INTRODUCTION
The nature and intensity of emotional experiences can be moderated by psychological operations collectively known as emotion regulation processes (MacLeod & Grafton, Reference MacLeod, Grafton and Gross2014). The ability to effectively regulate emotions is critical to successful social functioning, and disruptions to emotion regulation are associated with an increased risk of psychopathology (Campbell-Sills, Ellard, & Barlow, Reference Campbell-Sills, Ellard, Barlow and Gross2014; Joormann & Quinn, Reference Joormann and Quinn2014; Young, Sandman, & Craske, Reference Young, Sandman and Craske2019). Difficulty with emotion regulation has been documented in childhood brain tumor survivors; patients treated with high doses of craniospinal irradiation (CSI) exhibited more emotion regulation problems than their siblings (Armstrong et al., Reference Armstrong, Liu, Yasui, Huang, Ness, Leisenring and Packer2009), children treated for posterior fossa (PF) tumors displayed impaired cognitive control of emotions on an emotional Stroop task (Hopyan, Laughlin, & Dennis, Reference Hopyan, Laughlin and Dennis2010), and children treated for medulloblastoma made less use of adaptive cognitive emotion regulation strategies than typically developing children (Law et al., Reference Law, Smith, Greenberg, Bouffet, Taylor, Laughlin and Mabbott2017). There is also evidence that children treated for brain tumors experience social problems, anxiety, depression, and diminished adaptive functioning (Beebe et al., Reference Beebe, Ris, Armstrong, Fontanesi, Mulhern, Holmes and Wisoff2005; Schultz et al., Reference Schultz, Ness, Whitton, Recklitis, Zebrack, Robison and Mertens2007; Zeltzer et al., Reference Zeltzer, Recklitis, Buchbinder, Zebrack, Casillas, Tsao and Krull2009). Additionally, poor emotional control was recently shown to be a risk factor for poor social competence in pediatric brain tumor survivors (Barrera et al., Reference Barrera, Atenafu, Schulte, Bartels, Sung, Janzen and Zelcer2017). Thus, difficulty with emotion regulation may underlie some of the social and emotional challenges experienced by brain tumor survivors.
Emotion regulation is thought to be automatic or deliberate, and that both types exist on opposite ends of a continuum (Mauss, Bunge, & Gross, Reference Mauss, Bunge and Gross2007). In the dual-process model of emotion regulation, automatic emotion regulation is evoked by the stimulus and occurs without insight or awareness (implicit), whereas deliberate emotion regulation requires conscious effort, insight and awareness (explicit) (Gyurak, Gross, & Etkin, Reference Gyurak, Gross and Etkin2011). Despite being an inherently dynamic process, there are currently limited means to objectively evaluate emotion regulation in a manner that captures its time course.
Emotional functioning in children treated for brain tumors has most commonly been evaluated using standardized parent-report or self-report questionnaires (Beebe et al., Reference Beebe, Ris, Armstrong, Fontanesi, Mulhern, Holmes and Wisoff2005; Schultz et al., Reference Schultz, Ness, Whitton, Recklitis, Zebrack, Robison and Mertens2007; Zeltzer et al., Reference Zeltzer, Recklitis, Buchbinder, Zebrack, Casillas, Tsao and Krull2009), although a number of studies have utilized behavioral measures to assess social and emotional functioning in survivors (Bonner et al., Reference Bonner, Hardy, Willard, Anthony, Hood and Gururangan2008; Willard, Allen, Hardy, & Bonner, Reference Willard, Allen, Hardy and Bonner2017; Willard, Hardy, & Bonner, Reference Willard, Hardy and Bonner2009; Wolfe et al., Reference Wolfe, Walsh, Reynolds, Mitchell, Reddy, Paltin and Madan-Swain2013), and time-sensitive neural activation during a working memory task has been related to their psychosocial and behavioral/emotional outcomes as well (Robinson et al., Reference Robinson, Pearson, Cannistraci, Anderson, Kuttesch, Wymer and Compas2015). However, a behavioral measure specifically designed to capture a component process of emotion regulation, by evaluating attention and the control of attention to emotional stimuli, would constitute a novel approach to probe emotional functioning.
