Introduction
Cucurbits (Cucurbitaceae) are economically valuable and an important food crop for most of the world's population. Squashes, pumpkins, cucumbers and melons are in the top 20 most important food crops globally (Sebastian et al., Reference Sebastian, Schaefer, Telford and Renner2010). In the USA, cucurbit crops account for over US$1.43 billion of the vegetable industry, and 11.8% of total vegetable growing acreage (Cantliffe et al., Reference Cantliffe, Shaw and Stoffella2007). US Department of Agriculture Census data show that there has been an increase in cucurbit intensification in the USA over the past decade (United States Agriculture Census, Reference Vilsack and Clark2014). Fewer acres are being dedicated to cucurbit production, but more farmers are choosing to grow cucurbit crops and total production has increased (Cantliffe et al., Reference Cantliffe, Shaw and Stoffella2007; United States Agriculture Census, Reference Vilsack and Clark2014). This increase of intensification is largely based on the advancement in farming technologies and plant breeding over the past 20-years (Gusmini and Wehner, Reference Gusmini and Wehner2008).
Organic production is one of the fastest growing sectors of agriculture globally, and production is valued at US$43.3 billion yearly in the USA (OrganicTradeAssociation, 2016). Improvements in organic production over the past decades have lowered the yield gap between conventional and organic production, with organic systems producing 19.2% on average less than conventional systems (Ponisio et al., Reference Ponisio, Gonigle, Mace, Palomino, De Valpine and Kremen2014). Some of this yield loss is compensated for by well documented environmental benefits such as improved soil microbial activity and fertility, increased biodiversity and reduction of greenhouse gasses (Mäder et al., Reference Mäder, Fliessbach, Dubois, Gunst, Fried and Niggli2002; Hole et al., Reference Hole, Perkins, Wilson, Alexander, Grice and Evans2005; Garratt et al., Reference Garratt, Wright and Leather2011; Goh, Reference Goh2011); and by a premium price for organically grown products (Yiridoe et al., Reference Yiridoe, Bonti-Ankomah and Martin2005; Bonti-Ankomah and Yiridoe, Reference Bonti-Ankomah and Yiridoe2006; Batte et al., Reference Batte, Hooker, Haab and Beaverson2007). With the rise of organic agriculture, there has been an increasing demand for organic cucurbit production. It has been reported that in a well-managed system, organically produced cucurbits can have comparable yields with conventional (Nair and Ngouajio, Reference Nair and Ngouajio2010), but further research is needed to substantiate these results under diverse growing practices.
Cucurbit management plans have begun to focus on developing and exploring the incorporation of integrated pest management (IPM) techniques. The goal of IPM is to promote the use of sustainable and environmentally friendly production techniques that reduce the use of chemical-based pesticides (Kogan, Reference Kogan1998). Many IPM techniques have been developed for cucurbit production. These techniques include changes in cultivation methods, the use of non-chemical pest barriers (row covers, trap crops, pheromone trapping), altering of mechanical strategies, genetic modification, organic soil amendments, mulches, crop rotation plans and the use of biological control (Orozco-Santos et al., Reference Orozco-Santos, Perez-Zamora and Lopez-Arriaga1995; Keinath, Reference Keinath1996; Olson et al., Reference Olson, Nechols and Schuhle1996; Lewis et al., Reference Lewis, Van Lenteren, Phatak and Tumlinson1997; Pair, Reference Pair1997; Zehnder et al., Reference Zehnder, Gurr, Kühne, Wade, Wratten and Wyss2007; Cavanagh et al., Reference Cavanagh, Hazzard, Adler and Boucher2009; Waterfield and Zilberman, Reference Waterfield and Zilberman2012; Rojas et al., Reference Rojas, Batzer, Beattie, Fleischer, Shapiro, Williams, Bessin, Bruton, Boucher and Jesse2015; Leftwich et al., Reference Leftwich, Bolton and Chapman2016; Phillips and Gardiner, Reference Phillips and Gardiner2016). Organic agriculture is limited to IPM methods that meet organic standards. Currently, there are few certified organic pesticides on the market and they often have limited effectiveness in controlling key cucurbit pests (Cline et al., Reference Cline, Sedlacek, Hillman, Parker and Silvernail2008; Pedersen and Godfrey, Reference Pedersen and Godfrey2011; Tillman et al., Reference Tillman, Nair, Gleason and Batzer2015b), therefore non-chemical-based strategies may be preferable and needed for this system.
Tillage regime has been used as a management strategy for many production systems, and can have a major impact on the agroecosystem and crop yield. (Sheibani and Ahangar, Reference Sheibani and Ahangar2013). Many soil-forming factors are affected by tillage type and frequency. Intensive tillage can directly cause soil compaction, soil erosion and reduction in soil organic matter content, which can reduce plant productivity and alter the soil community (Batey, Reference Batey2009). It can dramatically affect the entire agroecosystem and have both on-farm and global impacts (Pimentel and Kounang, Reference Pimentel and Kounang1998). A poorly managed system can lead to overworking the field, causing negative impacts on the physical and chemical soil structure and arthropod and soil microorganism biodiversity (Wolters, Reference Wolters2000; Van Oost et al., Reference Van Oost, Govers, De Alba and Quine2006; Batey, Reference Batey2009; Sheibani and Ahangar, Reference Sheibani and Ahangar2013).
Cucurbit production systems use a variety of tillage systems, but two popular systems are conservation tillage and plasticulture. Conservation tillage systems can reduce soil disturbance, pest pressure and cause less of an impact on biodiversity than more rigorous tillage regimes (Stinner and House, Reference Stinner and House1990; Lupwayi et al., Reference Lupwayi, Rice and Clayton1998; Kladivko, Reference Kladivko2001; Aziz et al., Reference Aziz, Mahmood and Islam2013; Sheibani and Ahangar, Reference Sheibani and Ahangar2013). Plasticulture can create greater soil disturbance, but it results in earlier fruit harvest (Tarara, Reference Tarara2000; Lamont, Reference Lamont2005). The two systems can have comparable yields when growing conditions are similar (NeSmith et al., Reference Nesmith, Hoogenboom and Mccracken1994; Tillman et al., Reference Tillman, Nair, Gleason and Batzer2015b), but in years with cooler temperatures conservation tillage systems have slower plant growth and decreased yield (NeSmith et al., Reference Nesmith, Hoogenboom and Mccracken1994).
