Corn (Zea mays L.) and cotton (Gossypium hirsutum L.) cultivars containing both glufosinate and glyphosate resistance traits have been commercially released to help growers manage glyphosate-resistant weeds by applying two herbicides with distinct modes of action (Green Reference Green2012). Glyphosate and glufosinate are nonselective POST herbicides. Glufosinate inhibits the activity of glutamine synthetase enzyme, leading to ammonia accumulation, cell destruction, and direct quenching of photosystem I and photosystem II (PSII) (Wild et al. Reference Wild, Sauer and Rühle1987). Glyphosate inhibits activity of 5-enolpyruvyl-shikimate-3-phosphate synthase, causing a depletion of aromatic amino acid pools required for plant growth (Steinrücken and Amrhein Reference Steinrücken and Amrhein1980). Mixing systemic herbicides with different modes of action is considered a critical component of herbicide strategies based on best management practices (Norsworthy et al. Reference Norsworthy, Ward, Shaw, Llewellyn, Nichols, Webster, Bradley, Frisvold, Powles, Burgos, Witt and Barrett2012). By simultaneously targeting different sites of action within a weed species, herbicide tank mixtures may more efficiently delay the onset of herbicide resistance than rotating the same mode of action annually (Beckie and Reboud Reference Beckie and Reboud2009; Powles et al. Reference Powles, Preston, Bryan and Jutsum1996). However, herbicide combinations may also result in detrimental weed response, such as antagonism characterized by a less than expected outcome based upon the effect of each herbicide applied alone.
Antagonism between glyphosate and glufosinate has been reported in goosegrass [Eleusine indica (L.) Gaertn.] (Chuah et al. Reference Chuah, Teh, Cha and Ismail2008), wild mustard (Sinapis arvensis L.), and white mustard (Sinapis alba L.) (Kudsk and Mathiassen Reference Kudsk and Mathiassen2004), regardless of herbicide rates, formulation, or weed growth stage. In both studies, antagonism was hypothetically attributed to the faster action of glufosinate, which could have precluded uptake and translocation of the slower-acting glyphosate and impeded its activity. Field and greenhouse studies revealed an antagonistic interaction at 28 d after treatment (DAT) between glyphosate at 840 g ae ha−1 and glufosinate at 240 g ai ha−1 in velvetleaf, common lambsquarters, and giant foxtail (Bethke et al. Reference Bethke, Molin, Sprague and Penner2013). Fluorescence measurements confirmed the rapid action of glufosinate resulting in the breakdown of the PSII system, therefore supporting the hypothesis of reduced glyphosate translocation to explain the antagonistic interaction. Antagonism between glyphosate and other herbicides has also been reported. Rate and formulation of 2,4-D affected glyphosate efficacy on johnsongrass [Sorghum halepense (L.) Pers.] (Flint and Barrett Reference Flint and Barrett1989). Antagonism was also reported when glyphosate was tank mixed with atrazine and linuron (Selleck and Baird Reference Selleck and Baird1981), fomesafen and sulfentrazone (Starke and Oliver Reference Starke and Oliver1998), and diquat (Wehtje et al. Reference Wehtje, Altland and Gilliam2008).
Physiological investigations of antagonism between glyphosate and growth regulator herbicides in johnsongrass have shown that 2,4-D and dicamba reduced glyphosate uptake and altered the translocation pattern of absorbed glyphosate (Flint and Barrett Reference Flint and Barrett1989). Similar results were obtained with fomesafen. Fomesafen, when mixed with equal rates of glyphosate at 210 and 420 g ha−1 and applied to barnyardgrass [Echinochloa crus-galli (L.) Beauv.], pitted morningglory (Ipomoea lacunosa L.), and velvetleaf, reduced glyphosate absorption by 7% to 24% and decreased glyphosate translocation by 7% to 12% (Starke and Oliver Reference Starke and Oliver1998). Wehtje et al. (Reference Wehtje, Altland and Gilliam2008) conjectured that rapid tissue necrosis caused by diquat decreased glyphosate translocation through the fixation of glyphosate at the point of application in long-stalked phyllanthus (Phyllanthus tenellus Roxb.). However, to date, no studies have been conducted to identify herbicide absorption and translocation in response to application of mixtures of glyphosate and glufosinate. Therefore, the objectives of this study were (1) to confirm previously reported antagonism of glyphosate activity by glufosinate on velvetleaf, common lambsquarters, and giant foxtail; and (2) to determine the roles of glyphosate absorption and translocation in this antagonistic interaction.
