INTRODUCTION
Parasitic nematodes of the group Cyathostominae are a potential cause of serious disease in equids. These parasites have a high prevalence and most horses that graze encounter them (Matthews, Reference Matthews2008). Cyathostomins exist as a group of around 50 species (Lichtenfels et al. Reference Lichtenfels, Kharchenko and Dvojnos2008); however, most infections comprise 5–10 common species, with low numbers of rarer species present (Chapman et al. Reference Chapman, French and Klei2002a). Species compositions are similar across regions, with the same group appearing as the most prevalent globally (Ogbourne, Reference Ogbourne1976; Reinemeyer et al. Reference Reinemeyer, Smith, Gabel and Herd1984; Mfitilodze and Hutchinson, Reference Mfitilodze and Hutchinson1990; Bucknell et al. Reference Bucknell, Gasser and Beveridge1995; Gawor, Reference Gawor1995; Lichtenfels et al. Reference Lichtenfels, McDonnell, Love and Matthews2001; Collobert-Laugier et al. Reference Collobert-Laugier, Hoste, Sevin and Dorchies2002). For all species, the life cycle involves larval encystment in the wall of the large intestine and larvae can persist for up to 2 years (Gibson, Reference Gibson1953; Smith, Reference Smith1976; Murphy and Love, Reference Murphy and Love1997). In some horses, encysted larvae can constitute up to 90% of the total burden, with some individuals harbouring millions of worms (Dowdall et al. Reference Dowdall, Matthews, Mair, Murphy, Love and Proudman2002). Encysted larvae are important in the pathogenesis of cyathostomin infections, because these stages can emerge in great numbers to cause larval cyathostominosis, a colitis syndrome that can be fatal in up to 50% of cases (Giles et al. Reference Giles, Urquhart and Longstaffe1985; Love et al. Reference Love, Mair and Hillyer1992). Larval cyathostominosis is most commonly observed in horses of 2–5 years of age (Reid et al. Reference Reid, Mair, Hillyer and Love1995); however, animals can have a lifelong susceptibility to infection and disease has been observed in horses of all ages (Mair, Reference Mair1993). The potential clinical effects of cyathostomins are confounded by high levels of anthelmintic resistance in these nematodes, with resistance to benzimidazole and pyrantel compounds almost ubiquitous in some regions (Kaplan, Reference Kaplan2002; Matthews, Reference Matthews2014). The macrocyclic lactones are the most commonly used anthelmintics in horses and reduced efficacy against cyathostomins has been reported for ivermectin in Brazil (Canever et al. Reference Canever, Braga, Boeckh, Grycajuck, Bier and Molento2013), with several studies reporting shortened strongyle egg reappearances period following ivermectin (Geurden et al. Reference Geurden, van Doorn, Claerebout, Kooyman, De Keersmaecker, Vercruysse, Besognet, Vanimisetti, di Regalbono, Beraldo, Di Cesare and Traversa2014; Relf et al. Reference Relf, Lester, Morgan, Hodgkinson and Matthews2014) and moxidectin (Rossano et al. Reference Rossano, Smith and Lyons2010; Relf et al. Reference Relf, Lester, Morgan, Hodgkinson and Matthews2014) treatment. A shortened helminth egg reappearance period is regarded as an early indicator of resistance (Sangster, Reference Sangster1999), highlighting the threat of resistance to all available classes of broad spectrum anthelmintic.
Anthelmintic targeting of encysted larvae is now common practice in control programmes in some regions (Stratford et al. Reference Stratford, Lester, Morgan, Pickles, Relf, McGorum and Matthews2014). Moxidectin and fenbendazole (administered over 5 consecutive days) are registered for this purpose (Matthews, Reference Matthews2008); however, because of high levels of resistance to fenbendazole (Reinemeyer et al. Reference Reinemeyer, Prado and Nielsen2016), moxidectin is the only remaining compound effective against encysted larvae, so its efficacy needs to be preserved. To address this, a reduction in treatment frequency is recommended (Matthews, Reference Matthews2014). This can be achieved by improving grazing practices to reduce transmission via the environment, combined with specific targeting of treatments based on strongyle egg shedding (Nielsen et al. Reference Nielsen, Haaning and Olsen2006, Reference Nielsen, Pfister and von Samson-Himmelstjerna2014; Lester and Matthews, Reference Lester and Matthews2014). Such protocols do not address encysted larvae within individuals. Indeed, horses with sizeable encysted larval burdens often have no or low egg shedding (Dowdall et al. Reference Dowdall, Matthews, Mair, Murphy, Love and Proudman2002). Because of these issues, a test that informs on the presence or burden of encysted larvae would facilitate anthelmintic targeting of these stages and would assist in the definitive diagnosis of larval cyathostominosis, a challenge in practice due to the non-specific nature of the associated clinical signs (Giles et al. Reference Giles, Urquhart and Longstaffe1985).
Previously, these authors identified two native antigen complexes that showed promise as diagnostic markers of encysted larval infection (Dowdall et al. Reference Dowdall, Matthews, Mair, Murphy, Love and Proudman2002, Reference Dowdall, Proudman, Love, Murphy, Klei and Matthews2003, Reference Dowdall, Proudman, Klei, Mair and Matthews2004). The antigen preparations are labour intensive to prepare and rely on a continual source of equine tissue. Thus, steps were taken to identify genes that encode protein components of the complexes to develop a recombinant protein-based test. One component, cyathostomin gut associated larval antigen-1 (Cy-GALA-1) protein, was identified by immunoscreening a larval complementary (c)DNA library using sera from infected ponies (McWilliam et al. Reference McWilliam, Nisbet, Dowdall, Hodgkinson and Matthews2010). Sequence analysis of polymerase chain reaction (PCR) products amplified from DNA from individual worms indicated that Cy-GALA-1 was derived from the common species, Cyathostomum pateratum, so Cy-GALA-1 was re-designated Cy-GALA-pat (McWilliam et al. Reference McWilliam, Nisbet, Dowdall, Hodgkinson and Matthews2010). Recombinant Cy-GALA-pat was demonstrated to be the target of serum immunoglobulin G(T) (IgG(T)) responses in infected, but not in uninfected horses, and exhibited no reactivity to antibody in serum from horses specifically infected with non-cyathostomin helminths. In experimentally- and naturally-infected horses, antigen-specific IgG(T) levels were significantly higher than those in cyathostomin negative animals, with antigen-specific IgG(T) levels shown to have a significant positive correlation with encysted larval burden (McWilliam et al. Reference McWilliam, Nisbet, Dowdall, Hodgkinson and Matthews2010). As horses invariably harbour a range of cyathostomin species, a diagnostic test will need to take account of this complexity. Here, recombinant GALA proteins were generated from four common cyathostomin species and the specificity and immunogenicity of each examined. These proteins were assessed by the enzyme linked immuno sorbent assay (ELISA) for their ability to predict the presence of encysted larval infection and the level of burden.
