Introduction
During the Mesozoic, the conifer family Araucariaceae was distributed throughout the Northern and Southern Hemispheres (Stockey, Reference Stockey1982, Reference Stockey1994; Stockey and Ko, Reference Stockey and Ko1986; Hill, Reference Hill, Enright and Hill1995; Del Fueyo and Archangelsky, Reference Del Fueyo and Archangelsky2002; Axsmith et al., Reference Axsmith, Escapa and Huber2008). Its fossil record is remarkably rich from the Lower Jurassic to the Upper Cretaceous, although Triassic occurrences are less common and often have doubtful affinities with the family (Stockey, Reference Stockey1982; Dettmann et al., Reference Dettmann, Clifford and Peters2012; Rothwell et al., Reference Rothwell, Mapes, Stockey and Hilton2012). Fossil woods similar to Araucariaceae (i.e., isolated pieces of secondary xylem with araucarian tracheid radial pitting and araucarioid cross fields, together with mostly uniseriate rays) have been given mainly three generic names, Araucarioxylon Kraus, Reference Kraus and Schimper1870, Dadoxylon Endlicher, Reference Endlicher1847, and Agathoxylon Hartig, Reference Hartig1848 (Rößler et al., Reference Rößler, Philippe, van Konijnenburg-van Cittert, McLoughlin, Sakala and Zijlstra2014). The generalized characters shared by these genera could correspond to other gymnosperm groups besides Araucariaceae. Although the Triassic fossil record of South America comprises several fossil woods similar to Araucariaceae, their affinities with the family have been questioned because of the absence of other araucariacean macrofossils (e.g., leafy twigs, cones) in the same strata (Panti et al., Reference Panti, Pujana, Zamaloa and Romero2012).
Southern South American Triassic basins are narrow and elongated depressions oriented northwest–southeast and filled by continental sedimentation in Argentina and marine to transitional in Chile (e.g., Charrier, Reference Charrier1979; Spalletti et al., Reference Spalletti, Artabe and Morel2003). The Ischigualasto-Villa Unión Basin, located in west-central Argentina (Fig. 1), has one of the most extended and complete continental Triassic sections in the world (Colombi and Parrish, Reference Colombi and Parrish2008). The Upper Triassic Ischigualasto Formation is one of the upper units of this basin and comprises fluvial sandstone, mudstone, and paleosols (Milana and Alcober, Reference Milana and Alcober1994). This formation is known worldwide for its diverse and exceptionally well-preserved vertebrate assemblage (e.g., Rogers et al., Reference Rogers, Swisher, Sereno, Monetta, Forster and Martínez1993; Martínez et al., Reference Martínez, Fernandez and Alcober2011, Reference Martínez, Fernandez and Alcober2013). However, the paleobotanical studies have not received the same attention, and during recent decades published contributions have been scarce.
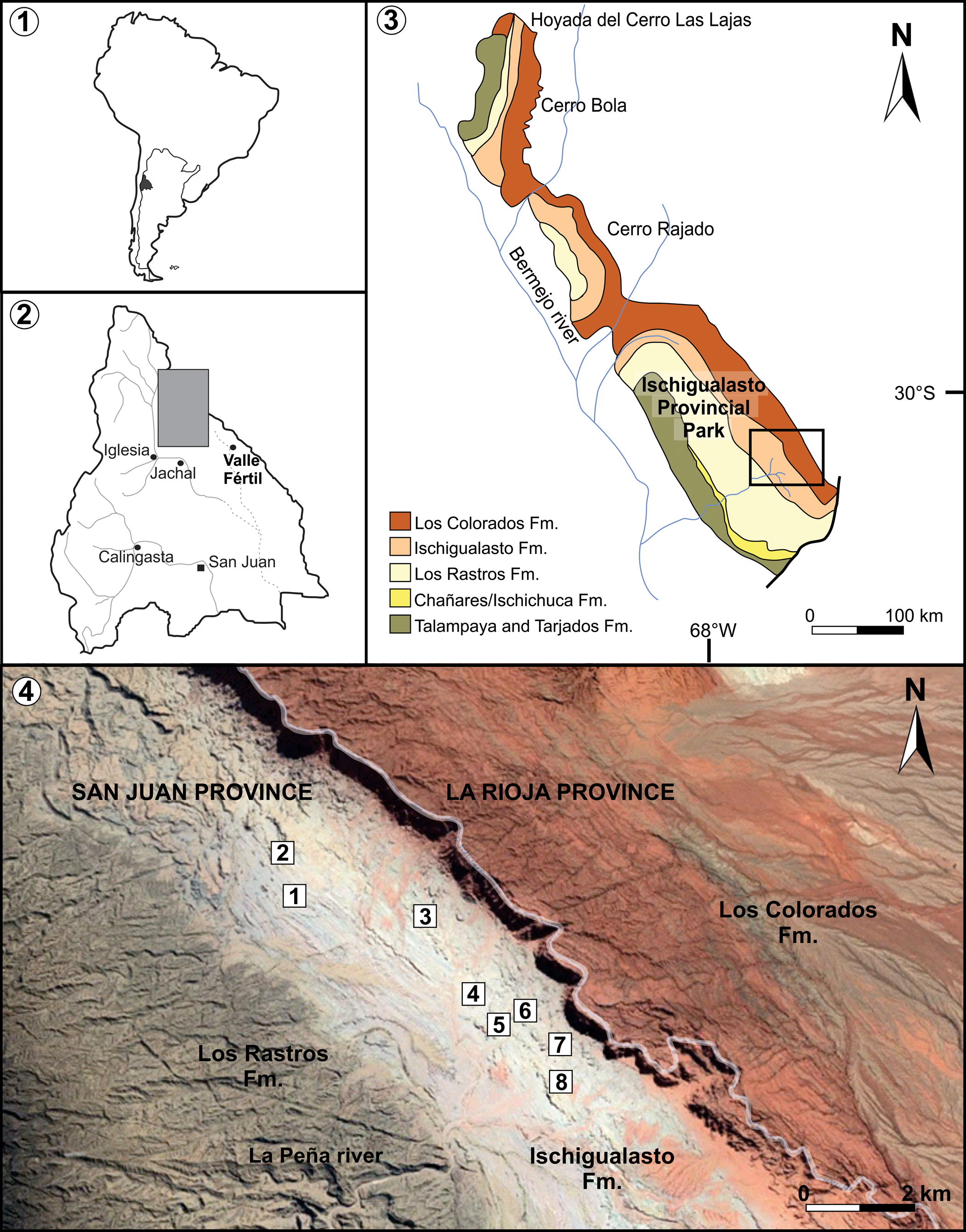
Figure 1. (1) Geographic location of the San Juan Province, at South America and Argentina. (2) Geographic location of the Ischigualasto-Villa Unión Basin at San Juan Province. (3) Geological map of the Ischigualasto-Villa Unión Basin, with the location of the studied area at the Ischigualasto Provincial Park. (4) Outcrops of the Ischigualasto Provincial Park showing the localities with PFLs (1–8). Modified from Colombi et al. (2021). Satellite image taken from Google Earth.
In this work, we describe permineralized araucariaceous woods from the Ischigualasto Provincial Park (San Juan, Argentina), discuss their systematic position, and report the results of a growth-ring analysis. The stratigraphic distribution and taxonomic composition of the plant-fossil-bearing levels (PFLs) were studied to analyze the paleofloristic changes along the Ischigualasto Formation. Furthermore, structures related to the degradation of wood are interpreted and characterized. Finally, the paleoclimatic implications of these findings are evaluated.
Geological setting
The Ischigualasto-Villa Unión Basin is located between ~29°S and ~31°S in northeastern San Juan and southwestern La Rioja provinces, Argentina (Golonka, Reference Golonka2007; Césari and Colombi, Reference Césari and Colombi2016; Fig. 1). This basin corresponds to an extensional half-graben, part of a series of Triassic basins that developed parallel to and cratonward of the proto-Andean arc (Uliana et al., Reference Uliana, Biddle, Cerdan, Tankard and Balkwill1988; Ramos and Kay, Reference Ramos, Kay, Harmon and Rapela1991; López-Gamundí et al., Reference López-Gamundi, Espejo, Conaghan, Powell, Veervers and Powell1994). The filling of the Ischigualasto-Villa Unión Basin began during the late Permian and continued until the Late Triassic and is represented by a 2,500 to >4,000 m thick succession of continental deposits subdivided into a lower red alluvial package of the Talampaya and Tarjados formations and the overlying Agua de la Peña Group (Melchor, Reference Melchor2007; Gulbranson et al., Reference Gulbranson, Ciccioli, Montañez, Marenssi, Limarino, Schmitz and Davydov2015; Césari and Colombi, Reference Césari and Colombi2016). The Agua de la Peña Group comprises, from base to top, the Chañares, Ischichuca, Los Rastros, Ischigualasto, and Los Colorados formations.
