Introduction
Human activities throughout the ages have led to dramatic alterations of the environment, but never as marked as in the 20th and 21st centuries (Behnke et al., Reference Behnke, Harris, Bajer, Barnard, Sherif and Cliffe2004). In many developing countries, unplanned growth in urban centers leads to the emergence of precarious dwellings in areas that are unsuitable for civil construction; this does not foster the practice of good hygiene habits and contributes to the deterioration of environmental conditions. Urbanization processes and problems related to the disposal of solid waste, drainage of rainwater, and sewage collection and treatment have become serious and almost uncontrollable issues (de Masi et al., Reference de Masi, Vilaça and Razzolini2009).
The disturbances created by city growth destroy the habitats of native species and create habitats for the relatively few species that can adapt to urban and suburban conditions, favored by the stable availability of food and shelter (Chace & Walsh, Reference Chace and Walsh2006; Bradley & Altizer, Reference Bradley and Altizer2007; Cavia et al., Reference Cavia, Cueto and Suárez2009). Clear examples of such invasive synanthropic species are the urban rodents: the Norway rat (Rattus norvegicus Berkenhput, 1769) and the black rat (Rattus rattus Linnaeus, 1758), originally from Southeast Asia and North Africa/West Asia, respectively, and the house mouse (Mus musculus Linnaeus, 1758), originally from Southeast Asia (Lobos et al., Reference Lobos, Ferres and Palma2005; Nagorsen, Reference Nagorsen2005; Bonnefoy et al., Reference Bonnefoy, Kampen and Sweeney2008; Puckett et al., Reference Puckett, Park, Combs, Blum, Bryant, Caccone and Himsworth2016). Many aspects of their biology, such as an enormous reproductive potential, their feeding behavior and adaptation to urban environments, contribute to the success of their worldwide invasion (Cavia et al., Reference Cavia, Cueto and Suárez2009; Himsworth et al., Reference Himsworth, Parsons, Jardine and Patrick2013; Vadell et al., Reference Vadell, Villafañe and Cavia2014; Kosoy et al., Reference Kosoy, Khlyap, Cosson and Morand2015).
Studies involving urban rodents are scarce compared to their importance to public health and there is a lack of interest in studies carried out on widely distributed species when the contributions are regional. However, this information is valuable for understanding the behavior of diseases transmitted by rodents to humans and animals. A large number of pathogens and parasite species utilize rodents to fulfill part of their life cycle (Battersby et al., Reference Battersby, Parsons and Webster2002; Milazzo et al., Reference Milazzo, de Bellocq, Cagnin, Casanova, Di Bella, Feliu and Santalla2003; Easterbrook et al., Reference Easterbrook, Kaplan, Vanasco, Reeves, Purcell and Kosoy2007; Meerburg et al., Reference Meerburg, Singleton and Kijlstra2009; Hancke & Suárez, Reference Hancke and Suárez2017; Panti-May et al., Reference Panti-May, Digiani, Palomo-Arjona, Gurubel-González, Navone, Williams and Robles2018). Among the most common helminth species recorded are Capillaria hepatica (Bancroft, 1893), Heterakis spumosa Schneider, 1866, Nippostrongylus brasiliensis Travassos, Reference Travassos1914, Hymenolepis diminuta Rudolphi, 1819, Rodentolepis nana (Von Siebold, 1852) and Hidatiguera taeniaeformis (Batsch, 1786), some of which present health risks (Landaeta-Aqueveque et al., Reference Landaeta-Aqueveque, Robles and Cattan2007; Kataranovski et al., Reference Kataranovski, Mirkov, Belij, Popov, Petrović, Gačić and Kataranovski2011; Zain et al., Reference Zain, Behnke and Lewis2012; Fitte et al., Reference Fitte, Robles, Dellarupe, Unzaga and Navone2017). Protozoa parasites with health implications, such as Giardia muris Blanchard, 1888, Neospora caninum Dubey et al., 1988, and Toxoplasma gondii Nicolle & Manceaux, 1908 (Franjola et al., Reference Franjola, Soto and Montefusco1995; Muradian et al., Reference Muradian, Ferreira, Lopes, de Oliveira Esmerini, de Jesus Pena, Soares and Gennari2012; Seifollahi et al., Reference Seifollahi, Sarkari, Motazedian, Asgari, Ranjbar and Abdolahi Khabisi2016) have also been recorded, as well as many bacteria that affect health, such as Leptospira spp. Noguchi 1917 and Yersinia pestis Lehmann & Neumann, 1896 (Meerburg et al., Reference Meerburg, Singleton and Kijlstra2009; Himsworth et al., Reference Himsworth, Parsons, Jardine and Patrick2013).