MATERIALS AND METHODS
Parasite material
Individual adult cyathostomins were removed from the large intestinal luminal contents of naturally-infected horses at post mortem (Dowdall et al. Reference Dowdall, Matthews, Mair, Murphy, Love and Proudman2002). The worms were identified to species by morphological means based on the key of Lichtenfels et al. (Reference Lichtenfels, Kharchenko and Dvojnos2008). Cyathostomin encysted larvae were recovered by pepsin-hydrochloric (HCl) digestion or by manual removal from the mucosa and submucosa as described previously (Dowdall et al. Reference Dowdall, Matthews, Mair, Murphy, Love and Proudman2002).
Serum samples
For immunoblotting and ELISA experiments, sera from different sources were used to examine the specificity and immunogenicity of each recombinant protein. First, sera were used from six ponies that had been raised in helminth free (HF) conditions in the UK. Three of these ponies (Ponies 102, 103, 106) were maintained HF until necropsy and the remaining three (Ponies 101, 104, 105), were subjected to a ‘trickle’ infection regime with cyathostomin third stage larvae as described previously (Murphy and Love, Reference Murphy and Love1997). The sera from these ponies were used as a source of cyathostomin negative control sera (classified here as HF) and cyathostomin positive control sera (classified here as cyathostomin infected, CI). A CI serum pool comprised samples from the three experimentally infected ponies taken at 12–16 weeks post-infection. These time points were selected on the basis of high serum IgG(T) reactivity in these ponies to native encysted larval antigen complexes identified in Dowdall et al. (Reference Dowdall, Matthews, Mair, Murphy, Love and Proudman2002). A pool of negative control serum was prepared from samples obtained from the three HF ponies. These serum pools were used as controls in all subsequent immunoblotting and ELISA studies. Serum was also available to examine, by immunoblotting, the cross reactivity of the GALA recombinant proteins to those present in other nematode species. In these studies, sera from horses infected with Strongylus edentatus or Strongylus vulgaris (Klei et al. Reference Klei, Torbert, Chapman and Ochoa1982) or with Parascaris equorum or Strongylus westeri (Dowdall et al. Reference Dowdall, Proudman, Love, Murphy, Klei and Matthews2003) were used. To assess and measure the immunogenicity of the recombinant proteins, serum was used that was derived from naturally or experimentally infected horses from the UK and USA. For some of these groups, enumerated nematode burden data were available, which allowed comparison of antigen specific IgG(T) levels with level of burden. For immunoblotting studies, serum IgG(T) reactivity was assessed in a serum pool from seven naturally-infected horses from the UK, each of which had a high total mucosal burden (TMB) of >100 000 encysted larvae (Dowdall et al. Reference Dowdall, Matthews, Mair, Murphy, Love and Proudman2002). In the immunoblotting studies, serum IgG(T) reactivity was also assessed in a pool of sera obtained from 11 clinical cases of larval cyathostominosis. These horses were classified as clinical cases based on their presenting signs and the detection of large numbers of cyathostomin larvae in their faeces (Hodgkinson et al. Reference Hodgkinson, Lichtenfels, Mair, Cripps, Freeman, Ramsey, Love and Matthews2003). Rabbit sera were also used in the immunoblotting studies. Here, sera raised to the native antigen complex (Dowdall et al. Reference Dowdall, Proudman, Love, Murphy, Klei and Matthews2003) and to Cy-GALA-pat (McWilliam et al. Reference McWilliam, Nisbet, Dowdall, Hodgkinson and Matthews2010) were used. Antigen reactivity in rabbit serum obtained before and after immunisation was compared. In the ELISA studies, serum samples from individuals from various populations were tested for reactivity to each GALA protein. First, sequential serum samples from the six experimental ponies (above) were used in time course experiments to measure levels of antigen specific IgG(T) in each pony from before infection until 16 weeks after the start of the ‘trickle’ infection. Antigen specific IgG(T) levels were also measured in a group (UK+) of horses that comprised 25 horses sampled at a UK abattoir (Dowdall et al. Reference Dowdall, Proudman, Klei, Mair and Matthews2004), as well as the three experimentally infected ponies as described above (Murphy and Love, Reference Murphy and Love1997). Cyathostomin burdens in these horses were quantified with the TMB and total worm burden (TWB) calculated as described previously (Dowdall et al. Reference Dowdall, Proudman, Klei, Mair and Matthews2004). Antigen-specific serum IgG(T) levels were also measured in each of the 11 larval cyathostominosis cases from the UK (UK+Clin). Serum IgG(T) levels in these cohorts were compared with those in serum obtained from the six experimental ponies before infection (UK-, Murphy and Love, Reference Murphy and Love1997). Several groups of CI horses from the USA (US+) were also assessed for serum IgG(T) levels to each antigen. One group comprised 10 naturally-infected horses (Monahan et al. Reference Monahan, Chapman, Taylor, French and Klei1996). Another comprised 38 ponies subjected to various cyathostomin experimental infection/treatment regimens (Monahan et al. Reference Monahan, Chapman, Taylor, French and Klei1997, Reference Monahan, Chapman, Taylor, French and Klei1998; Chapman et al. Reference Chapman, French, Taylor and Klei2002b). Serum IgG(T) levels in these cohorts were compared with those in US cyathostomin free ponies that were infected with S. vulgaris, P. equorum and S. westeri (US−).