Materials and Methods
Evaluation of Herbicide Interaction
Greenhouse studies were conducted at the Michigan State University Agronomy Research Center (42.42°N, 84.28°W) in East Lansing, MI. Plants of common lambsquarters, giant foxtail, and velvetleaf were grown from seed in 9-cm pots containing a commercial potting medium (Metro-Mix® 200, Sun-Gro Horticulture, Agawam, MA) and thinned to 1 plant pot−1 upon emergence. Plants were watered as needed, and temperature was maintained at 25±2 C. Natural sunlight was supplemented 4 h daily with metal-halide lamps emitting an average midday photosynthetic photon flux of 700 μmol m−2 s−1 to provide a 16/8 h light/dark photoperiod. Plants were fertilized as needed with a fertilizer solution containing 6 mg L−1 of 20% nitrogen, 20% P2O5, and 20% K2O.
To emphasize the expression of antagonistic or synergistic response to herbicide mixtures, herbicides were applied on 10- to 15-cm-tall plants, slightly beyond the maximum label-recommended height (8-, 10-, and 15-cm for velvetleaf, lambsquarters, and giant foxtail, respectively, on the glufosinate label; 15-cm for all species on the glyphosate label). On the basis of the 1X rate labeled for control of the weed species selected in our study, glyphosate (Roundup WeatherMax®, Monsanto, St Louis, MO) was applied at 110 (0.125X) and 220 (0.25X) g ae ha−1 on velvetleaf and giant foxtail or 170 (0.125X) and 340 (0.25X) g ha−1 on common lambsquarters, and glufosinate (Ignite®, Bayer CropScience, Research Triangle Park, NC) was applied at 20 (0.05X) and 40 (0.1X) g ai ha−1 on all species. Glyphosate and glufosinate were applied alone or in combination at a low (0.125X glyphosate and 0.05X glufosinate) or high rate (0.25X glyphosate and 0.1X glufosinate). A nontreated control was also included. Ammonium sulfate was included at 2% w/w in all treatments. Applications were made using a single-nozzle track sprayer equipped with an 8001 even flat-fan nozzle (TeeJet®, Spraying Systems, Wheaton, IL) calibrated to deliver 190 L ha−1 at 170 kPa. Weed control was visually evaluated at 7, 14, and 21 DAT for velvetleaf and giant foxtail and at 7 and 14 DAT for common lambsquarters on a 0% (no control) to 100% (death of all plants) scale, based on a composite estimation of density reduction, growth inhibition, and foliar injury (Frans et al. Reference Frans, Talbert, Marx and Crowley1986).
The experiment was conducted as a randomized complete block design with a factorial arrangement of treatments with four replications, each replication consisting of an individual plant. The experiment was repeated once. All data were subjected to ANOVA using PROC MIXED in SAS v. 9.4 (SAS Institute, Cary, NC), with replications considered as a random effect. Data were transformed to the arcsine square-root before ANOVA to stabilize the variances (Grafen and Hails Reference Grafen and Hails2002). Data were combined over experimental runs, as there were no significant treatment by runs or repetitions interactions. Because the transformation of the data did not change the separation of means, only nontransformed data are presented. Antagonistic, synergistic, or additive interaction between herbicide combinations was determined by comparing observed and expected weed control responses. Equation 1 was used for calculating the expected response (Colby Reference Colby1967):

where X and Y are the percent of weed control by herbicides A and B, respectively, and E is the expected percent weed control by herbicides A and B combined. Observed and expected responses were compared using Fisher’s protected LSD test at P≤0.05 level of significance. Determination of antagonistic, synergistic, or additive interaction was based on observed response less than, greater than, or similar to the expected response, respectively.
