau-Russell2003). The genus is also present in the upper Bathonian of the Kirtlington Cement Quarry (‘Kirtlington Mammal Bed’, Forest Marble Formation), Great Britain, where it is represented by the species A. priscus (Gardner et al. Reference Gardner, Evans and Sigogneau-Russell2003).

Fig. 7. Lissamphibians. (a) Fused frontals (A: KM-A2-7) of Anoualerpeton unicus in (a1) dorsal and (a2) ventral views. (b) Left dentary (B: KM-B’-12) of same in (b1) lingual and (b2) labial views. (c) Dorsal vertebra of cf. Alytidae indet. (KM-B’-18) in (c1) dorsal and (c2) ventral views.
4.b.4.b. cf. Alytidae (Fig. 7c)
The material collected in 2010 yielded 15 stegochordal, opisthocoelous vertebrae, wider than long, with short, robust and distally enlarged transverse processes that were unknown in the old collections and that we refer to cf. Alytidae (Holman, Reference Holman2003; S. Bailon, pers. comm. to ML, November 2018; Fig. 7c); this identification must be considered provisional, because the anuran postcranial skeleton is always more uniform than the skull and because the material is fragmentary. Before the discovery of the KM fauna, alytids were unknown in Gondwana until the Late Cretaceous period of India (Prasad & Rage, Reference Prasad and Rage1991). Consequently, the KM locality yields the oldest known Gondwanan alytids if the present identification is confirmed.
In the old collections, two other anuran species were discovered: the alytid aff. Enneabatrachus sp. and the pipioid or pelobatoid Aygroua anoualensis (Jones et al. Reference Jones, Evans and Sigogneau-Russell2003). A caecilian species, Rubricacaecilia monbaroni, was also recognized: it is the earliest Gondwanan caecilian record (Sigogneau-Russell et al. Reference Sigogneau-Russell, Evans, Levine, Russell, Lucas, Kirkland and Estep1998; Evans & Sigogneau-Russell, Reference Evans and Sigogneau-Russell2001).
4.b.5. Choristodera
Some robust vertebrae unknown in the old KM collections are found in the new material (Fig. 8): their centrum is amphicoelous or amphiplatyan, with an opened neurocentral suture and a distinct longitudinal ridge bordered laterally by longitudinal grooves on the floor of the neural canal, the cotyle rims are thick, and a slight ventral constriction gives them a coil form; all these characters are reminiscent of choristoderan reptiles (Evans, Reference Evans1991; Averianov et al. Reference Averianov, Martin, Evans and Bakirov2006; Vullo et al. Reference Vullo, Abit, Ballèvre, Billon-Bruyat, Bourgeais, Buffetaut, Daviero-Gomez, Garcia, Gomez, Mazin, Morel, Néraudeau, Pouech, Rage, Schnyder and Tong2014; Haddoumi et al. Reference Haddoumi, Allain, Meslouh, Métais, Monbaron, Pons, Rage, Vullo, Zouhri and Gheerbrant2016), so we assign them to this group, although this identification needs to be substantiated with additional material. These freshwater or amphibious diapsids are well known in Laurasia (Matsumoto et al. Reference Matsumoto, Buffetaut, Escuillié, Hervet and Evans2013), but up to now the first and only known Gondwanan choristoderes had been recovered from the Middle Jurassic of the Guelb el Ahmar locality, Morocco (Haddoumi et al. Reference Haddoumi, Allain, Meslouh, Métais, Monbaron, Pons, Rage, Vullo, Zouhri and Gheerbrant2016). Thus, the KM specimens represent one of the few occurrences of choristoderes in Gondwana.

Fig. 8. Dorsal vertebrae of Choristodera indet. (a) KM-B’-49 in (a1) ventral, (a2) dorsal and (a3) anterior (?) views. (b) KM-D1-18 in (b1) ventral, (b2) dorsal and (b3) anterior (?) views. lr = longitudinal ridge; ns – neurocentral suture.
4.b.6. Testudinata
The turtles from the KM material are currently under study. Tentative identification of shell fragments suggests they could be represented by three taxa with a great deal of reservation: Chelonii indet., Pelomedusoides indet. and ?Araripemydidae indet. (cf. Taquetochelys sp.?; Gmira, Reference Gmira1995; Zouhri et al. Reference Zouhri, Sigogneau-Russell, Haddoumi and Zouhri2017). The rest of the material is referred to as Pleurodira indet., Cryptodira indet. or Testudinata indet.
4.b.7. Lepidosauromorpha
The KM lepidosauromorphs are represented by sphenodontians and squamates. At least two different sphenodontians are present: Tingitana anoualae (Evans & Sigogneau-Russell, Reference Evans and Sigogneau-Russell1997) and an unnamed but distinct taxon referred to as ‘Sphenodontia B’ by Evans & Sigogneau-Russell (Reference Evans and Sigogneau-Russell1997). They were considered as the first post-Jurassic record of the group in Africa (Evans & Sigogneau-Russell, Reference Evans and Sigogneau-Russell1997; Zouhri et al. Reference Zouhri, Sigogneau-Russell, Haddoumi and Zouhri2017), but the Berriasian age for the KM fauna is here reassessed as possibly Jurassic (see Section 6.c below).
Squamates are represented by scincomorphs, with the species Paramacellodus marocensis (Richter, Reference Richter1994), and by an incertae sedis fossorial lizard, Tarratosaurus anoualensis (Broschinski & Sigogneau-Russell, Reference Broschinski and Sigogneau-Russell1996).
4.b.8. Crocodylomorpha
At least two types of crocodylomorphs were reported in the old KM collections (Sigogneau-Russell et al. Reference Sigogneau-Russell, Monbaron and de Kaenel1990, Reference Sigogneau-Russell, Evans, Levine, Russell, Lucas, Kirkland and Estep1998), but they have never been identified or studied. The new material highlights four types of crocodylomorphs: cf. Theriosuchus, teleosaurids, atoposaurids and a robust-toothed undetermined form.
4.b.8.a. cf. Theriosuchus (Fig. 9a)
Two fused premaxillae ornamented with subcircular grooves were recovered in the 2010 material (Fig. 9a). Given the small size of the premaxillary ascending process (Fig. 9a), we can suppose that the division of external nares was made essentially by the anterior nasal process, which is reminiscent of the genus Theriosuchus (T. pusillus and T. grandinaris; J. P. Tennant, pers. comm. to ML, May 2017); thus, we tentatively assign this specimen to cf. Theriosuchus (Tennant et al. Reference Tennant, Mannion and Upchurch2016).

Fig. 9. Crocodylomorpha. (a) Fused premaxillaries of cf. Theriosuchus sp. indet. (KM-B’-42) in (a1) dorsal, (a2) left lateral and (a3) ventral views. (b) Tooth of Teleosauridae indet. (KM-B’-53). (c) Tooth of Atoposauridae indet. (KM-C-2) in (c1) labial and (c2) lingual views. pap – premaxillary ascending process.
4.b.8.b. Teleosauridae (Fig. 9b)
Teleosaurids are identified by slender, elongated, conical teeth, slightly recurved backwards and bearing numerous thin and regularly spaced longitudinal striae, converging towards the apex. They could be confused with caniniform teeth of atoposaurids, but within teleosaurids the tooth ornamentation is more pronounced, with higher ridges (Vignaud, Reference Vignaud1997; Haddoumi et al. Reference Haddoumi, Allain, Meslouh, Métais, Monbaron, Pons, Rage, Vullo, Zouhri and Gheerbrant2016), and overall the cross-section is subcircular, the crown is slightly compressed labiolingually but less than in atoposaurids, and carinae are weak to absent (Haddoumi et al. Reference Haddoumi, Allain, Meslouh, Métais, Monbaron, Pons, Rage, Vullo, Zouhri and Gheerbrant2016), contrary to atoposaurid teeth (see, for example, Schwarz-Wings et al. Reference Schwarz-Wings, Rees and Lindgren2009). The ratio between the longitudinal diameter of the crown base and the crown height is >3.5 (S. Jouve, pers. comm. to ML, May 2017; Vignaud, Reference Vignaud1997; Fig. 9b). They closely resemble the teeth observed in teleosaurids such as Teleosaurus, Pelagosaurus and Steneosaurus (Vignaud, Reference Vignaud1997; Pierce & Benton, Reference Pierce and Benton2006) and to those identified as ‘Teleosauridae indet.’ in the GEA fauna (Haddoumi et al. Reference Haddoumi, Allain, Meslouh, Métais, Monbaron, Pons, Rage, Vullo, Zouhri and Gheerbrant2016). These teleosaurid teeth could be related to the morphotype A3 described by Vignaud (Reference Vignaud1997).