Sub-cloning and recombinant protein expression of Cy-Gala proteins from an additional four cyathostomin species
Like Cy-GALA-pat, two of the additional proteins were derived from clones selected by immunoscreening (McWilliam et al. Reference McWilliam, Nisbet, Dowdall, Hodgkinson and Matthews2010). Similar to the strategy used to ascribe a species identity to Cy-gala-pat, nucleotide sequences in these two clones were compared with gala sequences obtained by polymerase chain reaction (PCR) amplification from individual identified worms of various species. Based on this analysis, the clones were ascribed to the common species, Cylicocyclus ashworthi (sequence named as Cy-gala-ash) and Cyathostomum catinatum (sequence named as Cy-gala-cat). For recombinant protein expression, Cy-gala-ash and Cy-gala-cat sequences were used to design primers to facilitate PCR amplification of Cy-gala encoding sequence (minus the signal peptide sequence) from phage plaque eluates from respective clones selected by immunoscreening (McWilliam et al. Reference McWilliam, Nisbet, Dowdall, Hodgkinson and Matthews2010). Sequences encoding restriction enzyme sites were incorporated into each primer to facilitate unidirectional cloning [the nucleotide sequences reported in this paper have been submitted to GenBank, accession numbers: JN596964 (Cy-gala-ash), JN596966 (Cy-gala-cat), JN596967 (Cy-gala-gol), JN596968 (Cy-gala-lon)]. The primer sequences were: Cy-gala-ash (SacI, NotI sites underlined) sense 5′-ATTCGAGCTCCCATGAAGAACTTCGTCGTCAC-3′, antisense 5′-AGCTTGCGGCCGCATATCTTCTATCCGTGTTGAG-3′; Cy-gala-cat (NcoI, NotI sites underlined) sense 5′-ATGGCCATGGATGAGGATCGTGAAGAACATCGC-3′, antisense 5′- AGCTTGCGGCCGCATCTCTTTCATCTGTGTTGAGTCC-3′. The PCR conditions were as follows: 0·5 µm primers, 0·2 mm dNTPs and 1·5 mm MgCl2, with cycling conditions, 94 °C for 2 min, 30 cycles at 94 °C for 15 s, with an annealing temperature of 54 °C (Cy-gala-ash) or 53 °C (Cy-gala-cat) for 30 s, and 72 °C for 60 s, with a final cycle of 72 °C for 7 min. Amplifications were performed using Platinum Taq (Invitrogen) in combination with 2 µL of each phage plaque eluate in a reaction volume of 50 µL. For size determination, PCR products were analysed on 1·1% w/v agarose TAE gels using a TrackIt 100 bp DNA Ladder (Invitrogen) and stained with 1× GelRed (Biotium). Next, the expression plasmid (pET-22b(+), Novagen) and each PCR product were digested with the appropriate restriction enzymes and ligation of the PCR amplicons performed using T4 ligase (Promega). The derived plasmids were transformed into Escherichia coli JM109 competent cells (Promega) and selected on ampicillin-Luria-Bertani (LB) agar. For each species, one colony containing plasmid with an insert of the correct size was subjected to plasmid purification (Wizard Plus SV Miniprep kit, Promega) and the insert sequenced in full to confirm identity. On confirmation of sequence identity, plasmids were transformed into E. coli BL21-CodonPlus(DE3)-RIL competent cells (Stratagene) for expression of recombinant Cy-GALA-ash and Cy-GALA-cat. Recombinant protein expression was induced as described in McWilliam et al. (Reference McWilliam, Nisbet, Dowdall, Hodgkinson and Matthews2010). Similar to Cy-GALA-pat (McWilliam et al. Reference McWilliam, Nisbet, Dowdall, Hodgkinson and Matthews2010), Cy-GALA-cat was soluble and present in the bacterial lysate supernatant. This protein was purified directly on a HisTrapHP column (GE Healthcare), eluted in increasing concentrations of imidazole, then dialysed with 20 mm sodium phosphate, 0·5 m NaCl (pH 7·4), and stored at −20 °C. The Cy-GALA-ash protein was insoluble, so purification and dialysis were carried out as above, with the exception that 8 m urea was added to all buffers.
For isolation of gala sequences from Cylicostephanus goldi and Cylicostephanus longibursatus, conserved primers (sense 5′-ATGAACAAAACGTTAACATTTCTC-3′, antisense 5′-AGGAGGTGATGATGGCATG-3′) were designed using all of the gala sequences described above and used to PCR-amplify a portion of the gala gene from single, identified adult C. goldi and C. longibursatus using cDNA synthesized as described in Lake et al. (Reference Lake, Matthews, Kaplan and Hodgkinson2009). The PCR products were cloned into pGEM®-T Easy vector (Promega), plasmid preparations made and the inserts sequenced in both directions. To generate PCR products for sub-cloning for protein expression, species-specific primers were designed using the C. longibursatus and C. goldi sequences obtained from individual identified worms. For each, primer sequences (SacI and NotI restriction sites underlined) were: Cy-gala-gol sense 5′-ATTCGAGCTCCCAAGGTGTCATGGCCCTATTTG-3′, antisense 5′-ATTAGCGGCCGCCAGGTATCTTCTATCCGTGTTCAG-3′, and for Cy-gala-lon sense 5′-ATTCGAGCTCCCAAGGTGTCATGGACCTTTTTGG-3′, antisense 5′-ATTAGCGGCCGCATATCTTCTATCCGTGTTGATTCCG-3′. PCR conditions were as above, except that 0·25 µm primers and 0·4 µL of Platinum Taq were used in reaction volumes of 100 µL, and 30 amplification cycles performed with an annealing temperature of 58 °C. The PCR products and vector were digested with SacI (Promega) and NotI (Promega) and ligated into pET-22b(+) vector. Plasmids were transformed into E. coli JM109 Competent Cells (Promega) and selected on ampicillin-LB agar. Colonies were examined by PCR for the presence of an insert of the correct estimated size using vector-specific primers and plasmid preparations made from two colonies, which were sequenced using the same primers. Clones of the correct sequence were transformed into BL21-CodonPlus(DE3)-RIL cells as above. Recombinant Cy-GALA-lon and Cy-GALA-gol were insoluble, so were prepared and stored in the presence of 8 m urea as above. Nucleotide and amino acid sequence alignments were performed using ClustalW2 (Larkin et al. Reference Larkin, Blackshields, Brown, Chenna, McGettigan, McWilliam, Valentin, Wallace, Wilm, Lopez, Thompson, Gibson and Higgins2007) and sequence identity levels examined using MegAlign 10·0·1 (DNASTAR) based on the ClustalW2 alignments. Signal peptides were identified using SignalP 4·0 (Petersen et al. Reference Petersen, Brunak von Heijne and Nielsen2011). Sequences were translated and molecular mass estimated using the Sequence Manipulation Suite (http://www.bioinformatics.org/sms2/protein_mw.html).