Identifying and understanding the biology of rodent-associated parasites and pathogens, and their relationship with internal and/or external factors which favor their presence, is fundamental for the prevention of severe disease in humans and domestic animals (Bordes et al., Reference Bordes, Blasdell and Morand2015). Some helminths and protozoa species (e.g., T. gondii, Hymenolepis spp.) are worrying because they are not subject to mandatory reporting (Lykins et al., Reference Lykins, Wang, Wheeler, Clouser, Dixon, El Bissati and McLeod2016; Dellarupe et al., Reference Dellarupe, Fitte, Pardini and Campero2019). Also, the symptoms caused by some pathogens (e.g., Leptospira spp, Hantavirus spp.) are often confused with other common pathologies, leading to serious cases and even death when they are diagnosed late (Ministerio de Salud de la Nación, 2014).
The richness and composition of parasite assemblages depends on a series of factors related to the host species, their geographic range, body size, population density, social behavior and diet (Morand & Poulin, Reference Morand and Poulin1998; Arneberg, Reference Arneberg2002; Poulin, Reference Poulin2004; Poulin & George-Nascimento, Reference Poulin and George-Nascimento2007), as well as variations in environmental conditions that operate as specific sources of selection pressures (Behnke et al., Reference Behnke, Harris, Bajer, Barnard, Sherif and Cliffe2004; Deter et al., Reference Deter, Chaval, Galan, Berthier, Salvador and Garcia2007). In urban areas, structural and environmental conditions play a fundamental role in relation to the presence of rodents and the associated parasite fauna (Traweger & Slotta-Bachmayr, Reference Traweger and Slotta-Bachmayr2005; Traweger et al., Reference Traweger, Travnitzky, Moser, Walzer and Bernatzky2006; Cavia et al., Reference Cavia, Cueto and Suárez2009; Hancke et al., Reference Hancke, Navone and Suarez2011), and these become a threat to human health due to the proximity in which people, domestic animals and rodents coexist (Himsworth et al., Reference Himsworth, Parsons, Jardine and Patrick2013; Rothenburger et al., Reference Rothenburger, Himsworth, Nemeth, Pearl and Jardine2017). Moreover, community interactions in pathogen transmission are being increasingly studied to understand the multi-host ecology of zoonotic pathogens, since most infectious agents circulate in communities of hosts infected with multiple parasites (Johnson et al., Reference Johnson, De Roode and Fenton2015; Hassell et al., Reference Hassell, Begon, Ward and Fèvre2017). Changes in the community structure, including pathogens and hosts, have epidemiological consequences (Telfer et al., Reference Telfer, Lambin, Birtles, Beldomenico, Burthe, Paterson and Begon2010; Johnson et al., Reference Johnson, De Roode and Fenton2015).
The aim of this study was to record the parasite and pathogen fauna present in the three species of urban rodents in seven neighborhoods of the city of Gran La Plata, province of Buenos Aires, Argentina, analyzing the relation of parasite and pathogen infection with host characteristics, host community structure and environmental characteristics.
Material and methods
Study area
The study was conducted in the La Plata and Berisso departments in the area of Gran La Plata, in the north-east of the province of Buenos Aires, Argentina. Sampling was carried out in seven neighborhoods; five peripheral to La Plata (fig. 1): Malvinas Argentinas (34.5643°S, 58.0036°W), La Isla (34.5328°S, 57.5925°W), El Retiro (34.5751°S, 58.0017°W), La Latita (34.5831°S, 57.5830°W) and Abasto (34.5805°S, 58.0147°W); one peripheral to Berisso: El Carmen (34.5533°S, 57.5309°W); and one belonging to the inner city of La Plata: Casco Urbano (34.5516°S, 57.5716°W). These neighborhoods present different levels of urbanization. El Carmen, El Retiro, La Latita and Malvinas Argentinas are characterized by poor environmental and structural features such as inadequate garbage removal, lack of a sanitation network and potable water, with areas susceptible to flooding and with domestic animals without sufficient care. El Retiro, El Carmen and La Isla neighborhoods are crossed by highly contaminated streams. Abasto is situated in a rural area, with important agricultural activity. Casco Urbano, being part of La Plata city center, presents all services.