[14C]Glyphosate Absorption and Translocation Studies
Planting and growth conditions were similar to those described in the previous section. Herbicides were applied to 7.5-cm-tall common lambsquarters and giant foxtail and 10-cm-tall velvetleaf. Treatments consisted of glyphosate applied POST alone at 340 g ha−1 on common lambsquarters or 220 g ha−1 on giant foxtail and velvetleaf, and glyphosate at similar rates on the same species plus glufosinate at 40 g ha−1 using a spray chamber calibrated to deliver 190 L ha−1 at 170 kPa. All treatments included ammonium sulfate (AMS) at 2% w/w. Before each POST application of glyphosate, a 3-cm² area on the adaxial surface of the youngest fully expanded mature leaf was covered with aluminum foil held in place with paper clips to prevent the spray from coming into contact with the leaf surface. A spotting solution containing [14C]phosphonomethyl-labeled glyphosate acid (Sigma-Aldrich, St Louis, MO) with 98.7% purity and a specific activity of 481 kBq mg−1, and high-performance liquid chromatography–grade water was prepared to simulate a spray solution based on glyphosate rates previously described. After plants were sprayed, a microsyringe equipped to deliver 1-µl droplets was used to evenly spread ten 1-µl droplets of spotting solution containing a total of 3.3 kBq plant−1 on the previously covered leaf area of each species.
Plants were harvested at 4, 12, 24, 48, and 72 h after treatment (HAT). Absorption was determined by rinsing the treated leaf portion with 10 ml of methanol:water (1:1 v/v) plus 0.25% nonionic surfactant (Induce®, Helena Chemical, Collierville, TN) (Askew and Wilcut Reference Askew and Wilcut2002; Pline et al. Reference Pline, Price, Wilcut, Edmisten and Wells2001). A 1-ml aliquot from each leaf rinse was sampled, diluted in 20 ml of scintillation fluid (Scintiverse BD Cocktail®, Fisher Scientific, Fair Lawn, NJ), and quantified for radioactivity with liquid scintillation spectrometry (LSS) (Packard Tri-Carb Liquid Scintillation Counter, PerkinElmer, Waltham, MA) to determine the amount of nonabsorbed herbicide. All plants were then separated into (1) treated leaf, (2) above treated leaf, (3) below treated leaf, and (4) roots. The treated leaf was removed at the point of attachment to the stem, which determined the division for above and below treated–leaf sections. Plant parts were dried for 48 h at 40 C, weighed, ground for homogenization, and then combusted with a biological sample oxidizer (Harvey Biological Oxidizer, J. Harvey Instrument, Hillsdale, NJ) to recover absorbed [14C]glyphosate as 14CO2. Radioactivity in each oxidized sample was quantified by LSS, and a background reading was subtracted from all data points. Since metabolism of glyphosate by weeds is considered to be null or negligible (Duke Reference Duke2011), metabolites of [14C]glyphosate were not investigated in this study.
Absorption and translocation studies were analyzed as a factorial arrangement in a randomized complete block design, with treatments replicated three times each in two experimental runs. Factors included herbicide treatment (glyphosate alone or mixed with glufosinate), harvest timings (4, 12, 24, 48, and 72 HAT), and plant parts (treated leaf, above treated leaf, below treated leaf, and root). Following analysis and parameterization in R statistical software (R Foundation for Statistical Computing, Vienna, Austria), absorption was modeled over time in SigmaPlot v. 12.0 (Systat Software, San Jose, CA) using either asymptotic regression (Equation 2) or rectangular hyperbolic (Equation 3) models (Kniss et al. Reference Kniss, Vassios, Nissen and Ritz2011) with the following two-parameter functions:


where Y is expressed as the percentage of the applied dose, A max is the maximum percentage of applied [14C]glyphosate that was absorbed, t is the time, and t 90 is the time required for 90% of maximum absorption to occur. Similarly, translocation was modeled over time using either asymptotic regression (Equation 4) or rectangular hyperbolic (Equation 5) models with the following two-parameter functions:


where Y is expressed as the percentage of the absorbed dose, T max is the maximum percentage of absorbed [14C]glyphosate that was translocated, t is the time, and t 90 is the time required for 90% of maximum translocation to occur. Maximum absorption (A max) and maximum translocation (T max) values as predicted by nonlinear regression models for each herbicide treatment within each species were compared using a paired t-test. Percentage of [14C]glyphosate translocated was subjected to ANOVA using the generalized linear mixed model (PROC GLIMMIX) procedure in SAS v. 9.4 (SAS Institute). Herbicide treatments, harvest timings, plant species, plant parts, and their interaction were considered fixed variables, whereas replication, experimental run, and their interaction were considered random effects (Carmer et al. Reference Carmer, Nyquist and Walker1989). Treatment means were separated using Fisher’s protected LSD test at P≤0.05 level of significance.
