1. Introduction
Sandstone petrography and geochemical composition of shales have been used to constrain provenance of clastic supply and sedimentary evolution of sedimentary basins (e.g. Bhatia & Crook, Reference Bhatia and Crook1986; Cullers, Reference Cullers2000; McLennan, Hemming & Hanson, Reference McLennan, Hemming and Hanson1993; Taylor & McLennan, Reference Taylor and McLennan1985; Mongelli et al. Reference Mongelli, Critelli, Perri, Sonnino and Perrone2006; Critelli et al. Reference Critelli, Mongelli, Perri, Martín-Algarra, Martín-Martín, Perrone, Dominici, Sonnino and Zaghloul2008; Perri et al. Reference Perri, Mongelli, Sonnino, Critelli and Perrone2005, Reference Perri, Cirrincione, Critelli, Mazzoleni and Pappalardo2008, Reference Perri, Critelli, Mongelli and Cullers2010; Caracciolo et al. Reference Caracciolo, Le Pera, Muto and Perri2009; Zaghloul et al. Reference Zaghloul, Critelli, Perri, Mongelli, Perrone, Sonnino, Tucker, Aiello and Ventimiglia2010). The mineralogy of shales and siltstones has also been used in determining the provenance and to check changes in the minerals derived from source rocks during weathering and diagenesis. Trace element ratios such as La/Sc, Th/Sc, Th/Co, Th/Cr, Cr/Th and Eu/Eu* have been used to distinguish sediments derived from different source rocks and to infer tectonic settings (McLennan, Hemming & Hanson, Reference McLennan, Hemming and Hanson1993). In particular, the relative amount of silicic to basic rock input from the source to shales and siltstones can be determined from ratios of trace elements that are concentrated in silicic source rocks (La and Th) relative to those that are concentrated in basic rocks (Co, Cr, Ni). Also, the size of the Eu anomaly (Eu/Eu*), LREE/HREE (light/heavy rare earth element) ratios and three-component trace element plots (e.g. La–Th–Sc) may also be used to determine the provenance of shales and siltstones as most basic rocks contain no negative Eu anomaly and often contain low LREE/HREE ratios, while more silicic rocks are more likely to contain a negative Eu anomaly and often have high LREE/HREE ratios (e.g. Bhatia & Crook, Reference Bhatia and Crook1986; Cullers, Reference Cullers2000 and many others). The Western Mediterranean Alpine region was marked during Miocene time by intense deformation and high pressure/low temperature (HP/LT) metamorphism (at pressures in the range of 0.8–2 GPa), followed by a retrograde phase associated with decompression, suggesting subduction and subsequent exhumation of continental crust. Thick synorogenic siliciclastic turbidite successions started to accumulate within the Apennine foreland basin system (e.g. the Tufiti di Tusa Formation) and in the Maghrebian and Betic Flysch troughs during late Oligocene to early Miocene times (Perrone et al. Reference Perrone, Martìn-Algarra, Critelli, Decandia, D'Errico, Estèvez, Iannace, Lazzarotto, Martìn-Martìn, Martìn-Rojas, Mazzoli, Messina, Mongelli, Vitale, Zaghloul, Chalouan and Moratti2006 and references therein).
In this paper, we study the sandstone petrography and mudrock mineralogy and geochemistry of the lower Miocene volcaniclastic sediments of the Tufiti di Tusa Formation (Sicilide Complex; southern Apennines) and characterize their provenance signatures, climatic, geodynamic and palaeogeographic significance, and diagenetic evolution.
In southern Italy, the Sicilide Complex strata represent deposition in a remnant ocean basin related to the subduction of the Adria oceanic lithosphere beneath the Mesomediterranean microplate (e.g. Critelli, Reference Critelli1999; Guerrera, Martín-Algarra & Perrone, Reference Guerrera, Martín-Algarra and Perrone1993; Critelli et al. Reference Critelli, Mongelli, Perri, Martín-Algarra, Martín-Martín, Perrone, Dominici, Sonnino and Zaghloul2008). This subduction was active during Miocene time, producing an accretionary prism, the Sicilide Complex, and calcalkaline volcanism above it, which is now preserved in Sardinia. The active volcanic source and the crystalline sources of the Calabria-Peloritani Terrane are recorded within the Sicilide foredeep, forming distinct early Miocene siliciclastic turbidite systems with sand compositions ranging from volcanolithic (Tufiti di Tusa Formation) to quartzolithic and quartzofeldspathic (Albanella, Corleto and Colle Cappella formations) (e.g. Critelli & Le Pera, Reference Critelli and Le Pera1998; Critelli, Reference Critelli1999). The volcanic source of sediment is mainly related to calcalkaline volcanic arcs (Guerrera & Veneri, Reference Guerrera and Veneri1989) or, more recently (latest Neogene–Quaternary), to intraorogenic alkaline volcanism. Lower Miocene (Burdigalian) foreland basin clastic sediments are widespread in the southern Apennines. Sediments of the upper Sicilide Complex represent the oldest deposits of the foredeep basin (Critelli, Reference Critelli1999).
2. Geology
The southern Apennines is an E-verging accretionary wedge developed in Neogene times above W-dipping subduction of the Apulian–Ionian lithosphere (Doglioni et al. Reference Doglioni, Harabaglia, Martinelli, Mongelli and Zito1996). The southern Apennines and northern Calabria are associated with the Tyrrhenian back-arc basin and bear today a frontal active accretionary wedge, below sea-level, whereas the main elevated ridge to the west is undergoing uplift and extension.
The tectonic style of the area is dominated by a large-scale duplex system, consisting of a complex architecture of tectonic units derived from the internal Apulia carbonate platform, overlain by a rootless thrust sheet derived from the western Adria palaeomargin and distally related oceanic basins (e.g. Patacca & Scandone, Reference Patacca, Scandone, Mazzotti, Patacca and Scandone2007 and references therein).
The subduction of the Tethyan oceanic lithosphere located to the west of the Adria margin was active in Oligocene and Miocene time, and was associated with calcalkaline volcanism coeval with intracontinental rifting occurring in Sardinia. Subduction-related volcanism (between 32 and 11 Ma) was widespread along the western side of Sardinia (e.g. Assorgia et al. Reference Assorgia, Barca, Onnis, Secchi and Spano1986). This volcanism was subdivided into two main phases: calcalkaline eruptions of lavas from 32 to 26 Ma, and explosive eruptions, which alternated or were contemporaneous with effusive events, between 23 and 11 Ma (Boni, Del Vecchio & Lirer, Reference Boni, Del Vecchio and Lirer1990). The volcanic products of the first phase are andesite, basaltic andesite and basalt, whereas the second phase includes pyroclastic rocks from dacite to rhyolite composition.