The thickness of the Ischigualasto Formation varies between 300 m and 700 m (Currie et al., Reference Currie, Colombi, Tabor, Shipman and Montañez2009); nevertheless, the lack of intraformational unconformities indicates that deposition was relatively continuous (Milana and Alcober, Reference Milana and Alcober1994). The formation includes deposits of fluvial channels characterized by massive and trough cross-stratified sandstones and conglomerates intercalated with finer-grained proximal and distal floodplain facies, mainly sandstones and mudstones (Colombi and Parrish, Reference Colombi and Parrish2008; Currie et al., Reference Currie, Colombi, Tabor, Shipman and Montañez2009; Césari and Colombi, Reference Césari and Colombi2016; Fig. 2.1). The proximal floodplain deposits are composed of fine-grained sandstones and mudstones with horizontal and ripple lamination and are frequently disturbed by paleosol development; the distal floodplain facies consist of massive or finely laminated siltstones and mudstones that are always overprinted by mature paleosol development (Césari and Colombi, Reference Césari and Colombi2016). At the base of the formation there are many ash layers and a basalt (Colombi and Parrish, Reference Colombi and Parrish2008). The Ischigualasto Formation was subdivided into four members (Fig. 2.1), from base to top: La Peña, Cancha de Bochas, Valle de la Luna, and Quebrada de la Sal. These subdivisions are based on variations in the sedimentary architecture and paleosol development (Currie et al., Reference Currie, Colombi, Tabor, Shipman and Montañez2009).
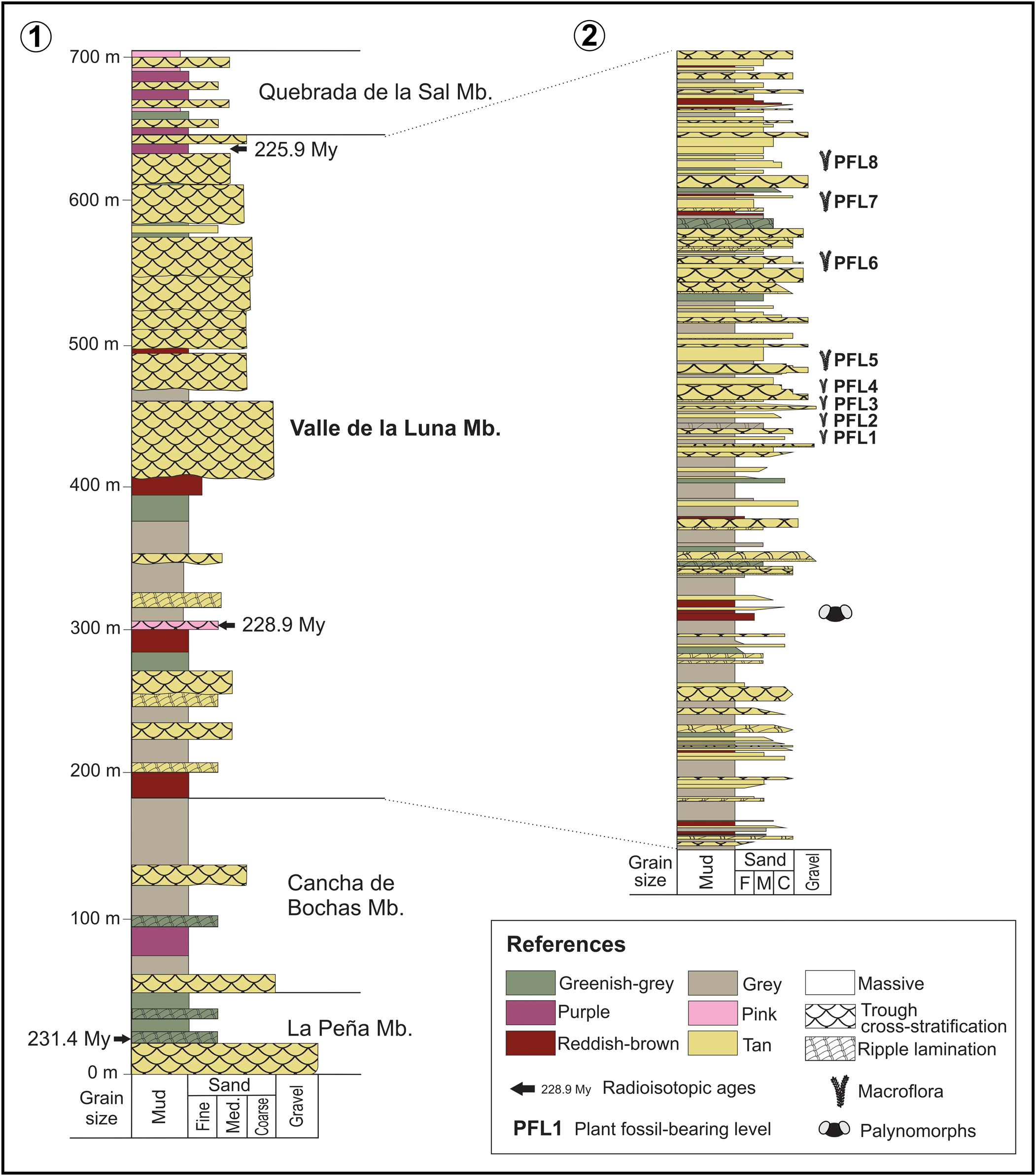
Figure 2. (1) General sedimentological section of the Ischigualasto Formation. (2) Detailed sedimentological section of the Valle de La Luna Member showing the stratigraphic position of the PFLs. Modified from Currie et al. (Reference Currie, Colombi, Tabor, Shipman and Montañez2009).
The Ischigualasto Formation is Carnian to Norian (231.4 to 225.9 Ma) according to radioisotopic information (Rogers et al., Reference Rogers, Swisher, Sereno, Monetta, Forster and Martínez1993; Martínez et al., Reference Martínez, Fernandez and Alcober2011). The Carnian Pluvial Event, also known as Carnian Humid Episode (Ruffell et al., Reference Ruffell, Simms and Wignall2016), was recognized in the underlying Los Rastros Formation at 234.47 ± 0.44 Ma (Dal Corso et al., Reference Dal Corso2020; Mancuso et al., Reference Mancuso, Benavente, Irmis and Mundil2020). The Carnian Pluvial Event was linked to a major extinction event and might have been the trigger to major diversifications and originations of conifers, insects, dinosaurs, crocodiles, lizards, turtles, and mammals on land (Dal Corso et al., Reference Dal Corso2020). The appearance and rapid diversification of dinosaurs in the overlying Ischigualasto Formation represent a major biotic event recorded in the basin (Martínez et al., Reference Martínez, Fernandez and Alcober2011, Reference Martínez, Apaldetti, Alcober, Colombi, Sereno, Fernandez, Santi Malnis, Correa and Abelin2012), corresponding with the Dinosaur Diversification Event (Benton et al., Reference Benton, Bernardi and Kinsella2018). Martínez et al. (Reference Martínez, Fernandez and Alcober2011, Reference Martínez, Apaldetti, Alcober, Colombi, Sereno, Fernandez, Santi Malnis, Correa and Abelin2012) characterized vertebrate biozones and recognized a crucial turnover between the Hyperodapedon–Exaeretodon–Herrerasaurus (HEH) and the Exaeretodon (E) biozones after the Carnian Pluvial Event and before the End-Triassic Extinction. Concerning the Ischigualasto Formation plant assemblages, including impressions, cuticles, and permineralized trunks, they were referred to as the BNP Biozone (Yabeiella brackebuschiana/Scytophyllum neuburgianum/Rhexoxylon piatnitzkyi; Spalletti et al., Reference Spalletti, Artabe, Morel and Brea1999) assigned to the Carnian (Morel et al., Reference Morel, Artabe and Spalletti2003).
Paleobotanical background
The first paleobotanical contribution about Ischigualasto Formation was made by Bodenbender (Reference Bodenbender1911), who indicated the presence of fossil leaves assigned to Thinnfeldia, Cladophlebis mesozoica (Kurtz) Frenguelli, 1947, and Podozamites elongatus (Morris) Feistmantel, Reference Feistmantel1889 and “Araucarites” fossil trunks. Afterward, Frenguelli (Reference Frenguelli1948) published the first detailed study on the stratigraphy, paleontology, and correlation of the Triassic succession that crops out at Ischigualasto Provincial Park. This author presented a list of fossil plants, mentioning the following taxa for the Ischigualasto Formation: Neocalamites carrerei (Zeiller) Halle, Reference Halle1908, Dicroidium lancifolium (Morris) Gothan, Reference Gothan1912, D. odontopteroides (Morris) Gothan, Reference Gothan1912, Desmiophyllum sp. (=Heidiphyllum elongatum (Morris) Retallack, Reference Retallack1981), and “Araucarites” fossil trunks.