Immunogenicity and specificity of recombinant GALA proteins assessed by immunoblotting
To assess immunogenicity and potential cross reactivity of each recombinant protein, binding of IgG(T) in sera from horses infected only with cyathostomins or with single-species experimental infections of non-cyathostomin helminths was investigated (for serum sources, see above). Serum IgG(T) reactivity was also assessed in serum pooled from naturally-infected horses from the UK (UK+) and in a pool of sera from larval cyathostominosis cases (UK+Clin). Finally, IgG reactivity in rabbit sera raised to a 20 kDa larval antigen complex and to recombinant Cy-GALA-pat (see above) were assessed. For blotting, 0·1 µg of each GALA protein was loaded, per lane, onto a 15-well, 12% NuPAGE gel with SeeBlue Plus2 protein standards used for size estimations (Invitrogen). An additional lane was loaded with 0·1 µg protein. After electrophoresis, this lane was removed and stained with Coomassie blue for comparison with the immunoblots. Blocking, primary, secondary and tertiary antibody steps and blot development were as described in McWilliam et al. (Reference McWilliam, Nisbet, Dowdall, Hodgkinson and Matthews2010).
Immunoreactivity of the recombinant GALA proteins assessed by ELISA
Once the immunogenicity and specificity of each new GALA protein was confirmed by immunoblotting, the ELISA was used to evaluate serum IgG(T) levels to each protein in various groups of equids (see above). First, IgG(T) levels to the GALA proteins were assessed in ponies over a time course of experimental infection (Murphy and Love, Reference Murphy and Love1997). Antigen-specific IgG(T) levels were then assessed in cohorts of horses from the UK (UK+) and from the USA (US+) for which the cyathostomin burden was known and in a cohort of larval cyathostominosis cases (see above). Serum IgG(T) responses to Cy-GALA-pat were compared in these studies. The ELISA plates (96-well flat bottomed Microlon High binding plates, Greiner Bio-One) were coated with recombinant GALA antigens at 2 µg mL−1. Each well was coated with 100 µL of antigen in coating buffer (0·1 m carbonate coating buffer, pH 9·6) overnight at 4 °C. Plates were washed six times with 0·05% Tween-20 in phosphate buffered saline (PBS) (PBS-T), then blocked using 200 µL block buffer (2% soya powder (Infasoy™ Cow and Gate Ltd), w/v in PBS), per well for 1 h at 37 °C. All serum dilutions were made in block buffer. The plates were washed three times (as above) and 100 µL sera (diluted 1:800) added to each well and incubated for 2 h at 37 °C. Each serum sample was tested in triplicate. Plates were washed six times, incubated for 1 h with (100 µL per well) goat anti-equine IgG(T) whole molecule (Serotec), diluted 1:400 in block buffer. The plates were washed six times, then 100 µL rabbit anti-goat Ig:HRP conjugate (Sigma), diluted 1:500 in block buffer, added to each well and incubated for 1 h at 37 °C. Reactions were developed by adding 100 µL o-Phenylenediamine dihydrochloride (OPD) solution prepared from SIGMAFAST OPD tablets (Sigma) to each well. After 15 min at room temperature, 50 µL 2·5 m H2SO4 were added to stop the reactions and the absorbance in each well read at 490 nm. On all plates, aliquots from the same pool of CI sera were tested in triplicate as a control for inter-plate variation. The results derived from all samples were then expressed as the percentage optical density (OD) of the CI sample mean for each plate. Minitab 17 Statistical Software for Windows was used to analyse the data. For the different infected groups (UK+, UKClin+, US+), the group medians of the percentage positivity were compared with those of the cyathostomin free horses (UK− and US−) by the Mann–Whitney test. A P value <0·05 was taken to indicate statistical significance.
Receiver operator characteristic (ROC) curve analysis
ROC curve analysis was performed to indicate the accuracy of each GALA protein ELISA result relating to cyathostomin infection (postive or negative) and to cyathostomin TMB and TWB by assessing the tradeoff of diagnostic sensitivity vs specificity over a range of cut-off values. As an estimate of test accuracy, the area under the curve (AUC) may be interpreted such that; AUC = 0·9–1·0 demonstrates an excellent level of discrimination between positive and negative results; AUC = 0·8–0·9 good discrimination; AUC = 0·7–0·8 fair discrimination; AUC = 0·6–0·7 poor discrimination and AUC = 0·5–0·6 no discrimination (Swets, Reference Swets1988). All ELISA data were subjected to ROC analysis using Prism 6 (Graphpad Software Inc, USA).