4.b.8.c. Atoposauridae (Fig. 9c)
Atoposaurids are identified by numerous lanceolate to leaf-shaped dental crowns, labiolingually compressed with a lingual concavity and both mesial and distal carinae; both lingual and labial faces bear thin vertical wrinkles diverging towards the carinae (Vullo et al. Reference Vullo, Abit, Ballèvre, Billon-Bruyat, Bourgeais, Buffetaut, Daviero-Gomez, Garcia, Gomez, Mazin, Morel, Néraudeau, Pouech, Rage, Schnyder and Tong2014; Fig. 9c). The teeth here described as belonging to atoposaurids are frequently assigned to Theriosuchus sp. in the case of Jurassic material (Vullo et al. Reference Vullo, Abit, Ballèvre, Billon-Bruyat, Bourgeais, Buffetaut, Daviero-Gomez, Garcia, Gomez, Mazin, Morel, Néraudeau, Pouech, Rage, Schnyder and Tong2014), even if an alternative attribution cannot be definitively rejected.
Atoposaurids and Theriosuchus are thought to be mostly, if not strictly, terrestrial crocodylomorphs, and their good representation in the KM fossil material points to a mostly terrestrial palaeoenvironment. It is worth noting that Theriosuchus is usually considered as an atoposaurid (Young et al. Reference Young, Tennant, Brusatte, Challands, Fraser, Clark and Ross2016), although for some authors this is a polyphyletic taxon that includes two groups of advanced neosuchians that would not belong to the Atoposauridae (Tennant et al. Reference Tennant, Mannion and Upchurch2016).
Teleosaurids are, by contrast, strictly aquatic, and are often considered as purely marine reptiles (Bardet, Reference Bardet1995). However, some recent discoveries showed that some teleosaurids were able to live in freshwater (Martin et al. Reference Martin, Deesri, Liard, Wattanapituksakul, Suteethorn, Lauprasert, Tong, Buffetaut, Suteethorn, Suan, Telouk and Balter2015); the KM specimens are among the first-known possible freshwater teleosaurids, and so constitute new evidence in favour of freshwater habitats for the family. Given the size of their teeth in the KM material they were probably young individuals (as for the GEA specimens; Haddoumi et al. Reference Haddoumi, Allain, Meslouh, Métais, Monbaron, Pons, Rage, Vullo, Zouhri and Gheerbrant2016), which might suggest that they lived in a freshwater ‘nursery’ before moving to the sea (S. Jouve, pers. comm. to ML, May 2017).
All these crocodylomorph taxa are under study and will be described in a dedicated paper (ML Ph.D. work in progress).
4.b.9. Pterosauria
Two pterosaur groups can be recognized in the KM fauna (Knoll, Reference Knoll2000): one with slender, elongated teeth quite similar to those of ctenochasmatid pterosaurs such as Gnathosaurinae (Fig. 10a), and another with stronger and smoother teeth, reminiscent of the Ornithocheiridae (Fig. 10b), according to Knoll (Reference Knoll2000). However, this second dental morphotype is also known within Rhamphorhynchidae (Averianov et al. Reference Averianov, Martin and Bakirov2005; Vullo et al. Reference Vullo, Abit, Ballèvre, Billon-Bruyat, Bourgeais, Buffetaut, Daviero-Gomez, Garcia, Gomez, Mazin, Morel, Néraudeau, Pouech, Rage, Schnyder and Tong2014), so the occurrence of Ornithocheiridae in the KM fauna cannot be confirmed without additional diagnostic material; furthermore, the small size (less than 20 mm) and the possible Jurassic age we suggest here for the KM fauna would rather favour its assignment to ?Rhamphorhynchidae.

Fig. 10. Pterosauria. (a) Tooth of Ctenochasmatidae (?Gnathosaurinae) indet. (KM-A2-21). (b) Tooth of ?Rhamphorhynchidae indet. (KM-A1-38). ca – carina.
Though the KM ?Gnathosaurinae teeth represent one of the rare Cretaceous occurrences of the group (Knoll, Reference Knoll2000; Sweetman & Martill, Reference Sweetman and Martill2010), and they are among the latest representatives of this group while being the first discovered in Africa (Knoll, Reference Knoll2000), these results must be considered with caution, since their teeth are frequently confused with those of teleosaurid crocodylomorphs (see, for example, Buffetaut & Jeffery, Reference Buffetaut and Jeffery2012), so a thorough revision of the KM pterosaur material is needed.
4.b.10. Dinosauria
4.b.10.a. Ornithischia (Fig. 11)
Most of the dinosaur remains from the old KM collections are still undetermined and unstudied. Here, we report the discovery in the 2010 material of a single ornithischian tooth (Fig. 11) that cannot be assigned to any known species and seems to represent a basal species of ornithischian, even if it is relatively young in geological age. The crown is slightly compressed laterally and shows a strong cingulum; it bears five well-developed cusps, aligned in the antero-posterior plane, with a central cusp about twice as high as the others; the lingual face of the crown is slightly concave; the root is unique, robust, and about one and a half times the size of the crown in height (Fig. 11). It is somewhat similar to the ‘Ornithopoda basal indet. forme A’ described by J. Pouech (unpub. Ph.D. thesis, Claude Bernard – Lyon 1 University, 2008) from the Berriasian of Cherves-de-Cognac (Charente, France). It can also be compared to a recently described neornithischian tooth from the Cenomanian of Utah (Avrahami et al. Reference Avrahami, Gates, Heckert, Makovicky and Zanno2018). To a lesser degree, it also resembles the tooth from the Barremian of England attributed to an undetermined ornithischian or possibly referable to a heterodontosaurid by Sweetman (Reference Sweetman2016). This tooth from the KM fauna is also reminiscent of those described by Thulborn (Reference Thulborn1973) from the Kimmeridgian of Portugal and referred to the hypsilophodontid Trimucrodon cuneatus (nomen dubium); however, in T. cuneatus, the mesialmost and distalmost denticles are salient and diverge away from the crown, which is not the case in the KM specimen. Further investigations are in progress, and this specimen will be described in a future publication (ML Ph.D. work in progress).

Fig. 11. Tooth of Ornithischia indet. (KM-A2-8). (a) Lingual view. (b) Labial view. (c) Occlusal view.
4.b.10.b. Theropoda
The theropods are only documented by teeth, and most of them are related to Theropoda indet., Maniraptoriformes indet. or Maniraptora indet. (Knoll & Ruiz-Omeñaca, Reference Knoll and Ruiz-Omeñaca2009). Knoll & Ruiz-Omeñaca (Reference Knoll and Ruiz-Omeñaca2009) reported the presence of three Velociraptorinae morphotypes, but this should be considered cautiously owing to the difficulty in identifying a theropod subfamily from only isolated teeth (Hendrickx et al. Reference Hendrickx, Mateus and Araújo2015). A large part of these taxa could rather be related to Dromaeosauridae, which would be one of the earliest occurrences of this group, together with the teeth from the Upper Jurassic of Guimarota (Rauhut, Reference Rauhut, Martin and Krebs2000) and the specimens from the Barremian of China (Norell & Makovicky, Reference Norell, Makovicky, Weishampel, Dodson and Osmólska2004). Isolated teeth tentatively attributed to dromaeosaurid-like theropods are reported from the Middle Jurassic of England (Metcalf et al. Reference Metcalf, Vaughan, Benton, Cole, Simms and Dartnall1992), but their identification is uncertain and no fossil bones have been recovered from the Middle Jurassic so far. However, it is possible that those potential dromaeosaurid teeth from the KM fauna belong instead to Noasauridae or a basal tyrannosauroid (C. Hendrickx, pers. comm. to ML, March 2018). An in-depth revision of the KM theropod material, in progress, is beyond the scope of the present study.
4.b.11. cf. Cynodontia
Two tooth morphotypes that cannot be related to any of the identified major groups at Ksar Metlili were found in the new material collected in 2010 (Fig. 12; Lasseron, Reference Lasseron2019). The first morphotype (Fig. 12a) consists of uniradiculate teeth with a slightly lingually curved and smooth crown, but some striae are observable on the labial face apex (Fig. 12a; see Lasseron, Reference Lasseron2019 for details). The second dental morphotype (Fig. 12b) is broadly similar, but the lateral compression is not symmetrical (the labial face is more convex; Fig. 12b3) and there is no apical ridge (see Lasseron, Reference Lasseron2019 for details).

Fig. 12. cf. Cynodontia. (a) Tooth of morphotype 1 (KM-B’44) in (a1) labial, (a2) lingual and (a3) occlusal views. (b) Tooth of morphotype 2 (KM-?-1) in (b1) labial, (b2) lingual and (b3) occlusal views.