The addition of early-season row cover is a common IPM practice used in many cucurbit production systems (Nair and Ngouajio, Reference Nair and Ngouajio2010). This system involves the use of spunbonded polyethylene sheets that are secured on the edge and placed over the crop until pollination is needed (Loy and Wells, Reference Loy and Wells1975; Perring et al., Reference Perring, Royalty and Farrar1989; Jensen and Malter, Reference Jensen and Malter1995). This creates a greenhouse effect, which alters the microclimate around the plants, greatly increasing plant quality, yield and development (Loy and Wells, Reference Loy and Wells1975; Soltani et al., Reference Soltani, Anderson and Hamson1995; Arancibia and Motsenbocker, Reference Arancibia and Motsenbocker2008; Nair and Ngouajio, Reference Nair and Ngouajio2010). The use of row cover has also been shown to reduce the number of pests and disease in both squash and melon systems (Webb and Linda, Reference Webb and Linda1992; Orozco-Santos et al., Reference Orozco-Santos, Perez-Zamora and Lopez-Arriaga1995), through mechanical exclusion. By reducing pest numbers on the crop, row covers reduce the number of insecticide applications needed; helping to decrease pest resistance, non-target effects on beneficial insects, pesticide residues in food and chemical run off (Condron et al., Reference Condron, Cameron, Di, Clough, Forbes, Mclaren and Silva2000).
There are three major pests that can decimate cucurbit yields through vectoring plant diseases and by causing feeding damage: squash bug (Anasa tristis [Hemiptera: Coreidae]) (SB), spotted cucumber beetle (Diabrotica undecimpunctata howardi [Coleoptera: Chrysomelidae]) (SPCB), and striped cucumber beetle (Acalymma vittatum [Coleoptera Chrysomelidae]) (STCB). SB can vector yellow vine decline (Serratia marcescens), and both STCB and SPCB can vector bacterial wilt (Erwinia tracheiphila). These diseases can devastate cucurbit crops (Margolies et al., Reference Margolies, Nechols and Vogt1998; Bruton et al., Reference Bruton, Mitchell, Fletcher, Pair, Wayadande, Melcher, Brady, Bextine and Popham2003; Cranshaw, Reference Cranshaw2004; Ellers-Kirk and Fleischer, Reference Ellers-Kirk and Fleischer2006; Schmidt et al., Reference Schmidt, Barney, Williams, Bessin, Coolong and Harwood2014). Damage and the cost of control for STCB and SPCB costs producers US$100 million a year in the USA (Schroder et al., Reference Schroder, Martin and Athanas2001). Chemical insecticides used to control these pests can have varying results, negatively impact non-target arthropods (including natural enemies and pollinators), and have the potential to create pest resistance (Margolies et al., Reference Margolies, Nechols and Vogt1998; Stark and Banks, Reference Stark and Banks2003; Desneux et al., Reference Desneux, Decourtye and Delpuech2006). Additionally, organically approved insecticides have little residual activity and may be much less effective than those that are synthetically derived (Pedersen and Godfrey, Reference Pedersen and Godfrey2011).
Our study focuses on several IPM techniques to develop more productive growing systems and reduce pest pressure in organic cucurbit systems. Our 2-year study looked at the organic production of summer squash (Cucurbita pepo) and cantaloupe melon (Cucumis melo), and the impact of tillage regime and row cover on the arthropod pests found in each system. Additionally, we compared the yields of each system with determine potential economic value to growers.
Materials and methods
Study site
Experiments were conducted in 2013 and 2014 at the University of Kentucky Horticulture Research Farm, located in Lexington, Kentucky (latitude: 37°58′25.92″N, longitude: 84°32′5.85″W). In both years of the study 0.135 hectare field sites located in the organic transition section of the farm were used, with a minimum of 3 m grass buffer separating them from adjoining fields.
Experimental design
A randomized complete split-block design was used in both 2013 and 2014. Each block was divided into two main plots (16.5 m × 7.5 m) using conventional tillage with raised beds and plasticulture as one treatment, and strip tillage as our other main plot treatment (Fig. 1). Each mainplot consisted of four rows (12 m) on 1.8 m centers, with 20 transplants per row. The two center rows of each main plot were used as subplot treatment rows, one row remaining uncovered and the other covered with spunbonded row cover (Agribon grade-20, Berry Plastics, Indiana, USA) at the time of transplanting. The two outer rows were uncovered buffer rows and were treated the same as the uncovered treatment rows, though no data were collected from these rows. Row cover treatments were randomly assigned to treatment rows and removed at the time of female flower anthesis to facilitate pollination.

Fig. 1. Example of experimental plot design used in organically managed summer squash and melon studies at the University of Kentucky Horticulture Research Farm, Lexington, Kentucky, 2013 and 2014.
Field preparation
All inputs were certified for organic use and experiments were conducted under organic management practices (Table 1). In the fall of 2012 and 2013 the experimental plots were planted with a cover crop mix of rye (66.7 kg ha−1), Austrian winter pea (56.8 kg ha−1) and tillage radish (17.3 kg ha−1). Organic compost (Woodford County University of Kentucky Farm, Kentucky, USA) was applied at a rate of 2224 kg ha−1 before flail mowing the cover crop in the spring prior to anthesis. In 2013, a walk behind tractor (BCS America Inc., Oregon, USA) with a strip-tiller attachment was used to till a 20 cm wide strip 15 cm deep, and the remaining cover crop residue was left in the field to act as a ground cover in the undisturbed areas. In 2014, a strip tiller (Hiniker Company Inc., Minnesota, USA) was used in the strip till rows. In the plasticulture subplots, a disk tiller was used to incorporate the cover crop residue. Post-tillage, pre-plant organic fertilizer (Nature Safe 10-2-8, Kentucky, USA) was spread at a rate of 140.9 kg N ha−1. In the plasticulture system, black plastic mulch raised bed (91 cm wide, 15 cm high) rows were constructed. Irrigation was provided to each row via a centrally placed drip line. Drip tape was placed under plastic mulch at the time of bed formation. For strip till rows, drip tape was placed on the soil surface and secured with sod stapes. Mechanical cultivation was used to control weeds in-between the rows in the plasticulture regime, and hand weeding was used in the strip till regime.
