1. Introduction
Magmatic rocks found in orogenic belts provide a record of the thermal and chemical evolution of the deep lithospheric root of the developing orogen. During the evolution of continental collision zones, the convergence is typically first accommodated by thrusts leading to lithospheric thickening and then by crustal-scale shear zones leading to synconvergent exhumation and lateral extrusion. Magmas of variable sources and types may be generated during the synconvergence post-thickening period. This type of magmatism, described as post-collisional, comprises a large variety of magmatic rocks including calc-alkaline or peraluminous granitoids and alkaline-type series (Whalen, Currie & Chappell, Reference Whalen, Currie and Chappell1987; Lagarde, Capdevila & Fourcade, Reference Lagarde, Capdevila and Fourcade1992; Bonin, Reference Bonin and Demaiffe1996; Whalen et al. Reference Whalen, McNicoll, van Staal, Lissenberg, Longstaffe, Genner and van Breeman2006); it is therefore potentially informative of a range of deep crustal processes.
In the Moroccan Variscan belt, the SW extremity of the Variscan orogen of Europe and North Africa, Variscan convergence was mainly accommodated by conjugate transcurrent shear zones that are locally associated with westward thrusting (Piqué, Jeannette & Michard, Reference Piqué, Jeannette and Michard1980; Lagarde & Michard, Reference Lagarde and Michard1986). Despite this very limited crustal thickening, numerous syntectonic calc-alkaline and peraluminous granitic plutons intruded the Cambrian–Carboniferous sedimentary formations of the Moroccan Meseta (Diot & Bouchez, Reference Diot and Bouchez1989; Lagarde, Aït Omar & Roddaz, Reference Lagarde, Aït Omar and Roddaz1990; Gasquet, Stussi & Nachit, Reference Gasquet, Stussi and Nachit1996). Some of these plutons are associated with coeval mafic rocks (Fig. 1), for example the Tichka massif (Vogel et al. Reference Vogel, Williams, Preston and Walker1976; Gasquet et al. Reference Gasquet, Leterrier, Mrini and Vidal1992) and Tanncherfi complex (Ajaji et al. Reference Ajaji, Weis, Giret and Bouabdellah1998), allowing evaluation of crustal- versus mantle-derived source contributions in the genesis of the granitic magmas. The geodynamic context and the thermotectonic event that allowed crust and mantle melting in the Moroccan Meseta remain a subject of discussion. Magmatism was related to subduction and wet melting of the metasomatized mantle lithosphere of the overriding plate (Roddaz et al. Reference Roddaz, Brusset, Soula, Beziat, Benabbou, Debat, Driouch, Christophoul, Ntarmouchant and Deramond2002; El Hadi et al. Reference El Hadi, Simancas Cabrera, Tahiri, González Lodeiro, Azor Pérez and Martínez Poyatos2006; Michard et al. Reference Michard, Soulaimani, Hoepffner, Ouanaimi, Baidder, Rjimati and Saddiqi2010) and/or to partial fusion of the lower crust during a post-collisional intracontinental deformation (Lagarde, Reference Lagarde1989; Gasquet, Stussi & Nachit, Reference Gasquet, Stussi and Nachit1996; Hoepffner et al. Reference Hoepffner, Soulaimani and Piqué2005). In their general plate-tectonic evolution of the SE Variscan belt (von Raumer & Stampfli, Reference von Raumer and Stampfli2008; von Raumer, Bussy & Stampfli, Reference von Raumer, Bussy and Stampfli2009), the Moroccan Meseta and the Anti-Atlas represent the northern and southern margins of the Palaeotethys, respectively. This ocean was consumed during the Carboniferous by combining N-dipping subduction, dextral strike-slip and collision between the continents of Gondwana and Laurussia.
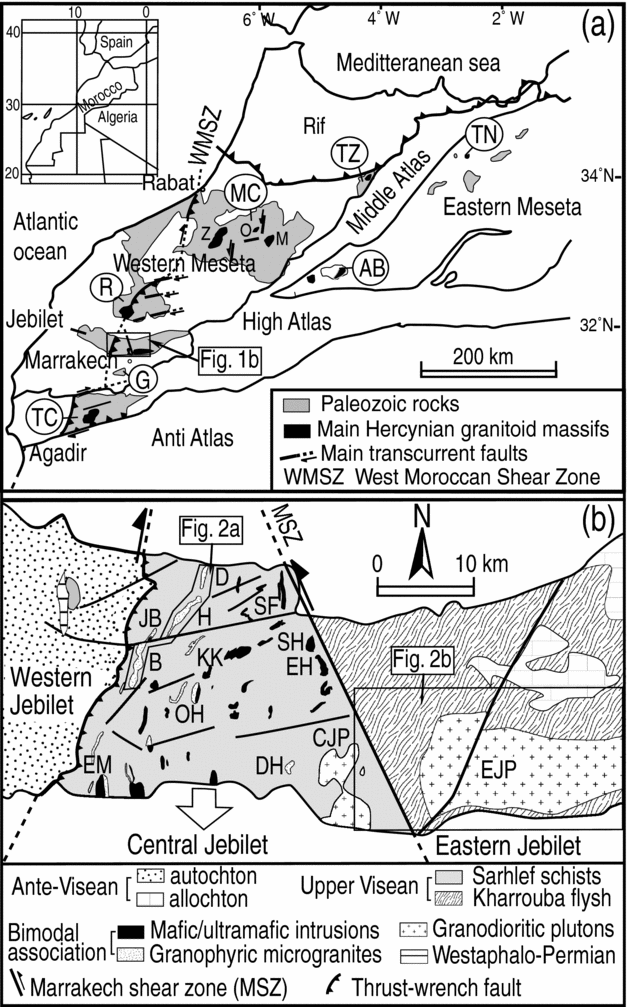
Figure 1. (a) Moroccan Variscan granitoids (black) and their Palaeozoic cover (grey) (TN – Tanncherfi; AB – Aouli-Bou Mia; TZ – Tazekka; TC – Tichka; MC – Maroc Central with Zaer (Z), Oulmes (O) and Ment (M) plutons; R – Rehamna; G – Guemassa). (b) Geological sketch map of the Jebilet massif (modified from Huvelin, Reference Huvelin1977). Slip on the regional fractures is accompanied by extrusion to the south of the central Jebilet block (large white arrow). BHD – Koudiat Bouzlaf, Hamra and Diab intrusions; DH – Draa El Harach; KK – Koudiat Kettara; OH – Oled Har; SF – Safsafat; SH – Sarhlef; EH – El Harcha; EM – El Mna; JB – Jbel Bouzlaf; CJP – Central Jebilet Pluton; EJP – Eastern Jebilet Pluton.
In the Jebilet massif, the syntectonic magmatic rocks can be divided into two main groups: a bimodal magmatic association including numerous intrusions of tholeiitic mafic–ultramafic rocks and alkaline granophyric microgranites; and calc-alkaline peraluminous granodioritic plutons cut across by leucogranitic dykes. It has been suggested that the bimodal association is mantle-derived, whereas the peraluminous granitoids largely have a source in the upper crust (Aarab & Beauchamp, Reference Aarab and Beauchamp1987; Mrini et al. Reference Mrini, Rafi, Duthou and Vidal1992). The presence of these two magmatic groups most likely reflects a systematic interaction between the continental crust and upper mantle in this region during the Variscan orogeny.
In this paper, petrographical, geochemical and isotopic (Rb–Sr, Sm–Nd) data for the different Carboniferous magmatic suites are integrated in order to estimate the composition of the source rocks, to constrain the petrogenesis of the varied magmatic rocks and to evaluate tectonic models for the evolution of the Moroccan Meseta during Late Palaeozoic time.
2. Geological setting and geology of the Jebilet magmatism
2.a. Geological setting
The Jebilet massif, located 7 km north of Marrakech, is one of the largest Palaeozoic massifs of the Variscan fold belt of Morocco. Together with the Rehamna and the central Palaeozoic massifs to the north and the high Atlas Palaeozoic block to the south, it constitutes the western Meseta (separated from the eastern Meseta by the folded Mesozoic–Cenozoic cover of the middle Atlas) (Fig. 1a). The Mesetas display a nearly complete Palaeozoic sedimentary sequence, folded and metamorphosed at greenschist–amphibolite facies, and intruded by widespread syn- to late-orogenic Carboniferous granitoids.
The granitoids of the Mesetas can be grouped into three main groups (Vogel et al. Reference Vogel, Williams, Preston and Walker1976; Gasquet, Stussi & Nachit, Reference Gasquet, Stussi and Nachit1996; El Hadi et al. Reference El Hadi, Simancas Cabrera, Tahiri, González Lodeiro, Azor Pérez and Martínez Poyatos2006): (1) calc-alkaline biotite±cordierite granodiorites, locally with associated mafic magmas; (2) two-mica leucogranites; and (3) subalkaline–alkaline granites. The calc-alkaline granodioritic plutons are dominant (e.g. Jebilet, Aouli BouMia, Tanncherfi; Fig. 1a). They display I-type or mixed S- and I-type characteristics (0.703 < Sri <0.711; −6.7 < εNd < +7.4) and are locally associated with mantle-derived basic magmas (e.g. Tichka pluton, Fig. 1a). Among these calc-alkaline granitoids, subduction-related plutonic rocks have been recognized in the Tanncherfi plutonic complex where coeval potassic (shoshonitic) and sodic (granodioritic) calc-alkaline series, with large-ion lithopile element (LILE) and light rare Earth element (LREE) enrichment and Nb, Ta, Ti depletion, were emplaced (Ajaji et al. Reference Ajaji, Weis, Giret and Bouabdellah1998). The leucogranites are typical S-type granites (0.707 < Sri < 0.718; −10.7 < εNd < –1.5) derived from metasedimentary source rocks (Mahmood & Bennani, Reference Mahmood and Bennani1984; Mrini et al. Reference Mrini, Rafi, Duthou and Vidal1992). They are intrusive into the granodiorites (e.g. Zaer pluton, Fig. 1a) or into Lower Palaeozoic metasediments (e.g. Oulmes pluton, Fig. 1a). The subalkaline–alkaline granites (0.704 < Sri < 0.707; −4.9 < εNd < 0.6) result from mixing between crust- and mantle-derived magmas (Gasquet, Stussi & Nachit, Reference Gasquet, Stussi and Nachit1996). They are associated with mafic rocks and coeval with the calc-alkaline granites (e.g. Tichka massif) or intrusive into Lower Palaeozoic metasediments (e.g. Rehamna, Fig. 1a).
In addition to two cordierite-bearing granodioritic plutons intruded by leucogranites, Carboniferous magmatism in the Jebilet massif includes a compositionally bimodal association of alkaline microgranites and mafic–ultramafic intrusions (Bordonaro, Gaillet & Michard, Reference Bordonaro, Gaillet and Michard1979; Gasquet, Stussi & Nachit, Reference Gasquet, Stussi and Nachit1996). Its spatial distribution is limited to the west by a NNE–SSW dextral thrust-wrench shear zone (Mayol & Muller, Reference Mayol and Muller1985; Le Corre & Bouloton, Reference Le Corre and Bouloton1987) separating the central Jebilet unit, a schistose and metamorphosed block of marine Visean shales (Sarhlef schists), from the western Jebilet, a weakly deformed to undeformed block of Cambro-Ordovician limestones, shales and sandstones (Fig. 1b) (Huvelin, Reference Huvelin1977). To the east, the granodioritic plutons are spatially associated with a NNW–SSE sinistral wrench shear zone (the Marrakech Shear Zone, Lagarde & Choukroune, Reference Lagarde and Choukroune1982; Essaifi, Lagarde & Capdevila, Reference Essaifi, Lagarde and Capdevila2001) corresponding to the boundary with the eastern Jebilet, a weakly metamorphosed to unmetamorphosed block of upper Visean syntectonic ‘flysch’ with olistostromes and inliers of Ordovician–Devonian sedimentary rocks (Huvelin, Reference Huvelin1977; Beauchamp, Izart & Piqué, Reference Beauchamp, Izart and Piqué1991). These two shear zones are located in the southern prolongation of the western Meseta shear zone (WMSZ, Fig. 1a), which is the western boundary of the Carboniferous basins of the Moroccan Meseta (Piqué, Jeannette & Michard, Reference Piqué, Jeannette and Michard1980). Westphalian–Permian continental conglomerates (Huvelin, Reference Huvelin1977) rest unconformably upon the Hercynian folded sequence.
Both the cordierite-bearing granodioritic plutons and the bimodal plutonic suite intrude weakly metamorphosed (lower greenschist facies) marine metapelites dated to upper–middle Visean (Huvelin, Reference Huvelin1977; Playford et al. Reference Playford, Gonzalez, Moreno and Alansari2008). Structural studies of the Jebilet plutons and the surrounding rocks have provided evidence of syntectonic emplacement at high crustal levels for both the bimodal association and the granodioritic plutons (Le Corre & Saquaque, Reference Le Corre and Saquaque1987; Lagarde, Aït Omar & Roddaz, Reference Loosveld and Etheridge1990; Essaifi, Lagarde & Capdevila, Reference Essaifi, Lagarde and Capdevila2001). Regional metamorphism was contemporaneous with ductile deformation developed during Late Carboniferous crustal shortening, associated with the main Variscan tectonic event in Morocco (Hoepffner et al. Reference Hoepffner, Soulaimani and Piqué2005; Michard et al. Reference Michard, Soulaimani, Hoepffner, Ouanaimi, Baidder, Rjimati and Saddiqi2010). The bimodal intrusions and the granodioritic plutons have induced in the surrounding metapelites a low-pressure contact metamorphism that reaches the hornblende- and the pyroxene-hornfels facies, respectively (Fig. 2a, b). Near the boundaries with the host rocks, numerous centimetre- to kilometre-scale enclaves of the contact hornfels exist in the eastern Jebilet granodioritic pluton. The contact metamorphism paragenesis suggests that the plutons were emplaced at less than 2.2 kbar corresponding to a maximum depth of 8 km (Bouloton, Reference Bouloton1992). Leucogranitic dykes and stocks cut across the granodioritic plutons and their host rocks. Triassic microdioritic dykes post-date the Variscan deformation and cut both the bimodal association and the granodioritic plutons. They contain numerous types of enclaves, particularly of the Proterozoic rocks (Huvelin, Reference Huvelin1977; Bouloton & Gasquet, Reference Bouloton and Gasquet1995; Dostal et al. Reference Dostal, Keppie, Hamilton, Aarab, Lefort and Murphy2005) that constitute the basement of the Variscan fold belt of Morocco (Michard, Reference Michard1976; Piqué et al. Reference Piqué, Bossière, Bouillin, Challouan and Hoepffner1993).