Eye-tracking is a noninvasive technique with proven utility for examining visual processing in normative and atypical development (Constantino et al., Reference Constantino, Kennon-McGill, Weichselbaum, Marrus, Haider, Glowinski and Jones2017; Dalton et al., Reference Dalton, Nacewicz, Johnstone, Schaefer, Gernsbacher, Goldsmith and Davidson2005; Karatekin, Reference Karatekin2007). Eye movements are ideally suited for detecting the implicit and explicit components of emotion regulation; automatic exogenous saccades that occur in response to stimuli without instructions (bottom-up) reflect implicit processes, whereas voluntary endogenous saccades that occur following instruction (top-down) reflect explicit processes (Mulckhuyse, Reference Mulckhuyse2018). Using eye-tracking, stimuli that initially capture attention (attentional orienting) can be distinguished from stimuli that maintain attention over time (attentional engagement), and the ability to voluntarily regulate these attentional processes can be investigated directly. Evaluating attention during an emotional response may help reveal if specific attentional components (e.g., orienting vs. engagement) are associated with poor outcomes. Given the prevalence of attentional decline in brain tumor survivors (Mabbott et al., Reference Mabbott, Spiegler, Greenberg, Rutka, Hyder and Bouffet2005), and that attention problems predict depression and difficulty with social relationships (Oh, Seo, Sung, & Joung, Reference Oh, Seo, Sung and Joung2017), it may be especially important to consider the attentional components of emotion regulation in this population.
White matter (WM) compromise has been well documented in groupwise analyses of patients treated for brain tumors (Liu et al., Reference Liu, Scantlebury, Tabori, Bouffet, Laughlin, Strother and Mabbott2015; Mabbott, Noseworthy, Bouffet, Rockel, & Laughlin, Reference Mabbott, Noseworthy, Bouffet, Rockel and Laughlin2006; Moxon-Emre et al., Reference Moxon-Emre, Bouffet, Taylor, Laperriere, Sharpe, Laughlin and Mabbott2016; Rueckriegel et al., Reference Rueckriegel, Driever, Blankenburg, Ludemann, Henze and Bruhn2010); however, studies that relate their WM organization to emotion regulation are limited. One study demonstrated that treatment and microstructure of the cerebello-thalamo-cerebral pathway together did not predict cognitive emotion regulation, in brain tumor survivors (Law et al., Reference Law, Smith, Greenberg, Bouffet, Taylor, Laughlin and Mabbott2017). Another study reported that microstructure of the inferior frontal occipital fasciculus (IFOF) and cingulum bundle explained the relationship between treatment for brain tumors and parent reports of behavioral regulation and internalizing symptoms in children (Wier et al., Reference Wier, Aleksonis, Pearson, Cannistraci, Anderson, Kuttesch and Hoskinson2019).
In the present study, we asked whether the regulation of attention to emotional stimuli and emotional control in daily life are disturbed in children treated for brain tumors. We developed and tested a novel eye-tracking measure to probe the attention-related component processes of emotion regulation; the experimental procedure is detailed in Figure 1. The overall objective was to better understand the attentional components of emotion regulation, and how they relate to emotional control and WM, in children treated for brain tumors.