These teeth are similar in morphology to single-rooted premolariform teeth of the small gobiconodontid BMNH M 45513 described by Sweetman (Reference Sweetman2006) from the Barremian of southern England (S. C. Sweetman, pers. comm. to ML, September 2018), and those of Gobiconodon borrisiaki from the Aptian–Albian of Mongolia (Kielan-Jaworowska & Dashzeveg, Reference Kielan-Jaworowska and Dashzeveg1998). However, they differ from both of them in several points (straight root, less clearly separated accessory cusps, presence of cingulum, etc. See Lasseron, Reference Lasseron2019 for details). These teeth are also somewhat similar to those figured by Lucas & Oakes (Reference Lucas and Oakes1988) and Godefroit & Battail (Reference Godefroit and Battail1997) and identified as non-mammaliaform cynodonts (Pseudotriconodon, Gaumia). They are also similar to the tricuspid postcanine teeth of ‘dromatheriids’, such as Microconodon tenuirostris (Simpson, Reference Simpson1926; Sues et al. Reference Sues, Olsen, Kroehler, Fraser and Sues1994; Sues, Reference Sues2001), Rewaconodon tikiensis (Datta et al. Reference Datta, Das and Luo2004) and Polonodon woznikiensis (Sulej et al. Reference Sulej, Niedźwiedzki, Tałanda, Dróżdż and Hara2018) from the lower Upper Triassic of the United States, India and Poland, respectively. Considering this ‘dromatheriid’ resemblance and the differentiation made by Datta et al. (Reference Datta, Das and Luo2004) between the teeth of Rewaconodon, the KM specimens pictured in Figure 12a (KM-B’-44) and in Figure 12b (KM-?-1) may represent an anteriormost ‘premolariform’ and a posterior postcanine tooth, respectively. Thus, the KM specimens are tentatively referred to one or two species of non-mammaliform cynodonts (F. Abdala, pers. comm. to ML, September 2018). If their identification is confirmed, they would be among the last representatives of the non-mammaliaform cynodonts, along with the tritylodontids Montiricus kuwajimaensis from the Barremian/Aptian of Japan (Setoguchi et al. Reference Setoguchi, Matsuda, Matsuoka, Wang and Deng1999; Matsuoka & Setoguchi, Reference Matsuoka and Setoguchi2000; Matsuoka et al. Reference Matsuoka, Kusuhashi and Corfe2016), Xenocretosuchus sibiricus and X. kolossovi from the ?Valanginian–Hauterivian–Barremian and Barremian–Aptian of Russia, respectively (Tatarinov & Mashchenko, Reference Tatarinov and Mashchenko1999; Lopatin & Agadjanian, Reference Lopatin and Agadjanian2008), and Stereognathus sp. from the Bathonian of Russia (Averianov et al. Reference Averianov, Martin, Lopatin, Schultz, Skutschas, Rico and Krasnolutskii2017). The detailed study of those possible cynodontian teeth will be published separately (Lasseron, Reference Lasseron2019).
4.b.12. Mammalia
Mammals are remarkably diverse in the KM fauna. At least 16 species were identified in the old collections, and unpublished specimens belonging to new taxa remain to be described. Eutriconodontans are represented by Dyskritodon amazighi, Ichthyoconodon jaworowskorum (Sigogneau-Russell, Reference Sigogneau-Russell1995b), Gobiconodon palaios and Kryptotherium polysphenos (Sigogneau-Russell, Reference Sigogneau-Russell2003). Sigogneau-Russell (Reference Sigogneau-Russell1995b) considered I. jaworowskorum and D. amazighi as semi-aquatic mammals. More recently, Gaetano & Rougier (Reference Gaetano and Rougier2011) referred I. jaworowskorum to the Volaticotherini, which are gliding mammals. Allotherians are represented by two species, Hahnodon taqueti (Sigogneau-Russell, Reference Sigogneau-Russell1991b; Hahn & Hahn, Reference Hahn and Hahn2003) and Denisodon moroccensis (Hahn & Hahn, Reference Hahn and Hahn2003), which are referred to the family Hahnodontidae. Recently Huttenlocker et al. (Reference Huttenlocker, Grossnickle, Kirkland, Schultz and Luo2018) showed that the Hahnodontidae does not belong to the Multituberculata but to the Haramiyida. Five species of ‘Symmetrodonta’ (Trechnotheria) are present: Atlasodon monbaroni, Microderson laaroussii (Sigogneau-Russell, Reference Sigogneau-Russell1991a), Thereuodon dahmanii (Sigogneau-Russell, Reference Sigogneau-Russell1989; Sigogneau-Russell & Ensom, Reference Sigogneau-Russell and Ensom1998; the holotype of this species has also been regarded as a deciduous posterior upper premolar of a holotherian, possibly zatherian, see Martin, Reference Martin2002), Minimus richardfoxi and Afriquiamus nessovi (Sigogneau-Russell, Reference Sigogneau-Russell1999). The dryolestoids are the most frequently found among the mammal material in the KM fauna, and they are represented by at least one species, Donodon perscriptoris (Sigogneau-Russell, Reference Sigogneau-Russell1991a). In addition, one species of ‘peramurid’ is present, Peramus sp. (Sigogneau-Russell, Reference Sigogneau-Russell1999). Finally, three stem boreosphenidan (tribosphenidans) species have been described by Sigogneau-Russell: Hypomylos phelizoni (Sigogneau-Russell, Reference Sigogneau-Russell1992, Reference Sigogneau-Russell1995a; Fig. 13a), H. micros (Sigogneau-Russell, Reference Sigogneau-Russell1995a) and Tribotherium africanum (Sigogneau-Russell, Reference Sigogneau-Russell1991c, Reference Sigogneau-Russell1995a; Fig. 13b). They are among the oldest known stem boresophenidan mammals and the first documented in Africa. Only four species of mammals, already described in the old collections, have been identified in the KM material collected in 2010: the dryolestoid D. perscriptoris, and the three stem boreosphenidans H. phelizoni (Fig. 13a), H. micros and T. africanum (Fig. 13b).

Fig. 13. Stem boreosphenidan mammals. (a) Left lower molar of Hypomylos phelizoni (KM-B’-4) in (a1) lingual, (a2) labial and (a3) occlusal views. (b) Right upper molar of Tribotherium africanum (KM-B’-5) in (b1) mesial, (b2) distal and (b3) occlusal views.
5. Quantitative analysis of the Ksar Metlili fauna
The number of identified taxa is broadly stable among the KM sites (Fig. 14; Table 1), with the notable exception of locality C, where only four taxa can be recognized with certainty (Figs 3, 14). However, this is not significant as locality C is much less sampled: only 81 kg of rocks were sampled there, versus 675 kg for A1, for example (12 %; Table 3).

Fig. 14. Identified species number in each locus of the Ksar Metlili site, based on the 2010 sampled material.
The fossil material from Ksar Metlili collected in 2010 has been subject to a quantitative analysis to determine the relative abundance of each taxon, in order to detect a possible taphonomic bias or a size sorting. This analysis was carried out on the raw data from the element counting (Minimum Number of Elements, MNE). However, it was impossible to reliably determine a Minimum Number of Individuals (MNI) from actinopterygian scales or teeth, archosaur teeth and turtle plates. Thus, it was considered preferable to limit the study to the MNE, in order to understand the overall structure of the vertebrate assemblage. We recovered 24 541 fossil specimens from the 1.5 tons of matrix collected from six different loci, and we identified 30 species. We have also examined the distribution disparities of the main taxa identified in the different localities (Table 3).
From the raw data from the 2010 material, it is apparent that the fauna is widely dominated by actinopterygians, representing more than 71 % of the elements, including a majority of ginglymodians (Fig. 15). Atoposaurids and other crocodylomorphs (except teleosaurids) represent 21 % of the fossils recovered in the KM fauna, and pterosaurs and turtles 2.5 % and 1.6 %, respectively (Fig. 15).

Fig. 15. Relative abundances of the Ksar Metlili taxa, based on the 2010 sampled material, considering all the elements or only teeth for actinopterygians and crocodylomorphs. Others = Vertebrata indet., Chondrichthyes, Pycnodontiformes, Osteoglossiformes, ?Siluriformes or Polypteriformes, Actinistia, Dipnoi, Tetrapoda indet., Albanerpetontidae, Anura, cf. Choristodera, Squamata, Rhynchocephalia, Teleosauridae, Ornithischia, Theropoda, ?Cynodontia, Mammalia.
6. Discussion
6.a. Faunal composition analysis and diversity: palaeoecological implications
Microvertebrate accumulations record a wide variety of taxa and provide important palaeoecological data that complement data from macroremain localities (Jamniczky et al. Reference Jamniczky, Brinkman, Russell, Sankey and Baszio2008). They provide a general overview of the local palaeobiodiversity and are important palaeoenvironmental indicators of great use for palaeoecological reconstructions (Estes & Berberian, Reference Estes and Berberian1970; Brinkman, Reference Brinkman1990; Brinkman et al. Reference Brinkman, Russell, Eberth and Peng2004; Vullo, Reference Vullo2007; Rogers & Brady, Reference Rogers and Brady2010; Cullen et al. Reference Cullen, Fanti, Capobianco, Ryan and Evans2016).