Figure 2. Low-pressure–high-temperature metamorphism around (a) the BHD granophyric microgranites (modified from Essaifi et al. Reference Essaifi, Lagarde and Capdevila2001) and (b) the eastern Jebilet cordierite-bearing granodioritic pluton (modified from A. Chemesseddoha, unpub. Ph.D. thesis, University of Rennes I, Rennes, 1986). Location of the Sr–Nd samples is indicated. The other samples were collected from Kettara intrusion (GSK, DK13, DK23, DK30, GK3, MK5 and DK25 mafic–ultramafic rocks; MTK and MGTK microgranites), Oled Har intrusion (OH6 and 00M03 gabbros; MOH1 and 00M04 microgranites), El Mna (EM4 gabbro, EM7 quartz-diorite and EM6 microgranite), Jbel Bouzlaf intrusion (BZN3 and MBZN3 microgranites) and central Jebilet pluton (00M01 granodiorite) (see Fig. 1b).
2.b. Geology of the Jebilet Carboniferous magmatism
At the present level of exposure, the bimodal association in the Jebilet massif comprises: (1) mafic–ultramafic plutons (peridotites and gabbros) with alkaline microgranitic stocks and dykes; and (2) volumetrically insignificant intermediate rock types (quartz diorites) found at gabbro/granitoid contacts, which may represent zones of magma mixing. Thin aplitic to perlitic rhyolites and rhyodacitic volcaniclastic rocks are also found in the Sarhlef schists (Bordonaro, Gaillet & Michard, Reference Bordonaro, Gaillet and Michard1979; Aarab & Beauchamp, Reference Aarab and Beauchamp1987). The calc-alkaline granodiorite plutons form two larger plutons.
2.b.1. Bimodal association
The bimodal plutonism (>65% mafic–ultramafic, the remainder is felsic) occurs as numerous felsic and mafic intrusions of some hundreds of metres in thickness and a few kilometres in length (Fig. 1b). They are arranged into three N–S to NE–SW lineaments that are broadly parallel to local shear zones.
The mafic–ultramafic rocks include mafic–ultramafic cumulates (gabbros, peridotites) forming stock or sill-like layered intrusions showing centimetre-scale banding, and dolerite dykes which cut across both the intrusions and the country rocks (Fig. 3a–c). Deformation within these intrusions is very heterogeneous, and subvertical centimetre- to metre-scale shear zones with strong planar fabrics enclose lenticular domains of sub-isotropic gabbros. The Kettara intrusion is a stratified sill composed of a lower banded series of ultramafic cumulates cut by an upper series of mafic cumulates (massive and layered leucogabbros) (Fig. 3a). In the undeformed rocks, magmatic textures are preserved despite an incipient–moderate recrystallization. The magmatic minerals include olivine, clinopyroxene, plagioclase, spinel, ilmenite, apatite and quartz, while minerals related to later alteration include amphibole, chlorite, muscovite, serpentine, epidote, prehnite, anatase and calcite. The cumulate rocks are medium–coarse-grained with ortho- to mesocumulate textures. The ultramafic cumulates are peridotites with olivine and chromian spinel as the cumulus phases and plagioclase, clinopyroxene and ilmenite as the intercumulus phases. The mafic cumulates are leucogabbros with olivine and plagioclase as the cumulus phases while clinopyroxene and ilmenite form the intercumulus phases. Minimum crystallization temperatures for the mafic–ultramafic cumulates were estimated from the clinopyroxene geothermometer (Lindsley & Anderson, Reference Lindsley and Andersen1983) at 1000–1100°C (Essaifi, Reference Essaifi1995). The non-cumulate mafic rocks form gabbros and dolerites with ophitic and subophitic textures, respectively. The gabbros vary from olivine gabbros and ilmenite-rich gabbros to quartz gabbros, while the dolerites range from olivine-bearing to quartz-bearing dolerites (Aarab & Beauchamp, Reference Aarab and Beauchamp1987). Mafic–ultramafic cumulates are also present in the majority of the other intrusions of the bimodal association. Mafic–ultramafic rocks crop out in the western part of the the El Mna composite intrusion (Fig. 1b) while intermediate–felsic rocks form the eastern part.