According to different palaeogeographic restorations (Ogniben, Reference Ogniben1969; Pescatore et al. Reference Pescatore, Renda, Schiattarella and Tramutoli1999; Critelli, Reference Critelli1999; Patacca & Scandone, Reference Patacca, Scandone, Mazzotti, Patacca and Scandone2007 and many others), the southern Apenninic tectonic units derive from deformation of different palaeogeographic domains, represented, from west to east, by the Liguride–Sicilide basin (Flysch Domain), the Apennine carbonate platform, the Lagonegro basin and the Apulian carbonate platform (External Domain). In addition, the Internal Domain is represented by the metamorphic units of the Calabria–Peloritani Terrane, which does not crop out in the study area, but far towards the SW (Bonardi et al. Reference Bonardi, Cavazza, Perrone, Rossi, Vai and Martini2001). The Liguride basin was formed on Tethyan oceanic crust, which was bounded, on its western and eastern sides, by continental crust belonging to the Internal (Calabria–Peloritani Terrane) and External Domains (Adria margin), respectively. The Adria margin includes the Apennine platform that was formed on the continental crust at the western margin, and towards the east the Lagonegro basin and the Apulian platform (e.g. Sgrosso, Reference Sgrosso1994 and references therein). The Lagonegro basin was located between the Apennine platform to the west and the Apulian platform to the east, the latter representing the proximal zone of the west Adria palaeomargin (e.g. Pescatore et al. Reference Pescatore, Renda, Schiattarella and Tramutoli1999; Patacca & Scandone, Reference Patacca, Scandone, Mazzotti, Patacca and Scandone2007). The Sicilide Unit has been interpreted as representing deposition on the eastward flank of the Tethyan oceanic basin (e.g. Ogniben, Reference Ogniben1969; Critelli, Reference Critelli1999; Lentini et al. Reference Lentini, Carbone, Di Stefano and Guarnieri2002; Cavalcante et al. Reference Cavalcante, Fiore, Piccarreta and Tateo2003; Patacca & Scandone, Reference Patacca, Scandone, Mazzotti, Patacca and Scandone2007).
The studied area is located in the Sicilide Unit cropping out at the front of the southern Apennines near Valsinni village (Fig. 1). In this sector, the Sicilide Unit overlies the Sannio Unit and is covered unconformably by Plio-Pleistocene siliciclastic sediments of the Bradano Trough (Patacca & Scandone, Reference Patacca, Scandone, Mazzotti, Patacca and Scandone2007 and references therein). Information on depositional characteristics and ages of the stratigraphic sequences representative of the thrust sheets and thrust-sheet-top deposits of the southern Apennines close to the studied area is given in a synoptic scheme (Fig. 2).
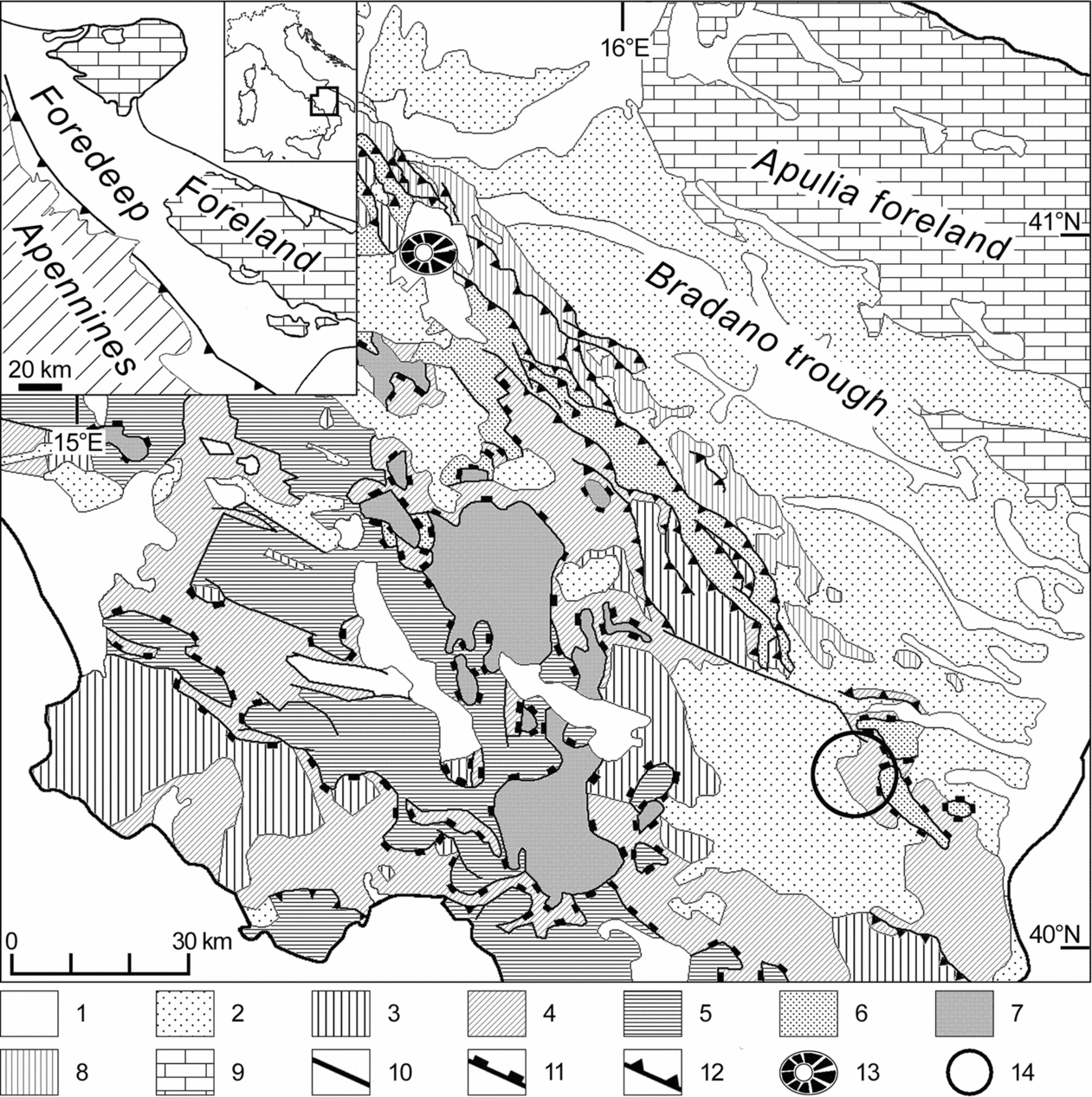
Figure 1. Geological sketch map of the southern Apennine and location of the studied area (modified from Patacca & Scandone, Reference Patacca, Scandone, Mazzotti, Patacca and Scandone2007). 1 – Continental and coastal deposits; volcanic rocks and volcaniclastic deposits (Holocene–middle Pleistocene). 2 – Middle Pleistocene–Pliocene thrust-top deposits. Terrigenous marine and paralic deposits filling the Bradano trough and unconformably overlying the Apennine Units. 3 – Miocene deposits (Gessosso–Solfifera Formation, San Bartolomeo Formation, Gorgoglione Formation, Albidona Formation). 4 – North Calabrian and Sicilide Units (lower Miocene–Cretaceous). 5 – Aburno-Cervati, Monti della Maddalena and minor units derived from the Campania–Lucania carbonate platform (lower Miocene–Upper Triassic). 6 – Sannio Unit (middle Miocene–Lower Cretaceous). 7 – Lagonegro Unit (Lower Cretaceous–Middle Triassic). 8 – Tufillo–Serra Palazzo and Daunia units (upper Miocene–Palaeogene). 9 – Cretaceous carbonates of the Murge foreland. 10 – Faults, including normal faults, lateral ramp and strike-slip faults. 11 – Low-angle thrust. 12 – High-angle thrust. 13 – Caldera rim (Vulture Volcano). 14 – Studied area.
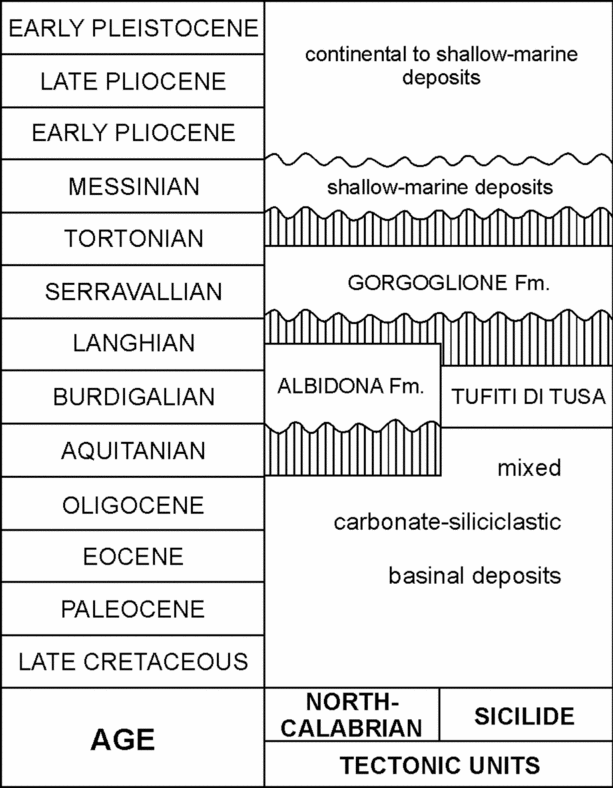
Figure 2. Synoptic scheme of depositional characteristics and ages of the stratigraphic sequences representative of the thrust sheets and thrust-sheet-top deposits of the southern Apennines close to the studied area (modified from Patacca & Scandone, Reference Patacca, Scandone, Mazzotti, Patacca and Scandone2007).
The Sicilide Unit, established in the southern Apennines by Ogniben (Reference Ogniben1969), is widespread in the Basilicata region. The Sicilide stratigraphic succession, in the northern sector of the Basilicata region, consists of lower Cretaceous varicoloured clay and the Paleocene–lower Miocene Corleto-Perticara Formation, whereas in the studied area it consists of upper Cretaceous varicoloured clay, the Maastrichtian–lower Eocene Monte S. Arcangelo Formation, upper Eocene–lower Miocene varicoloured clay and lower Miocene Tufiti di Tusa Formation (e.g. Patacca & Scandone, Reference Patacca, Scandone, Mazzotti, Patacca and Scandone2007 and references therein). Siliciclastic strata of the Sicilide Complex include quartzolithic, volcanolithic and quartzofeldspathic sandstones (Critelli, Reference Critelli1999).