The noticeably higher frequency of fishes in (micro-)vertebrate assemblages (71 % in the KM fauna) has been interpreted to be the result of mass mortality events, probably monotaxic or with a few taxa (Wenz & Poyato-Ariza, Reference Wenz and Poyato-Ariza1994; Martill et al. Reference Martill, Brito and Washington-Evans2008), which could be linked to anoxia, algal blooms or seasonal drought phenomenon (Martill et al. Reference Martill, Brito and Washington-Evans2008). The last hypothesis is the most consistent with the sedimentary context of the KM locality. This high frequency can also be due to the large number of bones released when a fish skeleton gets disarticulated (teeth, scales, spines, vertebrae, etc.). The proportion of crocodylomorphs in the KM fauna (21 %) is consistent with the frequency of crocodylomorphs usually observed in localities yielding microremains (Sankey, Reference Sankey, Sankey and Baszio2008). Finally, four major groups – actinopterygians, crocodylomorphs, pterosaurs and turtles – represent more than 95 % of the vertebrate remains found in the KM fauna, and this assemblage appears to be autochthonous or sub-autochthonous, because the lifestyle of these taxa is consistent with the river-dominated deltaic environment of the KM locality, i.e. they were probably permanent inhabitants of the local biotope. The presence of pterosaurs (2.5 %) in this major KM faunal association dominated by aquatic taxa could be the result of a trophic relationship: it seems reasonable to assume that pterosaurs were feeding on bony fishes and/or that some of them were eaten by crocodylomorphs. Fish-eating behaviour has been frequently reported for pterosaurs, based on their dental morphology or their gut content (Witton, Reference Witton2013, Reference Witton, Hone, Witton and Martill2018). Rhamphorhynchidae (or Ornithocheiridae, see pterosaur systematics in Section 4.b.9 above) are sometimes thought to use skim-feeding, pushing their lower jaw tip through the water to snap up food upon impact (Nessov, Reference Nessov1984); even if this hypothesis has been recently discounted (see Humphries et al. Reference Humphries, Bonser, Witton and Martill2007; M. P. Witton, unpub. Ph.D. thesis, Univ. Portsmouth, 2008; Witton, Reference Witton2013), several anatomical features support dip-feeding, in which the animal gleans food from the water’s surface like terns and frigate birds (Unwin, Reference Unwin1988, Reference Unwin2005; Veldmeijer et al. Reference Veldmeijer, Signore, Bucci and Elewa2006; Witton, Reference Witton2013). Some Ctenochasmatoidea, such as Gnathosaurus and other Gnathosaurinae, could have a feeding behaviour similar to that of modern spoonbills, because of their spatulate jaw and dental profile, snatching small fishes and other swimming animals from the water column (Swennen & Yu, Reference Swennen and Yu2004; Witton, Reference Witton2013). The low abundance of the other taxa can be explained by different reasons: shed teeth for dinosaurs; the fragility of amphibian, lepidosaurian and mammal small bones; the cartilaginous skeleton of chondrichthyans and lungfishes (lungfishes are really scarce in the KM fauna, in contrast to the GEA fauna where their dental plates are frequent among the macroremains); different habitats; more transported remains, etc.
Actinopterygians are over-represented in the KM material, because of the large number of scales. This can be explained by the accumulation of individuals, the large number of preserved scales per individual and their robustness, which is characteristic of the Anoual sites. In order to correct this bias and to get as close as possible to the MNI, a second analysis was developed, considering only their teeth (Fig. 15). In the same way, we also did not count the crocodylomorph osteoderms. In this analysis, actinopterygians only represent 13 % of the fauna, and the fauna appears dominated instead by crocodylomorphs, including atoposaurids (65 %), followed by pterosaurs (8 %) and turtles (5 %), as in the first analysis. Even if this correction still does not represent an exact picture of the palaeobiocoenosis, these results are consistent with the depositional environment of the KM locality. A similar pattern of relative abundances is found in all the KM sampled loci, suggesting the depositional environment and the faunal composition are broadly homogeneous in all the loci.
6.b. Taphonomy and representativeness of the identified faunal diversity
In order to establish comparisons of the taphonomic characters of the different studied sites, an analysis of fossil size-frequency distribution in the KM assemblage is essential (Blob & Fiorillo, Reference Blob and Fiorillo1996; Brinkman et al. Reference Brinkman, Russell, Eberth and Peng2004; Vullo, Reference Vullo2007). Blob & Fiorillo (Reference Blob and Fiorillo1996) showed that this distribution may vary considerably between sites, even if they have identical sedimentological characteristics, because of the taxonomic composition. Thus, in order to reduce this taxonomic bias, only undetermined fossil remains have to be included in the analysis (Blob & Fiorillo, Reference Blob and Fiorillo1996; Brinkman et al. Reference Brinkman, Russell, Eberth and Peng2004; Vullo, Reference Vullo2007). If microvertebrate assemblages have similar size distributions, the taphonomic explanation for faunal differences can be rejected (Blob & Fiorillo, Reference Blob and Fiorillo1996; Brinkman et al. Reference Brinkman, Russell, Eberth and Peng2004), and the taxonomic composition of the assemblages can be properly compared (in terms of relative abundances of taxa).
For each locality, the measurements of maximum length were made on a set of randomly drawn fossils (n = 278 to 729) (Fig. 16) devoid of any fresh breaks linked with the collecting or sorting process (see online Supplementary Material Table S2 for details). To test whether the size difference was significant and thus the similarity of the size-frequency distributions, non-parametric Kolmogorov-Smirnov tests were applied in the comparison of the size-frequency distributions (Brinkman et al. Reference Brinkman, Russell, Eberth and Peng2004). The null hypothesis (the observed differences among the sites are not significant, and so the sites are not significantly different) cannot be rejected at the 0.05 threshold (online Supplementary Material Table S3). Thus, faunal differences may have a biological basis, rather than a taphonomic one.
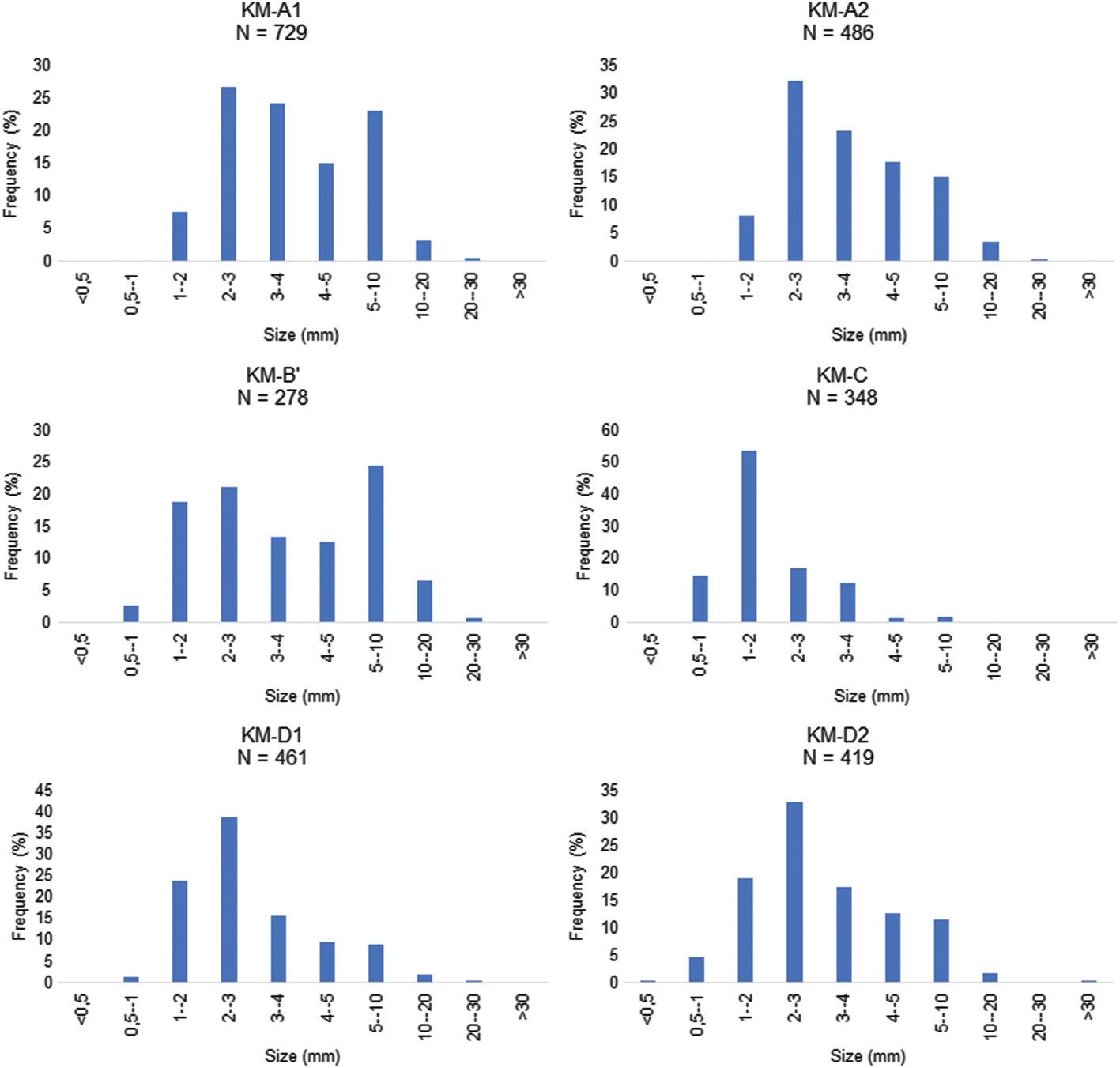
Fig. 16. Size-frequency distributions of unidentified vertebrate material for each locus of the Ksar Metlili site (size in millimetres). Only unidentified elements are used here, in order to avoid the taxonomic bias due to variation in taxonomic composition in the different loci (see Section 6.b).