Figure 3. Field photos of the Jebilet magmatism: (a) view within the Kettara mafic–ultramafic layered intrusion showing upper Visean metaturbidites (hV) cropping out in anticline window overlaid by ultramafic cumulates (UM) and leucogabbros (LCB); (b–e) outcrops of the Kettara intrusion showing (b) centimetre-scale layering in the ultramafic cumulates (scale piece is 58 mm across); (c) a rapidly chilled and weakly folded doleritic dyke cross-cutting ultramafic cumulates; (d) a felsic dyke cross-cutting ultramafic cumulates and enclosing mafic enclaves; (e) the mafic enclaves are elongated and occur most abundantly at the intrusive contact, decreasing rapidly towards the centre of the dyke; (f) mafic enclaves within quartz-diorite in the Jbel Bouzlaf intrusion (scale piece is 22 mm across); (g) view showing an E–W cross-section of the Koudiat Bouzlaf microgranites (μγ) enclosing synplutonic mafic dykes (δ) and intruding upper Visean metaturbidites; and (h, i) outcrops of the cordierite-bearing granodiorite of the eastern Jebilet pluton showing (h) a dark magmatic microgranular enclave and (i) a large xenocryst of andalusite (scale piece is 22 mm across).
The felsic rocks of the bimodal association crop out as metre-wide dykes enclosing mafic enclaves within the mafic–ultramafic intrusions (Fig. 3d, e) or as stocks within composite mafic–felsic intrusions (e.g. Oled Har, El Mna). They also form elongated and stretched intrusions of more than 10 km in length and less than 700 m wide in a western lineament composed of the Koudiat Bouzlaf, Hamra and Diab intrusions (the BHD intrusions, Fig. 2a). They consist of microgranitic rocks that contain cross-cutting mafic dykes (synplutonic dolerites; Fig. 3g) and leucocratic microgranular enclaves. These granitoids are locally highly deformed and have been metasomatically altered to gneissic trondhjemites and tonalites (Essaifi et al. Reference Essaifi, Capdevila, Fourcade, Lagarde, Ballèvre and Marignac2004 b). The less-deformed granitoids are observed near the boundaries with country rocks where deformation is heterogeneous. They are monzonitic microgranites that have millimetre-scale phenocrysts in a micrographic–granophyric groundmass showing a weak planar fabric. The primary minerals include quartz, plagioclase, microcline, biotite and amphibole as essential minerals and fluorite, apatite, zircon, ilmenite and allanite as accessory minerals. The phenocrysts are quartz, euhedral plagioclase and aggregates of Cl- and Fe-rich biotite (annite) and blue-green amphibole (hastingsite-ferropargasite). The groundmass is composed of quartz aggregates, plagioclase and microcline showing micrographic and granophyric (spherolitic) associations. Plagioclase phenocrysts in these weakly altered microgranites are sericitized (phengitic muscovite), while biotite is partially replaced by chamosite or by pumpellyite and ilmenite is altered to leucoxene.
Intermediate rocks (quartz-diorites) are present in some composite intrusions where they are localized at the contact between the felsic and the mafic–ultramafic rocks. In these intrusions field evidence, including cross-cutting relationships between intrusions of differing compositions, net veining structures and magma mixing/mingling features, indicates contemporaneous emplacement of the felsic and mafic magmas (Fig. 3f). In the El Mna intrusion (Fig. 1b), the quartz-diorite exhibits a coarse (up to 5 cm) pegmatitic texture and contains plagioclase, amphibole, biotite, K-feldspar, quartz, calcite, chlorite, ilmenite, leucoxene, apatite and zircon. The rock is characterized by abundant (30–40 vol%) centimetre-scale acicular amphibole (ferro-hornblende) which is partially or completely replaced by biotite and chlorite, and contains inclusion trails of ilmenite. Plagioclase is present as subhedral crystals altered into calcite and sericite. Biotite can reach 25% of the total rock volume, and some biotite crystals have inclusions of zircon. The groundmass contains quartz that invades K-feldspar (microcline) leading to formation of secondary granophyric associations. Apatite occurs as inclusions in the other phases.
A 330.5+0.68/–0.83 Ma age was obtained for a microgranitic sample of the BHD intrusions by U–Pb dating of zircon using isotope dilution thermal ionization mass spectrometry (ID-TIMS) (Essaifi et al. Reference Essaifi, Potrel, Capdevila and Lagarde2003).The gabbroic rocks have not been dated but, as mentioned above, field evidence demonstrates contemporaneous emplacement of felsic and mafic magmas.
2.b.2. Granodioritic plutons
There are two main cordierite-bearing granodioritic plutons in the area: the eastern and central Jebilet plutons. Within the country rocks, tightening of folds in the metamorphic aureole indicates an increase of strain intensity towards the pluton boundaries (Le Corre & Saquaque, Reference Le Corre and Saquaque1987; Lagarde, Aït Omar & Roddaz, Reference Lagarde, Aït Omar and Roddaz1990). In the northern contact aureole of the eastern Jebilet pluton, injection of granitic magma occurs either perpendicular to or along the stratification plane. Within the plutons strain is generally low, and the pre-full-crystallization planar fabric is weak or absent (Boummane & Olivier, Reference Boummane and Olivier2007), but strain intensity increases towards the pluton margins where a well-defined planar fabric is present and S/C structures are frequent. Ultramylonites are developed in kilometre- to metre-scale shear zones within the plutons. The two plutons are essentially composed of porphyritic biotite±cordierite-bearing granodiorite, but modal compositions range from monzogranites to tonalites (A. Chemesseddoha, unpub. Ph.D. thesis, University of Rennes I, Rennes, 1986; M. K. Ben Salah, unpub. Ph.D. thesis, University Cadi Ayyad, Marrakesh, 1989; El Amrani El Hassani, Reference El Amrani El Hassani1996). The granodiorite is composed of K-feldspar megacrysts (2–3 cm) enclosed in a mesostasis (3–7 mm) of biotite, plagioclase, K-feldspar, cordierite and quartz. Biotite and muscovite±tourmaline-bearing leucogranites are also present at the boundaries between the plutons and the country rock, or as dykes and stocks that cut across the granodiorite. The leucogranites are affected by centimetre-scale shear zones with a mylonitic fabric.
Two types of enclaves are found in the plutons: (1) homogeneously distributed mafic microgranular enclaves with a tonalitic–dioritic composition (Fig. 3h), interpreted as resulting from mingling between a mafic, mantle-derived magma and the granodioritic magma (El Amrani El Hassani, Reference El Amrani El Hassani1996); and (2) aluminous and ferromagnesian xenoliths (made up of aluminosilicates, cordierite, garnet, spinel, biotite and feldspars) present mainly in the eastern Jebilet pluton (Fig. 3i). Hydrothermal alteration is marked in the granodioritic plutons by transformation of biotite into chlorite, K feldspar into muscovite and plagioclase into sericite, while cordierite is altered into pyrophyllite, pinnite and chlorite. The leucogranites are locally affected by extensive alteration leading to total disappearance of feldspar and development of greisens composed of quartz, muscovite and tourmaline.
In the granodioritic plutons Mrini et al. (Reference Mrini, Rafi, Duthou and Vidal1992) obtained an isochron at 327±4 Ma, an age that was considered to record emplacement. In the leucogranites that cut across the biotite±cordierite granodiorite Mrini et al. (Reference Mrini, Rafi, Duthou and Vidal1992) obtained an Rb–Sr isochron at 295±15 Ma, consistent with the ages of the other leucogranitic rocks elsewhere in the Western Meseta (e.g. the Oulmes leucogranitic pluton dated at 298±6 Ma (Rb–Sr whole-rock); 296±3 Ma (SHRIMP, U–Pb on zircon) and 308±8 Ma (U–Pb on monazite); Mrini et al. Reference Mrini, Rafi, Duthou and Vidal1992; Baudin et al. Reference Baudin, Chevremont, Razin, Thieblemont, Rachdi, Roger, Benhaourch and Winckel2001; Fig. 1a).
In summary, both the plutons of the bimodal association and the granodioritic plutons were emplaced in the Jebilet massif at c. 330 Ma. Later dykes and stocks of leucogranite were intruded into the granodiorite plutons and the country rocks at c. 300 Ma. On the basis of Sr and Nd initial isotope ratios at the time of crystallization, the present investigation aims to distinguish the sources of the different rock types and test if mixing between melts from different sources has occurred. In addition, isotope studies on Variscan magmatism in Morocco are rare (Z. Mrini, unpub. Ph.D. thesis, University of Clermont Ferrand, Clermont Ferrand, 1985; Gasquet et al. Reference Gasquet, Leterrier, Mrini and Vidal1992; Mrini et al. Reference Mrini, Rafi, Duthou and Vidal1992; Ajaji et al. Reference Ajaji, Weis, Giret and Bouabdellah1998) and this study on the Carboniferous magmatic rocks of the Jebilet massif can be used to place constraints on the genesis of the granitic plutons of the Variscan belt of Morocco.
3. Sampling and analytical methods
Depending on the grain size, samples weighing up to 5 kg were collected to represent the range of rock types exposed and to cover the whole area of the bimodal intrusions. Whole-rock geochemical analyses were carried out on samples taken from the different rock types of the main intrusions within the bimodal association, and used with whole-rock geochemical data on the Jebilet peraluminous granodioritic plutons (El Amrani El Hassani, Reference El Amrani El Hassani1996). For Sr–Nd isotopic studies samples were selected as representative fresh rock, and aim to cover the range of lithological variation within the intrusions. Samples were selected from: (1) mafic–ultramafic rocks of the Kettara mafic–ultramafic intrusion and of the Oled Har, J. Bouzlaf and El Mna composite intrusions; (2) felsic rocks of the BHD microgranitic lineament and the felsic dykes that cut across the Kettara intrusion and felsic–intermediate rocks of the composite intrusions; and (3) cordierite-bearing granodiorites of both the eastern and the western Jebilet plutons. Samples were used with unpublished Sr–Nd isotope data on the ganodiorites and leucogranites (Z. Mrini, unpub. Ph.D. thesis, University of Clermont Ferrand, Clermont Ferrand, 1985). The powder samples were prepared using an agate mortar.
Whole-rock chemical analyses were performed at the University of Rennes (France) by X-ray fluorescence spectrometry using a Philips PW 1404 sequential spectrometer. Accuracy for major elements is estimated at 1–3%, except for MnO and P2O5 (5%). For trace elements, accuracy is of the order 5% for concentrations lower than 30 ppm and 3% for concentrations higher than 30 ppm. Selected samples were analysed for rare Earth elements by inductively coupled plasma mass spectrometry (ICP-MS) at the Centre de Recherches Pétrographiques et Géochimiques (CRPG), Nancy, France) and at the Laboratoire de Géodynamique des Chaînes Alpines, Grenoble using a VG PQ2+ spectrometer, following the procedures described by Barrat et al. (Reference Barrat, Keller, Amossé, Taylor, Nesbitt and Hirata1996). The accuracy is estimated at 5% when chondrite-normalized concentrations are >10 ppm and at 10% when they are lower. These geochemical data are presented in Table 1.
Table 1. Major (wt%) and trace element (ppm) data of representative samples from the intrusive rocks of the Jebilet massif (for locations, see Fig. 1b)

aTotal Fe calculated as Fe2O3; bOriginal data for granodiorites and leucogranites from El Amrani El Hassani (Reference El Amrani El Hassani1996).
ASI = molar Al2O3/(CaO+Na2O+K2O); Mg no. = molar Mg/(Mg+Fe); – not available; Prd – peridotite; Trt – troctolite; Lcb – leucogabbro; Q-D – quartz-diorite; μGab – microgabbro; Dol – dolerite; μGr – granophyric microgranite; TTg – trondhjemite/tonalite gneiss; Grd – granodiorite; Lgr – leucogranite.
Sr and/or Nd isotopic compositions were analysed in 30 samples (Table 2), including different rock types of the bimodal association and one clinopyroxene separated from one gabbro as well as two samples from each granodioritic pluton. In addition, Sr isotopes were analysed in three host rock samples (Sarhlef schists) (Essaifi et al. Reference Essaifi, Capdevila, Fourcade, Lagarde, Ballèvre and Marignac2004 a). Sr–Nd isotopic analyses were carried out at the University of Rennes (France) using a Finnigan MAT 262 multicollector mass spectrometer. All measured 87Sr/86Sr were normalized to 86Sr/88Sr = 0.1194 and measured relative to NBS 987 Sr Standard = 0.71025. The error of 87Sr/86Sr, including the statistical error obtained during the mass spectrometer run and other error sources such as instrumental reproducibility, is estimated to be ±0.0003. Nd isotopic ratios were normalized to 146Nd/144Nd = 0.7219. Additional Sm–Nd isotopic analyses were performed at Syracuse University (USA) following the procedures described by Samson, Hibbard & Wortman (Reference Samson, Hibbard and Wortman1995). During the course of this study, the NBS 987 Sr standard yielded a mean 87Sr/86Sr of 0.710253 at Rennes University; the 143Nd/144Nd value for the La Jolla standard was 0.511855 at the Syracuse Laboratory and 0.511858 at Rennes University. Even though no samples were run at both laboratories, samples collected from the same intrusion (e.g. MOH1 and 00M04 microgranitic samples or OH6 and 00M03 gabbroic samples) yielded similar results, indicating that the Sm–Nd isotopic data from the two laboratories are in good agreement.
4. Results
4.a. Secondary alteration
The Variscan magmatic rocks of the Jebilet massif underwent the combined effects of hydrothermal alteration and strain (Essaifi et al. Reference Essaifi, Capdevila, Fourcade, Lagarde, Ballèvre and Marignac2004 a, b; Essaifi & Hibti, Reference Essaifi and Hibti2008; M. K. Ben Salah, unpub. Ph.D. thesis, University of Cadi Ayyad, Marrakesh, 1989). Element mobility associated with hydrothermal and deformation processes has resulted in minor geochemical changes in the undeformed rocks comparatively to the deformed rocks, where most major elements, especially Na, K, Ca, Mg and Fe, were mobilized. The large-ion lithophile elements (LILE) such as Rb, Ba, Sr, Zn, Pb and Cu were also mobilized. However, there is no evidence that the high-field-strength elements (HFSE), Ti, P, Th, Zr, Nb and Y, or the REE experienced significant mobility during hydrothermal alteration, except inside the fluid channel ways (shear zones). LILEs and related elements will therefore be avoided for the purposes of petrogenetic discussion.
4.b. The ultramafic–mafic rocks of the bimodal association
The mafic–ultramafic rocks include peridotites, gabbros, leucogabbros and dolerites. The majority of these rocks have clearly formed by cumulate processes, producing igneous layering; some mafic rocks are however found in dykes which are more likely to represent magmatic compositions. High Mg, Ni, Co and Cr contents characterize the peridotites due to olivine and spinel accumulation, and high Ca and Al contents in leucogabbros are due to plagioclase accumulation (Fig. 4). The non-cumulate rocks display intermediate Al2O3 (c. 14–15 wt%), MgO (c. 6–9 wt%) and CaO (c. 8–11 wt%) contents (Table 1). A noticeable silica gap (53–59%) separates the mafic–ultramafic series from the other rock units. The mafic–ultramafic rocks have Mg no. (Mg/Mg+Fe) 0.62–0.86 in the cumulate rocks and 0.53–0.7 in the non-cumulate rocks (Fig. 5a). They show a Fe-enriched trend in the FeO*/MgO versus SiO2 diagram of Miyashiro (Reference Miyashiro1974) (Fig. 5c) and in FeO* versus FeO*/MgO (Aarab & Beauchamp, Reference Aarab and Beauchamp1987). The mafic–ultramafic rocks have lower Nb/Y (<0.67) than alkaline basalts. In the TiO2 versus Zr/P2O5 diagram of Winchester & Floyd (Reference Winchester and Floyd1976) the non-cumulate mafic rocks plot in the tholeiitic field (Fig. 6a), which is consistent with the Nb/Y ratios and the Fe-enrichment. The mafic–ultramafic rocks are metaluminous (Fig. 7a). The non-cumulate mafic rocks have higher TiO2 and Fe2O3* contents than the cumulate rocks, and plot within the field of experimental peridotite melts (Fig. 6b).