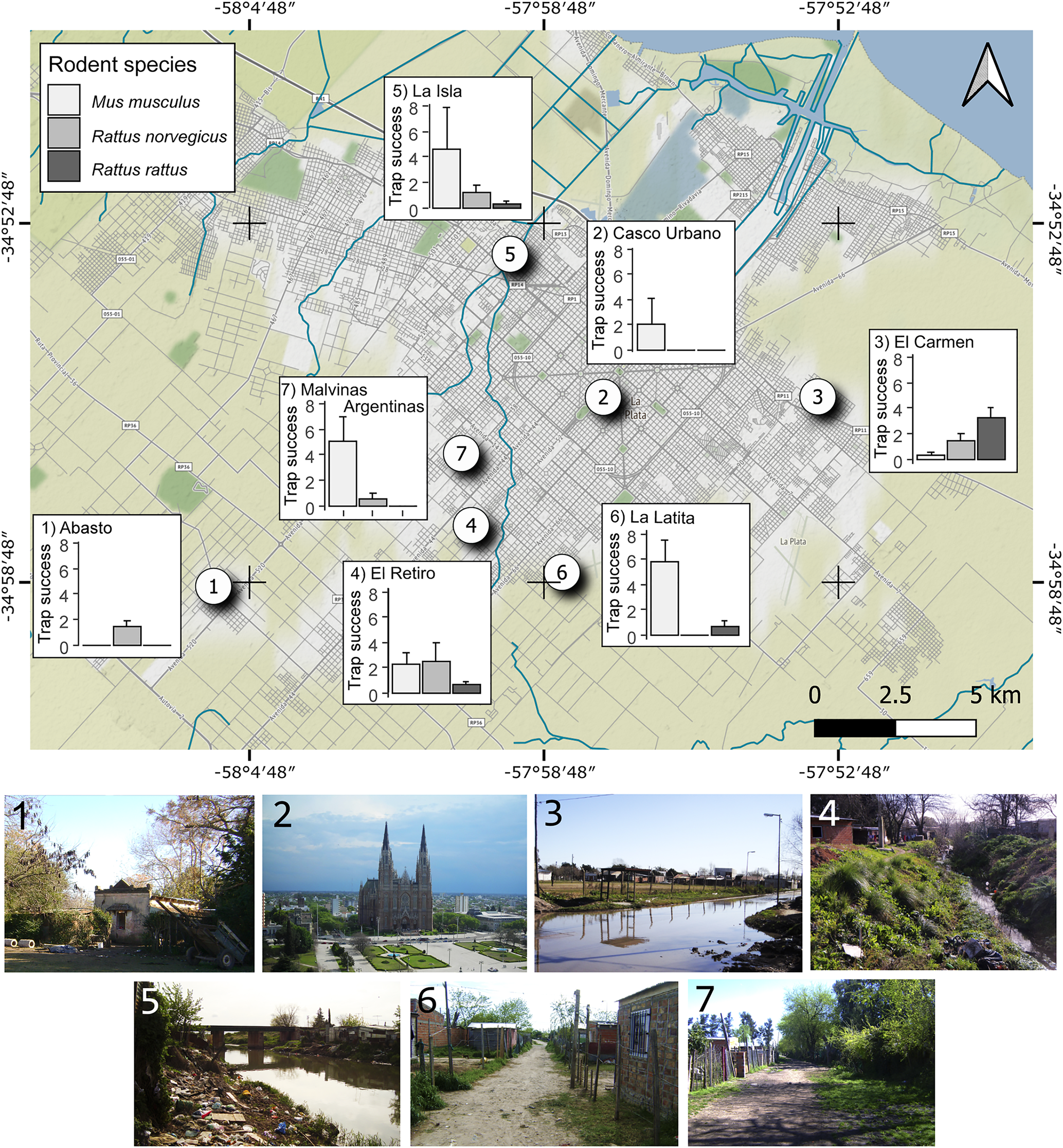
Fig. 1. Map of Gran La Plata, Buenos Aires province, Argentina; the annual mean trap success of each host species registered in each neighborhood and images of neighborhoods involved in the present study: (1) Abasto; (2) Casco Urbano; (3) El Carmen; (4) El Retiro; (5) La Isla; (6) La Latita; and (7) Malvinas Argentinas.
Sample collection
Rodent trapping surveys were performed from September 2014 to September 2015. Each neighborhood was sampled for four consecutive seasons. Rodents were captured using cage live traps (15 × 16 × 31 cm), Sherman traps (8 × 9×23 cm) and snap traps (17.5 × 8.5 cm). The bait consisted of a mixture of oats, banana and animal fat, carrot, and meat pieces. During seasonal samplings, between 30 and 50 traps were set daily for three consecutive nights inside or in the backyard of houses of each neighborhood, resulting in a trapping effort of 90–150 trap-nights in each neighborhood for each season.
The individuals captured were identified for species, sexed, and measured (full body and tail length). Specimens were sacrificed following procedures and protocols approved by national laws (Animal Protection National Law 14.346 and references in the provincial permits) and the Laboratory, Farm and Wildlife Animals Research Ethics Committee of the National Council of Scientific and Technical Research (CONICET).
Parasitological analysis
The digestive tract, kidneys, and fecal samples were fixed in 10% formalin or stored at −20°C, according to the technique applied to analyze the tissue. Rodent samples were studied in the Centro de Estudios Parasitológicos y de Vectores (CEPAVE) of La Plata and in the Laboratorio de Inmunoparasitología (LAINPA) of the Facultad de Ciencias Veterinarias (FCV) of the Universidad Nacional de La Plata (UNLP).