The studied succession consists of a nearly 100 m thick alternating sequence of volcaniclastic, calciclastic and quartzolithic turbidite strata. The sequence can be divided into two main parts based on both compositional and depositional characters: a lower fine-grained sandstone–mudstone part of calciclastic and quartzolithic composition, characterized by discontinuous and disorganized terrigenous supply; and an upper part, mostly of volcaniclastic composition, characterized by fine-grained yellow and grey sandstones with low-angle cross-bedding passing into parallel-laminated sandstones with some conglomerate beds alternating with grey centimetric mudrocks. The studied mudrock and sandstone samples were collected along the upper part of the stratigraphic succession (Fig. 3). The volcaniclastic turbidite strata form a thickening and coarsening upward sequence, and represent higher energy events of the stratigraphic succession (e.g. Critelli et al. Reference Critelli, De Rosa, Sonnino and Zuffa1990). Thick volcanolithic strata of the Tufiti di Tusa include syneruptive andesite and basaltic andesite sandstones, recording the climax of the calcalkaline volcanic arc activity (Critelli et al. Reference Critelli, De Rosa, Sonnino and Zuffa1990). Interbedded with carbonatoclastic sequences of the Adria forebulge (Capaccio–Roccadaspide and Cerchiara formations), similar andesitic volcanolithic sand testifies to wide dispersal of the neovolcanic detritus in the forebulge and back-bulge depozones (Critelli, Reference Critelli1999). Metamorphiclastic quartzolithic sandstones occur in the lower portions of the Tufiti di Tusa before deposition of volcaniclastic strata (Critelli et al. Reference Critelli, De Rosa, Sonnino and Zuffa1990). Thick carbonate (calcarenite-marl) turbidite strata are interbedded with quartzolithic and volcaniclastic strata of the Tufiti di Tusa Formation, testifying to provenance from the flexed forebulge domain (Critelli, Reference Critelli1999). Thus, the Tufiti di Tusa Formation caps the Sicilide Complex (Burdigalian–early Langhian) and represents the oldest sedimentary succession of the foredeep basin (e.g. Critelli, Reference Critelli1999).
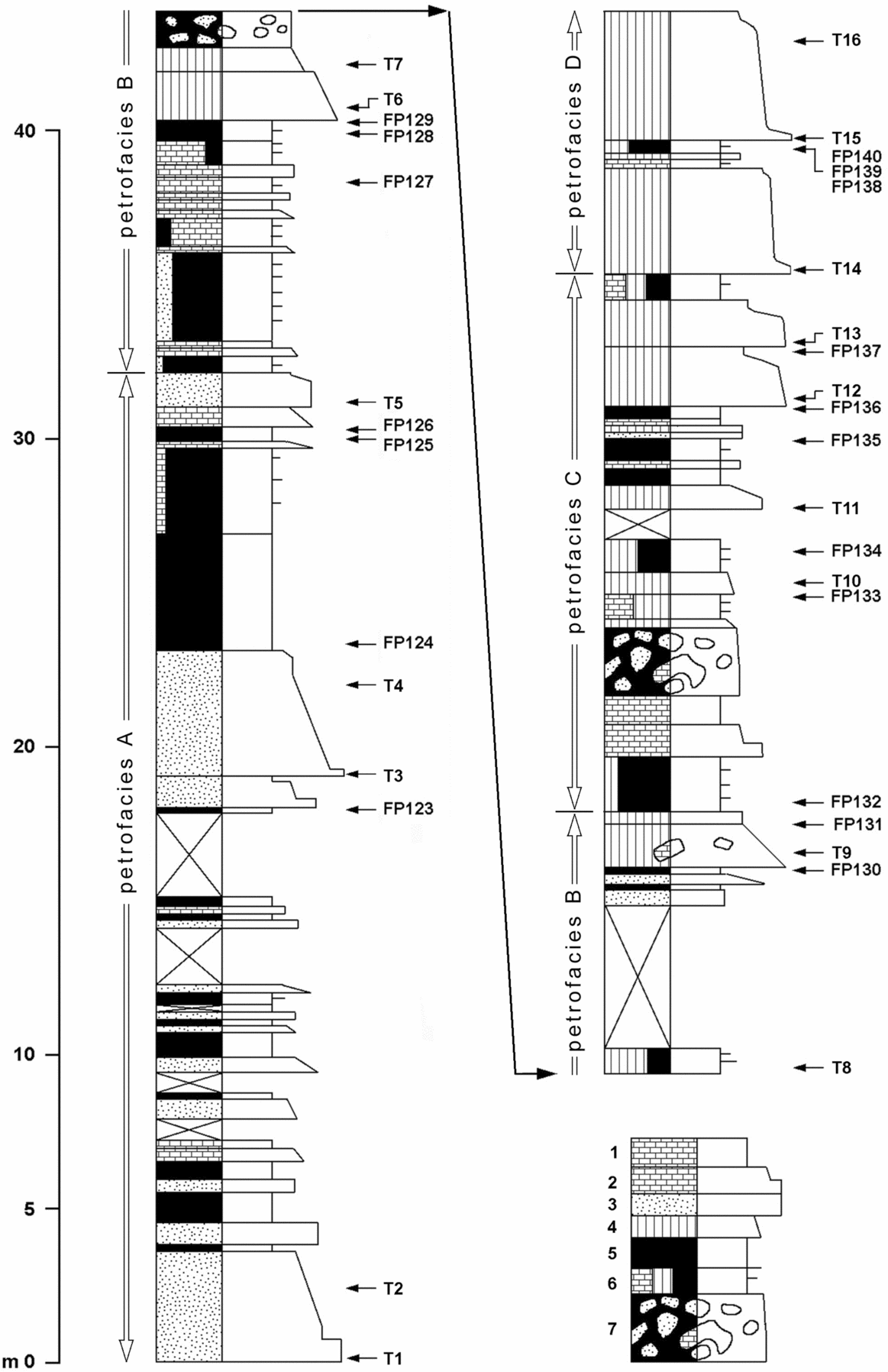
Figure 3. Sedimentological log of the volcaniclastic and carbonatoclastic deposits at the Canale Coppozzolo stratigraphic succession near Valsinni village, with the location of the studied mudrock (from FP123 to FP140) and sandstone (from T1 to T16) samples. 1 – calcilutites and marls; 2 – calciclastic sandstones; 3 – quartzolithic sandstones; 4 – volcaniclastic sandstones; 5 – mudrock; 6 – sandstone; 7 – slumping and debris flow bed. The sandstone petrofacies (A, B, C and D) are shown.
3. Sampling and methods
To obtain a representative suite of samples from the Tufiti di Tusa Formation, a set of 18 mudrocks (FP123-FP140) and 16 sandstones (T1-T16) were collected along the Canale Coppozzolo stratigraphic succession near Valsinni village (Fig. 1). Samples were collected from the bottom to the top of the studied succession (Fig. 3).
Mudrock samples were cleaned for geochemical and mineralogical analyses. Weathered coats and veined surfaces were removed. The rocks were crushed and milled in an agate mortar to a very fine powder.
3.a. X-ray diffraction (XRD) analyses
The powdered samples were placed in an ultrasonic bath for a few minutes for disaggregation; the < 2 μm grain-size fraction was then separated by settling in distilled water. The mineralogy of the whole-rock and of the fine fractions (< 2 μm) were determined by X-ray diffraction (XRD) using a Rigaku D/max 2200 diffractometer (Cu-Kα radiation; graphite secondary monochromator; sample spinner; step size, 0.02; speed, 3 sec steps). Oriented mounts were prepared, after Sr-saturation of the clay fraction, by evaporation of a clay-water suspension on the glass slides. XRD analyses were carried out on air-dried specimens, glycolated at 60°C for 8 hours, and heated at 375 °C for 1 hour (Moore & Reynolds, Reference Moore and Reynolds1997).
Quantitative mineralogical analysis of the bulk rock was carried out on random powders measuring peak areas for each mineral using the WINFIT computer program (Krumm, Reference Krumm1996). The strongest reflection of each mineral was considered, except for quartz for which the line at 4.26 Å was used instead of the peak at 3.34 Å because of its superimposition on that of the 10-Å minerals and of the I/S mixed layer series. The amount of phyllosilicates was estimated measuring the 4.5 Å peak area. The percentage of phyllosilicates in the bulk rock was split on the diffraction profile of the random powder, according to the following peak areas: 10–15 Å (I/S mixed layers), 10 Å (illite + micas) and 7 Å (kaolinite + chlorite) minerals (e.g. Cavalcante et al. Reference Cavalcante, Fiore, Lettino, Piccarreta and Tateo2007; Perri, Reference Perri2008). The amount of these latter phases was estimated by considering the height of the peaks at 3.58 Å and 3.53 Å, respectively. The percentage of clay minerals in the < 2 μm fraction was estimated by measuring the peak areas on diffraction profiles of the glycolated and heated oriented mounts and considering the height of the peaks at 3.58 Å and 3.53 Å on diffraction profiles of the heated oriented specimens (Laviano, Reference Laviano1987).
The percentage of illite (%I) and stacking order (Reichweite; R) of the I/S mixed layers was determined on the spectrum of the glycolated specimens according to Moore & Reynolds (Reference Moore and Reynolds1997). The 2θ peak position was determined by decomposition of X-ray pattern using asymmetric and symmetric function at low and high angles, respectively. Experimental X-ray profiles were compared with theoretical patterns calculated with the NEWMOD© computer program (Reynolds, Reference Reynolds1985).
3.b. Geochemical analyses
Elemental analyses for major and some trace element (Nb, Zr, Y, Sr, Rb, Ba, Ni, Co, Cr, V) concentrations were obtained by X-ray fluorescence spectrometry (Philips PW 1480) at the Università della Calabria (Italy), on pressed powder discs of whole-rock samples and compared to international standard rock analyses of the United States Geological Survey. X-ray counts were converted into concentrations by a computer program based on the matrix correction method according to Franzini, Leoni & Saitta (Reference Franzini, Leoni and Saitta1975) and Leoni & Saitta (Reference Leoni and Saitta1976). Loss on ignition (LOI) was determined after heating the samples for three hours at 900°C. Instrumental Neutron Activation Analysis (INAA) at the Activation Laboratories (Ancaster, Canada) was used to determine the abundance of the REEs and Sc, Zn, Cs, Th, Hf and U. Trace element concentration of the standard rocks is shown in Table 1. The estimated precision and accuracy for trace element determinations are better than 5%, except for those elements having a concentration of 10 ppm or less (10–15%).
Table 1. Trace element concentration of the standard rocks that were run together with the studied mudrocks, at the Activation Laboratories (Ancaster, Canada)