In the 1960s, the paleobotanical studies were resumed by Archangelsky (Reference Archangelsky1960, Reference Archangelsky1968), Archangelsky and Brett (Reference Archangelsky and Brett1961, Reference Archangelsky and Brett.1963), Bonetti (Reference Bonetti1966), and Brett (Reference Brett1968), focusing on permineralized trunks and leaf cuticles. Their investigations described new species of great systematic, paleophytogeographic, and biostratigraphic importance (i.e., Rhexoxylon piatnitzkyi Archangelsky and Brett, Reference Archangelsky and Brett1961 emend. Brett, Reference Brett1968 [corystosperm], Michelilloa waltonii Archangelsky and Brett, Reference Archangelsky and Brett.1963 [cycad], and Protojuniperoxylon ischigualastense Bonetti, Reference Bonetti1966 emend. Bodnar and Artabe, Reference Bodnar and Artabe2007 [conifer]) and proposed for the first time that the Dicroidium leaves and Rhexoxylon stems corresponded to the same plant. Petriella (Reference Petriella1978) followed this idea in his whole-plant reconstruction of the tree corystosperm dominant in the Ischigualasto Formation. Baldoni (Reference Baldoni1980) studied leaves with preserved cuticles from that unit and confirmed the occurrence of the corystosperm species Xylopteris argentina (Kurtz) Frenguelli, Reference Frenguelli1943 and X. elongata (Carruthers) Frenguelli, Reference Frenguelli1943.
In addition to the megafloras, the palynofloras of the Ischigualasto-Villa Unión Basin have been studied since the 1970s. Herbst (Reference Herbst1970, Reference Herbst1971) stated that in the Ischigualasto Provincial Park area, well-preserved palynomorphs occur only in the Los Rastros Formation. However, in the Cerro Bola area at La Rioja Province, Yrigoyen and Stover (1970) recognized a palynological assemblage at the base of the Ischigualasto Formation composed of trilete spores and bisaccate, monosulcate pollen grains. In the same stratigraphic level, these authors identified poorly preserved impressions of Podozamites, Yabeiella, Pterophyllum, and Phyllotheca. From this information, Yrigoyen and Stover (Reference Yrigoyen and Stover1970) correlated the lower section of the Ischigualasto Formation with the Quebrada de la Mina Formation and the upper section with the Carrizal Formation; both units crop out at the Marayes-El Carrizal Basin located at the southeast of Ischigualasto-Villa Unión Basin.
Zamuner (Reference Zamuner1992) completed a doctoral thesis on a paleofloristic assemblage from the Ischigualasto Formation at Ischigualasto Provincial Park, in which she analyzed in detail the anatomy and development of Rhexoxylon piatnitzky trunks and rhizomes, peltasperm leaves with preserved cuticles (Lepidopteris stormbergensis (Seward) Townrow, Reference Townrow1956 and Scytophyllum neuburgianum Dobruskina, Reference Dobruskina1969), and conifer wood assigned to a new species of Araucarioxylon. However, only the studies on Scytophyllum neuburgianum were published (Zamuner and Artabe, Reference Zamuner and Artabe1990), while the other results remain unpublished. Later, Zamuner et al. (Reference Zamuner, Zavattieri, Artabe, Morel, Artabe, Morel and Zamuner2001) and Artabe et al. (Reference Artabe, Morel, Spalletti, Artabe, Morel and Zamuner2001) summarized the paleobotanical content and interpreted the preserved paleocommunities of the Ischigualasto Formation, respectively. Bodnar and Artabe (Reference Bodnar and Artabe2007) revised the anatomy of the fossil wood Protojuniperoxylon ischigualastense and analyzed the growth rings, inferring that the species was an evergreen cupressaceous conifer.
Subsequent contributions included taphonomic, paleobiogeographic, and paleoclimatic analyses. The taphonomic characterization of the Ischigualasto Formation paleoflora was carried out by Colombi and Parrish (Reference Colombi and Parrish2008), who defined nine preservation styles (including root impressions, silicified trunks and stumps, cuticles, and palynomorphs) and seven taphofacies. Césari and Colombi (Reference Césari and Colombi2013, Reference Césari and Colombi2016) reported the first Argentinian occurrence of a rich palynofloral assemblage with European Tethys and Northwestern Australia characteristic species (Onslow Microflora) and a dominance of species indicative of humid climatic conditions. Later, Pérez Loinaze et al. (Reference Pérez Loinaze, Vera, Fiorelli and Desojo2018) conducted a cluster analysis of palynological assemblages from Gondwana (including the Chañares, Ischigualasto, and Los Rastros formations), which recovered the Ischigualasto Formation palynoflora as transitional between Ipswich and Onslow subprovinces (Pérez Loinaze et al., Reference Pérez Loinaze, Vera, Fiorelli and Desojo2018). The authors inferred a warm climatic belt that resulted in mixed Onslow–Ipswich microfloras for the Chañares and Ischigualasto formations, while the Los Rastros Formation would correspond to a pure Ipswich microflora of cooler and wetter conditions (Pérez Loinaze et al., Reference Pérez Loinaze, Vera, Fiorelli and Desojo2018).
Materials and methods
Locality information
The four fossil samples studied herein come from the Ischigualasto Provincial Park, San Juan Province, located between 29°56′6″S, 68°10′17″W and 30°12′34″S, 67°49′53″W (Fig. 1). The specimens come from the Valle de La Luna Member, which consists of 250–470 m of mudstone and sandstone. Distal floodplain facies including abandoned channel and swamp deposits are characterized by laminated mudstone deposits intercalated with abundant seams of compressed plant cuticles and abundant palynomorphs (Colombi and Parrish, Reference Colombi and Parrish2008; Currie et al., Reference Currie, Colombi, Tabor, Shipman and Montañez2009; Césari and Colombi, Reference Césari and Colombi2013). At Ischigualasto Provincial Park, Brett (Reference Brett1968) and Zamuner (Reference Zamuner1992) recognized eight localities where fossil plants are abundant. These correspond to different PFLs and were numbered from 1 to 8 according to their stratigraphic position (Fig. 1, Fig. 2.2, Table 1). Following the current stratigraphic scheme, all these levels are located in the top two-thirds of the Valle de La Luna Member of the Ischigualasto Formation. Both lower levels, PFL1 and PFL2, consist of compact layers of black plant compressions with preserved cuticles in a sandstone matrix. The PFLs 3 to 7 correspond to medium to coarse-grained sandstones and gray mudstones, where compressions with cuticles and permineralizations are preserved. The PFL 4 exhibits many large permineralized trunks, while in PFLs 3, 6, and 7, this type of fossil is less abundant. Most of the large permineralized trunks correspond to the corystosperm Rhexoxylon piatnitzkyi and usually consist of the basal part or stump. In PFLs 4, 6, and 7, conifer woods of the species Protojuniperoxylon ischigualastense were found. In PFL 6, together with the large Rhexoxylon piatnitzkyi specimens, a juvenile stem of the same species and fragments of cycad Michelilloa waltonii stems occur. At PFL 8, carbonaceous mudstones are intercalated with tuff beds in which silicified trunks, including those described here, occur in vertical position along with abundant compressions of Neocalamites carrerei and rare impressions of Dicroidium odontopteroides, D. lancifolium, and Desmiophyllum sp. (=Heidiphyllum elongatum) (Frenguelli, Reference Frenguelli1948).
Table 1. Megafossil plant content of the eight PFLs recognized in the Ischigualasto Formation at the Ischigualasto Provincial Park, San Juan Argentina. Information taken from Frenguelli (Reference Frenguelli1948) Archangelsky (Reference Archangelsky1968), Brett (Reference Brett1968), Zamuner (Reference Zamuner1992), and Zamuner et al. (Reference Zamuner, Zavattieri, Artabe, Morel, Artabe, Morel and Zamuner2001). *The informal name of each plant group precedes the formal names of orders and families in brackets.

Fossil study
Four fragments of permineralized wood from trunks preserved in vertical position with respect to the strata (probably in living position) were collected by Joaquín Frenguelli on field trips made during the 1940s. Zamuner (Reference Zamuner1992) studied one of the specimens. All samples are preserved by silica cellular permineralization, with excellent preservation (Fig. 3); only one shows evidence of diagenetic deformation. Although the amorphous form of silica is dominant (Fig. 3.5, 3.6), small quartz crystals occur inside fossil cracks (Fig. 3.7, 3.8).

Figure 3. Agathoxylon argentinum n. sp. (1, 2) General view of the silicified wood, paratype LPPB 495. (3, 4) Surface view of growth rings in transverse section; (3) paratype LPPB 495; (4) holotype LPPB 492. (5, 6) Tracheids in transverse section showing the form of silicification; (5) under plane polarized light; (6) under crossed nicol, paratype LPpm 1375. (7, 8) Transverse section of the secondary xylem showing a crack filled with amorphous silica and microcrystalline quartz; (7) under plane polarized light; (8) under crossed nicol, holotype LPpm 1367. (1, 2) Scale bars = 20 mm; (3) scale bar = 2 mm; (4) scale bar = 5 mm; (5, 6) scale bars = 100 μm; (7, 8) scale bars = 500 μm.