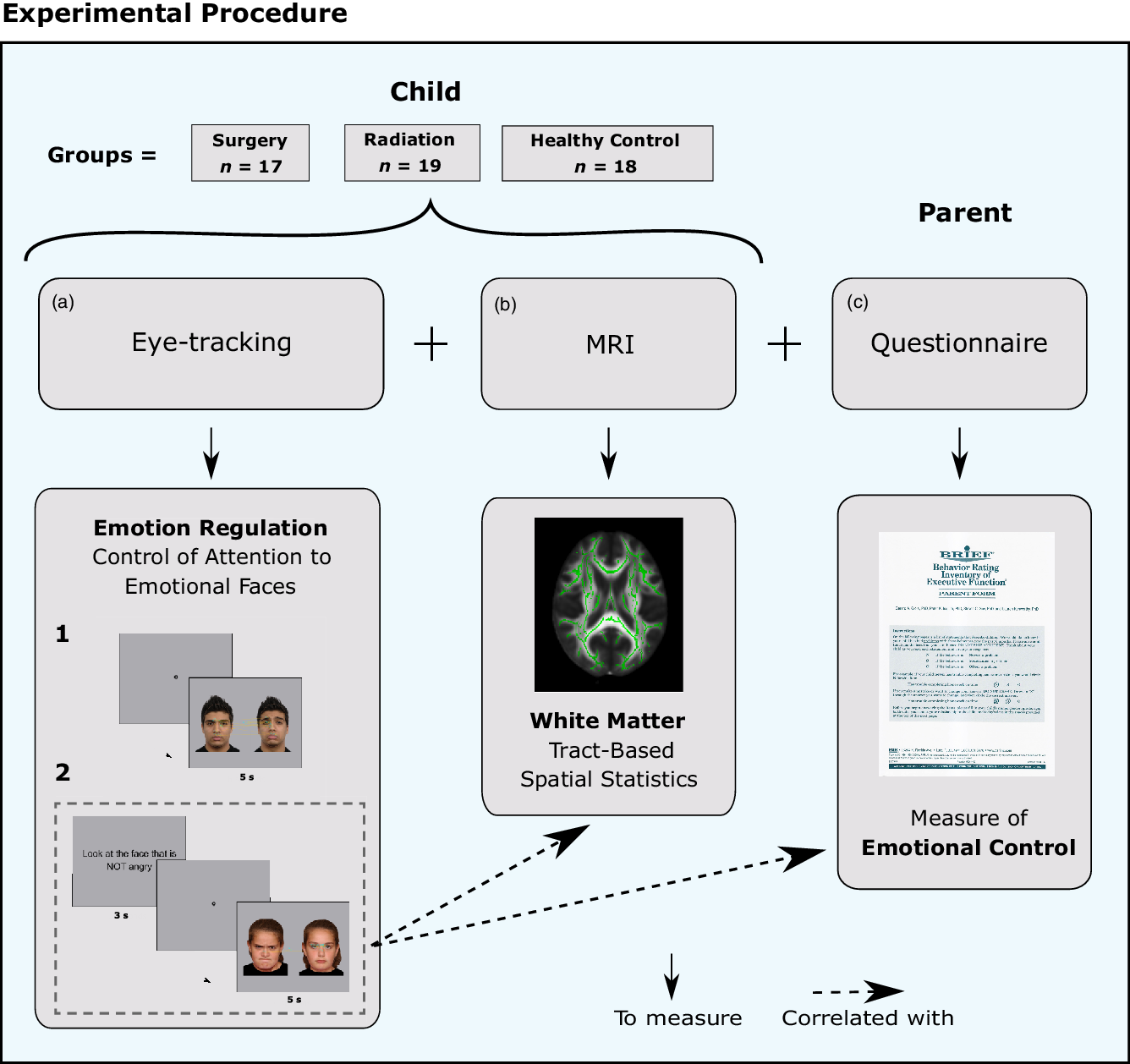
Fig. 1. Experimental procedure. (a) Eye-tracking paradigm designed to evaluate the attentional components of emotion regulation, (b) MRI to evaluate white matter (WM) microstructure. (c) Parental Behavior Rating Inventory of Executive Function (BRIEF) questionnaire; the Emotional Control scale was used to evaluate their child’s ability to modulate emotional responses in daily life.
METHOD
Participants
Fifty-four children (29 males/25 females) aged 8–17 years participated in this study; 36 children treated for PF tumors at the Hospital for Sick Children (SickKids; Toronto, Canada) and 18 typically developing children (healthy control group). Demographic variables are summarized in Table 1. All patients were >1 year post-diagnosis and had completed all therapy. Patients were excluded from participation if they had premorbid neurological disorders or if they were receiving palliative care. Healthy controls were free of all neurological or clinical disorders. Patients were recruited to the study via mailed letters and/or were approached during routine clinic visits. Healthy controls were either recruited from the community, were siblings of patients, or family members/friends of SickKids staff. All children were native English speakers or had completed at least 2 years of schooling in English at the time of participation. This study was approved by the SickKids’ Research Ethics Board. Prior to participation, parents provided written informed consent and children provided assent. Participants provided their own written consent when deemed capable to do so.
Table 1. Participant demographics and patient medical information

Note. CSI = cranial spinal irradiation. ANOVAs were used to assess differences between the three groups, and t-tests were used for the two group comparisons. Chi-square analyses were used for categorical variables with cell counts >5 and Fisher’s exact tests for cell counts <5. Effect sizes: η 2 p (small = 0.01, medium = 0.06, and large = 0.14), Cohen’s d (small = 0.2, medium = 0.5, and large = 0.8), and Cramer’s V (small = 0.1, medium = 0.3, and large = 0.5).
a Patients were classified as having mutism, or posterior fossa syndrome (PFS), if they had diminished speech output, linguistic difficulties, or dysarthria following surgery. Mutism is a transient dysfunction and had resolved in all participants by the time of assessment.
b One patient in the surgery group developed seizures following treatment. Ten of the healthy controls were siblings of brain tumor patients. This reflects an effort to include controls that were representative of the brain tumor population. Five of these controls were siblings of brain tumor patients that completed the present study and five were siblings of brain tumor patients that participated in other studies at the hospital (e.g., patients with tumors located outside the posterior fossa).