3.c. Petrography
Sixteen samples of unaltered medium-to-coarse sandstones were selected for thin-section analysis, covering the entire sedimentary succession. Thin-sections were prepared for these samples and were examined through a standard polarizing microscope. Thin-sections were etched and stained using hydrofluoric acid and sodium cobaltinitrite for plagioclase and potassium feldspar identification, respectively. The petrographic classes were recognized through quantitative petrographic point counting of the thin-sections, using the Gazzi–Dickinson method (Ingersoll et al. Reference Ingersoll, Bullard, Ford, Grimm, Pickle and Sares1984), and according to the petrographic classes defined by Zuffa (Reference Zuffa1980) (Table 2). The composition of the 16 sandstone samples is based on 245–582 points per thin-section at a spacing of 0.5 mm.
Table 2. Petrofacies of the studied sandstone samples

Qm – monocrystalline quartz; F – feldspars; Lt – aphanitic lithic fragments; Qt – total quartz; Qp – polycrystalline quartz; Lv – volcanic and metavolcanic lithic fragments; Lsm – sedimentary and metasedimentary lithic fragments; P – plagioclase; K – K-feldspar; L – lithic fragments; av – average; SD – standard deviation.
3.d. Scanning electron microscopy (SEM)
Samples of selected mudrocks and sandstones were gold coated and analysed using a SEM-EDX (ESEM Philips Electronics QUANTA 200F with EDX GENESIS 4000).
4. Petrography
Sandstones include dominantly volcanic (Fig. 4a–e) detritus, even if plutonic, metamorphic and extrabasinal carbonate detritus is also present (Fig. 4f, g). Volcanic detritus is mainly represented by single phenocrysts and lithic fragments. The metamorphic detrital grains are mainly high-grade metamorphic fragments of quartz and feldspar with biotite, amphibole and epidote. Subordinate low-grade metamorphic fragments are phyllites and schists (Fig. 4h). Phaneritic plutonic fragments are exclusively quartz and feldspar. Extrabasinal carbonates include biomicritic, biosparitic and sparitic limestone fragments (Fig. 4f, g). Intrabasinal carbonate particles include coeval plankton tests and intraclasts. The volcaniclastic interstitial component is mainly characterized by a non-carbonatic pseudomatrix (sensu Dickinson, Reference Dickinson1970) related to early diagenesis of volcanic detrital components.