Thin sections (transverse, longitudinal tangential, and longitudinal radial) obtained from the cellulose-acetate peel and polished surface techniques by Frenguelli (Reference Frenguelli1948) and Zamuner (Reference Zamuner1992) were studied. Furthermore, new microscopic slides were prepared by polished surface technique. The thin sections were studied under a Leica DM2500 light microscope and photographed with a Leica DMC2900 camera mounted on the microscope. Furthermore, the minerals were observed and photographed under polarized light with the same microscope. Small fragments were observed, mostly in longitudinal radial view, under JEOL JSM6360 LV scanning electron microscope, with low vacuum, at the electronic microscopy service of the Museo de La Plata, Facultad de Ciencias Naturales y Museo, Universidad Nacional de La Plata. Wood anatomic sections of extant species Araucaria araucana (Molina) K. Koch, Reference Koch1869, A. angustifolia (Bertoloni) Kuntze, Reference Kuntze1898, and A. cunninghamii Aiton ex D. Don in Lambert, Reference Lambert1837 were also observed under transmitted light microscope for comparison.
A minimum of 30 measurements of each anatomical character were made using a ruler installed in the light microscope. The measurements are expressed as the mean followed by the range between parentheses and correspond to the type specimens (holotype and paratype). We calculated percentages of tracheid radial pitting types using the method of Philippe et al. (Reference Philippe, Boura, Oh and Pons2014). The description followed the wood anatomy terminology of IAWA Committee (2004) and the template for the description of fossil tracheidoxyls (Boura et al., Reference Boura, Bamford and Philippe2021). For the nomenclatural and systematic treatment, the International Code of Nomenclature for Algae, Fungi, and Plants (Shenzhen Code, Turland et al., Reference Turland2018) was used, and the classification criterion of Philippe and Bamford (Reference Philippe and Bamford2008) was followed.
The growth rings of the fossil wood were quantitatively analyzed through the method of Falcon-Lang (Reference Falcon-Lang2000a, Reference Falcon-Langb). This method uses the term “ring boundary” to describe the discontinuity between the latewood cells of one ring and the earlywood cells of the following ring, and the term “ring increment” to describe the region between two adjacent ring boundaries. The radial diameters of successive tracheid cells were measured across each ring increment; five adjacent rows of tracheids were measured for each ring increment, and their values were averaged to obtain the final estimate. Using these averages, the cumulative algebraic sum of each cell's deviation from the mean of the tracheid radial diameters was calculated and plotted as a zero-trending curve (CSDM curve; after Creber and Chaloner, Reference Creber and Chaloner1984). For each ring increment, the percentage skew of the zenith of the CSDM curve with respect to the center of the graph was calculated (Falcon-Lang, Reference Falcon-Lang2000a, Reference Falcon-Langb). The percentage diminution (x) in a ring increment was obtained using the following formula: x = (b/a) × 100, where a = maximum cell diameter, and b = maximum cell diameter minus minimum cell diameter. The percentage latewood (y) in each ring increment was calculated using the Creber and Chaloner (Reference Creber and Chaloner1984) method: (y) = (d/c) × 100, where d = number of cells after the CSDM curve turns to zero for the last time, and c = number of cells in each ring increment. The product of these two parameters (x × y/100) provides the Ring Markedness Index (RMI) (Falcon-Lang, Reference Falcon-Lang2000a). Only ring increments derived from mature stems (as indicated by the fact that the growth ring boundaries were nearly straight in transverse section) and formed by continuous uniform growth were included in this study (Falcon-Lang, Reference Falcon-Lang2000a, Reference Falcon-Langb).
Repositories and institutional abbreviations
The four fossil specimens examined in this study are deposited in the collection of the División Paleobotánica of the Museo de La Plata, Facultad de Ciencias Naturales y Museo, Universidad Nacional de La Plata, La Plata, Buenos Aires Province, Argentina, under the acronyms LPPB and LPpm (microscopic slides). The wood sections of extant species analyzed are housed at the didactic xylotheque of the Cátedra de Xilología, also of the Facultad de Ciencias Naturales y Museo, Universidad Nacional de La Plata.
Systematic paleontology
Order Pinales (=Coniferales) Gorozhankin, Reference Gorozhankin1904
Family Araucariaceae Henkel and Hochstetter, Reference Henkel and Hochstetter1865
Genus Agathoxylon Hartig, Reference Hartig1848 sensu Philippe, Reference Philippe1995
Type species
Agathoxylon cordaianum Hartig, Reference Hartig1848, p. 188, by simultaneous publication of a single species name. Triassic, Coburg, Germany.
Agathoxylon argentinum new species
Figures 3–6
- Reference Zamuner1992
Araucarioxylon argentinum Zamuner, p. 57, pl. 24, figs. 1–7.
- Reference Kokogián, Spalletti, Morel, Artabe, Martínez, Alcober, Milana, Zavattieri and Papú1999
Araucarioxylon argentinum; Kokogian et al., p. 395.
- Reference Spalletti, Artabe, Morel and Brea1999
Araucarioxylon argentinum; Spalletti et al., p. 448.
- Reference Zamuner, Zavattieri, Artabe, Morel, Artabe, Morel and Zamuner2001
Araucarioxylon sp. A, Zamuner et al., p. 184.
- Reference Morel, Artabe, Zavattieri, Bonaparte, Artabe, Morel and Zamuner2001
Araucarioxylon sp. A, Morel et al., p. 238.
- Reference Artabe and Zamuner2007
Araucarioxylon sp. A, Artabe et al., p. 82.
- Reference Césari and Colombi2016
Araucarioxylon sp., Césari and Colombi, p. 380.

Figure 4. Agathoxylon argentinum n. sp. (1) General view of the transverse section of the secondary xylem, holotype LPpm 1367. (2) Detail of the growth-ring boundary in transverse section, holotype LPpm 1367. (3) Detail of transverse section of tracheids, paratype LPpm 1375. (4, 5) Tracheid radial pitting, with uniseriate to triseriate, contiguous to compressed and alternating pattern; (4) holotype LPpm 1370; (5) paratype LPpm 1376. (6) Detail of the tracheid radial pitting and the resin plate in a tracheid (arrow), holotype LPpm 1180. (7) Detail of tracheids with truncate terminal walls, holotype LPpm 1181. (8) General view of radial section of the secondary xylem, paratype LPpm 1376. (1) Scale bar = 650 μm; (2) scale bar = 250 μm; (3–5) scale bars = 120 μm; (6) scale bar = 60 μm; (7) scale bar = 70 μm; (8) scale bar = 200 μm.

Figure 5. Agathoxylon argentinum n. sp. (1) General view of the radial section of the secondary xylem showing the ray parenchyma cell types, the cross fields, and resin contents in ray cells, holotype LPpm 1370. (2, 3) Detail of araucarioid cross-field pitting; (2) paratype LPpm 1376; (3) holotype LPpm 1370. (4) General view of tangential section showing the mainly uniseriate rays and occasional biseriate in part rays, paratype LPpm 1377. (5, 6) Detail of rays and tracheid with spiral fissures and resin plates (arrows), holotype LPpm 1372. (7) Leaf trace in tangential section, holotype LPpm 1182. (8) Detail of leaf trace; arrows indicate tracheids. (9) Leaf trace in transverse section; arrows indicate tracheids, LPpm 1380. (1) Scale bar = 100 μm; (2) scale bar = 60 μm; (3) scale bar = 50 μm; (4) scale bar = 450 μm; (5, 6, 8, 9) scale bars = 250 μm; (7) scale bar = 350 μm.

Figure 6. Agathoxylon argentinum n. sp., scanning electron microscope images LPPB 492. (1) General view of the tracheid radial pitting. (2) Detail of biseriate, compressed, and alternating tracheids pits. (3) Detail of uniseriate, compressed, and alternating tracheids pits. (4) Detail of a cross field. (5) Detail of a uniseriate ray in tangential section. (6) Spiral fissures in tracheid tangential walls. (1, 5, 6) Scale bars = 50 μm; (2) scale bar = 60 μm; (3) scale bar = 40 μm; (4) scale bar = 30 μm.
Holotype
LPPB 492 (microscopic slides LPpm 1178–1183, 1365–1373).
Paratype
LPPB 495 (microscopic slides LPpm 1374–1377).
Diagnosis
Tracheidoxyl with slightly distinct to distinct growth rings, with gradual transition from earlywood to latewood. Tracheid pitting in radial walls distinctly bordered, uniseriate to triseriate, with contiguous or compressed and alternating arrangement, very rarely with spaced and subopposite arrangement. Tracheid pitting in tangential walls absent. Frequent resin plates in tracheids. Axial parenchyma absent. Cross fields of araucarioid type, with 2–9 oculipores per field. Homocellular and homogeneous rays, mainly uniseriate, occasionally biseriate in part, medium to high.
Occurrence
Ischigualasto Provincial Park, Ischigualasto-Villa Unión Basin, San Juan Province, Argentina; PFL 8, Valle de la Luna Member, Ischigualasto Formation, Agua de la Peña Group, Upper Triassic (Carnian-Norian)
Description
The studied samples correspond to wood fragments 6–8 cm in diameter with only secondary xylem preserved (Fig. 3.1–3.4). The secondary xylem is homoxylic and pycnoxylic. Growth rings are slightly distinct to distinct, with a gradual reduction of the radial diameter of tracheids toward the ring's outer margin (Fig. 4.1, 4.2). Growth-ring thickness varies from 11 to 81 cells. Secondary xylem tracheids are circular or polygonal (quadrangular to rectangular) in transverse section (Figs. 3.5, 4.1–4.3). The radial diameter of earlywood tracheids is 41.30 (25.61–66.97) μm, and the tangential diameter is 44.30 (27.80–57.35) μm. The earlywood tracheids are thin walled, with a double-wall thickness of 7.25 (3.79–9.54) μm and a radial lumen diameter of 38.47 (21.51–59.17) μm. The radial diameter of latewood tracheids is 22.95 (14.70–29.09) μm, and the tangential diameter of tracheids is 37.97 (26.06–47.20) μm. The latewood tracheids are thin walled, with a double-wall thickness less than 7.37 (4.77–11.06) μm and a radial lumen diameter of 17.87 (9.01–27.83) μm. The number of rows of tracheids between rays is 7 (2–19) (Fig. 4.1–4.3).