Patient Treatment Information
Of the 36 patients, 17 were treated with surgery with or without chemotherapy (surgery group), and 19 were treated with surgery and radiation, with or without chemotherapy (radiation group). In the radiation group, patients treated with photon beam CSI received either standard (3060–3600 cGy) or reduced (1800–2340 cGy) dose, and a boost to the tumor bed, whereas patients treated with focal radiation received 5400–5940 cGy to the tumor site. The patient groups did not differ on most medical variables (Table 1), except that the radiation group had more patients with hydrocephalus (p = .03). The only other differences arose from factors that determined their groupings; namely, patients in the radiation group were diagnosed with metastatic PF tumors, whereas patients in the surgery group were primarily diagnosed with benign PF tumors, or were diagnosed with medulloblastoma before three years of age and consequently did not receive radiation (p < .0001). Thus, the number of patients treated with chemotherapy, and the type of radiation delivered, differed between the patient groups (all p < .01).
Emotional Face Stimuli
Selection process: To include images of children and adults of multiple ethnicities, emotional faces from three databases were used. The Radboud Faces Database (RaFD) contains images of 39 Caucasian adults, 18 Moroccan males, and 10 Caucasian children, most of unknown age (Langner et al., Reference Langner, Dotsch, Bijlstra, Wigboldus, Hawk and van Knippenberg2010). The NimStim database contains 43 multiethnic adults (21–30 years) (Tottenham et al., Reference Tottenham, Tanaka, Leon, McCarry, Nurse, Hare and Nelson2009) and the National Institute of Mental Health Child Emotional Faces Picture Set (NIMH-chEF) database contains 59 multiethnic children (10–17 years) (Egger et al., Reference Egger, Pine, Nelson, Leibenluft, Ernst, Towbin and Angold2011).
Given that brain tumor patients have difficulty identifying facial emotions (Bonner et al., Reference Bonner, Hardy, Willard, Anthony, Hood and Gururangan2008; Moxon-Emre et al., Reference Moxon-Emre, Farb, Oyefiade, Bouffet, Laughlin, Skocic and Mabbott2019), only faces displaying clear emotions were utilized. All images displaying angry, sad, happy, and neutral expressions from each database were screened, and those displaying clear expressions were identified for potential inclusion. Facial expressions were paired by actor, and for each of the three mood pair categories (angry/neutral, happy/neutral, and sad/neutral) face pairs were rated by the study author (I.M.-E.) from 1 to 10 on: (1) clarity of emotional expression and (2) image quality. Ratings were added, then sorted from highest to lowest. The highest rated pairs from each actor face pair condition were selected for use in the eye-tracking task. Non-overlapping actor face pairs, containing an equal number of males, females, adults, and children, and the same number of faces from each database, were selected. This yielded 48 unique face pairs: 8 pairs in each of the 3 mood pair categories for both the task conditions (baseline and regulate, as detailed in the Eye-Tracking Task section). To validate the rating process, two additional individuals rated expression clarity (α = .72) and image quality (α = .92) for 36 face pairs selected at random. There were no significant disagreements in expression clarity between raters, and no faces were substituted as a result. However, some images received consistently low image quality scores, which prompted the implementation of a systematic image editing procedure.
Image editing: All images from the RaFD and NIMH-chEF databases were cropped just above shoulder level, to be consistent with the NimStim images. The background was set to gray for all images; this was done to maximize eye-tracking by limiting pupil constriction that occurs when viewing bright stimuli. Colored clothing worn by children in the NIMH-chEF database was edited to black, to minimize visual distraction. Edited images were then subject to face normalization in MATLAB to position the eyes, nose, and mouth in the same physical location. Examples of raw and edited images are provided in Figure 2(b).