Figure 4. Harker plots for selected major and trace elements of the Jebilet plutonic rocks. CaO, Al2O3, MgO and Fe2O3* contents of the main fractionating minerals in the mafic–ultramafic cumulates are also plotted. Ol – olivine; PLG – plagioclase; CPX – clinopyroxene.

Figure 5. Plot of (a) Mg no. and (b) K2O versus SiO2 for the Carboniferous Jebilet intrusive rocks. A silica gap exists between the mafic–ultramafic rocks and the granitoids where the scatter of data points is related to post-magmatic mobilization of K, Mg and/or Fe. The original data for the granodiorites and leucogranites are from El Amrani El Hassani (Reference El Amrani El Hassani1996). (c) Plot of the Jebilet mafic–ultramafic rocks in the FeO*/MgO versus SiO2 diagram of Miyashiro (Reference Miyashiro1974). Data points show a Fe-enriched trend.

Figure 6. (a) Plot of TiO2 versus Zr/P2O5 for non-cumulate mafic rocks of the Jebilet bimodal intrusive rocks. (b) Total Fe2O3 versus TiO2 diagram for the non-cumulate mafic rocks compared with fields for experimental peridotite melts (Falloon et al. Reference Falloon, Green, Hatton and Harris1988).

Figure 7. (a) SiO2 versus ASI (molar Al2O3/(CaO+Na2O+K2O)) index; and (b) cation ratio ((Na+K+2Ca)/(Al*Si)) versus Zr diagram (after Watson & Harrison, Reference Watson and Harrison1983). Symbols as in Figure 4. The original data for the granodiorites are from El Amrani El Hassani (Reference El Amrani El Hassani1996).
The mafic–ultramafic rocks have low but variable ∑REE contents (10–62 ppm, 1–10×chondrite), reflecting different abundances and compositions of intercumulus liquids. The lowest concentrations are in the peridotites (∑REE = 11 ppm) and the highest in the non-cumulate rocks (fringing microgabbros and doleritic dykes, ∑REE = 51–62 ppm), while the leucogabbros have intermediate concentrations (∑REE = 12–17 ppm). All the mafic–ultramafic rocks display flat ((Gd/Yb)N = 1.06–1.38) heavy rare Earth elements (HREE) patterns (Fig. 8a). The cumulate rocks have light rare Earth elements (LREE) depleted ((La/Yb)N = 0.4–0.9) patterns reflecting dominance of accumulated crystals over interstitial liquids, while the non-cumulate rocks (microgabbros and dolerites) have flat and linear patterns ((La/Yb)N = 1.1–1.7). Some mafic–ultramafic rocks display a small Eu anomaly, either positive (Eu/Eu* = 0.9–1.2) related to plagioclase accumulation or negative (Eu/Eu* = 0.65–0.9) related to olivine accumulation. All the analysed Jebilet mafic–ultramafic rocks have similar chondrite-normalized HREE patterns, suggesting that they were derived from a common mantle source.

Figure 8. Chondrite-normalized REE plot for representative rocks of the Jebilet magmatism: (a) mafic–ultramafic rocks; (b) granophyric microgranites; and (c) cordierite-bearing granodiorites. Normalizing values are from Evensen, Hamilton & O'Nions (Reference Evensen, Hamilton and O'Nions1978). Primitive mantle-normalized trace element diagrams for representative rocks of the Jebilet magmatism: (d) mafic–ultramafic rocks; (e) granophyric microgranites (εNd330 values of the microgranites in the corresponding intrusions are indicated); and (f) cordierite-bearing granodiorites. Normalizing values are from Sun & McDonough (Reference Sun, McDonough, Saunders and Norry1989). The original data for the granodiorites and leucogranites are from El Amrani El Hassani (Reference El Amrani El Hassani1996).
In the primitive mantle-normalized spiderdiagrams (Fig. 8d), the non-cumulate mafic rocks are characterized by flat HFSE patterns and lack the negative Nb anomaly that might be expected in calc-alkaline magmas. The cumulate samples generally have lower HFSE and REE contents than the non-cumulates, reflecting the incompatibility of these elements in the main cumulus minerals, and show positive or negative Sr anomalies due to plagioclase accumulation in leucogabbros and olivine accumulation in peridotites, respectively (Fig. 8d). The LILE are generally enriched relative to the REE and HFSE, a feature that can either be related to aqueous fluid involvement (Rollinson, Reference Rollinson1993, p. 146) or a characteristic of the source rocks.
The Sr isotopic compositions of the isotropic gabbros, both cumulate and non-cumulate, are variable. Initial 87Sr/86Sr calculated at 330 Ma lie in the range 0.7037–0.7087, and the intercumulus clinopyroxene measured on a mineral separate has an initial 87Sr/86Sr ratio of 0.7045 (Table 2). The 147Sm/144Nd are between 0.17 and 0.3 and the initial 143Nd/144Nd, calculated at 330 Ma, range from 0.51238 to 0.51265 (Table 2). The corresponding εNd(330) values vary from +8.7 to +2.5 (Fig. 9). εNd generally increases with increasing Mg no. (Fig. 10).

Figure 9. Initial 87Sr/86Sr versus initial εNd values of the Jebilet plutonic rocks. The granitoids of the Tichka plutonic complex are also plotted. A mixing curve between the mafic end-member deduced for the Tichka plutonic complex (Gasquet et al. Reference Gasquet, Leterrier, Mrini and Vidal1992) ((87Sr/86Sr)i = 0.7027, Sr = 700 ppm, Nd = 20 ppm, εNd,i = +7.6) and a bulk-sediment corresponding to the mean composition of the protolith from which most of the granites in Morocco were derived ((87Sr/86Sr)i = 0.718, εNd,i = −9, Sr = 150 ppm, Nd = 30 ppm) is shown. The bar represents (87Sr/86Sr)330 of the country rocks. Also shown are the fields of MORB and OIB (Wilson, Reference Wilson1989). The original data for the Jebilet granodiorites and leucogranites are from Z. Mrini (unpub. Ph.D. thesis, University of Clermont Ferrand, Clermont Ferrand, 1985) and data for the Tichka plutonic complex are from Gasquet et al. (Reference Gasquet, Leterrier, Mrini and Vidal1992).

Figure 10. Mg no. (as a differentiation index) versus εNd(330) in the Jebilet mafic–ultramafic rocks. The observed evolutionary trend (arrow) is consistent with mixing or assimilation and fractional crystallization (AFC) processes between a mafic end-member represented by GSK sample (Mg no. = 7 and εNd(330) = + 8.7) and an upper crustal end-member (bulk-sediment with Mg no. = 4 and εNd(330) = –9).
4.c. The microgranites and quartz-diorites of the bimodal association
The microgranites of the bimodal association are highly differentiated with SiO2 contents ranging from 70 to 80%. The abundances of immobile elements (Th, Nb, P, Zr, Ti, Y and REE) do not fluctuate significantly between samples (Fig. 8e), with low TiO2 and P2O5 contents in the ranges 0.2–0.5% and 0.03–0.06% respectively, except in the Oled Har microgranites where P2O5 content reaches 0.25% (Fig. 4). The microgranites have high Zr abundances of between 256 and 380 ppm except in the El Mna composite intrusion where the Zr content reaches 839 ppm (Fig. 4). Nb and Th contents are in the ranges 17–23 ppm and 29–39 ppm respectively, except in the Jbel Bouzlaf composite intrusion where Th content is as low as 17 ppm. Ga and Y contents are in the ranges 15–23 ppm and 57–115 ppm respectively. The least-altered granophyric microgranites, preserved in the outer parts of the BHD intrusions where deformation is heterogeneous, have relatively high K2O contents (Fig. 5b). They are metaluminous to weakly peraluminous (Fig. 7a) and show characteristics of A-type or ferroan granitoids: (1) petrographically, they contain Fe-rich biotite, hastingsite and fluorite; (2) geochemically, their Mg and Ca contents are low (MgO<0.5%, CaO<2%), total alkali contents are high (6–8%) and their Fe/Mg ratio is high (FeO/(FeO+MgO)>0.8; Fig. 11a); (3) they have relatively high zircon saturation temperatures (850–900°C, Fig. 7b), similar to temperatures based on zircon typology (850–900°C, Essaifi et al. Reference Essaifi, Potrel, Capdevila and Lagarde2003) and on amphibole geothermometry (Holland & Blundy, Reference Holland and Blundy1994; Essaifi, Reference Essaifi1995). There is no evidence for inherited zircon because separates obtained for previous geochronological studies show euhedral, transparent and colourless magmatic zircons that lack internal structures or visible cores (Essaifi et al. Reference Essaifi, Potrel, Capdevila and Lagarde2003). We therefore conclude that such temperatures reflect the temperature conditions during melting, indicating that the A-type microgranites represent high-temperature granitic melts. Transformation by loss of K and gain in Na and Ca resulted in moderately high total alkalis in the Jebilet microgranites (Essaifi et al. Reference Essaifi, Capdevila, Fourcade, Lagarde, Ballèvre and Marignac2004 b). The microgranites have relatively high Ga/Al (2.3–3.3) and plot within the A-type compositional field of Whalen, Currie & Chappell (Reference Whalen, Currie and Chappell1987), and especially within the A2-type granitoid field of Eby (Reference Eby1992) (Fig. 11b, c). This is also confirmed by the classification scheme of Frost et al. (Reference Frost, Barnes, Collins, Arculus, Ellis and Frost2001) using the diagram FeO*/(FeO*+MgO) versus SiO2 (Fig. 11a).