Table 1. Timeline of major field operations for summer squash and muskmelon studies in 2013 and 2014 at University of Kentucky Horticulture Research Farm, Lexington, Kentucky.
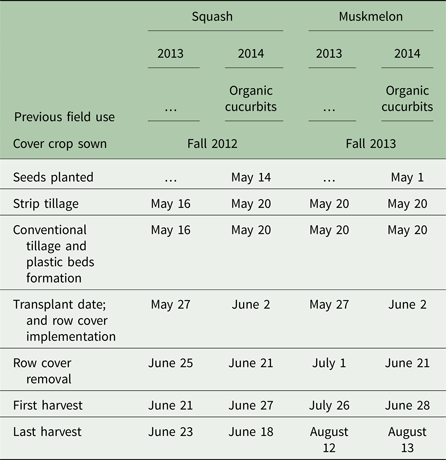
Summer squash and cantaloupe transplants were 5-weeks (2013) and 3-weeks-old (2014) when planted. A mechanical planter (Rain-Flo Irrigation LLC., Pennsylvania, USA) was used to set the plants in the field, and 20 plants were placed in each row with 61 cm in-row spacing. Row covers were implemented at the time of planting. In 2014, melons were fertigated (Phytamin 4-3-4, California Organic Fertilizers Inc. California, USA) at a rate of 17.3 kg N ha−1 using a homemade pressurized tank injector attached to the header line.
In both 2013 and 2014 insecticides approved for use by the Organic Materials Review Institute (OMRI) were used when pest density was greater than one cucumber beetle per plant, with 10 plants scouted. The insectides were: Entrust SC, Dow AgroSciences, Indiana, USA; PyGanic, Valent, Tokyo, Japan; Surround, NovaSource, Arizona, USA; Trilogy, Certis, Maryland, USA). OMRI approved fungicides (Nordox 75 WG, Nordox, Oslo, Norway; Trilogy, Certis, Maryland, USA) were applied as needed.
Data collection
Harvest data
Harvest data were collected for each treatment row throughout the growing season. Fruit was graded for marketability and insect damage, and both total weight and fruit number were recorded. In 2013, squash was harvested over a 6-week period, and melons were harvested over a 4-week period. In 2014, squash was harvested over a 4-week period, and melon was harvested over a 3-week period. The difference in harvesting time was based on cropping system and variations between the two growing seasons.
Insect pest data
Recording insect pest presence and abundance in each system was a main focus in this study. Two data collection methods were used to record the number of insect pests found in each treatment row. Visual surveys were conducted weekly in both 2013 (squash: June 5–July 16; melon: June 5–August 7) and 2014 (squash: June 16–July 14; melon: June 16–August 7); where three, 0.6 m2 quadrats were observed in each treatment row for a 60-s sampling period. All insect pests found on foliage or at ground level were recorded. In rows where row cover was used, sampling started after row cover removal. Pitfall traps were randomly placed in each treatment row weekly in 2013 (squash: June 11–July 23; melon: June 11–August 12) and bi-weekly 2014 (squash: June 23–July 21; melon: June 23–August 6); with one pitfall trap placed in each treatment row and filled with non-toxic antifreeze. A 473.2 ml solo cup (Dart Container Cooperation, Michigan, USA) was used in 2013, but the trap size was increased to a 946.4oz cup in 2014. Traps remained in the field for a 7-day period, at which point the contents were rinsed with water and stored in 70% ethanol for identification.
Pest numbers were calculated based on pitfall traps and observational survey data. Pest numbers were compared in both the squash and melon studies to determine which management strategies had the greatest impact on pest numbers. Differences between the growing seasons, such as weather and field conditions, were also recorded although these were factors outside of the control of the experiment.
Data analysis
Data were subject to analysis of variance using Proc GLM (SAS version 9.4, SAS Institute Inc., New York, USA). Total yield was compared across tillage regime and row cover treatment to determine which growing system and treatment had the greatest yield. Each study was analyzed for marketable and unmarketable (culled) yield in both years of the study. Each crop was analyzed individually, no comparisons were made between the squash and melon studies. Each crop should be viewed as a single study where only tillage regime and row cover treatment were compared within each system. Pests observed in surveys and collected in pitfall traps were combined for each treatment and weekly averages calculated.
Results and Discussion
Weather
Average monthly temperatures in both years of the study were lower than the 30-year average, except for the months of May and June (Table 2). Additionally, April of 2014 was cooler than 2013 and the 30-year average. Spring precipitation in both years of the study was higher than the 30-year average (Table 2), causing difficulty for field preparation. Nearly twice the average monthly rainfall was recorded in July 2013 and August 2013 and 2014, creating saturated field conditions and soil compaction, which contributed to yield loss.
Table 2. Monthly air temperature (°C) and total precipitation (cm) in 2013 and 2014 and 30-year monthly average for Fayette County, Kentucky.

a Monthly data acquired from Kentucky Mesonet, at the Fayette County, Kentucky, location located at the University of Kentucky Horticulture Research Farm.
b NOAA National Center for Environmental Information monthly summaries used to calculate a 30-year average from 1985–2014.