Fig. 2. Eye-tracking paradigm to quantify emotional attentional capture. (a)–(b). Two versions of an eye-tracking task were designed to evaluate (a) attention to emotional versus neutral faces (baseline condition – free viewing) and the ability to regulate attention to the emotional faces (regulate condition – directed viewing). Examples of viewing patterns to the faces are shown. Blue circles = fixations, yellow lines = saccades. (b) i. Examples of original images from the three different emotional face databases used in the paradigm (left image = NIMH-chEF database; middle image = Radboud Faces Database; right image = NimStim database). ii. The same images edited for the paradigm. To maximize attention to the faces, all bright colored clothing was changed to black and facial features were aligned (black dotted lines). Only fixations made to the faces (i.e., inside the blue circles) were included in the analyses.
Eye-Tracking Apparatus
Eye movements were recorded throughout all tasks using a SR Research Ltd. Eyelink 1000 plus (Mississauga, Canada) eye-tracking desktop monocular system (sampling rate = 500 Hz; spatial resolution = .01°). The right eye was tracked in all except five participants, where poor calibration prompted a switch to tracking the left eye. A nine-point calibration was performed prior to the experiment and was successful in all participants. Images were displayed on a 15 × 12.5 inch LCD monitor with a 1280 × 1024 pixel resolution. Photographs displaying facial emotions were 506 × 650 pixels in size. Participants were seated 26 inches from the monitor and a chin rest was used to limit head movement. The experiment was built using the SR Research Ltd. Experiment Builder software.
Self and Parent Questionnaires
Emotion regulation was assessed with the Behavior Rating Inventory of Executive Function (BRIEF) (Gioia, Isquith, Retzlaff, & Espy, Reference Gioia, Isquith, Retzlaff and Espy2002). Depression and anxiety were assessed with the Children’s Depression Inventory 2 (CDI-2) (Kovacs, Reference Kovacs2011) and Screen for Child Anxiety-Related Emotional Disorders (SCARED) (Birmaher et al., Reference Birmaher, Brent, Chiappetta, Bridge, Monga and Baugher1999), respectively. Scale details are provided in Supplementary Doc 1.
Eye-Tracking Task
An overview of the experimental paradigm is provided in Figure 1. Two conditions of an eye-tracking task, detailed in Figure 2a, were designed:
-
1. Baseline (free viewing) condition: Images of angry/neutral, happy/neutral, and sad/neutral face pairs were presented side by side, and participants were instructed to look at the faces freely.
-
2. Regulate (directed viewing) condition: A second set of angry/neutral, happy/neutral, and sad/neutral face pairs were presented side by side, and participants were instructed to look at the nonemotional face only. The instructions (e.g., “Look at the face that is NOT angry”) were presented on the screen for 3 s and were also stated verbally by the examiner. A circle then appeared in the center of the screen, to which a fixation would trigger the face presentation; this was done to ensure that participants had an equal chance of making their first fixation to each face, and that timing of stimuli presentation was consistent across all participants.
Each face pair was presented for 5 s, again followed by a circle in the center of the screen. The positions of the faces in the display were counterbalanced according to emotion, sex, and age (i.e., child vs. adult). Each condition included 24 trials: 8 angry/neutral, 8 happy/neutral, and 8 sad/neutral.
Neuroimaging Protocol
Magnetic resonance imaging (MRI) was performed at SickKids using a Siemens 3T whole-body MRI scanner (Prisma Fit) with a 20-channel head and neck coil. Imaging included a T1 AX 3D MPRAGE Grappa 2 protocol (T = 900 ms, TE/TR = 3.83/2300 ms, 160 contiguous axial slices, flip angle = 9°, 256 × 224 matrix, FOV = 256 × 224 mm, voxel size=1 mm ISO) and diffusion-weighted single-shot spin-echo diffusion tensor imaging (DTI) sequence with EPI readout (30 directions, b = 1000 s/mm2, TE/TR = 90/9000 ms, 70 contiguous axial slices, flip angle = 90°, 122 × 122 matrix interpolated to 244 × 244, FOV = 244 × 244 mm, voxel size = 2 mm ISO, interpolated to 1 × 1 × 2 mm). Diffusion-weighted images were denoized, eddy corrected for current distortions, motion corrected and bias corrected to correct B1 field inhomogeneities, with the MRTrix3 package (www.mrtrix.org) that utilizes FSL’s eddy tool. DTI index maps [fractional anisotropy (FA) and radial diffusivity (RD)] were also created using MRTrix3. Six participants did not undergo MRI, either because they had braces (n = 3; 2 patients, 1 healthy control), had MRI-incompatible programmable shunts (n = 2; patients), or declined the MRI portion of the study (n = 1; patient).