Results and Discussion
Herbicide Interaction Studies
Velvetleaf
By 14 DAT, glyphosate applied alone at 110 g ha−1 and 220 g ha−1controlled velvetleaf 46% and 73%, respectively (Figure 1A). Lower control with glufosinate alone was noted, with 40% and 53% control at 20 g ha−1 and 40 g ha−1, respectively. Glufosinate slightly antagonized velvetleaf control by glyphosate when both herbicides were applied at their respective lowest rate, with control reduced by 5% compared with Colby’s predicted value (P=0.072). A similar reduction occurred when glyphosate and glufosinate were combined at the highest rate, with 80% observed control, whereas Colby’s equation predicted 87% control (P=0.044). However, by 21 DAT, antagonism was no longer evident regardless of combinations (unpublished data). In a similar study, Bethke et al. (Reference Bethke, Molin, Sprague and Penner2013) reported antagonism in velvetleaf control for glyphosate applied at 210 g ha−1 and combined with glufosinate at 120 or 470 g ha−1 in the greenhouse, but also noted additive interaction in the field when both glyphosate and glufosinate were applied at their respective recommended rates of 840 g ha−1 and 470 g ha−1, respectively.
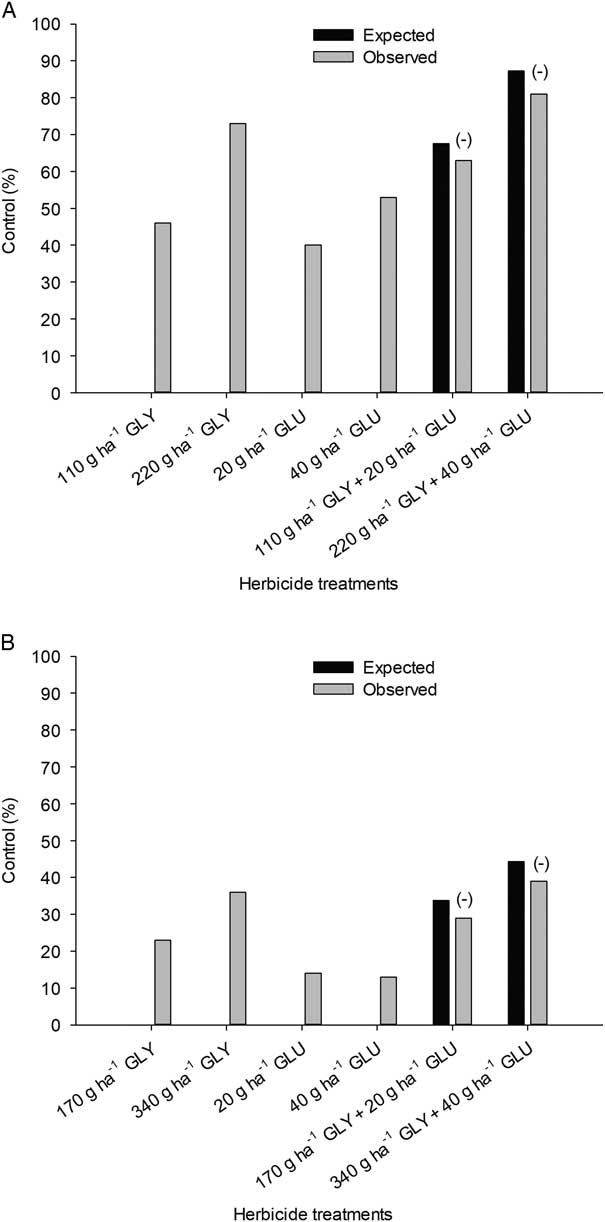
Figure 1 Control of velvetleaf (A) and common lambsquarters (B) with glyphosate (GLY) and glufosinate (GLU) 14 d after treatment. Antagonism by Colby’s method indicated by a minus sign (−). LSD=7 (A) and 5 (B).