Yield analysis
In each study, total yields were compared across tillage regime and row cover treatment to determine which growing system and treatment had the greatest yield. Each study was analyzed for marketable and unmarketable (culled) yield in both years of the study. Marketable yield represents the fruit that would be of marketable quality, culled fruit represents fruit that a grower would not be able to sell, and insect related culls (IRC) are a subset of culled fruit that are considered culls because of insect feeding damage to the fruit. Additionally, both fruit weight and number are reported, since many producers sell to wholesale markets by weight and direct markets per individual fruit.
Squash study
In both study years, plasticulture and row cover increased marketable yield (Table 3, Figs. 2A, B) and IRC (Table 3, Figs. 2C, D). In 2013, an interaction between tillage regime and row cover was observed; where plasticulture with row cover had the greatest marketable yield weight (F = 7.66; d.f. 1, 6; P ≤ 0.05) and number (F = 9.17; d.f. 1, 6; P ≤ 0.05) and the greatest IRC weight (F = 39.02; d.f. 1, 6; P ≤ 0.01) and number (F = 5.38; d.f. 1, 6; P ≤ 0.05). In 2014, no interaction between tillage and row cover was observed, but plasticulture had significantly greater marketable yield weight (F = 51.28; d.f. 1, 6; P ≤ 0.001) and number (F = 59.54; d.f. 1, 6; P ≤ 0.001) and greater IRC weight (F = 24.47; d.f. 1, 6; P ≤ 0.01) and fruit number (F = 26.88; d.f. 1, 6; P ≤ 0.01) than strip tillage. When yield was compared between 2013 and 2014, there was a significant interaction between row cover use and tillage regime for marketable fruit weight (F = 5.56; d.f. 1, 12; P ≤ 0.05) and number (F = 6.81; d.f. 1, 12; P ≤ 0.05).

Fig. 2. Total marketable yield (A, B) weight and culls due to insect damage weight (C, D) of summer squash harvested from organically managed summer squash study at the University of Kentucky Horticulture Research Farm, Lexington, Kentucky, 2013 and 2014.
Table 3. Mean marketable yield, unmarketable yield (culls), and culls related to insect damage (IRC) from organically managed summer squash and muskmelon studies at the University of Kentucky Horticulture Research Farm, Lexington, Kentucky, 2013 and 2014.
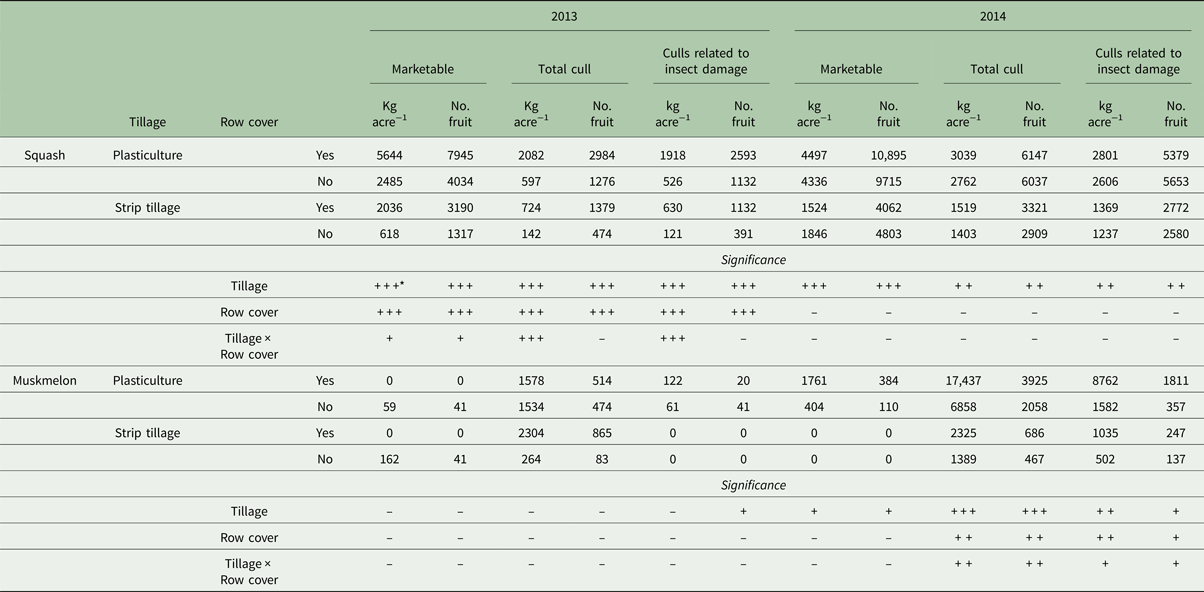
*−, +, + +, + + + Nonsignificant, significant at P ≤ 0.05, 0.01, 0.001 (respectively), based on F test.
Plasticulture increased marketable yield weight and number in both 2013 (3.1- and 2.7-fold, respectively) and 2014 (2.6- and 2.3-fold, respectively) as compared with the strip tillage system. Row cover use increased the marketable yield weight and number in 2013 (2.5- and 2.1-fold, respectively) as compared with the uncovered treatments, but in 2014 row cover use did not show this trend. The greatest marketable yield number and weight were from the plasticulture with row cover system in both years of the study. The greatest yield difference in 2013 was observed between the plasticulture/row cover system and strip till/uncovered system where yields were increased 9.1 (by weight)- and 6.0 (by number)-fold in the plasticulture/row cover system. In 2014, the greatest yield difference was observed between plasticulture/row cover and strip till/row cover with yield increases in the plasticulture/row cover system 3.0 (by weight)- and 2.7 (by number)-fold.
Our results support the findings in similar systems (Tillman et al., Reference Tillman, Nair, Gleason and Batzer2015b; Lilley and Sánchez, Reference Lilley and Sánchez2016) where plasticulture and row cover systems increase marketable yields in organic squash systems.