Tract-Based Spatial Statistics (TBSS)
Voxelwise analyses were conducted with tract-based spatial statistics (TBSS) (Smith et al., Reference Smith, Jenkinson, Johansen-Berg, Rueckert, Nichols, Mackay and Behrens2006). All participants’ FA data were aligned into a common space (MNI152; Montreal Neurological Institute, McGill, Montreal, Canada) using the nonlinear registration tool FNIRT (Andersson, Jenkinson, & Smith, Reference Andersson, Jenkinson and Smith2007a, Reference Andersson, Jenkinson and Smith2007b). Then, a cross-subject mean FA image was created and used to generate a skeleton FA map representing the center common to all tracts, thresholded at FA > .20. Finally, participant-specific FA and RD maps were aligned with the skeleton, and values along the width of each tract were considered in the cross-subject voxelwise statistics.
Analytic Plan
Hypotheses 1 and 2
(1) Emotional faces will capture attention, in all groups; (2) children treated for brain tumors will have difficulty regulating this attentional capture and will continue to attend to the emotional face despite being instructed not to.
Eye movement analyses were performed with respect to interest areas placed around the faces (Figure 2(b); blue circles). To evaluate attentional orienting, we recorded (1) the amount of time taken to maintain visual gaze, or fixate, on either face (i.e., time to first fixation) and (2) the probability of the first fixation occurring on either face; these two eye-tracking metrics provided information about attentional capture by the faces. To evaluate attentional engagement, we recorded (3) the number of fixations and (4) total dwell time on each face, over the entire (5 s) trial.
Two multivariate analyses of variance (MANOVAs) were conducted: one for the baseline condition (Hypothesis 1) and another for the regulate condition (Hypothesis 2). We compared all four eye-tracking metrics to each face, between the healthy control and patient groups, as follows: 2 (facial emotion: emotional, neutral) × 3 (group: healthy control, radiation, surgery). We followed up with a series of univariate ANOVAs, one for each eye-tracking metric, in each condition. All analyses were corrected for multiple comparisons with Bonferroni correction.
Hypothesis 3
Children treated for brain tumors will have worse emotional control and will experience more symptoms of anxiety and depression than typically developing children.
T-scores from the BRIEF (Emotional Control scale) and CDI-2 (all scales) and raw scores from the SCARED (all scales) were compared between the healthy control, surgery, and radiation groups, using an ANOVA and two MANOVAs with follow-up univariate analyses, respectively. Chi-square analyses were conducted to assess if the proportion of children with mildly to clinically elevated BRIEF scores (T-score ≥60) differed between patients and healthy controls.
Hypothesis 4
Our eye-tracking measure of emotion regulation will relate to emotional control in daily life.
For the regulate (directed viewing) condition, an emotion regulation difference score was calculated for each trial by subtracting the time to first fixation on the target face (i.e., the neutral face) from the time to first fixation on the nontarget emotional face (i.e., angry, happy, or sad face). Thus, values below zero indicate a better ability to override the attentional capture of emotional faces. This emotion regulation score was utilized to account for individual differences in fixation speed and to obtain a single value to correlate with the emotional control score from the BRIEF, and with WM microstructure throughout the brain (detailed below).
We evaluated the correlation between our eye-tracking emotion regulation score and the emotional control score from the BRIEF, across all children, using a Pearson correlation.
Exploratory aim
We took an unbiased voxelwise approach to evaluate the association between WM and our eye-tracking measure of emotion regulation.
Using TBSS, we assessed if RD and FA in any voxels throughout the generated WM map correlated with our eye-tracking emotion regulation score, in healthy control and patient groups considered separately. All patients were considered together because we recently demonstrated that children treated with radiation and surgery in the current cohort did not differ in WM microstructure (Moxon-Emre et al., Reference Moxon-Emre, Farb, Oyefiade, Bouffet, Laughlin, Skocic and Mabbott2019). Age was included as a covariate. TBSS controls for family-wise errors using a permutation methodology; the null distribution of the cluster size statistic was built up over 5000 random permutations. Cluster size was thresholded at p < .05, which was corrected for multiple comparisons. A mask was made for each significant cluster of >100 voxels, and the anatomic extent of each mask was labeled with reference to the JHU white-matter tractography atlas and the ICBM-DTI-81 white-matter labels atlas (Hua et al., Reference Hua, Zhang, Wakana, Jiang, Li, Reich and Mori2008).