Digestive tracts were dissected under stereoscopic microscopy (Olympus SZ61-TR). Parasites were removed and preserved in 70% alcohol. Nematodes were diaphanized with lactophenol and mounted. Cestoda were overstained with acid carmine, dehydrated through an alcohol series, diaphanized in eugenol and mounted in Canada balsam for their identification. Specimens were observed under Olympus BX 51 (40-1000 X) optic microscopy. Helminths were identified following the keys from Travassos (Reference Travassos1914), Anderson et al. (Reference Anderson, Chabaud and Willmott2009) and Gibbons (Reference Gibbons2010) for Nematoda, and Khalil et al. (Reference Khalil, Jones and Bray1994) for Cestoda, and specific literature, i.e., Wardle & McLeod (Reference Wardle and Mcleod1952); Robles et al. (Reference Robles, Navone and Villafañe2008); Guerreiro Martins et al. (Reference Guerreiro Martins, Robles and Navone2014); Fitte et al. (Reference Fitte, Robles, Dellarupe, Unzaga and Navone2017); Lavikainen et al. (Reference Lavikainen, Iwaki, Haukisalmi, Konyaev, Casiraghi, Dokuchaev and Nakao2016); and Swain et al. (Reference Swain, Routray, Panigrahi, Rath, Sahoo and Ganguly2016). Fecal samples were processed using a sedimentation technique modified for this study, which included homogenization, filtration and microcentrifugation in 1.5-ml Eppendorf tubes for 2 min. at 3000 rpm, to concentrate the largest number of eggs in the minimum possible volume for identifying protozoa and eggs or larvae of helminths. The identification was based on Thienpont et al. (Reference Thienpont, Rochette and Vanparijs1979). For the detection of Eimeria spp. Schneider, 1875, fecal samples were processed using the sedimentation technique modified for this study (detailed above) and observed under Olympus BX 51 (40-1000 X) optic microscopy. Two complementary analyses were conducted for detection of T. gondii and N. caninum. First, a simple polymerase chain reaction (PCR) technique was applied from rodent brain samples; for T. gondii Tox5-Tox8 primers, and for N. caninum Np6+/Np21+ primers were used (Moré et al., Reference Moré, Pardini, Basso, Machuca, Bacigalupe, Villanueva and Venturini2010). The PCR conditions used are detailed in Dellarupe et al. (Reference Dellarupe, Fitte, Pardini and Campero2019). Secondly, an indirect immunofluorescence (IFI) analysis was applied following Dubey & Frenkel (Reference Dubey and Frenkel1998), and Dellarupe et al. (Reference Dellarupe, Fitte, Pardini and Campero2019). For Leptospira spp. detection, a conventional PCR was applied targeting the lipL32 gene in a kidney sample (Agudelo-Flórez et al., Reference Agudelo-Flórez, Londoño, Quiroz, Ángel, Moreno, Loaiza and Rodas2009). The PCR was performed using the primers lipL32/270 F and lipL32/692R (Agudelo-Flórez et al., Reference Agudelo-Flórez, Londoño, Quiroz, Ángel, Moreno, Loaiza and Rodas2009). The PCR conditions are detailed in Agudelo-Flórez et al. (Reference Agudelo-Flórez, Londoño, Quiroz, Ángel, Moreno, Loaiza and Rodas2009). Samples positive for Leptospira spp. were identified as two species by sequencing and comparing with other closely related species (Fitte & Kosoy, Reference Fitte and Kosoy2021).
Statistical analysis
For each parasite and pathogen taxon, the prevalence (P) for each host and neighborhood was calculated, and for helminths, parasite mean abundance (MA) and mean intensity (MI) indexes were also estimated following Bush et al. (Reference Bush, Lafferty, Lotz and Shostak1997).
The effects of species, sex, and body length or age among host characteristics, as well as host (rodent) community structure, the presence of a stream in the neighborhood and the season as environmental characteristics, were analyzed in relation to parasite and pathogen infection and parasite abundance. The presence of streams in the neighborhoods was considered because of the role that these play in urban rodent settlement (Traweger & Slotta-Bachmayr, Reference Traweger and Slotta-Bachmayr2005; Cavia et al., Reference Cavia, Gómez Villafañe, Suárez, Gómez, Sánchez and León2019a) as well as in the transmission of some pathogens (Soares et al., Reference Soares, Latorre, Laporta and Buzzar2010). Age in months was estimated for R. norvegicus, based on body length following Gómez Villafañe et al. (Reference Gómez Villafañe, Cavia, Vadell, Suárez and Busch2012), while body length was used as an age reference for R. rattus and M. musculus.
To characterize the rodent community structure in the neighborhood where each individual host was caught, we first estimated the abundance of each rodent species in each season and neighborhood by trap success: the number of individuals captured/number of trap-nights (Mills et al., Reference Mills, Ellis, Mckee, Maiztegui and Childs1991). Then, to synthesize the host community structure, a principal components analysis (PCA) was performed from the covariance matrix with the mean annual trap success for each species captured in each neighborhood (Legendre & Legendre, Reference Legendre and Legendre2012). The scores from the first principal component from each study site were used to describe the host community structure of each neighborhood (from now on the host community score).
Due to the low number of individuals caught in some seasons, they were grouped by season: autumn–winter (April–September, with monthly temperatures below 16°C and monthly precipitation below 100 mm); and spring–summer (October–March, with mean monthly temperatures equal to or above 16°C and monthly precipitation averaging 100 mm). To analyze whether the parasite/pathogen assemblage present in each individual host depends on the host species, sex, body length or age, or the host community score, or if it was caught in the cold or warm season, or in a neighborhood with the presence of a stream, canonical correspondence analyses (CCA) were performed, using each helminth species abundance and the infestation status (infected or not infected) for protozoa and bacteria as the response variables, and host species, sex, body length or age, host community score, season, and presence of a stream as explanatory variables. A forward selection procedure was used based on Akaike information criterion (AIC) to include variables that explain pathogen/parasite assemblage structure in the individuals. This analysis was performed using the vegan package (Oksanen et al., Reference Oksanen, Blanchet, Kindt, Oksanen and Suggests2013) of the R software, version 3.0.1 (R Core Team, 2013).