Melon study
In both years marketable yield was unacceptably low in the melon study (Table 3, Figs. 3A, B) and culls were extremely high, especially 2014 IRC (Table 3, Figs. 3C, D). In 2013 no significant differences were observed in marketable yields or IRC. Few marketable melons were produced, and plasticulture increased the number of melon culled by insect damage. No row cover or interactions were observed in 2014, although plasticulture showed significantly increased fruit number (F = 6.94; d.f. 1, 6; P ≤ 0.05) and weight (F = 7.25, d.f. 1, 6; P ≤ 0.05). In 2014 IRC weight (F = 9.63, d.f. 1, 6; P ≤ 0.05) and fruit number (F = 7.74; d.f. 1, 6; P ≤ 0.05) had significantly different interactions, with plasticulture and row cover treatments having the greatest culls. There were significant interaction observed between row cover use and tillage regime with IRC weight (F = 11.62; d.f. 1, 12; P ≤ 0.01) and fruit number (F = 7.69; d.f. 1, 12; P ≤ 0.05) being greater in 2014 compared with 2013.
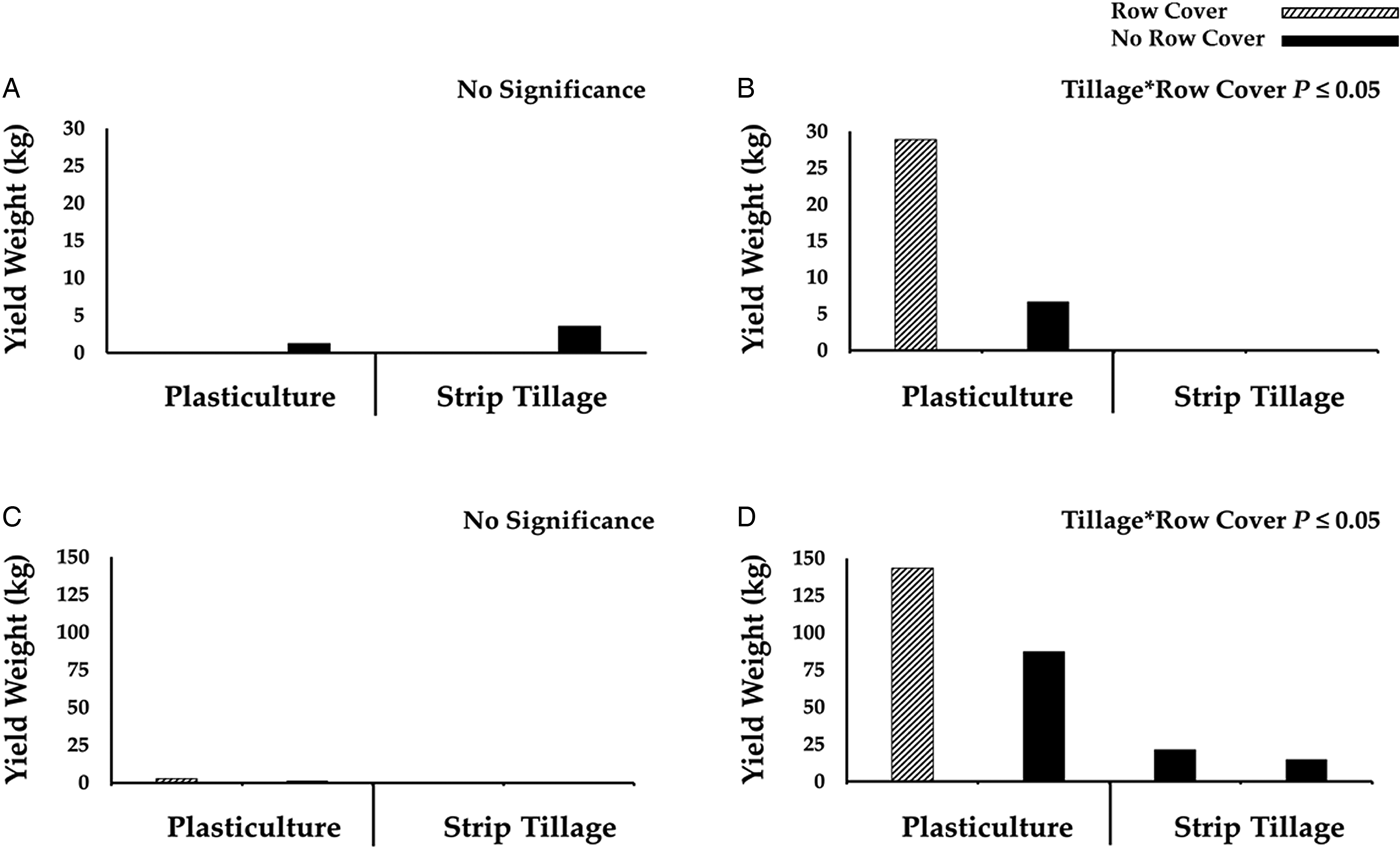
Fig. 3. Total marketable yield (A, B) weight and insect damaged cull weight (C, D) of muskmelon harvested from organically managed summer squash study at the University of Kentucky Horticulture Research Farm, Lexington, Kentucky, 2013 and 2014.
Overall, marketable melon yields were low in both years of the study. Plants under row cover in 2013 produced no marketable fruit, and in the uncovered treatments marketable plant yield was extremely low. In 2014, strip tillage systems produced no marketable yield, and row cover use increased marketable yields in the plasticulture system 4.4 (weight)- and 3.5 (number)-fold as compared with uncovered treatments. In 2013 poor growing conditions in the field (compaction, waterlogging, increased disease), resulted in low yields that a grower would find unacceptable. In 2014, we attribute weed pressure (as a result of increased precipitation) to the reduced marketable yield in the strip tillage system, which was similar to observations by Tillman et al. (Reference Tillman, Nair, Gleason and Batzer2015a).
These results are similar to other studies (Tillman et al., Reference Tillman, Nair, Gleason and Batzer2015a; Lilley and Sánchez, Reference Lilley and Sánchez2016), which have affirmed strip tillage resulted in poor yields in melon production systems. Plasticulture and row cover use have been shown to increase marketable yields in melon (Loy and Wells, Reference Loy and Wells1975; Ibarra et al., Reference Ibarra, Flores and DíAZ-Pérez2001; Tillman et al., Reference Tillman, Nair, Gleason and Batzer2015a; Lilley and Sánchez, Reference Lilley and Sánchez2016), which was reflected in our 2014 study.