Tracheids with bordered pits on radial walls. The pits are uniseriate (27.43%), biseriate (63.28%), and triseriate (9.29%) in the earlywood and exclusively uniseriate in the latewood (Figs. 4.4–4.6, 4.8, 6.1–6.3). Pits in the earlywood have a compressed or contiguous arrangement for the most part (98.23%) and, very rarely, a spaced arrangement (1.77%) (Figs. 4.4–4.6, 4.8, 6.1–6.3). In the latewood, tracheid pits have mainly a compressed or contiguous arrangement (79.17%) and, less frequently, a spaced arrangement (20.83%). Because of fossilization, some contiguous pits may appear spaced (“steinkern” preservation sensu Gothan, Reference Gothan1905). When biseriate or triseriate, pits are mainly alternating (92.07%), rarely subopposite (7.93%) (Figs. 4.4–4.6, 4.8, 6.1–6.3). This pattern belongs to the araucarian type of radial pitting sensu Philippe and Bamford (Reference Philippe and Bamford2008). Uniseriate pits are circular to flattened while biseriate and triseriate pits are circular to hexagonal; in all cases, they show circular apertures. Pits measure 12.98 (9.96–16.16) μm high and 16.26 (12.05–19.740) μm wide. Tracheids lack helical thickenings. Resin plates in tracheids are frequent (Figs. 4.6, 5.5, 5.6). Some tracheids have truncate terminal walls (Fig. 4.7). Spiral fissures, with a spiral angle of about 45° (Figs. 5.6, 6.6), were observed in some tracheids, which could be compression wood.
Cross fields have 2–9 oculipores with an oval outline, placed in one or two opposite to alternating vertical rows, contiguous (Figs. 4.8, 5.1, 5.2, 5.3, 6.4). They measure 6.60 (3.63–10.49) μm in minor diameter and 9.26 μm (5.81 μm–14.51 μm) in major diameter. Pit aperture is horizontal or oblique. Individual pits are of cupressoid type sensu Boura et al. (Reference Boura, Bamford and Philippe2021). Cross fields correspond to the araucarioid type sensu Boura et al. (Reference Boura, Bamford and Philippe2021). As in tracheid pits, some contiguous cross-field pits may appear spaced due to steinkern preservation.
Rays are homocellular and homogeneous with rectilinear trajectory in transverse section, mainly uniseriate (92.77%), occasionally biseriate in part (7.23%), and medium to high, 6 (1–27) cells high (Figs. 5.4–5.6, 6.5). They are composed of procumbent parenchyma cells, 29.68 (18.16 –42.03) μm high and 131.44 (54.25–193.95) μm long (Figs. 4.8, 5.1). Horizontal and end walls of the parenchyma cells are thin, smooth, and straight. The end walls are vertical or in a few cases oblique (Figs. 4.8, 5.1, 5.3). Resin contents are present in ray cells (Fig. 4.8). Axial parenchyma and ray tracheids are absent.
Leaf traces can be recognized. They have a rhomboid outline in tangential view (Fig. 5.7, 5.8). Around the trace, tracheids and xylem rays are curved (Fig. 5.7). The leaf trace consists of parenchyma cells, many of which have resin contents, and tracheids with reticulate thickenings (Fig. 5.9). In the center of the trace, several cells are completely degraded (Fig. 5.7–5.9).
Etymology
The specific name refers to the country where the specimens were collected. This name was chosen by Zamuner (Reference Zamuner1992) when she preliminarily described this species.
Materials
LPPB 499 (microscopic slides LPpm 1378–1380), LPPB 503 (LPpm 1381–1382).
Remarks
Fossil woods with the characters observed in the studied specimens, known as “Araucaria-like anatomy” (i.e., pycnoxylic secondary xylem, araucarian tracheid radial pitting, and araucarioid cross fields), have been assigned to three commonly known genera, Araucarioxylon Kraus, Reference Kraus and Schimper1870, Dadoxylon Endlicher, Reference Endlicher1847, and Agathoxylon Hartig, Reference Hartig1848. Philippe (Reference Philippe1993, Reference Philippe2011) and Bamford and Philippe (Reference Bamford and Philippe2001) pointed out that Araucarioxylon and Dadoxylon are both illegitimate and superfluous synonyms of Pinites Lindley and Hutton, Reference Lindley and Hutton1831, whereas Agathoxylon is a legitimate name having priority over the two other genera. Later, Rößler et al. (Reference Rößler, Philippe, van Konijnenburg-van Cittert, McLoughlin, Sakala and Zijlstra2014) argued for the use of Agathoxylon for fossil woods with Araucaria-like anatomy. Here we treat Agathoxylon as the appropriate genus for this type of fossil wood.
Agathoxylon is a widely distributed wood genus according to a brief list of characteristics that include araucarian tracheid pitting, araucarioid cross-field pits, axial parenchyma present or absent, and mainly uniseriate rays and smooth walls in the parenchyma cells (Hartig, Reference Hartig1848; Philippe, Reference Philippe1995). The studied fossil woods were compared with species of Araucaria-like tracheidoxyls of Mesozoic age from Gondwana to assess their identity (see Table 2). The wood anatomy of Ischigualasto specimens differs from the species of Agathoxylon previously recorded in the Argentinean Triassic—that is, A. amraparense (Sah and Jain, Reference Sah and Jain1964) Crisafulli and Herbst, Reference Crisafulli and Herbst2011, A. cozzoi Gnaedinger and Zavattieri, Reference Gnaedinger and Zavattieri2020, A. dallonii (Boureau, Reference Boureau1948) Crisafulli and Herbst, Reference Crisafulli and Herbst2010, A. lamaibandianus Crisafulli and Herbst, Reference Crisafulli and Herbst2011, and A. protoaraucana (Brea, Reference Brea1997) Gnaedinger and Herbst, Reference Gnaedinger and Herbst2009—because of the presence of resin plates (see Table 2). Among other Mesozoic species, the here described tracheidoxyls show more similarities with Agathoxylon pseudoparenchymatosum (Gothan) Pujana et al., Reference Pujana, Santillana and Marenssi2014, and A. santalense (Sah and Jain, Reference Sah and Jain1964) because of the cupressoid type of individual cross-field pits; the absence of tangential tracheid pitting, septate tracheids, and axial parenchyma; and the presence of resin plates and occasional partially biseriate rays. However, the Ischigualasto fossil wood differs from these species due to the tracheid transverse section (both polygonal and circular in the studied specimens and exclusively polygonal in the other species), the presence of occasional subopposite and spaced tracheid radial pitting in the studied material, and the higher rays (up to 27 cells high in the studied material and up to 10 cells high in the other species), and the number of pits per cross field (up to nine in the Ischigualasto wood and up to six in A. pseudoparenchymatosum and A. santalense) (Table 2). Another difference with A. pseudoparenchymatosum is the presence of triseriate tracheid radial pitting in the studied material. Agathoxylon santalense also can be differentiated from the Ischigualasto wood by the indistinct growth rings in Agathoxylon santalense (Table 2). All these characteristics have taxonomic value in extant conifer woods (García Esteban et al., Reference García Esteban, de Palacios, Guindeo Casasús and García Fernández2004) and are used to delimit Agathoxylon species (see Del Fueyo et al., Reference Del Fueyo, Carrizo, Poiré and Lafuente Díaz2021). Because of these differences, we proposed a new specific taxon for the Ischigualasto material.