For each host species separately and for each parasite or pathogen taxon, we analyzed the effects on individual infection of host sex and body length/age, the season and the presence of a stream. We also analyzed, for each parasite or pathogen taxon, whether the infection depends on the host species. For this, multiple regression analysis was applied with generalized linear mixed models, with binomial distribution of errors and logistic link function for individual infection, and Poisson distribution for helminth abundance (Zuur et al., Reference Zuur, Ieno, Walker, Saveliev and Smith2009). When overdispersion was observed in helminth abundance analyses, models with negative binomial distribution of errors and logit link function were used (Zuur et al., Reference Zuur, Ieno, Walker, Saveliev and Smith2009). Neighborhood was included in the model as a random effect because these were sampled repeatedly in each season. When the random effect did not improve the model, the neighborhood factor was removed and generalized linear models were used (Zuur et al., Reference Zuur, Ieno, Walker, Saveliev and Smith2009). A forward stepwise procedure was used for factor selection on these multiple regression analyses, based on the greater change in deviance for factors or interactions. When more than one candidate model was found, we employed the AIC for model selection, reporting only models with ΔAIC <3 in relation to the best-fit model with the lowest AIC (Burnham & Anderson, Reference Burnham and Anderson2002). For the accuracy measures, Kappa index (K) and proportion of correct classifications (PCC) are reported (Titus et al., Reference Titus, Mosher and Williams1984).
Results
A total of 136 rodents, of the urban species M. musculus, R. norvegicus, and R. rattus, were captured with a trapping effort of 972 Sherman trap-nights, 972 cage live trap-nights and 1044 snap trap-nights. The three host species were captured in El Carmen, La Isla and El Retiro, two species in La Latita and Malvinas Argentinas, and only one species was caught in Abasto and Casco Urbano (fig. 1). According to the PCA, abundance of the three rodent species was not independent in the neighborhoods. The first principal component (PC1) explained 76.6% of the association between the mean trap success of the captured host species in the neighborhoods. This component distinguishes neighborhoods with comparatively higher abundances of M. musculus (Pearson r = 0.95) and lower abundances of R. rattus (Pearson r = −0.20) and R. norvegicus (Pearson r = −0.24) from neighborhoods with comparatively higher abundances of R. rattus and R. norvegicus and lower abundances of M. musculus.
A total of 62.5% of the rodents were infected with at least one parasite or pathogen taxon. Rattus norvegicus was the dominant host species in Abasto and El Retiro with a total of 89.29% individuals parasitized; R. rattus was the dominant species in El Carmen with 90.48% of individuals parasitized; while M. musculus was the dominant host species in La Isla, La Latita, Malvinas Argentinas and Casco Urbano with 41.12% of individuals parasitized (fig. 1).
A total of 15 parasite and pathogen taxa were found: ten corresponded to helminths; three to protozoa; and two to bacteria. The occurrence of parasite and pathogen taxa varied between neighborhoods. Strobilocercus fasciolaris was the only species found in all the neighborhoods, followed by T. gondii, found in all the neighborhoods except Casco Urbano (table 1). The highest numbers of parasites and pathogens were recorded in neighborhoods with streams (table 1). Among the taxa with health risk, H. diminuta, Rodentolepis nana and S. fasciolaris (Helminths), T. gondii (Protozoa), and Leptospira borgpetersenii and Leptospira interrogans (Bacteria) were found (table 1).
Table 1. Prevalence % (P), mean abundance (MA), mean intensity (MI), parasite/pathogen richness (S) and number of individuals analyzed (n) for each neighborhood, and proportion of neighborhoods in which each parasite/pathogen were registered on rodents captured over the seven neighborhoods studied (Prop) in Gran La Plata, from 2014 to 2015.

Values of neighborhoods with rodent individuals <10 are indicated in fractions. The neighborhoods El Retiro, El Carmen and La Isla are crossed by streams.