Common Lambsquarters
Although glyphosate was applied at higher rates, lower common lambsquarters control at 14 DAT was recorded with glyphosate alone at 170 g ha−1 (23%) and 340 g ha−1 (30%) compared with velvetleaf (Figure 1B). This result is not unexpected. Common lambsquarters was about 10-cm tall when herbicides were applied. Previous studies have pointed out that glyphosate is less effective for control when common lambsquarters is 6 cm or taller (Boerboom et al. Reference Boerboom, Stoltenberg, Jeschke, Trower and Gaska2006; Schuster et al. Reference Schuster, Shoup and Al-Khatib2007; Sivesind et al. Reference Sivesind, Gaska, Jeschke, Boerboom and Stoltenberg2011). No combination of glyphosate and glufosinate significantly increased common lambsquarters control compared with glyphosate alone. Bethke et al. (Reference Bethke, Molin, Sprague and Penner2013) reported that the combination of 840 g ha−1 of glyphosate with 240 g ha−1of glufosinate produced a low level of antagonism on lambsquarters. In this study, both combinations of glyphosate and glufosinate at 7 (unpublished data) and 14 DAT (Figure 1B) produced a low level of antagonism. Additional studies reported no antagonism between glyphosate and glufosinate for common lambsquarters control when glufosinate was applied at higher rates relative to glyphosate (Chuah et al. Reference Chuah, Teh, Cha and Ismail2008; Kudsk and Mathiassen Reference Kudsk and Mathiassen2004). The overall conclusion from these studies is that the combination of glyphosate and glufosinate is additive to very weakly antagonistic for common lambsquarters control.
Giant Foxtail
Both rates of both herbicides controlled giant foxtail 20% to 50% at 7 DAT (Figure 2A). At 21 DAT, control for the slower-acting glyphosate increased to 85% and 95% for applied rates of 110 and 220 g ha−1, respectively, while control for the faster-acting glufosinate decreased to 14% and 44% for applied rates of 20 and 40 g ha−1, respectively (Figure 2B). Marked antagonism was observed for both herbicide combinations for both evaluation timings. At 7 DAT, observed control was 32% (P=0.013) and 19% (P=0.046) lower than predicted by Colby’s equation for glyphosate plus glufosinate combined at the low and high rates, respectively. At 21 DAT, levels of antagonism were reduced but still significant. At 21 DAT, observed control was 19% (P=0.021) and 16% (P=0.045) lower than predicted by Colby’s equation for glyphosate and glufosinate combined at the low and high rates, respectively. Similar results of antagonism were observed on giant foxtail at 28 DAT, with 14% and 11% lower observed control for glyphosate plus glufosinate applied at 420 and 120 g ha−1 and 840 and 240 g ha−1, respectively (Bethke et al. Reference Bethke, Molin, Sprague and Penner2013). Antagonism on goosegrass has also been noted (Chuah et al. Reference Chuah, Teh, Cha and Ismail2008). At 21 DAT, observed fresh weights of goosegrass decreased by 14% compared with Colby’s predicted value for the combination of 150 g ha−1 of glyphosate and 12.5 g ha−1 of glufosinate (Chuah et al. Reference Chuah, Teh, Cha and Ismail2008). Increasing the rate of glufosinate to 25 or 50 g ha−1 tended to decrease the amount of antagonism. Our results and other reported results support the hypothesis that very low rates of glufosinate in combination with glyphosate cause antagonism on grass weed species.