Figure 11. Plots of the (a) Jebilet intrusives in the classification diagram of Frost et al. (Reference Frost, Barnes, Collins, Arculus, Ellis and Frost2001) and (b) Jebilet microgranites (circles) and quartz-diorites (triangles) in the Zr versus 10000 Ga/Al diagram of Whalen et al. (Reference Whalen, Currie and Chappell1987), showing affiliation with A-type granites. (c) Nb–Y–Ga ternary diagram for the subdivision into A1- and A2-type granites (Eby, Reference Eby1992). Symbols as for Figure 4.
The granophyric microgranites have relatively high REE concentrations (∑REE = 288–386), the concentrations of those in the BHD felsic lineament being identical to those of the felsic rocks of the composite intrusions (Oled Har, El Mna, J. Bouzlaf). They are characterized by uniform patterns (Fig. 8b) with a moderate LREE to HREE fractionation ((La/Yb)N = 3.6–7.1, (La/Sm)N = 2.5–3.2), a constant negative Eu anomaly (Eu/Eu* = 0.3–0.4) and gently sloping HREE chondrite-normalized patterns ((Gd/Yb)N = 1–1.7). In a primitive mantle-normalized trace element plot, the microgranites show an overall enriched pattern except for depletion in Sr, P, Eu and Ti (Fig. 8e). In addition, Nb shows a significantly negative anomaly relative to the neighbouring elements ((Nb/La)N = 0.3–0.4).
Chemical compositions of the few intermediate rocks (quartz-diorites) present in the composite intrusions are variable. The SiO2 content varies between 59 and 69% and Fe2O3* between 5 and 12% (Fig. 4). In the El Mna composite intrusion, evolution from mafic–ultramafic rocks to felsic rocks is accompanied by Fe, Mn and P enrichments in the intermediate rocks (sample EM7, Table 1). Such enrichments are, however, absent from the Oled Har and Jebel Bouzlaf composite intrusions (samples OH4 and MBZN5, respectively). The quartz-diorites have contents of immobile elements similar to those in the felsic rocks, with the exception of Th and Nb contents which are lower and reach 8 and 10 ppm, respectively (sample EM7, Table 1). They have high Zr contents between 216 and 590 ppm (Fig. 4). The ranges of Ga and Y are 21–25 ppm and 42–99 ppm respectively. The quartz-diorites plot in the same alkaline fields as the granophyric microgranites (Fig. 11b, c).
The initial Sr isotopic ratios of the granophyric microgranites of central Jebilet range range from 0.7079 (0.7073 in the quartz-diorite of El Mna intrusion) to 0.7119. The147Sm/144Nd are between 0.13 and 0.15 and the initial 143Nd/144Nd, calculated at 330 Ma, range from 0.51189 to 0.51218. The corresponding εNd(330) values vary from –0.6 to –6.3 (Fig. 9). The isotopic range is more restricted when the intrusions are considered separately. εNd(330) values are: (1) –6.3 and –6.2 in the microgranites of the Oled Har intrusion; (2) between –2.6 and –3 in the BHD microgranitic intrusions; and (3) –0.6 and –0.7 in the felsic–intermediate rocks of El Mna and Jbel Bouzlaf composite intrusions. These results reveal a spatial variation in the microgranites characterized by an increase in the εNd(330) values westwards.
4.d. The cordierite-bearing granodiorites and associated leucogranites
The cordierite-bearing granodiorites form differentiated products of a calc-alkaline plutonic suite (Fig. 5b). The plutons also include monzogranites and tonalitic to dioritic magmatic enclaves (El Amrani El Hassani, Reference El Amrani El Hassani1996; Gasquet, Stussi & Nachit, Reference Gasquet, Stussi and Nachit1996). They have intermediate to acidic compositions (SiO2 = 64–76%), the most differentiated pluton being the central Jebilet granodiorite. They form continuous and regular trends, distinct from those of the microgranites (Fig. 4). Their biotite compositions correspond to those of calc-alkaline granitic magmas (El Amrani El Hassani, Reference El Amrani El Hassani1996; Gasquet, Stussi & Nachit, Reference Gasquet, Stussi and Nachit1996), but the systematic presence of magmatic cordierite in the plutons indicates the peraluminous character of the magma, which is also indicated by A/CNK > 1 (Fig. 7a) and a normative corundum content of 1.7–3.2% (El Amrani El Hassani, Reference El Amrani El Hassani1996; Gasquet, Stussi & Nachit, Reference Gasquet, Stussi and Nachit1996).
The cordierite-bearing granodioritic plutons show significant negative anomalies in Sr, Zr and Ti (Fig. 8f) and moderate negative anomalies in P and Eu. They have relatively low zircon saturation temperatures (700–800°C, Fig. 7b), similar to temperatures based on zircon typology and biotite geothermometry (El Amrani El Hassani, Reference El Amrani El Hassani1996). They have intermediate REE abundances (144–207 ppm); the contents in the central Jebilet pluton are identical to those of the eastern Jebilet pluton (El Amrani El Hassani, Reference El Amrani El Hassani1996). They are characterized by uniform REE patterns (Fig. 8c) with a slight to moderate LREE to HREE fractionation ((La/Yb)N = 4.56–6.98, (La/Sm)N = 2.93–3.34)), a moderate negative Eu anomaly (Eu/Eu* = 0.32–0.66) and flat to gently sloping HREE chondrite-normalized patterns ((Gd/Yb)N = 0.97–1.29). The leucogranites that cut across the granodiorites are highly differentiated (75 < SiO2 < 79%) and strongly peraluminous (A/CNK > 1, Fig. 7a). They have very low contents of CaO (<0.5%), Fe2O3* (<1.3%) and MgO (<0.2%) and higher Co contents than the microgranites (Fig. 4).
In the granodioritic plutons and their magmatic enclaves, the initial Sr isotopic ratios, calculated at 330 Ma, vary largely in the range 0.704–0.7108 while the initial Nd isotopic ratios range from 0.51186 to 0.51242 (Table 2). The corresponding εNd(330) values vary from –6.7 to –4.8 in the cordierite-bearing granodiorites, while an εNd(330) of +4.1 is found in a mafic microgranular enclave of dioritic composition (Fig. 9). The leucogranites have the highest initial 87Sr/86Sr isotopic ratios (0.7117–0.7177) and low εNd(300) values with the lowest εNd(300) value (−7.2) observed in the Jebilet Carboniferous magmatic rocks.
Table 2. Rb, Sr, Sm and Nd concentrations (ppm) and Sr and Nd isotopic ratios of the intrusive rocks of the Jebilet massif

aDepleted mantle model age (T DM) calculated following the model of DePaolo (Reference DePaolo1981 a).
bSamples with 147Sm/144Nd > 0.16 do not provide meaningful models.
cData from Z. Mrini, unpub. Ph.D. thesis, University of Clermont Ferrand, Clermont Ferrand, 1985.
Cpx – clinopyroxene separate from a gabbro; Mme – mafic magmatic enclave.
4.e. The host schist series
The country rocks of the Jebilet magmatism are predominantly metapelites derived from middle–upper Visean shales deposited in anoxic platform (Beauchamp, Reference Beauchamp1984) and are affected by a very-low- to low-grade metamorphism contemporaneous with a post-Visean shortening (Huvelin, Reference Huvelin1977). Their geochemical data suggest derivation from a continental magmatic arc (Moreno et al. Reference Moreno, Sáez, González, Almodóvar, Toscano, Playford, Alansari, Rziki and Bajddi2008). In the spiderdiagram of Figure 12, a representative sample of Sarhlef schists collected by composite sampling is compared to greywackes from passive-margin settings and active-margin settings, after Floyd (Reference Floyd, Morton, Todd and Haughton1991). A negative Sr anomaly, a positive V, Cr and Ni anomaly, a negative Ta–Nb anomaly and slight enrichments in Ti, Yb and LREE are observed in the Sarhlef schists relatively to the upper crust. There is a general correspondence to the continental arc and active-margin tectonic environment, but the presence of a negative Sr anomaly is similar to the passive-margin setting. Sr isotope analysis has been conducted on three samples collected by composite sampling (Essaifi et al. Reference Essaifi, Capdevila, Fourcade, Lagarde, Ballèvre and Marignac2004 a). Their 87Sr/86Sr values at the time of intrusion of the bimodal magmatic association and the granodioritic plutons (330 Ma) vary between 0.7077 and 0.7121 (Fig. 9).