These patterns of size-frequency distribution show that there is no sorting of the fossils due to the element size, i.e. there is no major granulometric bias in the representation of the microfauna between the different sampled loci. However, it appears that the most delicate taxa (lissamphibians, squamates, pterosaurs and mammals) are preserved in greater quantities in A2 and D2 (Table 3), suggesting a low-energy depositional environment. This observation is consistent with the fine and well-sorted granulometry of the associated sedimentary particles (lithoclasts) from the respective localities. The sedimentary facies and environment of all loci, corresponding to a local fluvial channel deposit, is actually similar and the preservational differences only reflect local hydrodynamic differences in the same channel: the bioclastic elements are not concentrated and sorted in the same way at the bottom or at the edge of the channel.
6.c. New data on the dating of the Ksar Metlili site
The 2010 expedition also resulted in the discovery of the new microvertebrate fossil locality of Guelb el Ahmar (GEA) in the Anoual Formation, which is stratigraphically right below the Ksar Metlili Formation, and where about 1.8 tons of sediments were sampled (Haddoumi et al. Reference Haddoumi, Allain, Meslouh, Métais, Monbaron, Pons, Rage, Vullo, Zouhri and Gheerbrant2016). The GEA fossil locality is Bathonian (Middle Jurassic) in age, and it is one of the richest continental vertebrate assemblages from the Middle Jurassic of Gondwana (Haddoumi et al. Reference Haddoumi, Allain, Meslouh, Métais, Monbaron, Pons, Rage, Vullo, Zouhri and Gheerbrant2016). The GEA and KM fossil localities from the Anoual Syncline are reference faunas documenting the evolution of vertebrates at or close to the Jurassic–Cretaceous transition, which remains noticeably poorly known in Africa and Gondwana.
The GEA fossil locality is less than 5 km northeast of the KM site, and it essentially consists of lacustrine deposits. The brachiopod fauna (Burmirhynchia gr. termeriae-aethiensis and Kallirhynchia oranensis) and, to a lesser extent, the ostracods and charophytes found in the marine upper member of the Anoual Formation strictly constrain the minimum age of the GEA fossil locality as early Bathonian (Haddoumi et al. Reference Haddoumi, Alméras, Bodergat, Charrière, Mangold and Benshili1998, Reference Haddoumi, Allain, Meslouh, Métais, Monbaron, Pons, Rage, Vullo, Zouhri and Gheerbrant2016). The GEA faunal assemblage is taxonomically close to that of the KM fauna, except for some taxa that are missing in the GEA fauna (Table 4). This can be explained in different ways, but an important point is the difference in faunal richness between these two sites, KM being far richer than GEA despite a similar volume of sampled sediments (1.5 and 1.8 tons, respectively). Terrestrial vertebrates from the GEA fauna are less diversified and less abundant than aquatic ones, which is consistent with the lacustrine sedimentary facies of the GEA fossiliferous levels (Haddoumi et al. Reference Haddoumi, Charrière, Andreu and Mojon2008). Moreover, the low-energy depositional (lacustrine) environment of the GEA locality did not concentrate fossil remains as much as in the KM locality, which explains the lower abundance (per square metre) and diversity of fossils in the GEA assemblage.
Table 4. Faunal list of the Guelb el Ahmar site (Bathonian, Middle Jurassic), compared with the Ksar Metlili microvertebrate fauna

* referred to as ‘Lissamphibia incertae sedis’ in Haddoumi et al. (Reference Haddoumi, Allain, Meslouh, Métais, Monbaron, Pons, Rage, Vullo, Zouhri and Gheerbrant2016). The taxa in grey are shared by the GEA and KM faunas.
By contrast, some taxa are present in the GEA fauna but not in the KM fauna, for example the ionoscopiforms, metriorhynchids and stegosaurs (Table 4). Ionoscopiforms and metriorhynchids, two strictly aquatic taxa (Bardet, Reference Bardet1995; Brandalise de Andrade et al. Reference Brandalise de Andrade, Young, Desojo and Brusatte2010; Xu et al. Reference Xu, Zao and Coates2014), confirm that the environment at the GEA site was more aquatic than at the KM site.
The KM and GEA faunas share numerous taxa (14 common taxa, Table 4), despite their noticeably distinct ages following current stratigraphic data and interpretation. The strong resemblance of these faunas seems to stand against the existence of a supposed major stratigraphic gap (~23 Ma) between the Bathonian Anoual Formation and the Ksar Metlili Formation, thus questioning the inferred Berriasian age of the KM site (Sigogneau-Russell et al. Reference Sigogneau-Russell, Monbaron and de Kaenel1990, Reference Sigogneau-Russell, Evans, Levine, Russell, Lucas, Kirkland and Estep1998; Duffin & Sigogneau-Russell, Reference Duffin and Sigogneau-Russell1993; Haddoumi et al. Reference Haddoumi, Charrière, Andreu and Mojon2008, Reference Haddoumi, Allain, Meslouh, Métais, Monbaron, Pons, Rage, Vullo, Zouhri and Gheerbrant2016; Mojon et al. Reference Mojon, Haddoumi and Charrière2009). However, we cannot definitely exclude an Early Cretaceous age for the KM fauna for several reasons. The first and the most important one is that the recognized similarities between the KM fauna and the studied Jurassic faunas (GEA and Guimarota) are at higher systematic levels, owing to the numerous uncertainties in the determinations as a consequence of the poor state of preservation of the fossil material, which results in low taxonomic resolution. Another explanation is that some of the shared taxa with the GEA fauna could be long-lived taxa spanning the Middle Jurassic and Early Cretaceous periods, or that the African KM localities could be a refugium for vertebrates with Jurassic affinities, as are sites in Western Siberia (Ilek Formation) and Yakutia (Batylykh Formation) (Skutschas, Reference Skutschas2016; Averianov et al. Reference Averianov, Martin, Lopatin, Skutschas, Schellhorn, Kolosov and Vitenko2018; Skutschas et al. Reference Skutschas, Kolchanov, Averianov, Martin, Schellhorn, Kolosov and Vitenko2018). However, the close taxonomic resemblance of the GEA and KM faunas indicates that an age as old as Late Jurassic for the KM fauna cannot be excluded in the current state of understanding of their taxonomic composition.
The KM site is not the first vertebrate-yielding locality from the ‘Continental Intercalaire’ for which a Cretaceous or Jurassic age remains debated. In Morocco, the dating of the ‘Red Beds’ has always been debated because of the absence of reliable chronostratigraphic markers (see Section 2 above and Charrière & Haddoumi, Reference Charrière and Haddoumi2016, Reference Charrière and Haddoumi2017 for more details). The Tiouaren Formation in Niger was assumed to be Lower Cretaceous by many authors (Lapparent, Reference Lapparent1960; Taquet, Reference Taquet1976; Moody & Sutcliffe, Reference Moody and Sutcliffe1991; Mateer et al. Reference Mateer, Wycisk, Jacobs, Brunet, Luger, Arush, Hendriks, Weissbrod, Gvirtzman, Mbede, Dina, Moody, Weigelt, El-Nakhal, Hell and Stets1992; Sereno et al. Reference Sereno, Wilson, Larsson, Dutheil and Sues1994, Reference Sereno, Beck, Dutheil, Larsson, Lyon, Moussa, Sadleir, Sidor, Varrichio, Wilson and Wilson1999, Reference Sereno, Wilson and Conrad2004), but even if the stratigraphic relationships of the Tiouaren Formation with the over- and underlying formations have helped to constrain its minimum age as Aptian, they do not provide a maximum age for that formation (Rauhut & López-Arbarello, Reference Rauhut and López-Arbarello2009). Some dinosaur taxa from the Tiouaren Formation would not be inconsistent with a pre-Kimmeridgian Jurassic age (Rauhut & López-Arbarello, Reference Rauhut and López-Arbarello2009). In fact, it is likely that the Tiouaren Formation and the Moroccan ‘Red Beds’, both spanning a large time interval, contain faunas of different ages and thus the ages inferred from stratigraphic and faunal data cannot be attributed to the entire formation.