Table 2. Comparison among main Mesozoic Agathoxylon species recorded in Gondwana. SGD = stratigraphic and geographic distribution; GR = growth rings; TEL = transition from earlywood to latewood; TS = tracheid section; TTD = tangential diameter of earlywood tracheids (mean value or range when the mean value was not provided); TRPSE = seriation of tracheid radial pitting; TRPA = arrangement of (two or more seriate) tracheid pitting in radial walls; TRPSP = spacing of tracheid radial pitting; TRPD = diameter of tracheid radial pits (mean values or range when the mean value was not provided); TTP = tracheid tangential pitting; TRP = tracheids with resin plates or plugs; ST = septate tracheids; AP = axial parenchyma; RW = ray width; RH = ray height; CP = type of individual cross-field pits; CA = arrangement of cross-field pits; CPN = cross-field pit number; CPD = cross-field pit diameter (mean values or range when the mean value was not provided). Between () characters which appear less frequently. *Information not provided in the species protologue and taken from a subsequent contribution. 1We used the combination Agathoxylon antarticum (Poole and Cantrill, Reference Poole and Cantrill2001) Pujana et al., Reference Pujana, Santillana and Marenssi2014, but we follow the original diagnosis of the basionym provided by Poole and Cantrill (Reference Poole and Cantrill2001). 2 The presence of resin plates is not mentioned in the diagnosis or in the description of Poole and Cantrill (Reference Poole and Cantrill2001), but some probable resin plates are observed in the photos (Poole and Cantrill, Reference Poole and Cantrill2001, pl. 1, fig, 8). 3Agathoxylon dallonni was previously cited in the Upper Triassic of Argentina by Crisafulli and Herbst (Reference Crisafulli and Herbst2010), but the specimens were reassigned to Agathoxylon lamaibandianus by Gnaedinger and Zavattieri (Reference Gnaedinger and Zavattieri2020). 4In the original diagnosis of Boureau (Reference Boureau1948), the cross-field pits are described as simple and in the photos seems to be bordered (Boureau, Reference Boureau1948, pl. 1, fig. 5). 5Although Pujana et al. (Reference Pujana, Santillana and Marenssi2014) proposed that Agathoxylon matildense is a junior synonym of Agathoxylon antarticum, we considered that the protologues of both species show enough differences to segregate them (i.e., tracheid section, the ray height, and the number of pits per cross field, see Poole and Cantrill, Reference Poole and Cantrill2001, p. 1086, pl. 1, 2–10; Zamuner and Falaschi, Reference Zamuner and Falaschi2005, p. 340, fig. 2).

Evidence of fungal activity
Structures related to the degradation of wood by fungi are preserved. It is common to observe resinous contents in the ray cells (Fig. 7.1, 7.2, 7.4). Two types of degradation patterns can be recognized: in some areas of the wood, differential degradation of cellulose and hemicellulose is observed, while lignin is at least partially preserved (Fig. 7.1, 7.2). This pattern is frequently associated with the boundary between earlywood and latewood (Fig. 7.1, 7.2) and is called brown rot (Schwarze et al., Reference Schwarze, Mattheck and Engels2004; Schmidt, Reference Schmidt2006; Schwarze, Reference Schwarze2007). The differential degradation of the polysaccharide layers of the lignified ones can also be observed by the detachment of the S3 layer, which folds toward the lumen of the tracheids (Fig. 7.3). Alternatively, the S3 layer can appear strongly thickened.

Figure 7. Structures related to the degradation of wood by fungi recognized in Agathoxylon argentinum n. sp. (1, 2) Transverse section showing resinous contents in the ray cells and tracheid walls with differential degradation of cellulose and hemicellulose and detached and strongly thickened S3 layer, LPpm 1380. (3) Collapsed tracheids in transverse section with detached S3 layer folded toward the lumen, LPpm 1380. (4) Complete degradation of the cell wall with small groups of spherical structures adhered to the cell wall (white arrow), LPpm 1380. (5) Tracheid walls with detached and strongly thickened S3 layer; white arrow indicates isolated spherical structures, LPpm 1379. (6) Isolated spherical structure adhered to a ray parenchyma cell; white arrow indicates a probable process for anchoring, LPpm 1379. (1) Scale bar = 70 μm; (2) scale bar = 50 μm; (3, 4) scale bars = 80 μm; (5) scale bar = 50 μm; (6) scale bar = 30 μm.

Figure 8. (1–10) Mean radial cell diameter (μm) curves (a and orange line) and CSDM curves (b and blue line) in Agathoxylon argentinum n. sp. from the measured ring increments 1 to 10. Vertical dotted gray line shows the zenith of each CSDM curve.
In turn, areas with completely degraded secondary xylem cells were observed, which is evidenced as empty spaces, sometimes with associated resinous contents (Fig. 7.4). Complete degradation of the cell wall is called white pocket rot (Schwarze et al., Reference Schwarze, Mattheck and Engels2004; Schmidt, Reference Schmidt2006; Schwarze, Reference Schwarze2007).
Although the presence of hyphae was not recognized, structures 3–6 μm in diameter that adhere to the cell wall can be observed in both areas affected by white rot and areas affected by brown rot (Fig. 7.4–7.6). These structures are approximately spherical and appear isolated (Fig. 7.5, 7.6) or in small groups (Fig. 7.4). The walls of these spheres are smooth, and they are surrounded by a thick translucent outer wall. The preservation of the samples did not allow us to observe the presence of openings or discharge papillae, although some of these spheres seem to present some type of process for anchoring (Fig. 7.6).
Growth-ring analysis
In total, 10 ring increments were included in this analysis. The results are summarized in Table 3 and Figure 8. Except for one curve, left-skewed CSDM curves correspond to ring increments smaller than 40 cells in width, while right-skewed curves belong to ring increments greater than 50 cells in width. The percentage skews of the CSDM curve are highly variable, from −27% to +75.6% (mean = +11.5). The percentage diminution ranges from 56.4% to 76.3% (mean = 62.9%). The percentage of latewood varies between 5.4% and 33.3% (mean = 22%). The RMI value varies from 3.2% to 25.4% (mean = 14.1%).
Table 3. Results of growth-ring analysis in Agathoxylon argentinum n. sp. using the Falcon-Lang (Reference Falcon-Lang2000a, Reference Falcon-Langb) method.

Composition of plant fossil assemblages
Fourteen species of plants are present in the Valle de la Luna Member of the Ischigualasto Formation as megafossils (Table 1). These include sphenophytes, corystosperms, and peltasperms and voltzialean, cupressaceous, and araucariaceous conifers. Other groups frequent in the Argentinean Triassic are not present, such as pleuromeialean lycophytes, ferns, petriellaleans, ginkgoaleans, and gnetaleans. The PFLs have low diversity, with one to six species in each one. The level PFL 1 is composed of only corystosperms, while levels PFL 2 and PFL 3 comprise both corystosperms and peltasperms. In PFL 4, cupressaceous conifers are recorded together with fossils of corystosperms and peltasperms. The level PFL 5 differs from the others since it bears exclusively sphenophyte remains. Corystosperms, cycads, and cupressaceous conifers occur in the level PFL 6, whereas only corystosperms and cupressaceous conifers continue at the level PFL 7. Finally, the PFL 8 consists of taxa that are not recorded in the previous levels (i.e., the corystosperm species Dicroidium lancifolium and D. odontopteroides and voltzialean, cupressaceous, and araucariaceous conifers) together with the sphenophyte Neocalamites carrerei, also present in PFL 5.
Discussion
Systematic affinities
It has been suggested that several Paleozoic and Mesozoic plant groups may have an Agathoxylon type of wood: seed ferns, cordaitaleans, and araucarian and cheirolepid conifers (Philippe et al., Reference Philippe2004; Taylor et al., Reference Taylor, Taylor and Krings2009; Degani-Schmidt and Guerra-Sommer, Reference Degani-Schmidt and Guerra-Sommer2016). Besides the fact that Glossopteridales and Cordaitales became extinct at the Permian–Triassic boundary (see Bodnar et al., Reference Bodnar, Coturel, Falco and Beltrán2021), the new species show dissimilarities with the wood anatomy of these Paleozoic lineages. According to Noll et al. (Reference Noll, Rößler and Wilde2005), cordaitalean wood is characterized by predominant multiseriate tracheid radial pitting (with three to five pit series) and very subordinate uni- and biseriate pitting, in contrast to Agathoxylon argentinum n. sp., which shows a dominance of the uni- and biseriate tracheid pitting. Recently, most fossil woods of Agathoxylon type related to glossopterids were classified as or reassigned to the genus Australoxylon (see Gulbranson et al., Reference Gulbranson, Ryberg, Decombeix, Taylor, Taylor and Isbell2014; Bamford, Reference Bamford, Linol and de Wit2016; Decombeix et al., Reference Decombeix, Taylor and Taylor2016; Bamford et al., Reference Bamford, Cairncross and Lombard2020). The main character distinguishing Australoxylon from Agathoxylon is the presence of mixed-type pitting on the tracheid radial walls, whereas Agathoxylon has only araucarian radial pitting (Merlotti and Kurzawe, Reference Merlotti and Kurzawe2006; Gulbranson et al., Reference Gulbranson, Ryberg, Decombeix, Taylor, Taylor and Isbell2014; Bamford et al., Reference Bamford, Cairncross and Lombard2020). For its part, the secondary xylem of Mesozoic seed fern groups, such as Hermanophytales, Pentoxylales, and Umkomasiales (=Corystospermales), can be differentiated from Agathoxylon by mixed type of tracheid pitting in Hermanophytales and most Umkomasiales, and cross fields with oopores or oculipores of podocarpoid type in Pentoxylales and Umkomasiales (see Arnold, Reference Arnold1962; Sharma, Reference Sharma1969, Reference Sharma1972; Bose et al., Reference Bose, Pal and Harris1985; Artabe and Brea, Reference Artabe and Brea2003; Artabe and Zamuner, Reference Artabe and Zamuner2007; Bodnar, Reference Bodnar2008, Reference Bodnar2012; Decombeix et al., Reference Decombeix, Bomfleur, Taylor and Taylor2014). In the case of Cheirolepidiaceae, three types of fossil woods have been related to the family—Protocupressinoxylon, Protopodocarpoxylon, and Brachyoxylon (Harris, Reference Harris1979; Alvin et al., Reference Alvin, Fraser and Spicer1981; Zhou, Reference Zhou1983), all of them characterized by mixed tracheid pitting (Axsmith, Reference Axsmith2006; Bodnar et al., Reference Bodnar, Escapa, Cúneo and Gnaedinger2013) and not araucarian pitting as Agathoxylon.