RESULTS
Analysis of GALA sequences from the four additional cyathostomin species
Alignment (Fig. 1) of the derived GALA protein sequences demonstrated that, like Cy-GALA-pat, all sequences from the additional four species contained an N-terminal histidine-rich motif and a highly-conserved domain (Marchler-Bauer et al. Reference Marchler-Bauer, Anderson, Derbyshire, DeWeese-Scott, Gonzales, Gwadz, Hao, He, Hurwitz, Jackson, Ke, Krylov, Lanczycki, Liebert, Liu, Lu, Lu, Marchler, Mullokandov, Song, Thanki, Yamashita, Yin, Zhang and Bryant2007), the function of which is unknown and which is termed as Domain of Unknown Function 148 in the two most closely related sequences from Caenorhabditis elegans. Comparative analysis (Table 1) indicated that the sequences were between 83·3% (Cy-GALA-ash) and 92·9% (Cy-GALA-cat) identical to Cy-GALA-pat at the amino acid level. The level of intra-specific identity in the derived amino acid sequences was higher than 90% for all five species examined.

Fig. 1. ClustalW alignment of Cy-GALA-pat with its orthologues in other cyathostomin species. Cy-GALA-pat (Accession Number: FJ882059) is aligned with GALA proteins from four additional cyathostomin species, Cylicocyclus ashworthi (Accession number, JN596964), Cyathostomum catinatum (Accession number, JN596966), Cylicostephanus longibursatus (Accession number, JN596968) and Cylicostephanus goldi (Accession number, JN596967). The domain of unknown function (DUF148) is boxed. The histidine-rich region is highlighted in grey.
Table 1. Details of GALA sequences representing recombinant proteins used in the ELISA

These are derived from the species: Cylicocyclus ashworthi (ash), Cyathostomum catinatum (cat), Cylicostephanus goldi (gol), Cylicostephanus longibursatus (lon) and Cyathostomum pateratum (pat).
Accession numbers of the sequences representative of each clone used for expression for each species are as follows: Cy-gala-pat – FJ882059·1, Cy-gala-cat – JN596966, Cy-gala-gol – JN596967, Cy-gala-lon – JN596968, Cy-gala-ash – JN596964.
Immunoreactivity and cyathostomin specificity of the recombinant GALA proteins
Coomassie staining of the four new recombinant GALA proteins (Fig. 2A) demonstrated that the approximate size observed for each corresponded to the calculated molecular mass. The immunoblot experiments demonstrated that IgG(T) in sera from HF ponies did not bind to any of the four recombinant GALA proteins, whilst IgG(T) in sera from ponies experimentally infected with cyathostomins (CI sera) bound to all four (Fig. 2B). In terms of cross reactivity to other helminth species, there was no, or negligible, IgG(T) binding to each of the four recombinant GALA proteins observed in sera from horses infected specifically with P. equorum, S. edentatus, S. vulgaris or S. westeri (Fig. 2B). To further examine immunogenicity of the proteins in naturally-infected horses, immunoblots were performed using sera pooled from horses that presented at an abattoir and which had high encysted larval burdens. Here, strong reactivity of IgG(T) to all four GALA proteins was observed (Fig. 3A). Likewise, IgG(T) in sera pooled from samples from larval cyathostominosis cases demonstrated strong reactivity to all proteins (Fig. 3B). The GALA proteins also bound IgG in sera from a rabbit immunised with the native 20 kDa complex, originally identified in encysted larvae (Dowdall et al. Reference Dowdall, Proudman, Love, Murphy, Klei and Matthews2003, Fig. 3C). Similarly, IgG in sera from a rabbit immunised with Cy-GALA-pat bound each of the four new recombinant GALA proteins (Fig. 3D). No binding was observed in pre-immunisation sera from either rabbit.

Fig. 2. Specificity of the four recombinant GALA proteins. (A) Coomassie stained sodium dodecyl sulphate polyacrylamide gel electrophoresis (SDS-PAGE) gel depicting all four new recombinant GALA proteins. ash = C. ashworthi recombinant protein; cat = C. catinatum recombinant protein, gol = C. goldi recombinant protein, lon = C. longibursatus recombinant protein, M = molecular weight marker. (B) Immunoblots of each recombinant protein (Cy-GALA-ash, -cat, -gol and -lon) probed for IgG(T) reactivity using sera from helminth free (HF) ponies, experimental cyathostomin infected (CI) ponies and horses infected specifically with Parascaris equorum (Pe), Strongylus edentatus (Se), Strongyloides westeri (Sw) or Strongylus vulgaris (Sv). M = molecular weight marker.
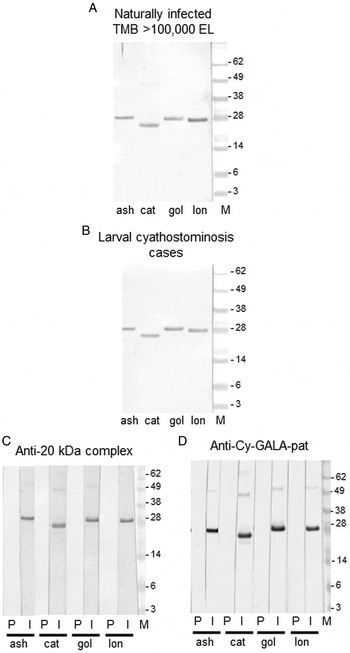
Fig. 3. Immunogenicity of the recombinant GALA proteins. (A) Immunoblot of each recombinant protein (Cy-GALA-ash, -cat, -gol and -lon) probed for binding of IgG(T) in a pool of serum from UK naturally infected horses, each of which had a total cyathostomin encysted larval burden of >100 000 larvae. (B) Immunoblot of each recombinant protein (Cy-GALA-ash, -cat, -gol and -lon) probed for binding of IgG(T) in a pool of serum from larval clinical cyathostominosis cases from the UK. (C) Immunoblot of each recombinant protein (Cy-GALA-ash, -cat, -gol and -lon) probed for binding of IgG in sera from a rabbit taken before (P) and after (I) immunization with the cyathostomin encysted larval 20 kDa complex (Dowdall et al. Reference Dowdall, Proudman, Love, Murphy, Klei and Matthews2003). (D) Immunoblot of each recombinant protein (Cy-GALA-ash, -cat, -gol and -lon) probed for binding of IgG in sera from a rabbit taken before (P) and after (I) immunization with Cy-GALA-pat (McWilliam et al. Reference McWilliam, Nisbet, Dowdall, Hodgkinson and Matthews2010).