A better characterization of the age of the KM fauna requires some large-scale comparisons with subcontemporaneous faunas, such as those from the Bathonian of Kirtlington, Britain, the Upper Jurassic Morrison Formation, North America, or the Lower Cretaceous Purbeck Limestone Group of Britain. Such comparisons have never been done and are currently still in progress, but we provide here a preliminary comparison with the Guimarota site (Portugal) that has been dated as Kimmeridgian (Upper Jurassic) on the basis of the ostracod fauna (Schudack, Reference Schudack, Martin and Krebs2000). This site has yielded a rich and diversified microvertebrate fauna (Zinke, Reference Zinke1998; Martin & Krebs, Reference Martin and Krebs2000; Martin, Reference Martin2001). The faunal assemblage from Guimarota shows affinity with that of the KM fauna at higher taxonomic levels. The two faunas share hybodont selachians (Kriwet, Reference Kriwet, Martin and Krebs2000, Reference Kriwet2004), pycnodontiform actinopterygians (Kriwet, Reference Kriwet, Martin and Krebs2000, Reference Kriwet2002), albanerpetontids (Wiechmann, Reference Wiechmann, Martin and Krebs2000; Gardner et al. Reference Gardner, Evans and Sigogneau-Russell2003), atoposaurids (Schwarz & Salisbury, Reference Schwarz and Salisbury2005), teleosaurids (Schwarz, Reference Schwarz2002), ornithischian dinosaurs (Zinke, Reference Zinke1998; Rauhut, 2000, Reference Rauhut2001), dromaeosaurid theropods (Zinke, Reference Zinke1998; Rauhut, Reference Rauhut, Martin and Krebs2000) and dryolestid and zatherian mammals (Martin, Reference Martin1999, Reference Martin, Martin and Krebs2000, Reference Martin2001, Reference Martin2002). Considering all these taxa together, this faunal association is consistent with a possible Late Jurassic age for the KM fauna, although the high taxonomic-rank affinity with Guimarota does not permit a definitive conclusion.
The faunal assemblage from the Langenberg Quarry, in the upper Oxfordian to upper Kimmeridgian (Fischer, Reference Fischer1991) of northern Germany, also shows numerous affinities with the KM fauna. Hybodont selachians (Thies, Reference Thies1995; A. Mudroch, unpub. Ph.D. thesis, Univ. Hannover, 2001), ginglymode, pycnodontiform and ionoscopiform actinopterygians (A. Mudroch, unpub. Ph.D. thesis, Univ. Hannover, 2001), paramacellodid lizards (Wings, Reference Wings, Zhang, Wu and Sun2015; Richter et al. Reference Richter, Knötschke, Kosma, Sobral and Wings2013; Schwarz et al. Reference Schwarz, Raddatz and Wings2017), teleosaurid and atoposaurid crocodylomorphs (Karl et al. Reference Karl, Gröning, Brauckmann, Schwarz and Knötschke2006; Wings & Sander, Reference Wings and Sander2012; Wings, Reference Wings, Zhang, Wu and Sun2015; Schwarz et al. Reference Schwarz, Raddatz and Wings2017), dromaeosaurid theropods (Lubbe et al. Reference Lubbe, Richter and Knötschke2009) and dryolestid mammals (Wings, Reference Wings, Zhang, Wu and Sun2015) are shared between the two faunas. Like the previous comparison with the Guimarota mine, this comparison with the Langenberg Quarry fauna is a new consistent element to propose a possible Late Jurassic age for the KM fauna even if, again, these higher taxonomic-level affinities do not permit a definitive conclusion.
There seems to be some similarity in faunal composition between the KM site and the Lower–Middle Jurassic Kota Formation of India: teeth similar to those referred to the Atoposauridae and Dromaeosauridae are also known from the Kota Formation (G. V. R. Prasad, work in progress). The Kota Formation also shares the triconodont mammal Dyskritodon (D. indicus) with the KM site, and further haramiyid teeth have been recovered from this formation (G. V. R. Prasad, work in progress). Thus, the Kota Formation vertebrates may represent some of the few Gondwanan affinities of the KM fauna (see Section 6.d.1 below) and, because of its Lower–Middle Jurassic age (Prasad & Manhas, Reference Prasad and Manhas2002), it could be another argument to propose a possible Late Jurassic age for the KM fauna.
Besides faunal comparisons with the GEA and Guimarota faunas, other elements of discussion may argue for a Late Jurassic age for the KM fauna instead of Early Cretaceous. The ornithischian tooth described here (see Section 4.b.10.a above) has quite a simple morphology, compared to other Jurassic and Cretaceous ornithischians, such as Lycorhinus, Abrictosaurus, Heterodontosaurus, Nanosaurus or Hypsilophodon (Norman et al. Reference Norman, Sues, Witmer, Coria, Weishampel, Dodson and Osmólska2004; Carpenter & Galton, Reference Carpenter and Galton2018). Because of its plesiomorphic morphology, it seems closely related to the early representatives of the group or could be a survivor of an ancient, basal lineage. Thus, this ornithischian tooth could argue in favour of a Jurassic age for the KM fauna. Another argument is the possible presence in the KM fauna of several relict lineages. This includes first the youngest known putative non-mammaliaform cynodonts outside Asia, with teeth that are morphologically close to those of Late Permian – Early Triassic species (see Section 4.b.11 above and references therein). It is more reasonable to hypothesize the survival of such an African relict lineage until the Late Jurassic rather than the Early Cretaceous. The same can be said about the presence of ?Gnathosaurinae pterosaurs, of which the KM species are among the latest representatives (see Section 4.b.9 above and references therein), and of haramiyidan mammaliaforms (see Section 4.b.12 above), which are scarce outside Eurasia (Huttenlocker et al. Reference Huttenlocker, Grossnickle, Kirkland, Schultz and Luo2018).
The palaeobiogeography also gives clues concerning a possible reassessment of the age of the KM fauna to the Jurassic. There are many taxa with Pangaean affinities and only a few with Gondwanan affinities in the KM fauna, despite its location in Gondwana (see Section 6.d below). The most parsimonious explanation of such a strong Pangaean affinity of the fauna is that it remained close in time to the vicariant event resulting from the Jurassic Pangaean fragmentation. If so, then the KM fauna could be older than previously thought, i.e. of Jurassic age, even if we still cannot exclude the Cretaceous hypothesis.
6.d. Palaeobiogeographical implications
6.d.1. Taxa with Gondwanan affinities
During the Jurassic–Cretaceous transition, Africa was still sutured with South America in the West Gondwanan block, but the incipient opening of the Western Tethys and Central Atlantic Ocean drifted it away from Laurasia (Cecca et al. Reference Cecca, Azema, Fourcade, Baudin, Guiraud, Dever, Dercourt, Ricou and Vrielynck1993; Gheerbrant & Rage, Reference Gheerbrant and Rage2006). Terrestrial faunal relationships between South America and Africa were possible until Aptian/Albian time. However, and unexpectedly, few taxa of Gondwanan affinities are currently identified in the KM fauna. They are only represented by osteoglossiform actinopterygians, cf. ptychoceratodontid lungfishes and ctenochasmatid pterosaurs. Other taxa, such as atoposaurids, dromaeosaurids and the mammal genus Dyskritodon (G. V. R. Prasad, work in progress), may represent Gondwanan affinities of the KM fauna, but we cannot exclude a trans-Tethyan dispersal from a Laurasian origin or an old Pangaean distribution for those taxa (see Sections 6.d.2 and 6.d.3 below). More generally, the known faunal relationships and dispersals between Africa and other Gondwanan continents are paradoxically scarce during the entire Cretaceous period (Gheerbrant & Rage, Reference Gheerbrant and Rage2006). There is no taxon of Gondwanan affinity in the GEA fauna, with the possible exception of the dipnoan Arganodus, which seems to be present in Gondwana between the Triassic and Cretaceous periods (Soto & Perea, Reference Soto and Perea2010; Haddoumi et al. Reference Haddoumi, Allain, Meslouh, Métais, Monbaron, Pons, Rage, Vullo, Zouhri and Gheerbrant2016).