Additional influence on yield
The land used in our study was considered ‘in transition’ to organic cultivation. For over 40 years the research plots had been under conventional management but were in the 3-year transitional period required for organic certification at the time of our experiment. Seufert et al. (68) reports improvement in organic production after 2 years of transition; this is supported by our results, with overall yields increasing between 2013 and 2014.
Pest analysis
STCB, SPCB and SB were found to be the most abundant pests in both the squash and melon studies. Additionally, SB egg masses were also found in the squash study, and recorded as they can be a predictor of future pest pressure (Harmon et al., Reference Harmon, Hladilek, Hinton, Stodola and Andow2003; Bonjour et al., Reference Bonjour, Fargo and Rensner2014). First pest appearance was recorded for these major species in both 2013 and 2014 (Supplemental Table 1). Differences in pest arrival to crops between study years were attributed to weather variation effecting plant growth and insect development. These major pests were analyzed to compare the pest pressure between the tillage regimes and row cover treatments in both studies (Table 4).
Table 4: Mean number of pests per week from organically managed summer squash and muskmelon studies at the University of Kentucky Horticulture Research Farm, Lexington, Kentucky, 2013 and 2014.
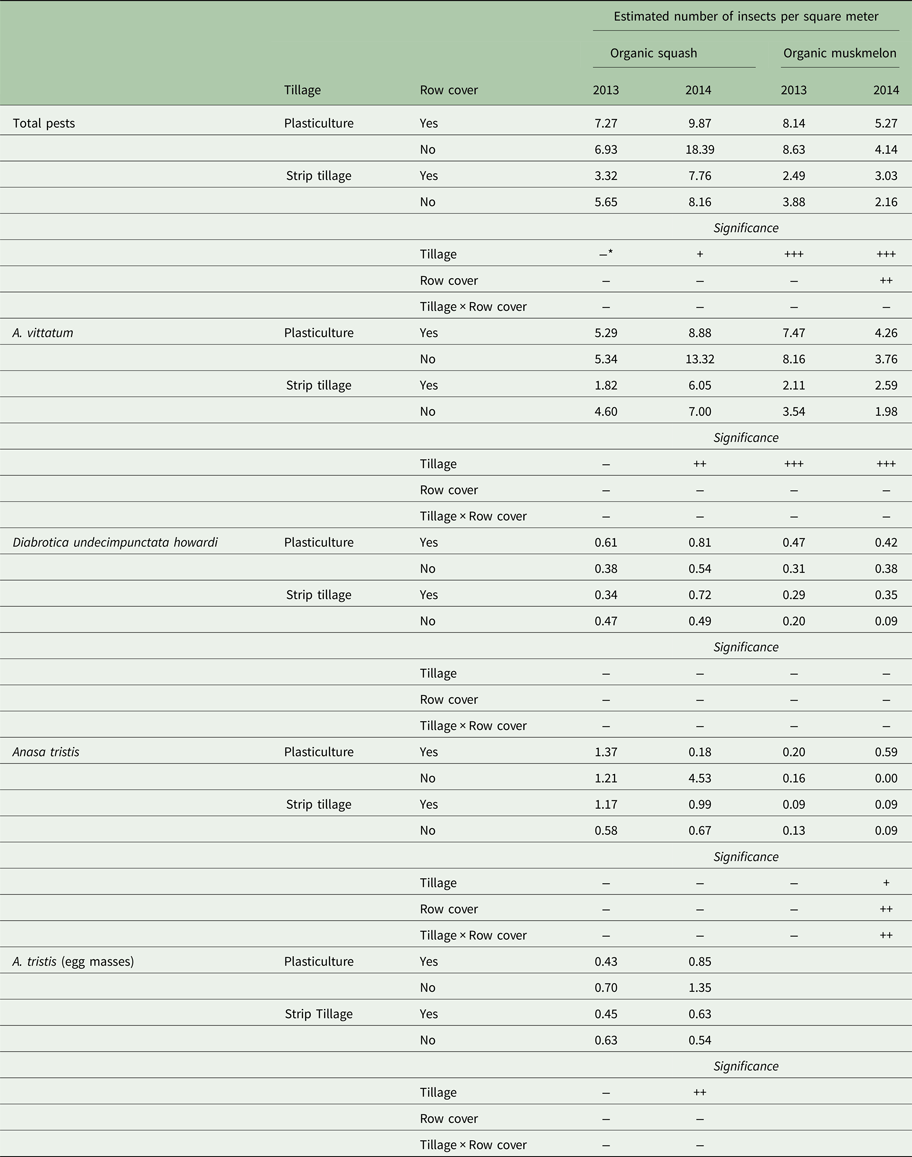
* -, +, + +, + + + Nonsignificant, significant at P ≤ 0.05, 0.01, 0.001 (respectively), based on F test.
The use of row cover early in the growing season reduced the number of insecticide applications compared with the conventional plots. Only three (2013; 2014) chemical applications were needed in the row cover treatments verses four (2013) and five (2014) chemical treatments in the squash study. In the melon study two (2013) and five (2014) chemical treatments verses six (2013) and seven (2014). Other studies have shown that in carefully managed systems row cover use can reduced chemical applications in cucurbits by up to 50% (Orozco-Santos et al., Reference Orozco-Santos, Perez-Zamora and Lopez-Arriaga1995; Rojas et al., Reference Rojas, Gleason, Batzer and Duffy2011).