ELISA and ROC curve analysis of serum IgG(T) responses to the recombinant GALA proteins in CI horses
A time course study was performed to analyse serum IgG(T) responses to each new GALA protein in experimentally-infected ponies across a trickle infection (Fig. 4). IgG(T) responses in infected ponies (Ponies 101, 104, 105) were analysed from before infection until 16 weeks after first challenge. GALA-specific IgG(T) in these individuals were compared with those in ponies that remained uninfected (Ponies 102, 103, 106). Similar to previous observations with GALA-pat (McWilliam et al. Reference McWilliam, Nisbet, Dowdall, Hodgkinson and Matthews2010), increases in antigen-specific IgG(T) were observed against all recombinant GALA proteins after infection; however, the dynamics of the repsonse to each varied amongst individuals. Serum IgG(T) responses to GALA-ash, lon and gol proteins increased more rapidly in Pony 104 than in the other two infected ponies. This was not the case with respect to responses to the GALA-cat protein, against which IgG(T) levels increased more slowly than to the other proteins. In Pony 104, IgG(T) levels to GALA-ash, gol and lon plateaued after 7 weeks post-infection and remained high until the end of the time course. Antigen-specific IgG(T) levels in Ponies 101 and 105 increased at a slower rate, but generally reached similar levels to those measured in Pony 104 by the end of the time course, with the exception of Pony 101's IgG(T) response to GALA-cat. Increases in antigen-specific IgG(T) levels were not observed in any of the HF ponies across the time course.

Fig. 4. IgG(T) responses in experimentally infected ponies to the four recombinant GALA proteins as measured by the ELISA. Recombinant GALA (A. GALA-ash, B. GALA-cat, C. GALA-gol, D. GALA-lon) protein-specific IgG(T) responses over an experimental trickle infection (Murphy and Love, Reference Murphy and Love1997). Six British native-breed ponies (6–12 months at the time of initial infection) were reared indoors with their dams and considered to be helminth naive prior to the start of the trial. Following weaning at 4 months, the ponies were maintained on a high-fibre pelleted ration and bedded on wood shavings. Three ponies (Ponies 101, 104 and 105) were infected with a total of 3·9 million cyathostomin third stage larvae (L3), administered as a trickle infection of 150 000 L3 by nasogastric tube, three times a week. Ponies 102, 103 and 106 were maintained as uninfected controls. Pony 104 was necropsied at 20 weeks post-infection and the remaining ponies at 60–62 weeks post-infection.
Next, levels of serum IgG(T) to all four new GALA proteins and GALA-pat were compared in equids for which cyathostomin burden data were available, thus allowing comparison of parasitological parameters with specific IgG(T) levels in matched end-point serum samples. Also analysed were GALA-specific serum IgG(T) responses in horses that presented with larval cyathostominosis. First, serum IgG(T) levels were compared in CI groups (UK+, US+, UKClin+) with those in cyathostomin negative groups (UK−, US−) from the UK and the USA. For the UK+ and UKClin+ cohorts, for all five proteins, specific IgG(T) levels were significantly higher than in cyathostomin negative horses (Table 2). Likewise, for the US population, levels of IgG(T) to each protein were significantly higher in the US+ group than in the US− (cyathostomin free) group. ELISA data from horses for which cyathostomin burdens were available were subjected to ROC curve analysis. For all five proteins, GALA-specific IgG(T) levels in equids, which were known to be truly cyathostomin negative (i.e. the UK− and US− groups) were compared with GALA-specific IgG(T) levels measured in CI horses from the UK or the US (Table 3). Here, high AUC values (i.e. >0·9) were obtained for all proteins (AUC values: ‘ash’ and ‘lon’ > ‘pat’ > ‘cat’ > ‘gol’). The highest sum of percentage sensitivity and specificity values generated here were then used to calculate cut-off percentage positivity thesholds for each GALA protein (Table 3). Based on these values, specificity ranged from 82·43% (ash) to 95·95% (cat) and sensitivity from 77·78% (cat, gol) to 100% (ash and pat). When the data were stratified at different cyathostomin TMB thresholds, taking into account that some horses negative for TMB were positive for cyathostmin luminal parasites (Table 4), AUC values and sensitivity and specificity values calculated were lower. Next, TWB thresholds were examined to assess if the outputs were likely to be confounded by the half life of GALA-specific serum IgG(T) responses; for example, in equids where there had been recent emergence of previously-encysted larvae or in those that had been administered recently with an effective larvicidal treatment. When the cohort was stratified on the basis of cyathostomin TWB, for all proteins, AUC and sensitivity and specificity values were higher than when the group was stratified on the basis of TMB alone (Table 4). For ‘0 TWB’ threshold, AUC values for IgG(T) responses to all five GALA proteins were high (i.e. >0·9). Finally, the cohort was statfied on the basis of a threshold of 5000 mucosal larvae or TWB. At a cutoff of a TMB of 5000 larvae, AUC values ranged from 0·69 (GALA-ash) to 0·77 (GALA-cat), so giving ‘good’ discrimination. At a cutoff of 5000 TWB, GALA-cat and GALA-lon (AUC values for both = 0·82) gave the highest values, with AUC values for the other protiens exceeding 0·7. When the data were partitioned such that the threshold value was 10 000 TMB or TWB, most AUC values generated were <0·7 (data not shown).