6.d.2. Taxa with Laurasian affinities: evidence for trans-Tethyan dispersals
In contrast to the occurrence of few Gondwanan taxa, the KM fauna includes numerous vertebrate taxa with Laurasian affinities that suggests either vicariance or dispersal events between Laurasia and Africa during Late Jurassic or Early Cretaceous times. An old Pangaean distribution cannot be excluded for some of these taxa based on the current state of the data presented here, but some other taxa clearly favour dispersal events.
The albanerpetontid and alytid anurans, previously unknown in Gondwana during Jurassic and Early Cretaceous times, are thought to be of Laurasian origin (Gheerbrant & Rage, Reference Gheerbrant and Rage2006). Their presence in the KM fauna, at the time of Pangaean fragmentation, results either from a vicariance event or more likely from a N–S trans-Tethyan dispersal event (Gheerbrant & Rage, Reference Gheerbrant and Rage2006). The hypothesis of a trans-Tethyan dispersal of the albanerpetontid and alytid anurans requires the existence of a terrestrial route across the Tethys, because the sea is an effective barrier for the dispersal of amphibians. It could have been provided by the Mediterranean Tethyan Sill during low eustatic levels (Vrielynck et al. Reference Vrielynck, Dercourt, Cottereau, Naim, Ricou, Vrielynck and Dercourt1995; Dercourt et al. Reference Dercourt, Gaetani, Vrielynck, Barrier, Biju-Duval, Brunet, Cadet, Crasquin and Sandulescu2000). Paramacellodid squamates, such as Paramacellodus marocensis from the KM fauna, are well known in Laurasia but have only two occurrences in Gondwana (Tendaguru, in the Upper Jurassic of Tanzania, and KM; Broschinski & Sigogneau-Russell, Reference Broschinski and Sigogneau-Russell1996; Broschinski, Reference Broschinski1999); so, the hypothesis of a trans-Tethyan dispersal from Laurasia towards Africa is plausible, although we cannot exclude a possible old Pangaean distribution. Choristoderan reptiles and atoposaurid crocodylomorphs are exclusively known in Laurasia during this period (Matsumoto et al. Reference Matsumoto, Buffetaut, Escuillié, Hervet and Evans2013; Tennant & Mannion, Reference Tennant and Mannion2014; Tennant et al. Reference Tennant, Mannion and Upchurch2016), but have Gondwanan occurrences as early as Bathonian time (from Morocco and Madagascar; Flynn et al. Reference Flynn, Fox, Parrish, Ranivoharimanana, Wyss, Harris, Lucas, Spielmann, Lockley, Milner and Kirkland2006; Haddoumi et al. Reference Haddoumi, Allain, Meslouh, Métais, Monbaron, Pons, Rage, Vullo, Zouhri and Gheerbrant2016); thus, a trans-Tethyan dispersal of these two groups is possible, although, and again, we cannot definitely exclude the Pangaean vicariance hypothesis.
Several mammals found in the KM fauna are also most likely of Laurasian origin. For example, the triconodont Gobiconodon and the ‘symmetrodont’ Thereuodon are known from the Jurassic and the Lower Cretaceous of Laurasia (Sigogneau-Russell & Ensom, Reference Sigogneau-Russell and Ensom1998; Sigogneau-Russell, Reference Sigogneau-Russell2003; Kielan-Jaworowska et al. Reference Kielan-Jaworowska, Cifelli and Luo2004; Gheerbrant & Rage, Reference Gheerbrant and Rage2006; J. Pouech, unpub. Ph.D. thesis, Claude Bernard – Lyon 1 University, 2008; Butler & Sigogneau-Russell, Reference Butler and Sigogneau-Russell2016). The gobiconodontid (eutriconodont) Gobiconodon palaios from the KM fauna seems to be close to G. bathoniensis from the Middle Jurassic of Great Britain (Butler & Sigogneau-Russell, Reference Butler and Sigogneau-Russell2016), and Thereuodon dahmani from the KM fauna is close to T. taraktes from the Lower Cretaceous of Britain (Sigogneau-Russell & Ensom, Reference Sigogneau-Russell and Ensom1998), which stands in favour of a trans-Tethyan dispersal of these genera. Likewise, dryolestoids are known only in Laurasia before the Late Cretaceous period, which indicates a Laurasian origin for the group (Kielan-Jaworowska et al. Reference Kielan-Jaworowska, Cifelli and Luo2004). Their presence in the KM fauna can be indeed explained by a trans-Tethyan dispersal event (but see Section 6.d.3 below). The KM fauna also includes the stem boreosphenidans Hypomylos and Tribotherium, which are the oldest known tribosphenidans (= Boreosphenida; Luo et al. Reference Luo, Cifelli and Kielan-Jaworowska2001) in Africa. On a global scale, they are even among the first representatives of the tribosphenidans (Sigogneau-Russell, Reference Sigogneau-Russell1991c; Kielan-Jaworowska et al. Reference Kielan-Jaworowska, Cifelli and Luo2004). The Laurasian diversity and the phylogenetic relationships of the Tribosphenida suggest a Laurasian origin for this group (Luo et al. Reference Luo, Cifelli and Kielan-Jaworowska2001, Reference Luo, Kielan-Jaworowska and Cifelli2002, Reference Luo, Ji, Wible and Yuan2003). The two stem boreosphenidan species from the KM site further support the hypothesis of one or more dispersal events between Laurasia and Africa by the beginning of the Cretaceous period and possibly well back into Late Jurassic time. The KM fauna provides additional data showing that trans-Tethyan biotic interchanges between Laurasia and Africa were not minor and accidental events (Gheerbrant & Rage, Reference Gheerbrant and Rage2006) and may have occurred as early as the Jurassic period.
As for the KM fauna, previous studies (Haddoumi et al. Reference Haddoumi, Allain, Meslouh, Métais, Monbaron, Pons, Rage, Vullo, Zouhri and Gheerbrant2016) have shown that the GEA fauna displays noticeable affinities with Laurasian faunas, with the presence of albanerpetontids, caudatan amphibians, anguimorph squamates, choristoderes, amphitheriids, dryolestoids and stem boreosphenidans (Table 4; Haddoumi et al. Reference Haddoumi, Allain, Meslouh, Métais, Monbaron, Pons, Rage, Vullo, Zouhri and Gheerbrant2016). Moreover, the atoposaurid teeth from the GEA and KM faunas represent some of the scarce occurrences of this group in Gondwana (Haddoumi et al. Reference Haddoumi, Allain, Meslouh, Métais, Monbaron, Pons, Rage, Vullo, Zouhri and Gheerbrant2016); atoposaurids are instead well known in Laurasia. However, because of their presence in both the KM and GEA faunas, we cannot exclude an old cosmopolitan distribution for all or part of these taxa, which thus could be vicariant Pangaean taxa.