Figure 4. Photomicrographs of sand grains of the Tufiti di Tusa sandstones. (a, b) Volcanic lithic fragments; (c–e) microlitic texture (volcanic lithic grains with plagioclase phenocrystals); (f, g) carbonate extrabasinal grains; (h) phyllite fragments.
Framework compositions of the studied sandstones can be subdivided into four distinctive petrofacies (sensu Dickinson, Reference Dickinson and Zuffa1985), marking the compositional evolution of the Tufiti di Tusa Formation (Table 2; Fig. 5).

Figure 5. Triangular plot (after Zuffa, Reference Zuffa1980; Ingersoll et al. Reference Ingersoll, Bullard, Ford, Grimm, Pickle and Sares1984; Dickinson, Reference Dickinson and Zuffa1985) of the sandstone samples from the Tufiti di Tusa Formation. From T1 to T16 – sandstone samples; A, B, C, D – petrofacies; Q – monocrystalline and polycrystalline quartz; F – feldspars; L – lithic fragments.
Petrofacies A (samples T1–T5) is quartzolithic in composition (Q46–F18–L36), with abundant medium- and high-grade metamorphic fragments (Table 2) where volcanic detritus is minor. This petrofacies represents the basal portions of the formation.
Petrofacies B (samples T6–T9) is lithofeldspathic volcaniclastic in composition (Q14–F15–L71) with P/F = 1 and a high Lv/L ratio (Table 2). Volcanic grains have dominantly microlitic and felsitic seriate textural attributes suggesting a provenance from andesitic volcanism. This petrofacies represents the middle portions of the formation.
Petrofacies C (samples T10–T13) is lithofeldspathic (Q17–F26–L57) with P/F = 1 and a high Lv/L ratio (Table 2), containing dominantly volcanic detritus. This petrofacies includes abundant microlitic and felsitic seriate volcanic textures, such as vitric volcanic textures and single euhedral amphibole and plagioclase crystals. This petrofacies represents the middle–upper portions of the formation.
Petrofacies D (samples T14–T16) is feldspatholithic (Q10–F52–L38) with P/F = 1 and a high Lv/L ratio (Table 2). This petrofacies includes abundant microlitic and felsitic seriate volcanic textures and single euhedral crystals of amphibole. Fine-grained strata of siltstone and mudrock interbedded with sandstone of this petrofacies consist exclusively of volcanic shards and pumice, and represent fine-grained ash turbidites. This petrofacies represents the upper portions of the formation.
The detrital modes of these arenites evolve from quartzolithic sandstones to lithofeldspathic and feldspatholithic sandstones.
The four petrofacies testify to an evolution from collisional orogen (petrofacies A) to volcanic arc (petrofacies B, C, D) (Fig. 5). The compositional evolution of the Tufiti di Tusa Formation is quite different to the general trend of circum-Pacific arc-trench systems related to volcanic-plutonic arc provenance (Dickinson, Reference Dickinson and Zuffa1985).
5. Mudrock mineralogy
5.a. Bulk rock composition
The results of whole-rock XRD analyses are reported in Table 3. Non-phyllosilicate minerals are represented by quartz, calcite and feldspars. Gypsum was detected in a few samples. Quartz has concentrations ranging from 13% to 41%. Feldspars are present in low abundances, which increases towards the top. This trend is quite similar to the above-mentioned evolution of the sandstone petrofacies. The amount of calcite is variable and shows an average value of 12.4%. The phyllosilicates are the main mineralogical components, ranging from 47% to 66% of the bulk rock; the highest values are present in the middle–low stratigraphic interval. The I/S mixed layers are the most abundant phyllosilicates, ranging from 14% to 52%. The contents of 10-Å minerals (illite and micas) and chlorite range from a few per cent to 25% and 18%, respectively. The kaolinite and chlorite/smectite (C/S) mixed layers are present as a few per cent or in trace amounts, and are not detected in some samples.
Table 3. Mineralogical composition of the bulk mudrock (wt%)

Qtz – quartz; Feld – feldspars (K-feldspars + plagioclase); Dol – dolomite; Calc – calcite; Σphy – sum of phyllosilicates; Gyp – gypsum; I/S – illite/smectite mixed layers; Ill – illite; C/S – chlorite/smectite mixed layers; Kao – kaolinite; Chl – chlorite; SD – standard deviation.
5.b. Clay mineralogy
The < 2 μm grain-size fraction (Table 4) is mainly composed of I/S mixed layers (73–96% in weight). Illite is present in variable amounts, from a few per cent up to 18%. Chlorite contents are usually low. Kaolinite and C/S mixed layers are present in very low or trace percentages in many samples (Table 4).
Table 4. Mineralogical features of the mudrock < 2 μm fraction

I/S – illite/smectite mixed layers; Ill – illite; C/S – chlorite/smectite mixed layers; Kao – kaolinite; Chl – chlorite; tr – trace; n.d. – not detected; R – Reichweite (stacking order of the illite/smectite (I/S) mixed layer).
As regarding the illite percentage in I/S mixed layers and the stacking order R, XRD patterns of the glycolated oriented slides show the occurrence of the ordered I/S R1 mixed layers in all samples, with illite percentage ranging from 65% to 85%, except for three samples collected from the top of the succession, which are characterized by I/S R3 with 85–90% illite. Some samples collected at the bottom of the succession are characterized by the presence of lower amounts of I/S R0 with 30–50% of illite associated with I/S R1.
6. SEM observations
The SEM images of selected mudrock samples show tiny glass shards that contain many vesicles similar to pumice. Some vesicles are oval and others are stretched into long, thin ‘capillary’ tubes (Fig. 6a–d). The smectite lattice, the mineral precursor to the (diagenetic) interstratified I/S identified in the studied samples, is commonly derived from the weathering of volcanic glass shards (e.g. Andreozzi, Dinelli & Tateo, Reference Andreozzi, Dinelli and Tateo1997; Fesneau et al. Reference Fesneau, Deconinck, Pellenard and Reboulet2009 and references therein). Thus, the abundance of smectite layers in the I/S mixed layers is probably related to the alteration of pyroclastic and/or volcaniclastic rocks. Diagenetic alteration of volcanic glass shards may characterize the source for the smectite and thus for interstratified I/S layers. Many studies have shown that the shard remnants are substituted by clay minerals and quartz, or rarely by calcite (Andreozzi et al. Reference Andreozzi, Dinelli and Tateo1997).

Figure 6. SEM (scanning electron microscope) images of mudrock samples. (a–d) Volcanic glass shards containing many vesicles. (e) Phyllosilicates and quartz grains. (f) Thin clay coating and microfossils.
7. Whole-rock geochemistry
7.a. Major element geochemistry
The elemental concentrations and some selected elemental ratios obtained from the studied mudrocks are given in Table 5. Figure 7 shows the major and trace elemental distributions normalized to Upper Continental Crust (UCC) averages (McLennan, Taylor & Hemming, Reference McLennan, Taylor, Hemming, Brown and Rushmer2006; diagram after Floyd, Winchester & Park, Reference Floyd, Winchester and Park1989).
Table 5. Major, trace element and ratios distribution in mudrock samples