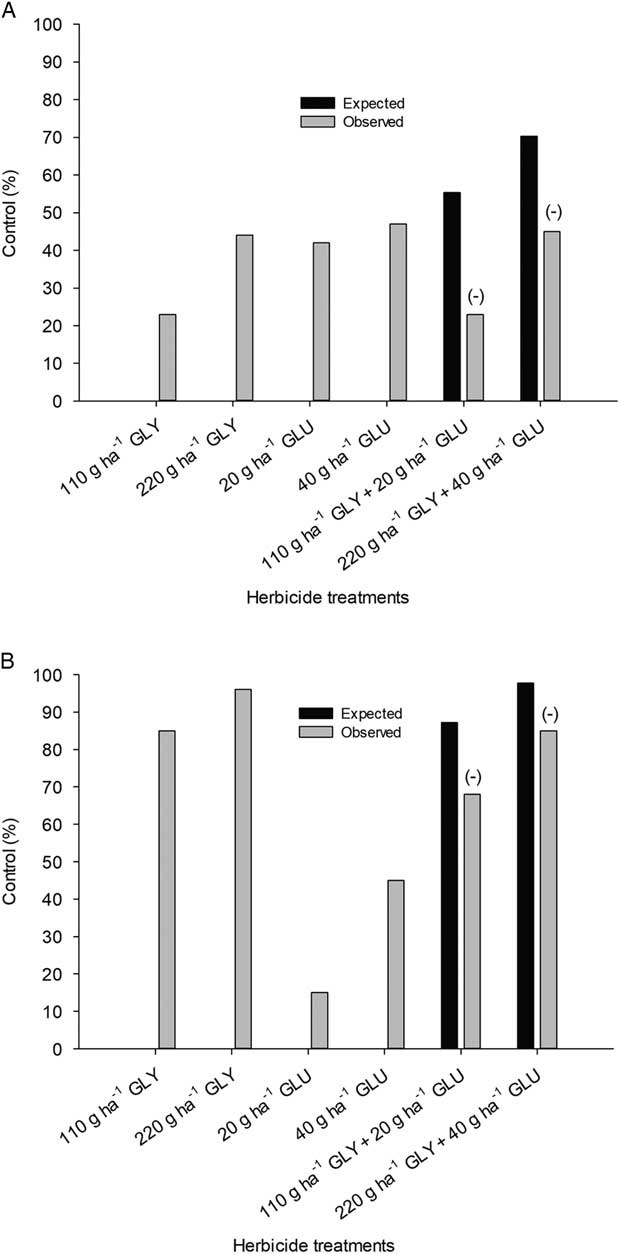
Figure 2 Control of giant foxtail with glyphosate (GLY) and glufosinate (GLU) 7 (A) and 21 d after treatment (B). Antagonism by Colby’s method indicated by a minus sign (−). LSD=19 (A) and 12 (B).
[14C]Glyphosate Absorption
The treatment by experimental run interaction was not significant for [14C]glyphosate absorption (based on total 14C recovered from leaf wash and plant parts) (P≥0.05); data were therefore pooled over runs. Nonlinear regression analysis revealed similar absorption patterns for glyphosate alone and glyphosate combined with glufosinate in velvetleaf (Figure 3A) and common lambsquarters (Figure 3B). However, higher glyphosate absorption was noted for glyphosate combined with glufosinate as compared with glyphosate alone in giant foxtail (Figure 3C). Predicted A max values for glyphosate alone were 21% for velvetleaf and 47% for common lambsquarters (Table 1). These results are consistent with results of previous studies that reported 20% to 50% absorption of applied glyphosate for the same weed species (Yerka et al. Reference Yerka, Wiersma, Lindenmayer, Westra, Johnson, de Leon and Stoltenberg2013; Young et al. Reference Young, Knepp, Wax and Hart2003). However, the addition of glufosinate increased A max of 14C from glyphosate in velvetleaf and giant foxtail by 9% and 23%, respectively. Steckel at al. (1997) reported that giant foxtail and velvetleaf also readily absorb glufosinate. At 24 HAT, giant foxtail and velvetleaf absorbed 67% and 42% of applied glufosinate, respectively, while common lambsquarters absorbed only 16% of applied herbicide during this same time interval (Steckel et al. Reference Steckel, Hart and Wax1997). In addition, several researchers have shown that inclusion of AMS in the spray mixture increases the absorption of glyphosate and glufosinate in velvetleaf and giant foxtail, but not in common lambsquarters (Maschhoff et al. Reference Maschhoff, Hart and Baldwin2000; Young et al. Reference Young, Knepp, Wax and Hart2003). In the present study, supplemental addition of ammonium ion from AMS may have interacted with glufosinate to form glufosinate-ammonium and allow glufosinate-ammonium to increase foliar absorption of glyphosate in velvetleaf and giant foxtail. Hall et al. (Reference Hall, Hart and Jones2000) proposed that AMS may enhance glyphosate absorption by overcoming the antagonistic interaction between the glyphosate anion and cations both on and within the plant, notably calcium, which has been shown to be two times more abundant in velvetleaf than in common lambsquarters plant parts. Analysis of the calcium content of giant foxtail would help to determine whether increased ammonium concentration due to the addition of glufosinate-ammonium may explain the increased absorption of [14C]glyphosate. Analysis of calcium was beyond the scope of this study.