Compared with extant conifer woods, the new species has a character combination present only in Araucariaceae, allowing us to assign it to this family, that is, presence of frequent resin plates in tracheids; compressed, polygonal, alternating, uniseriate to triseriate tracheid radial pits; absence of helical tertiary thickenings, Sanio's bars, and axial parenchyma (although this last character may be present in Araucariaceae, it is rare); and araucarioid cross fields with more than four pits (García Esteban et al., Reference García Esteban, de Palacios, Guindeo, García-Esteban, Lázaro, González, Rodriguez, García, Bobadilla and Camacho2002, Reference García Esteban, de Palacios, Guindeo Casasús and García Fernández2004; IAWA Committee, 2004; Table 4). In particular, the presence of resin plates or plugs in the tracheids is characteristic of the Araucariaceae (García Esteban et al., Reference García Esteban, de Palacios, Guindeo, García-Esteban, Lázaro, González, Rodriguez, García, Bobadilla and Camacho2002, Reference García Esteban, de Palacios, Guindeo Casasús and García Fernández2004; IAWA Committee, 2004). They are plate-like structures generally darker in color than the cell walls, which extend fully across the tracheid and are associated with adjacent rays (Heady et al., Reference Heady, Banks and Evans2002). Resin deposits in tracheids were cited occasionally for a few species of Pinaceae (Penhallow, Reference Penhallow1904; Record, Reference Record1918; Kukachka, Reference Kukachka1960; García Esteban et al., Reference García Esteban, de Palacios, Guindeo and Fernández2007, Reference García Esteban, de Palacios, García-Iruela, García-Fernández, García-Esteban and González de Vega2021), but it is not considered as diagnostic for that family (García Esteban et al., Reference García Esteban, de Palacios, García-Iruela, García-Fernández, García-Esteban and González de Vega2021). However, resin plates or resinous tracheids were mentioned for some Paleozoic woods with Cordaitalean or uncertain affinities, but in most cases, they would not correspond to the same structures as those present in Araucariaceae woods. For example, the “meniscus-like” deposits in the tracheids described in Dadoxylon brandlingii (Lindley and Hutton) Frentzen, Reference Frentzen1931 (Thomson, Reference Thomson1914, pl. 5, fig. 46) seem to be septa (i.e., transverse dividing walls inside the cells, Beck, Reference Beck2010), while the resin plates cited for Podocarpoxylon indicum (Bhardwaj) Bose and Maheshwari, Reference Bose, Maheshwari, Surange, Lakhanpal and Bhardwaj1974 (Crisafulli and Herbst, Reference Crisafulli and Herbst2008, fig. 10.2) and Agathoxylon kurmarpurensis (Bajpai and Singh) Crisafulli and Herbst, Reference Crisafulli and Herbst2008 (Crisafulli et al., Reference Crisafulli, Herbst and Storti2009, fig. 3.E) are not plate-like deposits since they filled whole tracheid cell lumen.
Table 4. Comparison among Agathoxylon argentinum n. sp. and the wood of extant Araucariaceae. GD = stratigraphic and geographic distribution; GR = growth rings; TEL = transition from earlywood to latewood; TS = tracheid section; TTD = tangential diameter of earlywood tracheids (mean values); TRPSE = seriation of tracheid radial pitting; TRPA = arrangement of (two or more seriate) tracheid pitting in radial walls; TRPSP = spacing of tracheid radial pitting; TRPD = diameter of tracheid radial pits (mean values); TTP = tracheid tangential pitting; TRP = tracheids with resin plates or plugs; ST = septate tracheids; AP = axial parenchyma; RW = ray width; RH = ray height; CP = type of individual cross–field pits; CA = arrangement of cross–field pits; CPN = cross–field pit number; CPD = cross–field pit diameter (mean values). *Measurements from the photo provided by Heady et al. (Reference Heady, Banks and Evans2002; fig. 13). Characters that appear occasionally are in parentheses. Information taken from Greguss (Reference Greguss1955), García Esteban et al. (Reference García Esteban, de Palacios, Guindeo, García-Esteban, Lázaro, González, Rodriguez, García, Bobadilla and Camacho2002, Reference García Esteban, de Palacios, Guindeo Casasús and García Fernández2004), Heady et al. (Reference Heady, Banks and Evans2002), IAWA Committee (2004), Farjon (Reference Farjon2010), http://insidewood.lib.ncsu.edu/search (2004 onward), https://www.gbif.org, and this work.

Although araucarian leaves and cones have not been found in the Ischigualasto Formation until today, fossil pollen grains often related to the Araucariaceae occur in the same strata (i.e., Araucariacites australis Cookson, Reference Cookson1947) (Césari and Colombi, Reference Césari and Colombi2016; Gnaedinger and Zavattieri, Reference Gnaedinger and Zavattieri2020), which supports the affinity of the studied fossil woods. When compared with the living species of Araucariaceae, the new species does not show a preferential affinity. Agathoxylon argentinum n. sp. shares the presence of resin plates in tracheids with Agathis australis (D. Don) Lindley in Loudon, Reference Loudon1829, Agathis macrophylla (Lindley) Masters, Reference Masters1892, Agathis ovata (Vieillard) Warburg, Reference Warburg1900, Agathis robusta (C. Moore ex F. Mueller) Bailey, Reference Bailey1883, Araucaria angustifolia, Araucaria montana Brongniart and Gris, Reference Brongniart and Gris1871, Araucaria muelleri (Carrière) Brongniart and Gris, Reference Brongniart and Gris1871, Araucaria rulei Mueller, Reference Mueller1860, and Wollemia nobilis Jones et al., Reference Jones, Hill and Allen1995 (Greguss, Reference Greguss1955; García Esteban et al., Reference García Esteban, de Palacios, Guindeo, García-Esteban, Lázaro, González, Rodriguez, García, Bobadilla and Camacho2002, Reference García Esteban, de Palacios, Guindeo Casasús and García Fernández2004; Heady et al., Reference Heady, Banks and Evans2002; http://insidewood.lib.ncsu.edu/search [2004 onward]; Table 4). However, the average tangential diameter of earlywood tracheids of the new species is similar to that of Agathis lanceolata Warburg, Reference Warburg1900, Agathis macrophylla, Araucaria columnaris (G. Forster) Hooker, Reference Hooker1852, and Araucaria montana (Greguss, Reference Greguss1955; García Esteban et al., Reference García Esteban, de Palacios, Guindeo, García-Esteban, Lázaro, González, Rodriguez, García, Bobadilla and Camacho2002, Reference García Esteban, de Palacios, Guindeo Casasús and García Fernández2004; Table 4). The ray height of Araucaria cunninghamii and Araucaria hunsteinii Schumann in Schumann and Hollrung, Reference Schumann and Hollrung1889 wood is comparable to that of the new fossil taxon (Greguss, Reference Greguss1955; García Esteban et al., Reference García Esteban, de Palacios, Guindeo, García-Esteban, Lázaro, González, Rodriguez, García, Bobadilla and Camacho2002, Reference García Esteban, de Palacios, Guindeo Casasús and García Fernández2004; Table 4). Finally, the cross fields of Agathoxylon argentinum n. sp. resemble those of Araucaria angustifolia, Araucaria araucana, Araucaria bidwillii Hooker, Reference Hooker1843, and Araucaria heterophylla (Salisbury) Franco, Reference Franco1952 because of the pit number per field (Greguss, Reference Greguss1955; García Esteban et al., Reference García Esteban, de Palacios, Guindeo, García-Esteban, Lázaro, González, Rodriguez, García, Bobadilla and Camacho2002, Reference García Esteban, de Palacios, Guindeo Casasús and García Fernández2004; Table 4).
Leaf phenology implication
Quantitative analysis of growth rings, including CSDM curves and other parameters (percentage diminution, percentage latewood, and RMI), have proven to be powerful tools in understanding tree growth and leaf longevity as well as climate and environment (Falcon-Lang, Reference Falcon-Lang2000a, Reference Falcon-Langb). The CSDM method has been previously applied to three fossil wood species from the Triassic of Argentina: Elchaxylon zavattierae Artabe and Zamuner, Reference Artabe and Zamuner2007 (Upper Triassic Río Blanco Formation, Mendoza Province), Protojuniperoxylon ischigualatense (from same strata where the here studied species occurs, Upper Triassic Ischigualasto Formation, San Juan Province), and Agathoxylon protoaraucana (Middle Triassic, Paramillo Formation, Mendoza Province). All of them were interpreted as evergreen plants (Artabe et al., Reference Artabe, Morel and Ganuza2007; Bodnar and Artabe, Reference Bodnar and Artabe2007; Brea et al., Reference Brea, Artabe and Spalletti2008).