RESULTS
Emotional Faces Capture Attention
First, we verified that emotional faces captured attention in the baseline condition of the eye-tracking task. Our omnibus MANOVA revealed a significant main effect of facial emotion (F (4,48) = 8.97, p < .001, η 2 p = .43; Table 2), a significant main effect of group (F (8,98) = 3.52, p = .001, η 2 p = .22; Table 2), and a nonsignificant interaction (F (8,98) = 1.12, p = .36, η 2 p = .08; Table 2). Univariate analyses for each eye-tracking metric are detailed in turn:
Table 2. Eye-tracking metrics in the baseline and regulate conditions. Results from the multivariate analyses of variance (MANOVAs) that included all eye-tracking metrics and follow-up univariate ANOVAs for each eye-tracking metric, for the baseline and regulate conditions. Measures of attentional orienting include time to first fixation and probability of first fixation. Measures of attentional engagement over the entire trial (i.e., 5 s) include number of fixations and total dwell time

Note. Facial emotion = emotional face, neutral face; Groups = healthy control, surgery, radiation.
Attentional orienting: Emotional faces captured attention in all groups; there was a main effect of facial emotion for the time to first fixation, with the first fixation being made earlier to the emotional face (F (1,51) = 32.18, p < .001, η 2 p = .39; Figure 3a; Table 2). In each group, children were faster to make their first fixation on the emotional face compared to the neutral face (all F > 4.16, all p < .05, all η 2 p > .08). However, children in the radiation group were slower to make their first fixation, to either face; a main effect of group (F (2,51) = 4.0, p = .02, η 2 p = .14; Table 2) indicated that compared to healthy controls, patients treated with radiation had a longer time to first fixation [p = .02, (95% CI:26.01, 401.76)], whereas patients treated with surgery did not differ from either the radiation [p = .77, (95% CI: −279.39, 101.99)] or healthy control [p = .35, (95% CI: −68.00, 318.35)] groups. Overall, all children were also more likely to make their first fixation on the emotional face; there was a main effect of facial emotion for the probability of first fixation (F (1,51) = 13.34, p = .001, η 2 p = .21; Table 2); however, because post hoc pairwise comparisons revealed that patients in the surgery group did not exhibit this effect (F (1,51) = 1.27, p = .27, η 2 p = .03), the more consistent metric, time to first fixation, was chosen as our baseline measure of attentional orienting for cross-task comparisons and correlations.

Fig. 3. Children treated for brain tumors have difficulty regulating emotional attentional capture. (a)–(b). Boxplots showing all data points with the mean (white diamond) and median (black line) for the time to first fixation to the emotional face versus neutral face in the: (a) baseline condition: a shorter time to first fixation, to the emotional face, indicates that across all three groups, the emotional face captures attention and (b) regulate condition: a significant emotional face-by-group interaction (p = .006) revealed a shorter time to first fixation, to the neutral face in the healthy control group, namely, only the healthy controls were able to override (or regulate) the attentional capture of the emotional face. *p < .05; Bonferroni-corrected pairwise comparison between the time to first fixation, to the faces, within each group. See Supplementary Figures 1 and 2 for viewing patterns during each emotional face trial type (i.e., happy/neutral, angry/neutral, and sad/neutral) that together constituted the emotional/neutral condition.
Attentional engagement: Although the measures of attentional orienting were utilized to characterize attentional capture, it is noteworthy that over the full trial (i.e., 5 s) greater attention was directed to the emotional face; there was a main effect of facial emotion and group, for both the number of fixations made, and the total dwell time (detailed in Table 2).
Attentional orienting and engagement: No facial emotion by group interaction, for any eye-tracking metric, was detected in the baseline condition (Table 2). For completion, viewing patterns during each mood pair category trial type, that together constituted the emotional/neutral condition, are provided in Supplementary Figures 1 and 2.