Figure 3 Absorption of foliar-applied [14C]glyphosate (GLY) alone or with glufosinate (GLU) over time in velvetleaf (A), common lambsquarters (B), and giant foxtail (C) (data pooled over trials).
Table 1 Maximal absorption (A max) of foliar-applied [14C-]glyphosate in three plant species depending on glufosinate application, as predicted by nonlinear regression models (data pooled over trials).

a Abbreviations: ABUTH, velvetleaf; CHEAL, common lambsquarters; SETFA, giant foxtail.
[14C]Glyphosate Translocation
Translocation of 14C from glyphosate was not affected by experimental runs or harvest timing (P≥0.05); thus, data were averaged over trials and harvest timings (Table 2). The greatest quantities of 14C remained in treated leaves for all species and herbicide treatments. For the glyphosate-only treatments, 15% or less of absorbed [14C]glyphosate translocated out of treated leaves in velvetleaf and common lambsquarters, while more than 52% of absorbed [14C]glyphosate translocated out of treated leaves in giant foxtail. These results are in agreement with previous studies reporting no more than 25% of [14C]glyphosate translocated out of treated leaves in common lambsquarters (Schuster et al. Reference Schuster, Shoup and Al-Khatib2007; Yerka et al. Reference Yerka, Wiersma, Lindenmayer, Westra, Johnson, de Leon and Stoltenberg2013) or in velvetleaf (Belles et al. Reference Belles, Shaner, Westra and Brunk2006; Young et al. Reference Young, Knepp, Wax and Hart2003). To our knowledge, no data pertaining to glyphosate translocation in giant foxtail have been published. However, substantial translocation of glyphosate in giant foxtail is anticipated, because Pline et al. (Reference Pline, Wu and Hatzios1999) reported about 80% of the absorbed radioactivity of [14C]glufosinate translocated out of treated leaves in giant foxtail in all of their harvest timings.
Table 2 Translocation of foliar-applied [14C]-glyphosate averaged over runs and harvest timings.Footnote a

a Abbreviations: ABUTH, velvetleaf; CHEAL, common lambsquarters; SETFA, giant foxtail; ATL, above treated leaf; BTL, below treated leaf; R, roots; TL, treated leaf.
b Means followed by the same letter are not different according to Fisher’s protected LSD test at P=0.05.
For the glyphosate-only treatments, partitioning of translocated radioactivity differed among species (Table 2). Average acropetal translocation to foliage above treated leaves (6.9%) and basipetal translocation to foliage below treated leaves (8.1%) were statistically equivalent in common lambsquarters. Translocation of [14C]glyphosate to roots of common lambsquarters was negligible. Acropetal prevailed over basipetal translocation in velvetleaf and giant foxtail. However, translocation of [14C]glyphosate to velvetleaf roots was limited (1.2%), while a substantial portion (17.4%) of absorbed [14C]glyphosate translocated to roots in giant foxtail. Glyphosate is translocated predominantly to actively growing meristems. The meristematic region for shoot growth is located at the base of the whorl in giant foxtail. In this study, giant foxtail plants were 7.5-cm tall when herbicides were applied. At this growth stage, meristematic regions for shoot and root growth in giant foxtail are very close physically. Similar translocation patterns of glyphosate have been observed in other grass species such as browntop millet [Urochloa ramosa (L.) Nguyen] and barnyardgrass (Burke et al. Reference Burke, Koger, Reddy and Wilcut2007; Kirkwood et al. Reference Kirkwood, Hetherington, Reynolds and Marshall2000).