6.d.3. Pangaean vicariant taxa
Several taxa from the KM fauna, including mammals, suggest a likely old inherited Pangaean distribution. This includes the hahnodontids that were initially described as belonging to an endemic African multituberculate family during Early Cretaceous time (Sigogneau-Russell, Reference Sigogneau-Russell1991b; Hahn & Hahn, Reference Hahn and Hahn2003). Their recent discovery in the Lower Cretaceous of North America led Huttenlocker et al. (Reference Huttenlocker, Grossnickle, Kirkland, Schultz and Luo2018) to the inclusion of the family in the Haramiyida, and to explaining their presence in both North America and Africa by the existence of Pangaea-wide dispersal corridors, accessible to relictual clades of vertebrates such as haramiyidans. However, an old Pangaean distribution seems more likely because of the already reported presence of haramiyidans in the African Jurassic (Tanzania; Heinrich, Reference Heinrich1999, Reference Heinrich2001, Reference Heinrich2004) and the Indian Jurassic and Cretaceous (Anantharam et al. Reference Anantharam, Wilson, Das Sarma and Clemens2006; G. V. R. Prasad, work in progress). The dryolestoids are known to occur in the Upper Jurassic and Lower Cretaceous of both Europe and North America and in the Upper Cretaceous of South America (Kielan-Jaworowska et al. Reference Kielan-Jaworowska, Cifelli and Luo2004; Cifelli et al. Reference Cifelli, Davis and Sames2014), which cannot exclude their wider early distribution in both Gondwana and Laurasia. Triconodontids are known from as early as the Jurassic of South America, with Argentoconodon fariasorum (Gaetano & Rougier, Reference Gaetano and Rougier2010, Reference Gaetano and Rougier2011); this species shares four unambiguous synapomorphies with Ichthyoconodon jaworowskorum from the KM fauna and with Volaticotherium antiquus from the Middle Jurassic of China (see Gaetano & Rougier, Reference Gaetano and Rougier2007, Reference Gaetano and Rougier2010, Reference Gaetano and Rougier2011). These three species thus represent a monophyletic group of triconodontids, the tribe Volaticotherini, nested within the Alticonodontinae (Gaetano & Rougier, Reference Gaetano and Rougier2011). Considering the Early Jurassic age of A. fariasorum, ghost lineages for Ichthyoconodon and Volaticotherium must extend back at least to Early Jurassic time. Ichthyoconodon might have arisen from vicariant evolution in Africa following Pangaean fragmentation (Gheerbrant & Rage, Reference Gheerbrant and Rage2006). The genus Dyskritodon might also have had an old Pangaean distribution, because of the presence of the species D. indicus in the Lower or Middle Jurassic of India (Prasad & Manhas, Reference Prasad and Manhas2002). The following other non-mammalian taxa from the KM fauna also have Pangaean affinities: the ginglymodians of cosmopolitan distribution; the ionoscopiform actinopterygians, widely distributed in Europe, Africa and the Americas from the Middle Triassic to the end of the Cretaceous period (Vullo & Courville, Reference Vullo and Courville2014); the mawsoniid actinistians of Pangaean Triassic distribution (Miguel et al. Reference Miguel, Gallo and Morrone2014); the rhynchocephalians, known from the Triassic in Europe, Madagascar and the Americas, and from the Lower Jurassic in Asia, Africa and India (Evans et al. Reference Evans, Prasad and Manhas2001; Martínez et al. Reference Martínez, Apaldetti, Colombi, Praderio, Fernandez, Malnis, Correa, Abelin and Alcober2013); the scincomorphans, present in North America, Europe and Africa since Late Jurassic time (Evans, Reference Evans2003) and in Asia since Middle Jurassic time (Averianov et al. Reference Averianov, Martin, Skutschas, Danilov, Schultz, Schellhorn, Obraztsova, Lopatin, Sytchevskaya, Kuzmin, Krasnolutskii and Ivantsov2016); and the teleosaurid crocodylomorphs that have a cosmopolitan distribution in Middle Jurassic time (Haddoumi et al. Reference Haddoumi, Allain, Meslouh, Métais, Monbaron, Pons, Rage, Vullo, Zouhri and Gheerbrant2016). Apart from triconodontid mammals, all these taxa are also present in the GEA fauna (Table 4; Haddoumi et al. Reference Haddoumi, Allain, Meslouh, Métais, Monbaron, Pons, Rage, Vullo, Zouhri and Gheerbrant2016), which is significant.
6.d.4. African endemism
Some of the KM taxa seems to be endemic to Africa or even to North Africa, despite its connection with South America and thus with other Gondwanan landmasses. Some taxa, such as stem zatherian and boreosphenids, are present in the KM fauna but unknown in South America (Kielan-Jaworowska et al. Reference Kielan-Jaworowska, Cifelli and Luo2004). Australosphenids are known in the whole of Gondwana during that time, except in Africa (Kielan-Jaworowska et al. Reference Kielan-Jaworowska, Cifelli and Luo2004). The hypothesis of a North African provincialism, with a geographic and/or biological isolation of North Africa within Africa and Gondwana before the separation of Africa and South America, has been suggested in previous studies (Gheerbrant & Rage, Reference Gheerbrant and Rage2006; Haddoumi et al. Reference Haddoumi, Allain, Meslouh, Métais, Monbaron, Pons, Rage, Vullo, Zouhri and Gheerbrant2016): it could explain the presence of some taxa in North Africa but not in South America, and the absence of others from North Africa despite their presence in South America. No significant endemism has been detected in the GEA vertebrate fauna, but this fauna is still poorly documented, so this is not a definitive statement (Haddoumi et al. Reference Haddoumi, Allain, Meslouh, Métais, Monbaron, Pons, Rage, Vullo, Zouhri and Gheerbrant2016).
6.d.5. Summary of the palaeobiogeographical significance of the KM fauna
The current study of the KM fauna shows that it has, as a whole, a complex palaeobiogeographical composition. This North African fauna includes a predominant number of vicariant Pangaean taxa. It also includes several Laurasian immigrants implying trans-Tethyan dispersals. The involved dispersal events might be as early as Middle Jurassic for the taxa that were already present in the GEA fauna (albanerpetontids, choristoderes, atoposaurids and dryolestoids mammals), although we cannot exclude that the Jurassic taxa known in the GEA fauna could alternatively have an old Pangaean distribution. The Gondwanan taxa are by contrast few in the KM fauna, as was already noted in the GEA fauna (Haddoumi et al. Reference Haddoumi, Allain, Meslouh, Métais, Monbaron, Pons, Rage, Vullo, Zouhri and Gheerbrant2016). Some endemic taxa also suggest evolution in the context of a ?Jurassic North African provincialism.
7. Conclusions
The KM and GEA fossil localities from the Anoual Syncline provide critical fossil data on the palaeobiodiversity and evolution of vertebrate faunas from the Middle–Upper Jurassic/Lower Cretaceous of Africa and Gondwana, which remain poorly known. Their unrivalled richness and diversity open an exceptional window on the Mesozoic palaeoecosystems of Africa. Considering both the old and new collections from the KM locality, at least 47 distinct species are identified. Some of them, such as mammals, are highly diversified in the KM fauna. The Crocodylomorpha is the dominant taxon, which indicates a more terrestrial environment at the KM than at the GEA site. A predominating four-taxa association is identified: actinopterygians, crocodylomorphs, pterosaurs and turtles, representing more than 95 % in relative abundance of the recovered fossil material. The KM fossil locality has produced some remarkable taxa including the oldest possible ?Siluriformes or ?Polypteriformes, the earliest Gondwanan record of caecilians and alytids, and one of the scarce Gondwanan records of albanerpetontids (with the albanerpetontid genus Anoualerpeton also recorded from the Middle Jurassic of England) and of choristoderes. Ksar Metlili also yielded one of the first occurrences of freshwater teleosaurids and an ornithischian with surprisingly primitive dental features relative to other contemporaneous ornithischians. Finally, the KM locality preserves some of the last-known non-mammalian cynodonts outside Asia and some of the first-known stem boreosphenidan mammals, which are also the earliest known from Africa. Moreover, the KM fauna has a complex composite palaeobiogeographic history: it is mainly composed of taxa with Pangaean and Laurasian affinities, whereas only a few Gondwanan taxa have been found. This assemblage seems to be the result of Pangaean fragmentation and vicariance and of several trans-Tethyan dispersal events in Africa. The taxonomic comparisons between the GEA, Guimarota and KM faunas provide new data questioning the previously proposed earliest Cretaceous age for the KM fauna. The taxonomic similarities between these three key faunas seem to stand against the existence of an important time gap between the microvertebrate sites from the Anoual and Ksar Metlili formations. This leads us to conclude that a Late Jurassic age for the KM fauna, instead of Early Cretaceous, still cannot be rejected.
The study of the KM fauna is still in progress. Turtles, crocodiles, non-mammalian cynodonts and several mammal taxa have to be further studied, and the taxonomy of lissamphibians and theropods must be revised. More fossil remains from the KM locality, currently in preparation, might provide important data on the palaeobiodiversity, evolutionary and palaeobiogeographical history of African and Gondwanan vertebrates during the Jurassic period.
Acknowledgements
This paper is dedicated to the memory of our friend and co-author Jean-Claude Rage, who suddenly passed away on March 30, 2018. We thank S. Jouve (Sorbonne Université) and J. P. Tennant (Imperial College London) for discussions on crocodylomorphs and especially on the teleosaurids and atoposaurids. We thank G. Clément for his help with the identification of the coelacanth. Thanks also to J. E. Martin for discussions on the ornithischian tooth. We thank M. Debuysschere (CR2P, MNHN), F. Abdala (University of the Witwatersrand) and S. C. Sweetman (University of Portsmouth) for their help and discussions on non-mammaliaform cynodonts. We thank L. Cazes and P. Loubry (CR2P, MNHN) for the photographs. We are grateful to A. Gailliègue (ISCD, Sorbonne Université) for the script ‘Count Image Elements’ that she specially developed for this study and which has been very helpful for the counting of the numerous specimens. We also want to thank M. Forissier, J. Marchand and V. Prugneaux (SAGA) for their assistance in the long and difficult sorting of the clastic samples. We are grateful to A. Benlakhdim (Ministry of Energy, Mines and Sustainable Development, Morocco) for the field work authorization. We thank P. Skutschas, three anonymous reviewers and the editor, for their corrections and useful comments which helped to improve the manuscript. The 2010 field work in Anoual area (Morocco) that allowed the recovery of the KM and GEA material was supported by the National Geographic Society (CRE GRANT #8706-09; PI: E. Gheerbrant).
Supplementary Material
To view supplementary material for this article, please visit https://doi.org/10.1017/S0016756819000761.