Figure 12. Trace-element concentrations of the Sarhlef schists normalized to upper custal values (Taylor & McLennan, Reference Taylor and McLennan1995) and compared to expected patterns of sediments in active-margin and passive-margin settings, after Floyd (Reference Floyd, Morton, Todd and Haughton1991) and unpublished data (Essaifi).
5. Discussion
5.a. Potential magmatic processes
In situ fractional crystallization (FC) clearly played a major role in the development of the ultramafic–mafic rocks as indicated by mineral layering, cumulate textures and major and trace element geochemistry (e.g. Eu anomalies). However, field relationships, geochemistry and Sr and Nd isotopic compositions allow us to recognize that other petrogenetic processes, such as crustal assimilation, magma mixing and hydrothermal alteration, were also operative in the formation of the Jebilet plutons.
Field relationships provide evidence for magma mixing and mingling (dioritic zones and enclaves in the plutons), incorporation of crustal material (crustal xenoliths in the granodiorites) and metasomatic alteration. As far as possible, samples for geochemical and isotopic analysis were selected from areas that were not affected by significant metasomatism (therefore preserving a record of other petrogenetic processes). If fractional crystallization was the dominant process by which these magmas evolved, little change would be expected in some incompatible element ratios (e.g. La/Nb, Zr/Nb) or in isotopic ratios across the igneous suite. The wide variation in these ratios clearly indicates that magmas were derived from more than one source.
The Carboniferous Jebilet magmatic rocks show large variations in both initial Sr isotopic ratios and εNd values, which display a broad inverse correlation in the εNd versus Sri space (Fig. 9). Since the whole-rock Sm–Nd system is more resistant to late-stage low-grade perturbations than the Rb–Sr system, this indicates that the scatter of whole-rock Rb–Sr data is not solely related to post-magmatic perturbations but reflects complex petrogenesis. In the mafic–ultramafic rocks, increasing degrees of differentiation (monitored by the Mg no., Fig. 10) are associated with increasing amounts of crustal components (lower εNd values with decreasing Mg no.). Such a trend is consistent with mixing between mantle-derived and crust-derived magmas or an assimilation and fractional crystallization (AFC) process (DePaolo, Reference DePaolo1981 b). In the granodioritic samples and the mafic rocks there is a negative correlation between Yb contents and the size of the Eu anomaly, indicating that feldspar fractionation contributes to the size of the negative Eu anomaly in the mafic rocks and the granodiorites. There is however no correlation of Yb contents with the size of the negative Eu nomaly in the microgranites. The Eu anomaly in the microgranites is therefore not due to fractional crystallization of feldspar but is more likely a feature of the source rocks in which plagioclase was partly a residual phase.
Contamination of the microgranites during ascent and emplacement seems probable since they share the same initial isotopic ratios with the host schists (0.707–0.712). Simple contamination is however unlikely in the light of the Nd concentration. The microgranites have higher Nd concentrations (50.4–65.47 ppm) than the metasedimentary rocks (14.4–41.5 ppm; Belkabir et al. Reference Belkabir, Marcoux, Gibson, Lentz and Rziki2008; Essaifi, unpublished data) and the granodiorites and leucogranites (4.52–48.14 ppm) (Fig. 13). Simple contamination would require that the host schists provide an unacceptably high amount of the bulk Nd. In simple crustal assimilation models, the size of Nb anomaly and concentration of elements most affected by contamination (Ba, Rb, K, LREE, Sr; Thompson et al. Reference Thompson, Dickin, Gibson and Morrison1982) are expected to increase with progressively more negative εNd(T) values. These relationships are not observed among the microgranites (Fig. 8e). The Nb negative anomaly in the microgranites is therefore difficult to explain by AFC processes; rather it is a characteristic of the source rocks, similar to that of subduction-related magmas. Xenoliths and xenocrysts from the country rocks are widespread in the granodioritic plutons but are absent from the A-type microgranites, suggesting that chemical exchanges between the microgranites and country rocks are of limited extent. Furthermore, preservation of the different Nd isotopic signatures in the different microgranitic intrusions implies that different batches of magma were produced from different sources or mixtures of different sources. It is therefore suggested that distribution of data points in Figure 13 originates through mixing of different end-members rather than by simple contamination processes. Such end-members are discussed in more detail in the following.

Figure 13. (a) Plot of initial εNd values versus Nd concentrations.
5.b. Inferences on mantle and crustal sources
The mafic–ultramafic rocks have the most primitive isotopic signatures with the lowest (87Sr/86Sr)i and highest εNd,i values (Fig. 9), whereas the leucogranites and some microgranites are most evolved with the highest (87Sr/86Sr)i and lowest εNd,i values. The other microgranites and the quartz-diorites on the one hand and the granodiorites and their microgranular enclaves on the other form an intermediate group between the mafic–ultramafic rocks and the leucogranites, suggesting a hybrid origin.
5.b.1. Origin of the mafic–ultramafic rocks
Despite their large variations, the available initial εNd values (+8.7 to +2.5) and initial 87Sr/86Sr ratios (0.7037 to 0.7087) support a mantle-derived origin for the mafic–ultramafic series. Although many mantle reservoirs have been proposed (e.g. Rollinson, Reference Rollinson1993, p. 233), major possible sources are the subcontinental lithospheric mantle, the mantle wedge above a subduction zone or the asthenospheric mantle. Some fine-grained mafic rocks forming dykes or chilled margins in some of the intrusions may be considered to represent near-liquid compositions. These mafic rocks have consistent, flat trace element and REE patterns, in contrast to the cumulate samples which have more variable trace element compositions. However, even these rocks have rather variable isotopic compositions, suggesting that they have fractionated from slightly or significantly different batches of basic magma. The composition of the source region and parent magma of the mafic–ultramafic series can be approached from the least-contaminated samples in terms of Sr–Nd isotopes. The GSK sample is a fine-grained troctolite dyke that has the most primitive isotopic signature currently recorded in the Jebilet massif (εNd,i = +8.7, (87Sr/86Sr)i = 0.7037, Nd = 4.68 ppm, Sr = 65 ppm). This rapidly chilled dyke may represent a melt with a pristine asthenospheric mantle heritage and hence furnish a reliable estimate of the Sr and Nd isotopic composition of the mantle-derived suite. Its high Mg no. (0.7) and MgO as well as compatible element contents suggest that it represents a primary or near-primary melt. This is consistent with the flat to LREE depleted patterns of the non-cumulate mafic rocks (Fig. 8a), patterns that are similar to those of normalized mid-ocean ridge basalt (N-MORB). These non-cumulate rocks have high TiO2 contents and plot within the field defined by experimental melts of fertile peridotites (Fig. 6b), suggesting that they were most probably derived from fertile asthenosphere. Instrumental neutron activation analysis (F. Kharbouch, unpub. Ph.D. thesis, University of Bretagne occidentale, Brest, 1994) has however revealed that some mafic rocks of the bimodal association display geochemical features, implying that the asthenospheric mantle was affected by subduction processes. These rocks are relatively enriched in LILE and depleted in HFSE with Nb–Ta negative anomalies. Their Nb/U (14–56) and Th/U ratios (1.9–5.19) can be lower than those of MORB and ocean island basalt (OIB). Such geochemical features cannot be attributed solely to crustal contamination because Th/U ratios of crustal rocks are high (c. 5.0) and crustal assimilation will elevate Th/U ratios higher than those of MORB (c. 3.0) and OIB (c. 3.4) (Jiang et al. Reference Jiang, Zhao, Zhou, Liao and Gin2009). Th/U ratios of the slab-released hydrous fluid are low because U, relatively to Th, is preferentially transported in the aqueous fluid from the subducted slab to the mantle wedge (Keppler, Reference Keppler1996; Ayers, Reference Ayers1998). Based on immobile-discrimination diagrams such as the Th–Hf–Ta diagram of Wood (Reference Wood1980), the Jebilet mafic rocks include both MORB-like and destructive plate-margin-like basaltic compositions (Fig. 14a). Such a coexistence of MORB-like and arc-like tholeiitic basalts has also been described in other bimodal associations involving A2-type rhyolites and tholeiitic basalts (e.g. the Topsails igneous suite in the Newfoundland Appalachians, Whalen et al. Reference Whalen, McNicoll, van Staal, Lissenberg, Longstaffe, Genner and van Breeman2006). For this reason we believe that, although contamination has played a role in modifying their composition, mafic rocks of the Jebilet massif are thought to be derived from different mantle sources: a depleted MORB mantle and a mantle wedge above a subduction zone.