The addition of glufosinate to glyphosate did not alter the total amount or the pattern of partitioning of [14C]glyphosate in common lambsquarters (Table 2). However, the addition of glufosinate to glyphosate did alter partitioning patterns of glyphosate in velvetleaf and giant foxtail. Only 2.6% of [14C]glyphosate translocated out of the treated leaf in velvetleaf, with the majority (2.4%) of herbicide translocated acropetally to shoot tissue above the treated leaf.
In giant foxtail, glufosinate significantly increased the total amount of [14C]glyphosate remaining in the treated leaf (78.4%) compared with glyphosate alone (47.6%) (Table 2). Glufosinate significantly decreased (P<0.0001) acropetal translocation of glyphosate at all evaluation timings (Figure 4A). Maximum translocation of glyphosate to leaf tissue above the treated leaf was predicted to be 26.7% of the total absorbed amount for the glyphosate-only treatment versus 9.2% for the combination treatment of glyphosate plus glufosinate. Similar results were obtained for translocation of glyphosate to giant foxtail roots. Predicted values for maximum translocation of [14C]glyphosate dropped from 21.7% to 6.8% in the absence and presence of glufosinate, respectively (Figure 4C). No significant difference (P=0.077) between the two treatments was found for basipetal translocation to plant shoot tissue below the treated leaf (Figure 4B).

Figure 4 Translocation of foliar-applied [14C]glyphosate (GLY) alone or with glufosinate (GLU) over time in giant foxtail above the treated leaf (A), below the treated leaf (B), and to the roots (C), with data pooled over trials.
Bethke et al. (Reference Bethke, Molin, Sprague and Penner2013) showed, via fluorescence measurements, that glufosinate rapidly breaks down the PS II system and masks the effects of the slower-acting glyphosate. Chuah et al. (Reference Chuah, Teh, Cha and Ismail2008) and Kudsk and Mathiassen (Reference Kudsk and Mathiassen2004) observed similar results and drew similar conclusions in their studies. Both reports hypothesized that antagonism between glyphosate and glufosinate occurs because glufosinate rapidly destroys the photosynthetic apparatus and reduces glyphosate translocation throughout the plant. Our results support this hypothesis by showing that addition of glufosinate to glyphosate significantly reduced glyphosate translocation in giant foxtail and, to a lesser extent, in velvetleaf, despite increased absorption of glyphosate in the presence of glufosinate in both species. These test results matched those obtained in the greenhouse study conducted by Bethke et al. (Reference Bethke, Molin, Sprague and Penner2013), who reported that glufosinate at the 120 (0.25X) to 240 (0.5X) g ha−1 rates combined with glyphosate at 210 (0.25X) to 840 (1X) g ha−1 antagonized the herbicidal activity of glyphosate on giant foxtail and velvetleaf. Kudsk and Mathiassen (Reference Kudsk and Mathiassen2004) hypothesized that glufosinate may antagonize glyphosate because different adjuvants used in the product formulations may result in a suboptimal adjuvant system for glyphosate absorption when the commercial products are mixed. Our test results for common lambsquarters support this hypothesis, with 46.8% versus 35.6% [14C]glyphosate uptake when glyphosate is applied alone or mixed with glufosinate, respectively (Table 1). However, results observed for velvetleaf and giant foxtail are not in agreement with this hypothesis, as glufosinate mixed with glyphosate increased absorption of glyphosate (Table 1).
In conclusion, the results from this study suggest that reduced translocation of glyphosate is the physiological mechanism responsible for the antagonism observed between glyphosate and glufosinate in giant foxtail, and to a lesser extent, in velvetleaf.