Unlike the other Argentinean Triassic species, Agathoxylon argentinum n. sp. shows both left-skewed and right-skewed CSDM curves (Fig. 8, Table 5); left-skewed curves correspond mostly to ring increments smaller than 40 cells. In narrow rings, values of percentage latewood are usually anomalously high compared with wide rings in the same tree (Creber and Chaloner, Reference Creber and Chaloner1984), and this can explain our results. In general, the new species exhibits lower values of percentage diminution, percentage latewood, and RMI than the other Argentinean Triassic species (Table 5) because of the poorly defined growth rings observed in the studied specimens. The ring parameter values obtained in Agathoxylon argentinum n. sp. are comparable to those of extant evergreen conifers with leaf retention of one to two years (Table 5).
Table 5. Comparison of growth-ring parameters of Agathoxylon argentinum n. sp. with other Triassic species from Argentina (*) and selected extant conifer species. Mean values in parentheses. Information taken from Falcon-Lang (Reference Falcon-Lang2000a, Reference Falcon-Lang2000b), Artabe et al. (Reference Artabe, Morel and Ganuza2007), Bodnar and Artabe (Reference Bodnar and Artabe2007), and Brea et al. (Reference Brea, Artabe and Spalletti2008).

Paleofloristic changes
Spalletti et al. (Reference Spalletti, Artabe, Morel and Brea1999) recognized five assemblage biozones (Dictyophyllum castellanosii/Johnstonia stelzneriana/Saportaea dichotoma [CSD] Biozone, Yabeiella mareyesiaca/Scytophyllum bonettiae, Rhexoxylon (=Protophyllocladoxylon) cortaderitaense [MBC] Biozone, Yabeiella brackebuschiana/Scytophyllum neuburgianum/Rhexoxylon piatnitzkyi [BNP] Biozone, Dicroidium odontopteroides/D. lancifolium [OL] Biozone, and Dictyophyllum tenuiserratum, Linguifolium arctum, Protocircoporoxylon marianaensis [DLM] Biozone) in the Triassic System of Argentina based mainly on associations of characteristic plant taxa. In PFLs 1–7 of the Ischigualasto Formation, species diagnostics of the early Late Triassic (Carnian) Biozone Yabeiella brackebuschiana/Scytophyllum neuburgianum/Rhexoxylon piatnitzkyi (BNP) are recognized. However, the species present in the PFL 8 level are more related to the middle Late Triassic (Norian) Biozone Dicroidium odontopteroides-D. lancifolium (OL) (Spalletti et al., Reference Spalletti, Artabe, Morel and Brea1999; Morel et al., Reference Morel, Artabe and Spalletti2003). Among other floristic events, the OL Biozone is characterized by the dominance of shrubby corystosperms and a decline in diversity of tree corystosperms as the genus Zuberia (Spalletti et al., Reference Spalletti, Artabe, Morel and Brea1999).
Paleoenvironmental and paleoclimatic considerations
The presence of compression wood in one of the studied samples (LPPB 492) suggests that it could be a stem growing on a hillside. As the growth ring boundaries are nearly straight in transverse section in the sample and it is a fragment of the main trunks preserved in vertical position, we infer that it was not a tree branch.
Both white and brown rot are the product of the activity of basidiomycetes fungi (Schwarze et al., Reference Schwarze, Mattheck and Engels2004; Schmidt, Reference Schmidt2006; Schwarze, Reference Schwarze2007). Although no hyphal remains were observed in the studied materials, the degradation patterns are comparable to other examples of interactions between basidiomycetes fungi and Araucariaceae woods (e.g., Sagasti et al., Reference Sagasti, García Massini, Escapa and Guido2018 and citations therein). The spherical structures attached to the walls of the tracheids are comparable to holocarpic chytrids (Taylor et al., Reference Taylor, Krings and Taylor2015), whose parasitic activity has been suggested in vascular plants since the Devonian (e.g., Stubblefield et al., Reference Stubblefield, Taylor and Beck1985; Taylor et al., Reference Taylor, Remy and Hass1992; Hass et al., Reference Hass, Taylor and Remy1994; Krings et al., Reference Krings, Dotzler, Galtier and Taylor2009; Sagasti et al., Reference Sagasti, García Massini, Escapa and Guido2018). Members of Chytridiomycota are saprobes or parasites in or on organisms (Ogawa et al., Reference Ogawa, Hayashi, Degawa and Yaguchi2001; Hurdeal et al., Reference Hurdeal, Gentekaki, Hyde and Jeewon2020). Chytrids are known to live on pollen grains, insect exoskeletons, protists, small invertebrates, amphibian skin, other fungi, pieces of plants, fruits, and water-logged twigs (Boyle et al., Reference Boyle, Hyatt, Daszak, Berger, Longcore, Porter, Hengstberger and Olsen2003). Fungal degradation of wood and the presence of probable chytrids would indicate at least locally humid conditions (Schmidt, Reference Schmidt2006; Engelund et al., Reference Engelund, Thygesen, Svensson and Hill2013; Thybring et al., Reference Thybring, Kymäläinen and Rautkari2018).
The palynoflora studied by Césari and Colombi (Reference Césari and Colombi2016) from a level lower than the megaflora levels presents an association linked to humid conditions. PFLs 1–3 are composed exclusively of corystosperms and peltasperms, both considered xerophytic plants, which could show a change to drier conditions. At PFLs 4, 6, and 7, woods of Cupressaceae are recorded together with fossils of corystosperms and peltasperms. Cupressaceae grow in a wide variety of environments, but in general, they are xerophytic plants—with xeromorphic adaptations—that can live in arid or semi-arid environments or oligotrophic soils (Scagel, Reference Scagel1987). In PFL 8, Araucariaceae occurs, Cupressaceae and peltasperms are not recorded, and the large tree corystosperms are replaced by shrubby corystosperms. Members of the Araucariaceae family are currently restricted to the South American and Southwest Asia–Western Pacific region despite their extensive distribution in both hemispheres during the Mesozoic (Stockey, Reference Stockey, Taylor and Taylor1990; Kunzmann, Reference Kunzmann2007). They include three living genera, Araucaria, Agathis, and Wollemia, mostly distributed in tropical and subtropical humid regions (e.g., Kershaw and Wagstaff, Reference Kershaw and Wagstaff2001; Farjon, Reference Farjon2010). The history of the family indicates that araucarians prospered and became widespread forest dominants during periods of low fire frequency and generally wet climate (Kershaw and Wagstaff, Reference Kershaw and Wagstaff2001). Therefore, we infer a change to more humid climates for the PFL 8 level.
Conclusions
The studied fossil woods from the Triassic Ischigualasto Formation are assigned to a new species since they present anatomical differences of taxonomic importance from the other species of Agathoxylon recorded in Mesozoic strata from Gondwana. Agathoxylon argentinum n. sp. is characterized by uniseriate to triseriate radial tracheid pitting with mainly contiguous or compressed and alternating arrangement, frequent resin plates, cross fields of araucarioid type with 2–9 oculipores per field, uniseriate to occasional partially biseriate rays up to 27 cells high, and the absence of tangential tracheid pitting, septate tracheids, and axial parenchyma. This species is assigned to Araucariaceae because of the combination of uniseriate to triseriate alternating contiguous and compressed radial tracheid pitting, the presence of resin plates in tracheids, and araucarioid cross fields. These characters differentiate woods of Araucariaceae from those of other Mesozoic gymnosperms. Wood samples show evidence of moderate biodeterioration. Wood-decaying patterns comparable to the activity of basidiomycetes is recognized as white and brown rot. Moreover, spherical structures attached to the walls of the tracheids were recognized, which are interpreted as holocarpic Chytridiomycetes fungi that could have had parasitic or saprotrophic modes of life.
Quantitative growth-ring analysis results lead us to infer that Agathoxylon argentinum n. sp. was an evergreen gymnosperm with a low leaf-retention time. The fossil levels PFL 1–7 of the Ischigualasto Formation bear a floristic assemblage characteristic of the Carnian. Agathoxylon argentinum n. sp. occurs in the fossil level PFL 8, whose plant-fossil assemblage is typical of the Norian. The vegetation change recorded in the fossil level bearing the new species, in addition to evidence of fungal activity, indicates humid paleoclimatic conditions for the uppermost part of the Valle de La Luna Member of the Ischigualasto Formation.
Acknowledgments
We especially thank A. Zamuner (1959–2012), who generously invited the authors of this work (J.B. and A.J.S.) to finish the research that she had begun in her Ph.D. thesis. We also thank P. Sarmiento (Museo de La Plata, Facultad de Ciencias Naturales y Museo, Universidad Nacional de La Plata, Argentina) for preparing the materials and taking the pictures of fossils under SEM. This manuscript improved significantly due to the remarks and suggestions made by E. Vera, an anonymous reviewer, and N. Jud (associate editor). This study was supported by the Agencia Nacional de Promoción Científica y Tecnológica (PICT 2014-2751) and Universidad Nacional de La Plata (Projects N686, N807).