Figure 7. Major and trace element compositions of Tufiti di Tusa mudrocks normalized to average Upper Continental Crust (UCC) (McLennan, Taylor & Hemming, Reference McLennan, Taylor, Hemming, Brown and Rushmer2006; diagram after Floyd, Winchester & Park, Reference Floyd, Winchester and Park1989). The composition of Post-Archaean Australian Shales (PAAS; Taylor & McLennan, Reference Taylor and McLennan1985) is shown for comparison.
The studied samples are characterized by narrow compositional changes for Al2O3, Fe2O3, and K2O, which have concentrations close to those of UCC (McLennan, Taylor & Hemming, Reference McLennan, Taylor, Hemming, Brown and Rushmer2006). MgO and TiO2 show a moderate enrichment (Fig. 7). MgO enrichment may be related to the 2:1 expandable clay minerals (e.g. smectite). CaO is the most enriched oxide compared to UCC, and shows highly variable concentrations ranging from 1.61 to 17.42 wt%. MnO is also variable, with concentrations ranging from 0.02 to 0.10 wt%. SiO2, P2O5 and Na2O are strongly depleted relative to UCC (Fig. 7). High variations in Na2O concentrations are related to plagioclase contents. These variations may reflect an andesitic volcanic arc input, as testified by the petrographical signature (e.g. abundant plagioclase in petrofacies B–C–D).
Most oxides (Al2O3, SiO2, Fe2O3, MgO, Na2O and K2O) are positively correlated whereas CaO is negatively correlated with the other oxides, reflecting variations in the carbonate component. Mobile and immobile element oxides (e.g. Al2O3, CaO, Na2O and K2O) have been used as a proxy for chemical weathering in sediment source areas, on the basis of the chemical index of alteration (CIA = 100*(Al2O3/(Al2O3 + CaO* + Na2O + K2O))) (Nesbitt & Young, Reference Nesbitt and Young1982). The CaO* values used are related exclusively to the silicate fraction (thus excluding the CaO related to the carbonate and sulphate minerals calculated via XRD). The Tufiti di Tusa mudrocks have CIA values ranging from 63.20 to 71.64 (average = 66.55 ± 2.89).
7.b. Trace element composition and rare earth elements
Strontium, like calcium, is enriched relative to UCC. Rubidium is also enriched and shows a significant correlation with K (r = 0.93) suggesting these elements are mostly controlled by the mica-like clay minerals.
High-field-strength trace elements (HFSE) have concentrations similar to those of UCC (Fig. 7). The lack of correlation between HFSE and Al2O3 is consistent with these elements being generally contained in accessory phases in mudrocks (e.g. Slack & Stevens, Reference Slack and Stevens1994). We infer that these elements occur in the sediment mostly as resistate phases, supporting the idea that they efficiently record the provenance (McLennan, Hemming & Hanson, 1993 and many others). Concentrations of the transition elements (Cr, Co, Ni and V) are generally higher than in UCC. All the transition metals appear to covary positively with Al2O3 and Fe2O3 suggesting these trace elements are mostly hosted by mica-like clay minerals.
Trace metals V, Mn, Cr, Zn and U can be used to trace an anoxic environment in which these elements are enriched (e.g. Bertolino, Zimmerman & Sattler, Reference Bertolino, Zimmermann and Sattler2007 and references therein). U is relatively unstable under oxidizing conditions but much more stable in anoxic environments (McLennan, Hemming & Hanson, Reference McLennan, Hemming and Hanson1993). Generally, high U/Th ratios point to an anoxic depositional environment where U can be enriched. The studied mudrocks have low U/Th ratios (average = 0.28 ± 0.05), similar to the PAAS (Post-Archaean Australian Shales) (0.21) and UCC (0.26) values, typical of oxidizing environments.
The REEs have been normalized to PAAS (Taylor & McLennan, Reference Taylor and McLennan1985); they display a pattern similar to the PAAS (Fig. 8). Most samples show a moderate depletion for both LREEs and HREEs, likely owing to a dilution effect exerted by the detrital carbonate component, which furnishes negligible REEs (e.g. Stevenson, Whittaker & Mountjoy, Reference Stevenson, Whittaker and Mountjoy2000), and a positive Eu anomaly possibly related to the feldspar content with respect to the PAAS standard, as also evident in mineralogical analyses.

Figure 8. Rare earth element compositions of Tufiti di Tusa mudrocks normalized to Post-Archean Australian Shales (PAAS) (Taylor & McLennan, Reference Taylor and McLennan1985).
8. Discussion
8.a. Provenance of the detrital material
The sandstone samples show abundant microlitic, felsitic seriate and vitric volcanic lithic fragments, with low-grade metamorphic fragments of phyllite, slate and quartzite, and intrabasinal and extrabasinal carbonate grains. The importance of the volcaniclastic supply is also testified by volcanic glass shards found in mudrocks. SEM images show tiny glass shards, consisting of many gas-bubble holes. The smectite lattice is commonly derived from the weathering of volcanic glass shards (Andreozzi, Dinelli & Tateo, Reference Andreozzi, Dinelli and Tateo1997; Fesneau et al. Reference Fesneau, Deconinck, Pellenard and Reboulet2009). Since the studied mudrocks contain abundant interstratified I/S, characterized also by R0 values with a high percentage of smectite in I/S mixed layers, the volcanic glass shards and, thus, the volcaniclastic input may play an important role in the sedimentary evolution of the studied succession.
The more immobile chemical elements, including Zr, Ti, Y and Nb, are generally used to determine the initial composition of the volcanic ashes and the type of magma (e.g. Winchester & Floyd, Reference Winchester and Floyd1977; Zimmermann & Spalletti, Reference Zimmermann and Spalletti2009). The compositional trend of sedimentary rocks can be estimated by considering ratios of Nb/Y and Zr/Ti, recently used for clastic sediments (e.g. Fralick, Reference Fralick and Lentz2003). In the Nb/Y versus Zr/Ti plot (Winchester & Floyd, Reference Winchester and Floyd1977), the mudrock samples point to a mainly andesitic and trachy-andesitic composition, close to the alkaline and the andesitic/basaltic trend, partly caused by the relative depletion of Zr in relation to UCC, which consequently lowers the Zr/Ti ratio (Fig. 9). However, high Nb concentrations shift the Nb/Y ratios to an alkaline composition. Basic source terranes would have high ferromagnesian mineral abundances, resulting in high Cr/V ratios (> 8) and low Y/Ni (< 0.5). The studied mudrocks are characterized by a lower Cr/V ratio suggesting a minor basic input. The minor importance of a mafic supply is confirmed also by the La–Th–Sc ternary plot (Fig. 10). The mudrocks fall in a region close to the PAAS point, between the granite and andesite fields (Fig. 10), indicating intermediate-felsic compositions of the source areas. However, based on trace element contents (see the transition element contents in Table 5), a mixed source, characterized by intermediate-felsic rocks with minor mafic input, cannot be excluded. Both Cr/Th and Th/Sc ratios are considered robust provenance indicators (Floyd & Leveridge, Reference Floyd and Leveridge1987; Taylor & McLennan, Reference Taylor and McLennan1985; McLennan, Hemming & Hanson, Reference McLennan, Hemming and Hanson1993). The diagram based on Cr/Th v. Th/Sc ratios (Fig. 11) shows that the mudrocks are consistent with mixing between felsic and mafic end-members, with a major contribution from an intermediate-felsic source; the mafic input is lower but not negligible.

Figure 9. Nb/Y v. Zr/Ti plot (after Winchester & Floyd, Reference Winchester and Floyd1977; Zimmermann & Spalletti, Reference Zimmermann and Spalletti2009) for the studied mudrocks, to reveal differences in the whole-rock composition of the studied mudrock samples.

Figure 10. La–Th–Sc plot (Bhatia & Crook, Reference Bhatia and Crook1986) for Tufiti di Tusa mudrocks. The mudrocks fall in a region close to PAAS that rules out important mafic supply.

Figure 11. Cr/Th v. Th/Sc plot (Condie & Wronkiewicz, Reference Condie and Wronkiewicz1990) for Tufiti di Tusa mudrocks. Two mixing curves have been shown between a felsic end-member and mafic and ultramafic end-members.
The relative abundances of trace elements such as La and Th (indicative of a felsic igneous source) and Co, Cr and Sc (indicative of mafic sources) and ratios such as La/Sc, Th/Sc, Th/Cr, Cr/Th and Eu/Eu* were used to differentiate the source of sediments (e.g. Cullers, Reference Cullers2000; Roser et al. Reference Roser, Coombs, Korsch and Campbell2002). These ratios allow assessment of aspects of bulk source composition because they are resistant to alteration processes and, apart from the effects of dense mineral concentration, they may be only slightly modified during transport (Roser et al. Reference Roser, Coombs, Korsch and Campbell2002). The studied sediments show ratios similar to the PAAS and UCC and generally fall in the range of similar published data (e.g. Cullers, Reference Cullers2000), suggesting provenance from intermediate-felsic sources (Table 6).
Table 6. Range of elemental ratios of mudrock samples compared to the ratios of felsic and mafic rocks, Upper Continental Crust and Post-Archaean Australian Shales