According to the CCA, the host species (F2,83 = 9.56, P < 0.001) and the PC1 (host community structure, F1,83 = 4.87, P < 0.001) were the only features that explained the variation of the composition and abundance of parasite and pathogen assemblages, explaining 23.92% of their variation (fig. 2; CCA, F3,83 = 8.70, P < 0.001). Ten parasite and pathogen taxa were found in R. norvegicus, and nine in R. rattus and M. musculus. Nippostrongylus brasiliensis, H. spumosa, S. fasciolaris, Eimeria spp. and T. gondii were recorded in the three host species. The rest of the parasite and pathogen taxa were found in two or one host species (table 2). The CCA indicated that both Rattus species showed a similar composition and abundance of parasites and pathogens, which at the same time differed from those of M. musculus individuals. A stronger association was observed between Rattus species and H. diminuta, R. nana and Strongylides ratti Sandground, 1925, and between M. musculus and L. borgpetersenii and Syphacia obvelata Rudolphi, 1802 (tables 1 and 2, fig. 2). Moreover, parasite abundance or pathogen infestation depended on whether the host community was dominated by M. musculus or by the Rattus species. The abundance of S. fasciolaris and S. ratti and the frequency of infestation of S. obvelata and L. borgpersenii were comparatively higher on individuals caught in neighborhoods where M. musculus was more abundant, while the abundance of N. brasiliensis and H. diminuta was higher on individuals in neighborhoods with higher abundance of R. norvegicus or R. rattus (fig. 2). The frequency of T. gondii infection (prevalence) was higher in individuals of R. norvegicus than in M. musculus, while R. rattus showed intermediate values (tables 2 and 3A). The prevalence values of T. gondii were similar for both sexes, for the different body lengths/ages, for both seasons and for neighborhoods with or without streams in the three host species (P > 0.05 for all cases).

Fig. 2. Spatial ordering of components 1 and 2 (canonical correspondence analyses (CCA) axis 1 and CCA axis 2, respectively) of the (left) host individuals based on helminth abundance, and protozoa and bacterial infection restricted by (right) host species and host community structure (first principal component (PC1)) according to the CCA. [inset: Spatial ordering of components 1 and 2 (PC1 and second principal component (PC2), respectively) according to principal components analysis of mean annual trap success in each neighborhood for each host species used to synthesize the host community structure on the CCA]. Different symbols indicate individuals of different host species. Rn: Rattus. norvegicus, Rr: Rattus rattus, Mm: Mus musculus, TS: trap success, PC1: first principal component used to characterize the host community structure in the neighborhoods. Increasing values of PC1 indicate comparatively higher abundance of M. musculus and lower abundance of R. rattus and R. norvegicus, while decreasing values of PC1 indicate the opposite. Numbers in the inset plot correspond to neighborhoods as in fig. 1.
Table 2. Prevalence % (P), mean abundance (MA), mean intensity (MI), parasite/pathogen richness (S) and number of individuals analyzed (n) for each host species, and proportion of host species in which each parasite/pathogen occurred over the three rodent species (Prop) captured in the seven neighborhoods studied in Gran La Plata, from 2014 to 2015.

Table 3. Analysis of the individual infection of parasite and pathogen taxa in relation to hosts and environmental variables in Gran La Plata, from 2014 to 2015 (see text). In all cases, variables were introduced in models when they produced a significant deviance (P < 0.05).

For all cases, the random effect of the neighborhood was deleted because it did not improve the model. Therefore, generalized linear models were applied. LRT: likelihood-ratio test, d.f.: degrees of freedom, PCC: proportion of correct classifications, SE: standard error.
* Marginal significant values.
The prevalence of H. spumosa was higher in Rattus species than in M. musculus (table 3B). None of the factors analyzed explained the prevalence differences in R. norvegicus and R. rattus (P > 0.05 for all cases). For H. spumosa comparisons, M. musculus was eliminated because only three individuals were parasitized. The abundance of this helminth was similar for both Rattus species (likelihood-ratio test (LRT) = 0.031; gl = 1; P = 0.859; table 1). None of the variables analyzed explained the variations in the abundance of this parasite (P > 0.05 for all cases).
The prevalence of N. brasiliensis was higher in Rattus species than in M. musculus (table 3C). Its prevalence in R. norvegicus was higher during the cold season than in the warm season (89.47% and 55.56%, respectively; table 3 D). In R. rattus, all the infected individuals corresponded to neighborhoods with streams (table 1). Abundance was not analyzed in M. musculus, since only three individuals were parasitized. The abundance was similar for both Rattus species (LRT = 1.748; gl = 1; P= 0.186; table 2). For R. norvegicus, abundance tended to be higher in males than in females (table 4A). For R. rattus, one outlier was observed with 228 parasites and it was therefore dropped from the analyses. After this, none of the variables analyzed explained the variations in abundance of this parasite (P > 0.05 for all cases).
Table 4. Analysis of helminth abundance in relation to hosts and environmental variables in Gran La Plata, from 2014 to 2015 (see text). In all cases, variables were introduced in models when they produced a significant deviance (P < 0.05). The error distribution used for each model is reported in parentheses. LRT: likelihood ratio test, d.f.: degrees of freedom.

* Marginal significant values.
Strobilocercus fasciolaris tended to be more prevalent in R. norvegicus than in the other host species (tables 2 and 3 E). The prevalence was higher in R. norvegicus and M. musculus during the cold season (prevalence in R. norvegicus: 47.37% in cold seasons – 11.11% in warm seasons; prevalence in M. musculus: 35.71% in cold seasons – 10.96% in warm seasons; table 3F and G). Its abundance was higher in R. norvegicus and R. rattus than in M. musculus (tables 2 and 4B). Rattus norvegicus and M. musculus presented higher abundance in cold seasons than in warm seasons (table 4C and D), but no variable explained the abundance differences in R. rattus (P > 0.05 for all cases).