Figure 14. (a) Distribution of the Jebilet mafic rocks in the Th–Hf–Ta discrimination diagram of Wood (Reference Wood1980). (b) Plot of Th/Yb versus Ta/Yb. Vectors shown indicate the influence of subduction components (S), within-plate enrichment (W), crustal contamination (C) and fractional crystallization (F). Dashed lines separate the boundaries of the tholeiitic (TH), calc-alkaline (CA) and shoshonitic (SH) fields (after Pearce, Reference Pearce, Hawkesworth and Norry1983). Original data from F. Kharbouch, unpub. Ph.D. thesis, University of Bretagne Occidentale, Brest, 1994. The mafic rocks of Table 1 are also included in (a) considering the ratios Nb/Ta = 16 and Zr/Hf = 39.
Gasquet et al. (Reference Gasquet, Leterrier, Mrini and Vidal1992) showed that mafic rocks (gabbros and diorites) from the nearby Variscan Tichka plutonic complex (Fig. 1a) are derived from an upper mantle source, and that this high-temperature magma provided the heat for the production of granitoid magmas by partial melting of the continental crust. On the Nd–Sr isotope diagram (Fig. 9), the Tichka plutonic rocks plot within the mantle array as defined by uncontaminated oceanic basalts, whereas most of the Jebilet mafic rocks plot to the right of this field. Clearly the Nd–Sr isotope data of the Jebilet mafic rocks could be accounted for simply by partial melting of a depleted asthenospheric mantle wedge (MORB source mantle) enriched in radiogenic Sr by slab-derived fluids. The fact that Th/Yb ratios are displaced towards higher values in the Th/Yb versus Ta/Yb diagram (Fig. 14b) provides strong evidence for involvement of such slab-derived fluids in the genesis of the Jebilet mafic rocks.
5.b.2. Origin of the microgranites
The Jebilet granophyric microgranites show geological and geochemical features that are characteristic of A-type or ferroan granites (according to criteria proposed by several authors e.g. Collins et al. Reference Collins, Beams, White and Chappell1982; Whalen, Currie & Chappell, Reference Whalen, Currie and Chappell1987; Eby, Reference Eby1992; Frost et al. Reference Frost, Barnes, Collins, Arculus, Ellis and Frost2001): (1) they were intruded to very high levels in the crust as indicated by granophyric intergrowths along with comagmatic subvolcanics emplaced at the same structural level; (2) they contain interstitial Fe and Cl-rich biotite, hastingsite and fluorite; (3) their Mg and Ca contents are low and their REE (except Eu) and HFSE contents are high (Fig. 8b, e); and (4) they have high magmatic temperatures (up to 900°C, Fig. 7b) and their Fe/Mg and Ga/Al ratios are high (Fig. 11a, b). High Rb/Nb and Y/Nb ratios (Fig. 11c) further suggest that these granites belong to the A2 group of Eby (Reference Eby1992), a group of alkaline granitoids derived from continental crust or underplated crust that has been through a cycle of continent–continent collision or the waning stages of arc magmatism (whereas the A1-type granites emplaced in continental rifts or during intraplate magmatism and represent differentiates of magmas derived from OIB-like sources) (Eby, Reference Eby1992).
The A-type magmas are regarded as differentiation products of mantle-derived melts through extensive fractional crystallization (e.g. Turner, Foden & Morrisson, Reference Turner, Foden and Morrisson1992; Bonin, Reference Bonin and Demaiffe1996; Litvinovsky et al. Reference Litvinovsky, Jahn, Zanvilevich, Saunders, Poulain, Kuzmin, Reichow and Titov2002; Mushkin et al. Reference Mushkin, Navon, Halicz, Hartmann and Stein2003) or as partial melts of specific crustal protoliths, either: a granulitic residue from which a granitic melt was previously extracted (Collins et al. Reference Collins, Beams, White and Chappell1982; Clemens, Reference Clemens1986; Whalen, Currie & Chappell, Reference Whalen, Currie and Chappell1987; Creaser, Price & Wormald, Reference Creaser, Price and Wormald1991); a charnockitic rock (Landenberger & Collins, Reference Landenberger and Collins1996); a hornblende-bearing granitoid (Patiño Douce, Reference Patiño Douce1997); or a granulitic metasedimentary rock (Huang et al. Reference Huang, Li, Li and Li2011). They could also result from hybridization between anatectic granitic and mantle-derived mafic magmas (Bédard, Reference Bédard1990; Kerr & Fryer, Reference Kerr and Fryer1993; Mingram et al. Reference Mingram, Trumbull, Littman and Gerstenberger2000; Yang et al. Reference Yang, Wu, Chung, Wilde and Chu2006), coupled with fractionation processes (Barker et al. Reference Barker, Wones, Sharp and Desborough1975; Wickham et al. Reference Wickham, Alberts, Zanvilevich, Litvinovsky, Bindeman and Schauble1996).
The presence of a ‘Daly gap’ between mafic–ultramafic rocks and the microgranites and some crust-like geochemical and isotopic features argues against a simple magma differentiation model. Th concentrations in the Jebilet microgranites (20–40 ppm; Table 1) are higher than in the oceanic plagiogranites (Th<5 ppm; Pearce, Harris & Tindle, Reference Pearce, Harris and Tindle1984), suggesting that these microgranites do not result from the differentiation of the penecontemporaneous tholeiitic basalts (Th≤2 ppm). For the same SiO2 content, the microgranites of Central Jebilet are especially poor in Ti and Fe compared to the granophyres associated with well-known layered tholeiitic intrusions (e.g. McBirney, Reference McBirney1989; Turner, Foden & Morrisson, Reference Turner, Foden and Morrisson1992). In any case, the Sr initial isotopic ratios and the εNd values of the granophyric microgranites are distinct from those of the associated gabbroic rocks (Fig. 9) and leave no doubt that the microgranites did not form simply by extensive fractional crystallization from the coeval mantle-derived mafic magmas. Their origin should therefore involve continental crust, either lower or upper crust.
High REE contents but moderate εNd(330) values imply that the sources are not strongly evolved and preclude the derivation of the A-type microgranites from granulitic metasedimentary rocks. The high HREE (Yb>6.22 ppm, up to 10.5 ppm) and Y (>69 ppm, up to 115 ppm) contents and flat to gently sloping HREE patterns (Fig. 8b) of the Jebilet microgranites preclude garnet as a residual phase, which implies low pressure (<7 kbar, Patiño Douce, Reference Patiño Douce1997). Based on comparisons with experimentally produced melts from a variety of crustal lithologies (Holloway & Burnham, Reference Holloway and Burnham1972; Helz, Reference Helz1976; Spulber & Rutherford, Reference Spulber and Rutherford1983; Beard & Lofgren, Reference Beard and Lofgren1991; Skjerlie & Johnston, Reference Skjerlie and Johnston1993; Patiño Douce, Reference Patiño Douce1997), tonalitic sources are found to be the most consistent with observed compositions of these granophyric microgranites. Melting experiments of Patiño Douce (Reference Patiño Douce1997) demonstrated that dehydration melting of hornblende-bearing granitoids in the shallow crust (P≤4 kbar, at depths of 15 km or less) is a likely origin for high-silica metaluminous A-type granites. At 4 kbar and a melt fraction of 20–40%, plagioclase and clinopyroxene are the dominant residual phases of dehydration melting of hornblende-bearing granitoids (Patiño Douce, Reference Patiño Douce1997). Good matches between the composition of the microgranites and the experimentally produced melts from tonalitic sources support a crustal anatectic origin.
The Jebilet granophyric microgranites have Proterozoic depleted-mantle model ages (T DM c. 1.91–1.36 Ga), suggesting that an older crustal component exists in these Carboniferous granitoids. Such old crust, which crops out under the Palaeozoic cover in the Anti-Atlas inliers and is recognized by gravity data under the Palaeozoic formations of the Jebilet massif (Bernardin et al. Reference Bernardin, Cornée, Corsini, Mayol, Muller and Tayebi1988), has been dated in granulitic enclaves of the Triassic lamprophyre dykes that cross-cut the Jebilet massif (Dostal et al. Reference Dostal, Keppie, Hamilton, Aarab, Lefort and Murphy2005) and in the nearby Rehamna massif (Baudin et al. Reference Baudin, Chevremont, Razin, Youbi, Andries, Hoepffner, Thieblemont, Chihani and Tegyey2002). The microgranitic samples from the Oled Har intrusion have the lowest εNd value (–6.3) of the microgranites. Their T DM ages (c. 1.9 Ga) point to the Eburnean basement (c. 2 Ga) while the other microgranites have lower T DM ages (1350–1550 Ma) that seemingly require a mafic or juvenile (mantle) component. The coeval association of the microgranites with mafic magmas and the presence of net-veining structures and mafic enclaves within the microgranites suggest interaction between felsic and mafic magmas. In the εNd versus Sri space (Fig. 9), the microgranites are characterized by higher initial 187Sr/186Sr ratios and lower initial εNd values than the mafic rocks. Their field appears to extrapolate back to that of the associated mafic rocks, suggesting some form of mixing process, but this broad inverse correlation cannot be fitted by a single curve diagnostic of a simple two-component mixing. The microgranites trend could represent a variable degree of mixing between a mantle source variably enriched by slab-derived fluids and partial melts of the Eburnean metamorphic basement. This is consistent with the fact that the microgranites show an increase in the mantle contribution from east to west, suggesting the existence of independent reservoirs in which magma batches evolved independently by fractional crystallization and magma mixing processes.
Curved evolutions for some elements (Ti, Fe, P, V, Zr) in the bimodal association indicate that mineral fractionation was a main petrogenetic process (Fig. 4). Such evolutions are observed in the El Mna intrusion where the quartz-diorite and the microgranite have a same initial εNd value (−0.6), consistent with fractional crystallization. The εNd value (+2.5) of the associated mafic rock requires assimilation of continental crust in order to produce the Nd isotopic composition of the quartz-diorite and microgranite. The chemical and Sr–Nd isotopic compositions of some microgranites can therefore be explained by a complex petrogenetic process combining fractional crystallization and magma mixing between mantle-derived magmas and crust-derived magmas (represented by the Oled Har microgranites). The differences in the isotopic compositions of the microgranites can be related to the heterogeneity of the mantle sources and to variable degrees of crustal and mantle contribution.
5.b.3. Origin of the granodiorites and leucogranites
The Jebilet granodioritic plutons are emplaced at high structural levels, they contain biotite, cordierite and ilmenite and are strongly peraluminous. They can therefore be attributed to the S-type granite group of Chappell & White (Reference Chappell and White1974). Such granites are largely produced by partial melting of metasedimentary rocks. Barbarin (Reference Barbarin1996) has distinguished two groups among the S-type granites: (1) biotite-rich, cordierite-bearing granitoids (CPG-type) and (2) muscovite-bearing granitoids (MPG-type). The CPG granitoids are suggested to be produced by ‘dry’ anatexis of crustal rocks enhanced by underplating or injection of hot mantle-derived magmas, which can be preserved as microgranular mafic enclaves within the CPG (Barbarin, Reference Barbarin1996). As the Jebilet granodioritic plutons contain numerous microgranular mafic enclaves, they can be attributed to the CPG type.
The T DM ages of the granodioritic plutons (1.76–0.85 Ga) require a Proterozoic source rock. Their strongly peraluminous signature suggests that the source rocks were mainly metasedimentary rocks rather than meta-igneous rocks. However, the plutons display large variations in Sr–Nd isotopic compositions that may indicate the presence of a mantle-derived component, and the granodiorite plutons contain numerous microgranular mafic enclaves. As suggested by Barbarin (Reference Barbarin1996), these rocks might therefore be derived from anatexis of crustal rocks induced by underplating or injection of hot mantle-derived magmas. The granodioritic plutons have intermediate HREE (2.42 ppm < Yb < 4.47 ppm) and Y (21–34 ppm) contents and overall display flat to gently sloping HREE patterns ((Gd/Yb)N = 1–1.7). Fractionation among the HREE, a common feature of S-type granites (e.g. Bernard-Griffiths et al. Reference Bernard-Griffiths, Peucat, Sheppard and Vidal1985), would indicate the presence of garnet in the residual source, but this feature is lacking in the cordierite-bearing granodiorites of the Jebilet. These magmas were therefore more likely produced by relatively low-temperature (c. 750°C) anatexis of pelitic sources induced by injection of basalt magmas in the shallow crust. The produced crustal melts underwent hybridization with the coeval basalt magmas and assimilation of pelitic metasediments during ascent of the magmas in the crust. The process of wall-rock assimilation was documented in the Jebilet granodioritic plutons by the occurrence of inherited metamorphic cordierites (which are partly digested pelitic xenoliths), picked up at 750°C and 3.5 kbar by the ascending magma (Bouloton, Reference Bouloton1992), and therefore representing relics of the assimilated rocks (Fourcade et al. Reference Fourcade, Capdevila, Ouabadi and Martineau2001). The large variation in the initial Sr isotopic ratios (0.704–0.7108) and the εNd(330) values (−6.7 to +4.1) in the granodioritic plutons indicates isotopic heterogeneity that results from mixing between crust-derived and mantle-derived magmas (Mrini et al. Reference Mrini, Rafi, Duthou and Vidal1992) and wall-rock assimilation. The granodiorites define continuous and regular trends in major and trace element diagrams (Fig. 4). In the εNd versus Sri diagram (Fig. 9) the granodiorites and their microgranular enclaves scatter around a hyperbolic mixing curve between a crustal end-member, corresponding to the mean composition of the protolith from which most of the granites in Morocco were derived (Z. Mrini, unpub. Ph.D. thesis, University of Clermont Ferrand, Clermont Ferrand, 1985), and the mantle end-member of the Tichka plutonic complex.
The two mica-leucogranites are strongly peraluminous and can be described as S-type granites of Chappell & White (Reference Chappell and White1974) and muscovite-bearing granitoids (MPG-type) (Barbarin, Reference Barbarin1996). The initial Sr isotopic ratio (0.7177) and the εNd(330) value (−7.2) of the Jebilet leucogranites are consistent with direct derivation from crustal sources with a major contribution of aluminous metasedimentary rocks.
5.c. Magma generation/tectonic implications
The question of the heat source required for crustal melting during granitoid genesis is a long- debated topic, with (1) purely crustal mechanisms involving crustal thickening and total heat supply by decay of radioactive elements (e.g. England & Thompson, Reference England, Thompson, Coward and Ries1986) and (2) mantle–crust mechanisms involving heat input from the mantle into the crust in various geodynamic situations, such as asthenospheric upwelling (hot spots or extension zones), arc genesis and lithospheric delamination or slab break-off in orogenic zones (e.g. Harris, Pearce & Tindle, Reference Harris, Pearce, Tindle, Coward and Ries1986; Bussy, Hernandez & von Raumer, Reference Bussy, Hernandez and von Raumer2000; Whalen et al. Reference Whalen, McNicoll, van Staal, Lissenberg, Longstaffe, Genner and van Breeman2006).
The spatial and temporal association of mafic magmatism with alkaline and calc-alkaline felsic magmatism in the Jebilet massif argues for mafic magma-driven partial melting. Among the geodynamic settings where the mantle is classically implicated, hot spots or extension zones can be ruled out on the basis of regional tectonics. Relationships between deformation, magmatism and metamorphism indicate that emplacement of the granodioritic plutons and the bimodal plutonism in the Jebilet massif, as well as emplacement of the wider Carboniferous plutonic suite in the Moroccan Meseta, was contemporaneous with a syntectonic low-pressure regional metamorphism and development of ubiquitous, upright axial plane cleavages, unambiguously indicating horizontal shortening at the orogenic scale (Lagarde, Aït Omar & Roddaz, Reference Lagarde, Aït Omar and Roddaz1990). Models involving local lithospheric extension in strike-slip-induced basins (e.g. Mitjavila, Marti & Soriano, Reference Mitjavila, Marti and Soriano1997; Essaifi et al. Reference Essaifi, Potrel, Capdevila and Lagarde2003) are also not sustainable because the lithospheric thinning required to induce asthenospheric melting is much too great to be a result of strike-slip shearing during plate convergence. In central Jebilet, syntectonic emplacement of magmatic intrusions is consistent with the regional strain pattern that involves NE–SW extension (Fig. 1b), parallel to the southern prolongation of the western Meseta shear zone (Piqué, Jeannette & Michard, Reference Piqué, Jeannette and Michard1980; Lagarde & Michard, Reference Lagarde and Michard1986). The NE–SW extension is accompanied by lateral extrusion of the central Jebilet block and by synconvergence exhumation of the Proterozoic basement and intermediate P–T metamorphic rocks in the Rehamna massif (Aghzer & Arenas, Reference Aghzer and Arenas1998; Baudin et al. Reference Baudin, Chevremont, Razin, Thieblemont, Rachdi, Roger, Benhaourch and Winckel2001). Such an orogen-parallel extension with exhumation is intimately related to continental collision and occurs even during the early stages of convergence (Seyferth & Henk, Reference Seyferth and Henk2004).
The Jebilet Carboniferous magmatic rocks were emplaced at c. 330 Ma into slightly older upper Visean (350–333 Ma) marine syntectonic ‘flysch’ metasediments. The positive V–Cr–Ni–Ti anomalies observed in these sediments (Fig. 12) are indicative of a mafic input involving oceanic crust, possibly obducted onto the continent during a collision event (Floyd, Reference Floyd, Morton, Todd and Haughton1991; Totten, Hanan & Weaver, Reference Totten, Hanan and Weaver2000). Their spiderdiagram, with a general correspondence to the continental arc and active-margin tectonic environment and the presence of a negative Sr anomaly similar to the passive-margin setting, is best described by mixing between a dominant passive-margin source and a sediment from an active margin setting (Totten, Hanan & Weaver, Reference Totten, Hanan and Weaver2000). In Central Morocco, Carboniferous syntectonic flysch and catastrophic sediments were deposited in a compressional retro-foreland basin where interbedded basaltic lavas, doleritic dykes and gabbro sills were emplaced during thrusting (Ben Abbou et al. Reference Ben Abbou, Soula, Brusset, Roddaz, Ntarmouchant, Driouch, Christophoul, Bouabdelli, Majesté-Menjoulas, Béziat, Debat and Déramond2001; Roddaz et al. Reference Roddaz, Brusset, Soula, Beziat, Benabbou, Debat, Driouch, Christophoul, Ntarmouchant and Deramond2002). In the Guemassa massif at the southern prolongation of the Jebilet massif (Fig. 1), basaltic dykes and rhyolites were also emplaced during thrusting. The Moroccan Variscan crust therefore seems to have thickened by extensive sedimentation, volcanism and minor intrusion in the upper crust, with synchronous magmatic underplating and ductile deformation at depth. Such crustal thickening characterizes subduction-related orogens (Lamb et al. Reference Lamb, Hoke, Kennan, Dewey, Burg and Ford1997).
In this context one possible extrusive equivalent of the bimodal association of Central Jebilet is the bimodal basalt-subalkaline/peralkaline rhyolite province of the Southern British Caledonides which is also associated with polymetallic sulphide mineralization (Leat et al. Reference Leat, Jackson, Thorpe and Stillman1986; Eby, Reference Eby1992; Thorpe et al. Reference Thorpe, Leat, Mann, Howells, Reedman and Campbell1993). This association was emplaced within a shallow marine environment in a tectonic setting associated with closure of the Lower Palaeozoic Iapetus Ocean, cessation of oceanic subduction and development of strike-slip tectonism. Because the Moroccan Hercynides are thought to be related to continental subduction (Lagarde, Reference Lagarde1989; Piqué & Michard, Reference Piqué and Michard1989; Piqué et al. Reference Piqué, Bossière, Bouillin, Challouan and Hoepffner1993; Roddaz et al. Reference Roddaz, Brusset, Soula, Beziat, Benabbou, Debat, Driouch, Christophoul, Ntarmouchant and Deramond2002), the bimodal association of central Jebilet can be interpreted as resulting from cessation of continental subduction and development of strike-slip tectonism during collision (Fig. 15).