Total adult insect pests
The total number of adult pests collected throughout the season is important for understanding the pest pressure associated with each treatment. Plasticulture had significantly higher total number of pests in both squash (2014: F = 9.25; d.f. 1, 6; P ≤ 0.05) and melon (2013: F = 31.29; d.f. 1, 6; P < 0.001; 2014: F = 45.75; d.f. 1, 6; P ≤ 0.001), except for the 2013 squash study. This is consistent with reports that strip tillage reduces pest numbers (Stinner and House, Reference Stinner and House1990). Although row cover decreased the early season exposure of the plants to the pests and disease, it increased the number of pests in the 2014 melon study (F = 10.33; d.f. 1, 6; P ≤ 0.05). No interactions were observed between tillage regime and row cover use in either system in either year of the study. When results were compared between 2013 and 2014 tillage was significant for total adult pests in both the squash (F = 10.40; d.f. 1, 12; P ≤ 0.01) and melon system (F = 46.44; d.f. 1, 12; P ≤ 0.001). These differences between the seasons were attributed to field conditions and weather.
Total pest numbers were highest in plasticulture in both the squash and melon studies. With the exception of the 2014 melon study, pests were highest in the uncovered treatments. When the total number of adult pests were compared directly with marketable yield they had no significant impact on squash yield in either year of the study. When the marketable yield of melon was compared with total pests there was no significant impact in 2013, but pests significantly reduced yield weight (F = 5.11; d.f. 1, 14; P ≤ 0.04) and number (F = 7.05; d.f. 1, 14; P ≤ 0.02) in 2014. When total pest number, tillage system and row cover were compared, the impact of pests on yield was not significant in either squash or melon in either year of the study. The effects observed in melon in 2014 were outweighed by the impact of tillage and row cover, and results were as described above.
Striped cucumber beetle
The most abundant insect pest in both 2013 and 2014 in both the squash and melon studies was STCB. Plasticulture significantly increased the number in both the squash (2014: F = 14.22; d.f. 1, 6; P ≤ 0.01) and melon studies (2013: F = 27.76; d.f. 1, 6; P ≤ 0.01; 2014: F = 31.70; d.f. 1, 6; P ≤ 0.001), except in the 2013 squash study. Aside from increased plant size, one reason for this may be sampling error in the strip tillage observations. STCB adults often bury themselves in the soil around the plants for protection and egg oviposition (Mumtaz, Reference Mumtaz2014), therefore observers may have missed beetles buried in the tilled strip or under the plastic. In the squash study there was a significant increase in STCB numbers in 2014 from 2013 (F = 10.39; d.f. 1, 12; P ≤ 0.01). In the 2013 melon study there was a significantly greater number of STCB, with tillage having a significant impact (F = 39.47; d.f. 1, 12; P ≤ 0.001).
In both the squash and melon studies, STCB exceeded the standard economic injury level throughout both 2013 and 2014. All scouting events found greater than our tolerance of one beetle per plant and the recommended 0.5–1 beetle per plant (Brust et al., Reference Brust, Foster and Buhler1996; Burkness and Hutchison, Reference Burkness and Hutchison1998; Brust and Foster, Reference Brust and Foster1999).
Spotted cucumber beetles
Tillage regime, row cover and their interaction had no impact on SPCB numbers in either squash or melon systems, though they were still found in the fields. When data from 2013 and 2014 was compared, year effects were significant with significantly greater number of SPCB in 2013. SPCB are gregarious and pests of many other crops (Branson and Krysan, Reference Branson and Krysan1981; Szalanski and Owens, Reference Szalanski and Owens2003). As active foragers, they have the ability to move with or leave the cropping system for more favorable foraging and reproduction sites. These pests should still be considered in an IPM system because of their ability to spread bacterial wilt and cause feeding damage.
An economic injury level for SPCB has not been reported, but our tolerance threshold was the same as for the STCB.
Squash bug
Plasticulture regimes had greater numbers of SB in both of the squash and melon. SB numbers were not significantly different in the squash study, showing that tillage system and row cover have little effect on population numbers. In the melon study, the interaction between row cover and plasticulture significantly increased the number of squash bugs in 2014 (F = 11.37; d.f. 1, 6; P ≤ 0.05). There were no significant differences between the 2 years in the melon study; but the interaction between tillage and row cover in the squash system was nearly significant between 2013 and 2014 (F = 4.79; d.f. 1, 12; P ≤ 0.06). Similar results were found by Cartwright et al. (Cartwright et al., Reference Cartwright, Palumbo and Fargo1990) when comparing bare ground with plastic mulch systems. Plasticulture is suspected to create a more suitable habitat, by increasing temperature and harborage areas for adults and nymphs (Cartwright et al., Reference Cartwright, Palumbo and Fargo1990). Economic injury levels for SB have not been reported, but the industry standard economic industry level is two adult SB per plant in most cucurbit crops (Dogramaci et al., Reference Dogramaci, Shrefler, Giles and Edelson2006). SB numbers never reached this threshold in the squash or melon studies, but the insecticide applications for STCB and SPCB are suspected to have contributed to the reduced number of SB.
Squash bug egg masses
SB egg masses were found in both squash and melon studies, but in the melon study numbers were too low to be considered for analysis. In the squash study there were no differences in 2013, but there were significantly more egg masses in the plasticulture system in 2014 (F = 11.96; d.f. 1, 6; P ≤ 0.01). When the 2 years were compared, tillage (F = 5.03; d.f. 1, 12; P ≤ 0.05) and row cover (F = 5.92; d.f. 1, 12; P ≤ 0.05) significantly impacted the number of egg masses found; but no interaction was observed. The use of plasticulture appears to reflect the results found in the SB system. Row cover use early in the season prevented overwintering SB adults from laying egg masses on covered plants, therefore more egg masses were found in uncovered treatments both years.