For S. ratti, only specimens of R. norvegicus and R. rattus were found parasitized, with similar prevalence in both species (LRT = 0.247; gl = 1; P = 0.619; table 1). Abundance of this helminth was also similar in both Rattus species (LRT = 0.496; gl = 1; P = 0.481). Due to the low number of rodent specimens parasitized, the relation to different factors was not analyzed. However, all the infected individuals of R. norvegicus were caught in neighborhoods with streams (table 1).
For S. obvelata in M. musculus, overdispersion could not be controlled in models (overdispersion parameter >20). However, females in the warm season showed the highest prevalence and abundance (Supplemental material).
For Leptospira spp., there were no differences in the prevalence in R. norvegicus and M. musculus (LRT = 0.266; gl = 1; P = 0.606). None of the variables analyzed could explain the infection variations of L. borgpetersenii in M. musculus (P > 0.05 for all cases). Differences in L. interrogans infection in R. norvegicus were not analyzed due to the low number (two) of infected individuals. For the other pathogen and parasite taxa studied, analyses could not be conducted because few individuals were infected.
Discussion
Although there are studies on the relationship of urban rodents, pathogens and parasites (Jittapalapong et al., Reference Jittapalapong, Herbreteau, Hugot, Arreesrisom, Karnchanabanthoeng, Rerkamnuaychoke and Morand2009; Himsworth et al., Reference Himsworth, Parsons, Jardine and Patrick2013; Costa et al., Reference Costa, Porter, Rodrigues, Farias and de Faria2014; Panti-May et al., Reference Panti-May, Hernández-Betancourt, Rodríguez-Vivas and Robles2015), only a few focus on the composition of parasite fauna and their relationships with hosts, host community structure and environmental characteristics (Panti-May et al., Reference Panti-May, Hernández-Betancourt, Rodríguez-Vivas and Robles2015). Moreover, studies that summarize and compare results, including the three urban rodent species in an integrated way, are scarce (e.g., Hancke & Suárez, Reference Hancke and Suárez2017). In this survey, a community approach (Johnson et al., Reference Johnson, De Roode and Fenton2015) was used in a scenario with zoonotic multi-pathogens and parasites and multi-hosts. This is the first study carried out in Gran La Plata, which serves as an example of the situation of cities of Argentina and Latin America. Here, six parasite and/or pathogen taxa with health risk were found, with evidence that the composition and abundance of parasites and pathogens are influenced not only by host and environmental characteristics, but also by the host community structure, as Johnson et al. (Reference Johnson, De Roode and Fenton2015) proposed. Among environmental characteristics, the changes in environmental conditions due to seasonality and the presence of a stream, explained the variations observed in the prevalence and abundance of some parasite and/or pathogen taxa in the host species.
The three urban rodent species are sympatric, but they are exploiting different microenvironments with different degrees of urbanization (Cavia et al., Reference Cavia, Cueto and Suárez2009, Reference Cavia, Gómez Villafañe, Suárez, Gómez, Sánchez and León2019a, Reference Cavia, Gómez Villafañe, Suárez, Piudo, Sánchez and Monteverde2019b, Reference Cavia, Gómez Villafañe, Suárez, Piudo, Sánchez and Monteverde2019c; Coto, Reference Coto2015). If these microenvironments are considered separately, R. norvegicus could be expected to share its parasite fauna with M. musculus rather than with R. rattus, given that those two species live in contact with the ground and water, while the latter lives in higher strata (Coto, Reference Coto2015; Cavia et al., Reference Cavia, Gómez Villafañe, Suárez, Gómez, Sánchez and León2019a, Reference Cavia, Gómez Villafañe, Suárez, Piudo, Sánchez and Monteverde2019b, Reference Cavia, Gómez Villafañe, Suárez, Piudo, Sánchez and Monteverde2019c). However, our results show that the parasite fauna of both Rattus species are similar, and different from those of M. musculus (fig. 2; table 2), in agreement with other studies in which a phylogenetic conservationism is observed, with two closely related host species tending to harbor more similar parasite fauna than two distantly related species (Poulin, Reference Poulin2014).
None of the other host and environmental characteristics explained the structure of the parasite assemblage in each host individual. Future studies with bigger sample sizes, conducted in more neighborhoods, would help to understand the importance of these characteristics in parasite assemblages that have been previously documented at population level (Gómez Villafañe et al., Reference Gómez Villafañe, Robles and Busch2008; Lovera et al., Reference Lovera, Fernández, Jacob, Lucero, Morici, Brihuega and Cavia2017).
Notably, pathogen and parasite richness were similar among all the host species, despite the differences in parasite fauna composition between Rattus species and M. musculus (table 2). Moreover, all host species presented pathogen and parasite taxa with health implications: five in R. norvegicus and R. rattus and two in M. musculus, proving the risk of infection that is found when there is a close coexistence between urban rodents and the human population.