1Cullers (Reference Cullers2000); 2McLennan et al. (Reference McLennan, Taylor, Hemming, Brown and Rushmer2006); Taylor & McLennan (Reference Taylor and McLennan1985).
Starting from these considerations, based on the sandstone petrography and the mineralogical-geochemical data of the associated mudrocks, we suggest that the Tufiti di Tusa Formation contains clastic contributions from different sources: (a) a terrigenous (siliciclastic) source mainly from metasedimentary and magmatic sources (Calabrian Palaeozoic and related magmatites), with minor, but not negligible mafic rock supply; (b) a volcaniclastic source from subduction-related andesites, probably located in Sardinia; and (c) a calciclastic source, probably derived from the Apenninic forebulge.
8.b. Diagenetic grade
There is a broad consensus in the literature about the hypothesis that smectite-to-illite conversion is a progressive trend mainly controlled by temperature (e.g. Merriman & Peacor, Reference Merriman, Peacor, Frey and Robinson1999). Furthermore, this process may be influenced by time, and K-availability, whose origin has been assigned to feldspars and/or mica (e.g. Moore & Reynolds, Reference Moore and Reynolds1997; Cavalcante et al. Reference Cavalcante, Fiore, Lettino, Piccarreta and Tateo2007).
The abundance of I–S R1 mixed layers in the studied samples suggests that the Tufiti di Tusa Formation was affected by medium diagenetic grade (Merriman & Peacor, Reference Merriman, Peacor, Frey and Robinson1999 and references therein). These conditions are equivalent to those found in Sicilide successions located to the northwest of the studied area (Cavalcante et al. Reference Cavalcante, Fiore, Lettino, Piccarreta and Tateo2007).
The upper part of the studied succession is characterized by samples showing I/S R3 with higher illite amounts and a higher concentration of feldspars and K2O (Tables 3, 5). This suggests that the illitization of smectite is influenced by K-availability, as most models have proposed to explain the smectite-to-illite transformation (e.g. Moore & Reynolds, Reference Moore and Reynolds1997; Cavalcante et al. Reference Cavalcante, Fiore, Lettino, Piccarreta and Tateo2007).
8.c. Source-area weathering, recycling and sorting
Various indices of weathering have been proposed based on different molecular proportions of mobile element oxides (Na2O, CaO, MgO, K2O) relative to immobile element oxides, Al2O3, ZrO, and Ti2O. The CIA has been widely used as a proxy for chemical weathering in the sediment source area. The Al2O3, Na2O and K2O values, and the CaO* belonging exclusively to the silicate fraction, have been plotted in the A–CN–K triangular diagram (Fig. 12). The Tufiti di Tusa mudrocks form a tight group above the A50 line (the feldspar join; Fedo, Nesbitt & Young, Reference Fedo, Nesbitt and Young1995) with a vector parallel to the CN–A join and a vector parallel on the A–K join, close to the illite–muscovite point (Fig. 12). Illite and other illitic minerals (I/S mixed layers) are the dominant clay minerals that characterize the studied samples. The degree of weathering is variable within the individual suites. The variations of CIA values along the stratigraphic section are related to the mineralogical variations. The samples with higher CIA values (lower part of the succession) are characterized by abundant phyllosilicates and minor content of feldspars, whereas the samples with lower CIA values show lower percentages of phyllosilicates and higher contents of feldspars. This feature emphasizes the coherent nature of the mineralogical transformation (from primary feldspars to secondary clay minerals) that are clearly related to the CIA value distribution.

Figure 12. A–CN–K diagram (in molecular proportions) for mudrock samples (Nesbitt & Young, Reference Nesbitt and Young1982). Note only the top 60% of the triangle is shown. Legend: A – Al2O3; C – CaO; N – Na2O; K – K2O; Gr – granite; Ms – muscovite; Il – illite; Ka – kaolinite; Ch – chlorite; Gi – gibbsite; Sm – smectite; Bi – biotite; Ks – K-feldspar; Pl – plagioclase.
Likewise, the degree of chemical weathering can be assessed on the basis of K/Cs ratios in fine-grained sedimentary rocks (e.g. McLennan, Hemming & Hanson, Reference McLennan, Hemming and Hanson1993). Caesium is enriched in clay minerals during weathering, which leads to a decrease in K/Cs ratios of the rocks with increasing weathering. Correlation between K and Cs (r = 0.85) is strongly indicative of weathering affecting K-bearing minerals (e.g. Feng & Kerrich, Reference Feng and Kerrich1990). The studied samples show K/Cs values ranging from 2200 to 3400 (average = 2800), which are similar to the PAAS value (2100), testifying to a moderate weathering in the source area. At the same time, source area weathering can be evaluated by clayeyness (Al/Si ratio), which increases owing to clay production during pedogenesis (e.g. Mitchell & Sheldon, Reference Mitchell and Sheldon2009 and references therein). The Tufiti di Tusa sediments show an average Al/Si ratio (0.34 ± 0.03) close to the PAAS and UCC averages (respectively, 0.34 and 0.26; Taylor & McLennan, Reference Taylor and McLennan1985; McLennan, Taylor & Hemming, Reference McLennan, Taylor, Hemming, Brown and Rushmer2006). This suggests that the degree of source-area weathering was most probably moderate rather than intense.
One approach to estimate the amount of primary source relative to weathered minerals in shales and siltstones is to use the index of compositional variability (ICV = (Fe2O3 + K2O + Na2O + CaO + MgO + TiO2)/Al2O3) (Cox & Lowe, Reference Cox and Lowe1995); in this equation, CaO includes also the Ca-sources related to detrital carbonate and phosphate (e.g. LaMaskin, Dorsey & Vervoort, Reference LaMaskin, Dorsey and Vervoort2008). The ICV values tend to be highest for detrital ferromagnesian minerals and feldspars and lowest for minerals that form during weathering (clay minerals). Thus, the ICV values for siltstones and shales with abundant relatively unweathered detrital minerals should be greater than one. In contrast, siltstones and shales composed of abundant clay minerals have an ICV less than one. Most terrigenous sedimentary rocks with ICV values greater than one tend to occur in first-cycle deposits, whereas sedimentary rocks with ICV values less than one usually form where intense weathering and minimal tectonic uplift have occurred (Cox & Lowe, Reference Cox and Lowe1995). Some first-cycle shales and siltstones formed during intense chemical weathering may form, however, abundant clay minerals and thus contain ICV values less than one (Johnsson, Reference Johnsson2000). The ICV values of the studied samples are more than one (average ICV = 1.6) and, consequently, they indicate input of first-cycle sediments without important recycling processes.
Generally, mature sediments consisting of both sandstones and shales show a wide range of TiO2/Zr variations whereas immature sediments show a more limited range of TiO2/Zr variations in the Al–Ti–Zr ternary diagram (Garcia et al. Reference Garcia, Fonteilles and Moutte1994). The studied sediments are confined to the centre of this diagram, with a limited range of TiO2/Zr variations, suggesting poor sorting and rapid deposition of the sediments (Fig. 13).
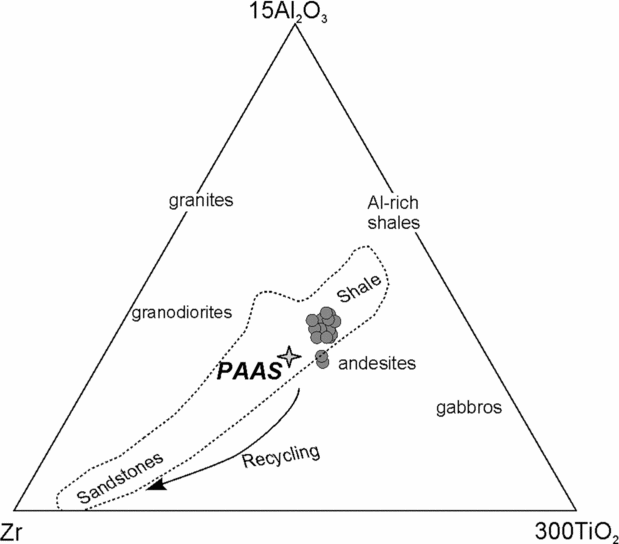
Figure 13. Ternary 15Al2O3–300TiO2–Zr plot (after Garcia, Fonteilles & Moutte, Reference Garcia, Fonteilles and Moutte1994) for Tufiti di Tusa mudrocks, showing poor sorting and rapid deposition of the sediments.
The sedimentary sorting and recycling can be further monitored by a plot of Th/Sc against Zr/Sc (McLennan, Hemming & Hanson, Reference McLennan, Hemming and Hanson1993); as the studied mudrocks are not recycled sedimentary rocks, the samples plot along the simple positive correlation between these ratios (i.e. Th/Sc v. Zr/Sc). The studied sediments follow a general trend consistent with their direct derivation from igneous rocks in the Th/Sc v. Zr/Sc diagram (Fig. 14). This consideration is in agreement with the ICV values and the Al–Ti–Zr diagram constraints.