Figure 15. Cartoons showing crust–mantle interaction and subsequent melting and intrusion of Carboniferous granitic and gabbroic intrusions of the Moroccan Mesetas. (a) Westward subduction, partial melting of the lithospheric mantle and generation of potassic–shoshonitic granitic intrusions in the eastern Meseta at c. 350 Ma. (b) Cessation of subduction at c. 330 Ma, synconvergence extension and exhumation of intermediate P–T metamorphic rocks, upwelling of asthenospheric melts, convective removal of the lithospheric mantle, partial melting in the shallow crust and generation of coeval A-type granitic magmas and cordierite-bearing granodioritic plutons. (c) Progressive replacement of the lithosphere by the asthenosphere, leading to generation of leucogranites of purely crustal origin at c. 300 Ma.
Possible models leading to the generation of mafic magmas and crustal melting in collisional orogens include slab break-off (e.g. Davies & von Blanckenburg, Reference Davies and von Blanckenburg1995), convective lithospheric erosion (Houseman, McKenzie & Molnar, Reference Houseman, McKenzie and Molnar1981) and large-scale delamination of the lithosphere (e.g. Nelson, Reference Nelson1992). Taking into account the evolution of magmatism in the Jebilet massif as well as in the Moroccan Meseta (Mrini et al. Reference Mrini, Rafi, Duthou and Vidal1992; El Hadi et al. Reference El Hadi, Simancas Cabrera, Tahiri, González Lodeiro, Azor Pérez and Martínez Poyatos2006) from calc-alkaline granitoids and mafic magmas of mixed origin to leucogranites of a purely crustal origin and synchronous emplacement of these magmas with regional deformation, convective lithospheric erosion during crustal thickening (Loosveld & Etheridge, Reference Loosveld and Etheridge1990) seems more consistent with geochemical and Sr–Nd isotopic constraints. Convective thinning/erosion of the lithospheric mantle induces partial melting of subduction-metasomatized subcontinental lithospheric mantle, producing early potassic magmas and progressing to intra-plate depleted asthenospheric melts over time (Mahéo et al. Reference Mahéo, Guillot, Blichert-Toft, Rolland and Pecher2002). Potassic to shoshonitic calc-alkaline I-type granitoids crop out in the eastern Moroccan Meseta where they post-date an early Eo-Variscan folding phase. According to Ajaji et al. (Reference Ajaji, Weis, Giret and Bouabdellah1998), the Tanncherfi intrusive complex, emplaced at 344±6 Ma, was derived from mantle sources enriched in LILE and LREE by a subduction process. The evolution from continental lithospheric mantle signature in the Tanncherfi intrusive complex to the asthenospheric signature in the Jebilet massif, as well as in the Tazekka and Tichka massifs (Gasquet et al. Reference Gasquet, Leterrier, Mrini and Vidal1992; Chalot-Prat, Reference Chalot-Prat1995), reflects a lithosphere being progressively heated from below (Houseman, McKenzie & Molnar, Reference Houseman, McKenzie and Molnar1981; Nelson, Reference Nelson1992). Progressive replacement of the lithosphere by the asthenosphere results in elevated Moho temperatures and thus favours crustal melting at sequentially shallower levels (Fig. 15). The calc-alkaline granitoids become increasingly crustally contaminated and are succeeded by partial crustal melts (Turner et al. Reference Turner, Platt, George, Kelly, Pearson and Nowell1999; Wang et al. Reference Wang, Chung, O'Reilly, Sun, Shinjo and Chen2004). According to structural, geochemical and Sr–Nd isotopic data, erosion of the mantle lithosphere (thinning) was initiated during crustal thickening and the induced thermal anomaly was responsible for both magmatism and metamorphism in the Variscan Moroccan Meseta.
6. Conclusion
In the Jebilet massif, the post-Visean crustal shortening was accompanied by an orogen-parallel extension, lithospheric thinning, mantle uplift and progressive melting of the depleted mantle, which produced basaltic magmas. These basalts ascended into the continental crust, including the upper crust, where they formed crustal magma chambers. Temperature elevation due to mantle uplift and basalt emplacement induced crustal anatexis. High-temperature (c. 900°C) melting of tonalitic sources produced A2-type granitoids, while at low temperatures (c. 750°C) anatexis of metasediments produced cordierite-bearing granitoids. During this evolution, contamination/mixing occurred between mantle-derived magmas and crustal melts, contributing to the large variations of εNd(330) and (87Sr/86Sr)i values observed in both the cordierite-bearing granitoids and the A2-type microgranites. Further contamination occurred by assimilation of country rocks during ascent of the magmas to high crustal levels.
The Jebilet magmatism is an example of granitoid magma production in a complex tectonic setting where plate convergence interacts with a deep process, promoting mantle and crust activation. Structural, geochemical and Sr–Nd isotopic constraints argue for convective thinning/erosion of the lithospheric mantle during thickening. The thermal anomaly induced by the convective thinning of the mantle lithosphere is likely to have brought the heat energy that caused melting of the underlying asthenosphere and coeval production of calc-alkaline and alkaline granitoids, followed by the production of leucogranites.
Acknowledgements
Constructive comments and suggestions by M. Fowler and another anonymous reviewer greatly improved the presentation of data and the discussion; these comments are highly appreciated, although some wishes could unfortunately not be fulfilled. We wish to thank J. A. Barrat and G. Gruau from Geosciences Rennes for providing some of the Sr–Nd isotope and REE data used in this study. Sm–Nd isotopes were analysed at Syracuse University during post-doctoral research funded by the Fulbright program. We thank S. Fourcade for a review of an early version of this paper. This work represents a contribution to the project URAC 43.