Prevalence values match those of previous studies and confirm urban rodents as important reservoirs and sources of infection of pathogens and parasites (Milazzo et al., Reference Milazzo, de Bellocq, Cagnin, Casanova, Di Bella, Feliu and Santalla2003; Panti-May et al., Reference Panti-May, Hernández-Betancourt, Rodríguez-Vivas and Robles2015). At the same time, the higher prevalence of parasites and pathogens in Rattus species in comparison to M. musculus agrees with previous studies (Milazzo et al., Reference Milazzo, de Bellocq, Cagnin, Casanova, Di Bella, Feliu and Santalla2003; Panti-May et al., Reference Panti-May, Hernández-Betancourt, Rodríguez-Vivas and Robles2015) and may be explained from the host abundance and host community structure (Johnson et al., Reference Johnson, De Roode and Fenton2015; Lovera et al., Reference Lovera, Fernández, Jacob, Lucero, Morici, Brihuega and Cavia2017).
In terms of the prevalence and abundance of each pathogen and parasite taxon at an individual level, different results were reached. Host characteristics were less important than environmental ones to explain individual infection, which agrees with recent studies (Panti-May et al., Reference Panti-May, Hernández-Betancourt, Rodríguez-Vivas and Robles2015). The trends of difference between the abundances of N. brasiliensis in R. norvegicus and S. obvelata in M. musculus suggest the need for exhaustive studies to provide more data. In relation to the environmental changes, higher infestation levels were observed in the cold season for N. brasiliensis and S. fasciolaris. For the case of N. brasiliensis in R. norvegicus, these results disagree with previous studies showing this parasite as the most prevalent species in countries with warm temperatures (Calero et al., Reference Calero, Ortiz and Souza1950; De León, Reference De León1964; Waugh et al., Reference Waugh, Lindo, Foronda, Ángeles-Santana, Lorenzo-Morales and Robinson2006) and with laboratory studies that report that the best temperatures for its development are 22–30°C (Haley, Reference Haley1962). The association between S. fasciolaris in R. norvegicus and M. musculus suggests that, when their energy requirements increase, individuals may increase their home range, thus presenting a higher risk of infection.
In relation to the presence of streams, although the life cycles of neither N. brasiliensis nor S. ratti include stages that depend on water bodies, the microenvironments of the banks of streams may be favorable for the development of the infective stage of both parasite species. This topic needs to be more deeply investigated. Also, future studies need to include other factors, as the variations in infection of some parasites and pathogens were not explained in our study.
This study confirms the role that urban rodents play as reservoirs and infection sources of zoonotic parasites and pathogens. It is one of the few studies that include the pathogen and parasite assemblage in the entire rodent assemblage in various neighborhoods of a city in South America. Our results suggest the need for stronger public health policies, particularly in the field of zoonosis. The results are valuable given that the peripheral neighborhoods of our region have different urban characteristics from other cities of the world and, consequently, different ecological and epidemiological scenarios. During the survey, we observed the closeness with which humans and rodents coexist, even recording rodent attacks (bites) to children while sleeping, reflecting the permanent risk people face.
In many similar cities of Latin America, peripheral neighborhoods are growing dramatically, with characteristics that are a constant health risk to the population (Soares et al., Reference Soares, Latorre, Laporta and Buzzar2010; Chaiblich et al., Reference Chaiblich, Lima, Oliveira, Monken and Penna2017). Despite limitations related to sampling and consequent analyses, plus logistical issues involved in conducting these kind of studies in such urban areas (Childs et al., Reference Childs, McLafferty, Sadek, Miller, Khan, DuPree and Glass1998; Cavia et al., Reference Cavia, Cueto, Suárez, Larramendy and Soloneski2012), our results may serve as a starting point for future studies for each specific zoonotic taxon, and/or for studies with longer sampling periods in larger areas, also using different methods to contribute to greater knowledge about rodent population sizes and variations. Lastly, surveys that include synanthropic animals as reservoirs and/or vectors of pathologies at a regional level should be considered as pieces of the puzzle that help to better understand these issues at a global level (Schapira, Reference Schapira2001).
Supplementary material
To view supplementary material for this article, please visit https://doi.org/10.1017/S0022149X21000523
Acknowledgements
We thank all the people who offered their homes for carrying out the trapping. We are grateful to Kevin Steffen, Juliana Sánchez, Macarena Zarza, Paola Cociancic and Lorena Zonta for collecting hosts; to Carlos Galliari and Pablo Teta for the identification of hosts; and to Emilio Topa for the preparation of the slides of tissue fragments. This study was funded by PIO CONICET-UNLP.
Author contributions
BF was in charge of the rodent sampling, prospection and identification of parasites and pathogens. RC carried out the statistical design and analyses. MRR coordinated the project and participated in rodent sampling as well as in the identification of parasites and pathogens. JMU and AD participated in rodent sampling and carried out molecular analyses for the identification of protozoa. GTN coordinated and managed the project. All authors reviewed the results and approved the final version of the manuscript.
Financial support
This study was funded by PIO (Proyecto de Investigación Orientado) CONICET-UNLP.
Conflicts of interest
None.
Ethical Standards
This research did not involve human and/or laboratory animal experimentation.