Table 2. Comparison of serum IgG(T) levels in cyathostomin-infected vs non-infected groups from the UK and USA

Horses were naturally infected or subjected to experimental infection. UK+ comprised 26 cyathostomin naturally-infected horses sampled at an abattoir. UK− comprised 6 cyathostomin-negative ponies raised helminth-free. UKClin+ comprised 11 equids with larval cyathostominosis. US+ (n = 48) comprised natuarally or experimentally infected equids from the USA. US− comprised 3 experimental equids from the US riased cyathostomin-free. For the infected groups (UK+, UKClin+, US+), group medians of the percentage positivity were compared with that of cyathostomin-free horses (UK− and US−) by the Mann–Whitney test. A P value <0·05 was taken to indicate statistical significance.
Table 3. Receiver operator characteristic (ROC) curve analysis of ELISA data relating to antigen-specific IgG(T) levels in horses comparing cyathostomin-positive vs cyathostomin-negative horses

Horses were naturally infected or subjected to experimental infection. The UK population comprised 26 cyathostomin-positive and 6 cyathostomin (true) negative equids. The US population comprised 48 cyathostomin-positive and 3 cyathostomin (true) negative equids. The area under the curve (AUC), 95% confidence intervals (CI) and P values for data generated by ROC curve analysis for each protein are shown. A cut-off percentage positivity value indicated for each protein is based on the value calculated as the highest sum of percentage sensitivity and specificity values obtained in the ROC analysis.
Table 4. Receiver operator characteristic (ROC) curve analysis of ELISA data relating to antigen-specific IgG(T) levels in horses from the UK and USA at various thresholds of larvae in the mucosa (total mucosal burden, TMB) and of larvae in the mucosa plus lumen (total worm burden, TWB)

Horses were naturally infected or subjected to experimental infection. The area under the curve (AUC), 95% confidence intervals (CI), P values, cut-off values and % sensitivity and specificity are shown.
DISCUSSION
Cyathostomins have high pathogenic potential in equids due to their capacity to cause life-threatening colitis when encysted larvae emerge from the intestinal wall. For this reason, the administration of anthelmintics that have activity against these developmental stages is regarded by many as an essential component of equine parasite control programmes in developed regions (Nielsen, Reference Nielsen2012; Matthews, Reference Matthews2014; Nielsen et al. Reference Nielsen, Pfister and von Samson-Himmelstjerna2014). As it is likely that most horses will not carry life-threatening levels of encysted larvae, the availability of a diagnostic test that can inform on the presence or burden of these stages would help in directing the strategic application of larvicidal anthelmintic treatments.
The development of a diagnostic test for cyathostomins is complicated by the complex nature of this group of nematodes. There are 50 recognized cyathostomin species (Lichtenfels et al. Reference Lichtenfels, Kharchenko and Dvojnos2008); however, whilst a range of species is found in individuals, the majority of the burden has been found consistently across geographic regions to comprise 5–10 common species. The species investigated here were selected on the basis that they were the most prevalent species identified across multiple studies. In particular, C. longibursatus and C. catinatum are regularly recorded as the commonest species recovered from horses (Krecek et al. Reference Krecek, Reinecke and Horak1989 [South Africa]; Mfitilodze and Hutchinson, Reference Mfitilodze and Hutchinson1990 [Tropical Australia]; Bucknell et al. Reference Bucknell, Gasser and Beveridge1995 [Victoria, Australia]; Gawor, Reference Gawor1995 [Poland]; Lichtenfels et al. Reference Lichtenfels, McDonnell, Love and Matthews2001 [UK], Chapman et al. Reference Chapman, French and Klei2002a [USA]; Boxell et al. Reference Boxell, Gibson, Hobbs and Thompson2004 [Western Australia]; Kuzmina et al. Reference Kuzmina, Kharchenko, Starovir and Dvojnos2005 [Ukraine]). A representative protein for C. ashworthi was also selected here. This species has been regarded as a synonym of Cylicocyclus nassatus; the two are similar morphologically but are now regarded as separate (Lichtenfels et al. Reference Lichtenfels, Kharchenko, Sommer and Ito1997). Earlier studies may have misidentified C. ashworthi (Chapman et al. Reference Chapman, French and Klei2002a), so this species may have been under-represented. Indeed, where C. ashworthi has been defined as distinct from C. nassatus, it has been found at high prevalence (Lichtenfels et al. Reference Lichtenfels, McDonnell, Love and Matthews2001; Kuzmina et al. Reference Kuzmina, Kharchenko, Starovir and Dvojnos2005; Kornaś et al. Reference Kornaś, Skalska, Nowosad, Gawor, Kharchenko and Cabaret2009). Likewise, C. goldi and C. pateratum are species detected in high abundance in surveys performed in different regions (Reinemeyer et al. Reference Reinemeyer, Smith, Gabel and Herd1984; Krecek et al. Reference Krecek, Reinecke and Horak1989; Mfitilodze and Hutchinson, Reference Mfitilodze and Hutchinson1990; Gawor, Reference Gawor1995; Collobert-Laugier et al. Reference Collobert-Laugier, Hoste, Sevin and Dorchies2002; Boxell et al. Reference Boxell, Gibson, Hobbs and Thompson2004; Traversa et al. Reference Traversa, Milillo, Barnes, von Samson-Himmelstjerna, Schurmann, Demeler, Otranto, Lia, Perrucci, Frangipane di Regalbono, Beraldo, Amodie, Rohn, Cobb and Boeckh2010). As there does not appear to have been an obvious shift in the prevalence ranking of cyathostomin species over time, despite the advent and spread of anthelmintic resistance (Chapman et al. Reference Chapman, French and Klei2002a), it is with confidence that inclusion of the species here represent common components of cyathostomin infections now, as well as in the future.