Summary
Our study shows that marketable yield is greatest in plasticulture systems for both melon and squash, supporting the results of previous studies (Mahadeen, Reference Mahadeen2014; Tillman et al., Reference Tillman, Nair, Gleason and Batzer2015a, Reference Tillman, Nair, Gleason and Batzerb; Lilley and Sánchez, Reference Lilley and Sánchez2016). Plasticulture systems have been shown to have increased soil temperatures as compared with strip tillage, even at depths of 15 cm (Tillman et al., Reference Tillman, Nair, Gleason and Batzer2015b), and this increased soil temperatures has been attributed to increased cucurbit biomass and yield (Jenni et al., Reference Jenni, Cloutier, Bourgeois and Stewart1996; Baker and Reddy, Reference Baker and Reddy2001; Tillman et al., Reference Tillman, Nair, Gleason and Batzer2015b). For growers looking to increase plant growth early in the season, the increase in soil temperature in a plasticulture system could be beneficial; alternatively plasticulture's increase in soil temperature later in the season may negatively impact late season plantings and yield (Baker and Reddy, Reference Baker and Reddy2001). Strip tillage increases soil moisture, which impacts vine growth and yield by creating cooler soils temperatures, which would negatively impact early season plantings but benefit late season plantings (Hoyt et al., Reference Hoyt, Monks and Monaco1994; Johnson and Hoyt, Reference Johnson and Hoyt1999; Verhulst et al., Reference Verhulst, Govaerts, Verachtert, Castellanos-Navarrete, Mezzalama, Wall, Deckers and Sayre2010; Tillman et al., Reference Tillman, Nair, Gleason and Batzer2015a). Plasticulture had greater pest pressure than the strip tillage system in both systems in both years of our study, establishing that cucurbits in strip tillage have reduced pest pressure. Tillage regime is important in management decisions. A tillage system that produces greater marketable yields may result in a grower willing to invest in other management methods to control the pests.
Our results show that row cover does increase yield weight and number in squash and melon systems, this is similar to results of previous studies (Orozco-Santos et al., Reference Orozco-Santos, Perez-Zamora and Lopez-Arriaga1995; Cline et al., Reference Cline, Sedlacek, Hillman, Parker and Silvernail2008; Tillman et al., Reference Tillman, Nair, Gleason and Batzer2015a; Reference Tillman, Nair, Gleason and Batzerb). Row covers are beneficial in organic IPM systems for increasing temperatures in early season production, for acting as a pest barrier, to prevent plant disease and reduce the use of insecticide (Webb and Linda, Reference Webb and Linda1992; Orozco-Santos et al., Reference Orozco-Santos, Perez-Zamora and Lopez-Arriaga1995; Condron et al., Reference Condron, Cameron, Di, Clough, Forbes, Mclaren and Silva2000). In melon production systems it has been shown that row covers can create extremely warm temperatures that can injure plants (Jenni et al., Reference Jenni, Cloutier, Bourgeois and Stewart1996; Ibarra et al., Reference Ibarra, Flores and DíAZ-Pérez2001); which, in combination with weak transplants, may have contributed the yield losses seen in 2013. Row cover did not have a significant impact on overall pest presence, except for the melon study in 2014 where it increased pest presence. It did reduce the amount of organic insecticide used and protected plants from predation and disease, resulting in more productive plants. Products like ProtekNet mesh (Brown, Reference Brown2014; Hanna et al., Reference Hanna, Carlson, Steward and Rosentrater2015), or a thinner polyethylene cover could be used as an alternative row cover in future studies to create a more favorable growing climate while protecting from pest damage.
A major component of an IPM system is the use of multiple methods to control pests and reduce the environmental impacts of an agriculture system, while still providing producers an economically viable production system (Kogan, Reference Kogan1998). It has been shown that pest arthropod populations increase in organic production systems, but research suggests pest impact is reduced when considered at the farm level (Garratt et al., Reference Garratt, Wright and Leather2011). Plasticulture with the use of row cover produced the greatest marketable yields, but also increases the overall pest abundance. The greatest IRC were observed in plasticulture systems, but insect damage only significantly decreased marketable yields in the 2014 melon study. Strip tillage has been shown to support greater natural enemy and microbial biomass (Mäder et al., Reference Mäder, Fliessbach, Dubois, Gunst, Fried and Niggli2002; Hole et al., Reference Hole, Perkins, Wilson, Alexander, Grice and Evans2005; Verhulst et al., Reference Verhulst, Govaerts, Verachtert, Castellanos-Navarrete, Mezzalama, Wall, Deckers and Sayre2010; Lewis et al., Reference Lewis, Fleischer and Roberts2016), but reduced yields do not make these systems viable. Yield decreases observed in the strip tillage and uncovered treatments were unacceptably low, particularly in the melon system. This agrees with the findings of our collaborators (Tillman et al., Reference Tillman, Nair, Gleason and Batzer2015a; Lilley and Sánchez, Reference Lilley and Sánchez2016), leading to the conclusion that plasticulture is a more productive system for squash and melon production.
Conclusions
Developing alternative pest management solutions while maintaining or increasing yield should be a future research focus. Future work must focus on the incorporation of more effective means to control insect pests in organic cucurbit systems. The potential for row cover to be beneficial has been clearly demonstrated, but future improvements in row cover material and season long applications must be investigated. While strip tillage with the use of row cover may be ideal from an IPM perspective, yield reductions do not make this system economically feasible. Future plant breeding research to improve yields may make this tillage system a viable alternative to plasticulture.
The results of our study can be directly applied to an organic cucurbit IPM system. Although there is strong evidence towards the benefits of strip tillage in certain agricultural settings, it does not appear that strip tillage is preferable to plasticulture in the production of organic cucurbit crops, particularly melons. Alternative IPM methods will need to be investigated for these cropping systems. Our results show that it is important to understand the combined impact of growing practices, such as tillage regime and row cover, on a cucurbit system.
Supplementary material
The supplementary material for this article can be found at https://doi.org/10.1017/S1742170517000503.
Acknowledgements
We thank Jessica Bessin, Luke Hanks, Joseph Tucker, Steve Diver, Eric Newberg, Nathan Skidmore, Zachariah Helton, Luke Box, Andrew Collins and members of the University of Kentucky Horticulture Research Farm for their technical support and assistance. We are grateful to Kacie Athey for her assistance with statistical analysis and helpful comments during manuscript preparation. Funding was provided by US Department of Agriculture, National Institute of Food and Agriculture, Specialty Crop Research Initiative. This is publication number 17-08-019 of the Kentucky Agricultural Experiment Station and is published with the approval of the Director.