Figure 14. Th/Sc v. Zr/Sc plot (McLennan, Hemming & Hanson, Reference McLennan, Hemming and Hanson1993) for Tufiti di Tusa mudrocks. Studied mudrocks fall close to the andesite composition.
Commonly, recycling processes are related to the amount of feldspars in the sediments; the paucity or absence of feldspars in the rocks may record a recycling effect (Mongelli et al. Reference Mongelli, Critelli, Perri, Sonnino and Perrone2006; Perri et al. Reference Perri, Cirrincione, Critelli, Mazzoleni and Pappalardo2008, Reference Perri, Critelli, Mongelli and Cullers2010; Zaghloul et al. Reference Zaghloul, Critelli, Perri, Mongelli, Perrone, Sonnino, Tucker, Aiello and Ventimiglia2010). Many of the studied samples are characterized by significant amounts of plagioclase feldspars; this testifies to poor recycling effect for the studied sediments. Plagioclases are abundant in sandstone; they are fresh and dominantly derived from active volcanic source areas, testifying to poor recycling and low–moderate weathering.
8.d. Geodynamic and palaeogeographic significance
The compositional variations recorded in the studied succession, as shown above, are related to palaeogeographic and geodynamic change during early Miocene time (Fig. 15).

Figure 15. Palaeogeographic reconstructions (early Miocene) of the western Mediterranean during progressive closure of the remnant ocean basin and onset of collision in the southern Apennines (modified from Critelli, Reference Critelli1999).
In the southern Italy domain, the Sicilide Complex strata represent deposition in a remnant ocean basin related to the western subduction of the Adria oceanic lithosphere beneath the Iberia plate (e.g. Guerrera, Martín-Algarra & Perrone, Reference Guerrera, Martín-Algarra and Perrone1993; Critelli & Le Pera, Reference Critelli and Le Pera1998; Critelli, Reference Critelli1999). The subduction was active for all the Palaeogene and early Miocene, producing an accretionary prism, the Sicilide Complex and calcalkaline volcanism in Sardinia. The Mesoalpine (Eocene to early Oligocene) tectonic phase in southern Italy corresponds to the subduction of the Adria–Ionian oceanic lithosphere beneath the Iberian plate. During early to middle Miocene time, the Apenninic domain is the place where an immense volume of turbiditic sedimentation is a response to E–NE accretionary processes along the Adria plate (e.g. Patacca & Scandone, Reference Patacca, Scandone, Mazzotti, Patacca and Scandone2007 and references therein).
The Tufiti di Tusa Formation, representing the first foredeep deposit within the southern Apennine orogenic belt, records interbedded provenances from the (i) Fold–Thrust Belt (quartzolithic petrofacies), where metamorphic, plutonic and extrabasinal carbonate detritus are derived from the internal units of the growing orogen, that in the southern Italy orogenic system correspond to the Calabria–Peloritani Terrane and the Longobucco Unit (Fig. 15), (ii) Forebulge Region (carbonatoclastic strata), where carbonate turbidites interbedded with siliciclastic turbidites are derived from the flexed forebulge depozones of the Adria margin (Fig. 15), and (iii) the active volcanic arc from Sardinia (volcaniclastic petrofacies). Huge quartzose supply (Numidian sandstones; Fig. 15), during the early stage of collision, is widespread along the Adria margin, representing key signatures from the cratonic provenance from the northern African continental margin (Fig. 15) that are partially coeval with deposition of the Tufiti di Tusa Formation, and accommodated in subsiding depozones of the Adria margin (e.g. Critelli, Reference Critelli1999 and references therein).
9. Conclusions
The studied succession from the Tufiti di Tusa Formation (Sicilide Complex, southern Italy) is characterized by an evolution from calcilcastic–siliciclastic sediments to mostly volcaniclastic sediments; both mudrock mineralogy and sandstone petrography show this trend. The lower part of the succession is characterized by sandstone quartzolithic beds (petrofacies A) and carbonate-rich mudrocks, whereas the mudrock and, mostly, the sandstone samples collected along the upper part show an increase in feldspar content (petrofacies D) related to volcanic arc provenance.
The mudrocks studied are mineralogically characterized by phyllosilicates, quartz, calcite and feldspars, with a small amount of gypsum detected in some samples. I/S mixed layers are the main phases among the phyllosilicates, followed by 10-Å minerals (illite and micas) and chlorite, while kaolinite and C/S mixed layers are present in a few per cent or trace amounts. The abundance of I/S R1 and R3 mixed layers suggest that the Tufiti di Tusa Formation has been affected by medium diagenetic grade. The upper part of the succession shows stronger illitization. This suggests an influence of K-availability on the smectite illitization.
The source-area weathering has been evaluated by the CIA index. The studied mudrocks are characterized by CIA values in the range 65–72, testifying to moderate weathering at the source areas. Furthermore, low K/Cs ratios of the studied sediments suggest that the weathering degree of the source area was moderate rather than intense. In general, warm climates favour an intense source-area weathering, and the formation of residual clays like kaolinite. The studied mudrocks are characterized by low kaolinite, which is only present in a few samples. Thus, weathering occurred under warm and episodically wet climates with a dry season (typical Mediterranean climate) that promoted rapid sedimentation without strong sedimentary recycling (e.g. Mongelli et al. Reference Mongelli, Critelli, Perri, Sonnino and Perrone2006 and references therein). The high ICV values of the studied samples suggest that they are related to first-cycle material and not dominated by recycling. The distribution of the studied mudrocks in the Al–Ti–Zr ternary diagram and in the Th/Sc v. Zr/Sc plot also suggests poor sorting and rapid deposition of the sediments. The sandstone petrology confirms the poor sorting without significant recycling processes as shown by detrital grain features.
The source areas of the studied sediments are mainly characterized by metasediments (low-to-high grade metamorphic rocks) of the Palaeozoic basement with andesitic to felsic volcanic bodies. The limited importance of a mafic supply is confirmed by the Cr/V and Y/Ni ratios, by the K2O v. Rb plot and by the La/Sc, Th/Sc, Th/Co, Th/Cr, Cr/Th and Eu/Eu* ratios, that suggest a provenance from source areas fairly intermediate–felsic rather than mafic in composition.
Direct evidence of volcaniclastic supply is shown by volcanic glass shards found in thin mudrock levels, and the abundance of smectite layers in the I/S mixed layers are probably related to the diagenetic alteration of the weathered original pyroclastic and/or volcaniclastic rocks, which were the source for the smectite, and thus for the interstratified I/S layers.
A large amount of volcanic detritus rapidly filled the basin; the volcaniclastic sedimentation gave rise either to fine-grained, small pure beds or, more frequently, to thick arenitic impure beds. According to the palaeogeographic and geodynamic settings, the volcanic ash-falls may originate at and be derived from the subduction-related orogenic volcanism of Sardinia, characterized by andesitic–rhyolitc volcanic products during Oligocene–Miocene time.
Acknowledgements
This research has been carried out within the MIUR-ex60% Projects (Palaeogeographic and Palaeotectonic Evolution of the Circum-Mediterranean Orogenic Belts, 2001–2005; and Relationships between Tectonic Accretion, Volcanism and Clastic Sedimentation within the Circum-Mediterranean Orogenic Belts, 2006; Resp. S. Critelli) and the 2006–2008 MIUR-PRIN Project 2006.04.8397 (‘The Cenozoic clastic sedimentation within the circum-Mediterranean orogenic belts: implications for palaeogeographic and palaeotectonic evolution’; Resp. S. Critelli). The authors are indebted to Agustín Martín-Algarra, two anonymous referees and the editor Phil Leat for their reviews and suggestions on an earlier version of the manuscript.