The level of intra-specific variation observed in the GALA sequence was low in all species studied (Table 1). Further, the diversity observed in GALA sequence between these species was far lower than that seen when the GALA sequences were compared with orthologous sequences present in non-cyathostomin species (data not shown), thus indicating that the test is unlikely to be affected by cross reactivity to non-cyathostomin infections. In agreement with this, the specificity of each recombinant GALA protein was confirmed in immunoblotting experiments when each was probed with sera from horses specifically infected with large strongyle species, P. equorum or S. westeri, and no reactivity was observed. Further immunoblotting experiments demonstrated that all four GALA proteins bound strongly to IgG(T) in serum pooled from CI horses and in serum from clinical cases of larval cyathostominosis. These results were substantiated by the ELISA data, which demonstrated significantly higher specific serum IgG(T) levels to each GALA protein in the USA and UK CI groups compared with the respective cyathostomin negative groups (Table 2). The time course study supported these findings, demonstrating robust anti-GALA serum IgG(T) responses in all infected ponies. Similar to previous results obtained with Cy-GALA-pat (McWilliam et al. Reference McWilliam, Nisbet, Dowdall, Hodgkinson and Matthews2010) and with native antigens (Dowdall et al. Reference Dowdall, Matthews, Mair, Murphy, Love and Proudman2002), specific IgG(T) increased to GALA-ash, -gol and -lon in Pony 104 earlier than in the other infected ponies. The more severe clinical parameters observed in Pony 104 indicated that it had developed the greatest burden of mucosal larvae during the infection protocol and, when this animal was euthanized (at 20 weeks post-infection), it was found to have a very high (>700 000 cyathostomin larvae) burden (Murphy and Love, Reference Murphy and Love1997). The other two ponies had substantially lower burdens, but they were not necropsied until 60 and 62 weeks post-infection. The differences in the dynamics in IgG(T) response to the GALA-cat protein may reflect the relative proportions in species present over the trickle infection period (Murphy and Love, Reference Murphy and Love1997). The observations here indicate that the long-term goal of this work should be the development of a test incorporating several GALA proteins derived from the commonest species due to the potential risk of false negative results in some individuals in which a single species may be less abundant.
The ROC AUC values indicate that all proteins provide excellent discriminatory information when comparing true cyathostomin negative with true cyathostomin postive individuals (Table 3). The AUC values obtained were similar for all five GALA proteins. When horses were stratified on the basis of mucosal larval burden (Table 4), the derived ROC AUC values were lower than the values obtained when the true cyathostomin negative group was compared with the cyathostomin positive horses. This is likely due to the fact that some horses in the TMB-negative group harboured luminal stages of cyathostomins; such horses may have residual GALA-specific IgG(T) in their serum stimulated by recent encysted larval infection. This observation is unlikely to be due to cross-reactivity to antigens in luminal worms, as it was demonstrated previously that GALA protein and gala transcript could not be detected in these stages (McWilliam et al. Reference McWilliam, Nisbet, Dowdall, Hodgkinson and Matthews2010). The serum half-life of equine IgG(T) has been measured as 21 days (Sheoran et al. Reference Sheoran, Timoney, Holmes, Karzenski and Crisman2000), so a limitation of this test is that, in horses that have had recent emergence of encysted larvae or have received a recent effective larvicidal treatment, GALA-specific IgG(T) levels will still be elevated. With this in mind, a future objective will be to investigate the dynamics of circulating GALA protein-specific serum IgG(T) after anthelmintic treatments known to be effective against encysted larval stages. This issue is not unique to the cyathostomin test and is a concern with the commercially-available diagnostic assay for the tapeworm, Anoplocephala perfoliata, in horses. The tapeworm diagnostic is also based on the binding of serum IgG(T) to parasite proteins (Barrett et al. Reference Barrett, Farlam and Proudman2004; Abbott et al. Reference Abbott, Mellor, Barrett, Proudman and Love2008). Despite these concerns, the A. perfoliata ELISA is still regarded as a useful tool in practice (Kjaer et al. Reference Kjaer, Lungholt, Nielsen, Olsen and Maddox-Hyttel2007; Abbott and Barrett, Reference Abbott and Barrett2008). For these reasons, the test output must always be interpreted alongside the clinical and treatment history of the individual or population of animals being assessed.
In terms of informing on cyathostomin burden, the GALA tests performed well when used to discriminate horses at an encysted larval burden threshold of 5000 worms (Table 4). At higher TMB thresholds (10 000 +), the ROC AUC values were lower (i.e. <0·7) indicating that the tests could not discriminate well horses above and below thresholds >10 000 larvae. When the ROC curve analysis was repeated with horses segregated on the basis of threshold of TWB (i.e. mucosal and luminal nematode burdens, Table 4), the AUC values obtained were higher at the 5000 TWB threshold compared with when the horses were grouped according to TMB. Again, with this in mind, the test results will need to be interpretated in the context of the clinical or treatment history and also with respect to the time of year that the sample is anlysed. In northern temperate climates, experimental studies (Reinemeyer et al. Reference Reinemeyer, Smith, Gabel and Herd1986) have shown a peak of cyathostomin larval luminal stages in spring (presumably derived from emerged encysted larvae acquired during the previous season). These larvae develop to adults in late spring, which persist through summer and are added to by immature worms, which co-contribute to a second adult worm peak in late summer. In the Reinemeyer et al. (Reference Reinemeyer, Smith, Gabel and Herd1986) studies, there was a loss of luminal stages in autumn; hence, the GALA ELISA is likely to provide most diagnostic value if used in these regions in autumn and winter when it can be used to inform on the need for larvicidal treatment, as well as in the differenential diagnosis of the disease in horses presenting with weight loss and/or diarrhoea. A cocktail of the five proteins, and combinations thereof, will now be assessed to examine which should be combined to optimise the test. A balance will need to be struck between the resource required to generate each protein and the diagnostic value provided by the various combinations.
ACKNOWLEDGEMENTS
The authors would like to thank all veterinary surgeons who supplied the sera used in this study and Professor Tom Klei (Louisanna State University, USA) and Professor Sandy Love (University of Glasgow) for the supply of sera from the experimental studies.
FINANCIAL SUPPORT
The authors would like to thank the Horserace Betting Levy Board (HBLB, Project Grant 723 to JBM) and the Thoroughbred Breeders Association (Part contribution of project grant awarded by HBLB to JBM) for their generous financial support of this project.