Children Treated for Brain Tumors have Difficulty Regulating the Attentional Capture of Emotional Faces
In the second phase of the eye-tracking task, participants were instructed to avoid looking at the emotional face. Our omnibus MANOVA revealed a significant main effect of facial emotion (F (4,48) = 194.17, p < .001, η 2 p = .94; Table 2), a significant main effect of group (F (8,98) = 2.24, p = .03, η 2 p = .15; Table 2), and a nonsignificant interaction (F (8,98) = 1.51, p = .16, η 2 p = .11; Table 2). Univariate analyses for each eye-tracking metric are detailed in turn:
Attentional orienting: Only typically developing children were able to override the attentional capture of emotional faces when instructed to; a significant facial emotion by group interaction (F (2,51) = 5.58, p = .006, η 2 p = .18; Figure 3(b); Table 2) revealed that only the healthy control group had a shorter time to first fixation to the neutral face than to the emotional face (F (1,51) = 13.04, p = .0007, η 2 p = .20). Time to first fixation did not differ between the emotional and neutral faces in the surgery (F (1,51) = .67, p = .41, η 2 p = .01) and radiation (F (1,51) = 1.11, p = .30, η 2 p = .02) groups, indicating that patients were unable to override the attentional capture of emotional faces by orienting more quickly to the neutral face (Figure 3(b)).
To determine if group differences in the ability to override attentional capture occurs regardless of baseline viewing, we conducted a follow-up one-way ANOVA; we assessed group differences in the time to first fixation in the regulate condition, while controlling for the time to first fixation at baseline. A significant group difference (F (2,50) = 4.15, p = .02, η 2 p = .14) revealed that patients treated with radiation differed from healthy controls [(controls: mean = −192.23; SE = 57.19; radiation: mean = 40.68; SE = 56.87), p = .02, (95% CI:28.52, 437.49)]; thus, the inability for patients treated with radiation to override the attentional capture of emotional faces is independent from their natural viewing tendencies.
Attentional engagement: Viewing patterns throughout the entire trial duration of the regulate condition revealed that children in all groups were capable of successfully completing the emotion regulation task; there was a main effect of facial emotion for both the number of fixations and dwell time (all F (1,51) > 390.00, all p < .001, all η 2 p > .88; Table 2). Across all groups, children made more fixations and had longer dwell times, on the neutral face compared to the emotional face (all p < .05; Supplementary Figure 2).
Attentional orienting and engagement: Viewing patterns during each mood pair category trial type, that together constituted the emotional/neutral condition, are provided in Supplementary Figures 1 and 2.
Daily Emotional Control is Mildly Impaired in Pediatric Brain Tumor Patients
Patients exhibited worse emotional control than healthy controls (F (1,47) = 4.63, p = .04, η 2 p = .09), yet there were no differences between the three groups (F (2,46) = 2.27, p = .11, η 2 p = .09; Figure 4a; Table 3). Notably, the mean scores for all three groups were within the normal range, suggesting that patients in our sample are not experiencing clinically significant emotional control difficulties. However, patients had more variable scores than healthy controls; 19.36% of patients had mildly to clinically elevated scores, whereas all controls were within the normal range (χ2 (1, n = 49) =3.97, p = .046, Cramer’s V = .29).

Fig. 4. Poorer performance on the directed viewing task is correlated with worse emotional control in daily life. (a) Emotional control scores from the Behavior Rating Inventory of Executive Function (BRIEF) scale. (b) The emotion regulation score [calculated by subtracting the time to first fixation to the target (i.e., neutral) from the nontarget (i.e., emotional) face, during the directed viewing task] correlated with the Emotional Control scale from the BRIEF. Higher emotion regulation and BRIEF scores both indicate worse functioning in daily life. Thus, children who have difficulty overriding the attentional capture of emotional faces during the earliest components of their visual response displayed poorer emotional control in daily life.
Table 3. Group differences in parent-report emotional control and self-report depression and anxiety

Matching letters in different rows indicate a significant difference (p < .05) between groups as follows: a p = .02. b p = .049. c p = .02. Note that higher values for CDI-2 measures indicate more problems.
BRIEF = Behavior Rating Inventory of Executive Function; CDI-2 = The Children’s Depression Inventory 2; SCARED = Screen for Child Anxiety-Related Emotional Disorders.
* The BRIEF was missing from five participants whose parents did not return the completed questionnaire. All missing BRIEFs were from the radiation group, thus n = 15 for the Emotional Control scale. η 2 p values: small = .01, medium = .06, and large = .14 effect sizes.
The patient groups did not experience heightened levels of anxiety (SCARED total score: F (1,47) = .59, p = .56, η 2 p = .02) or depression (CDI-2 total score: F (1,47) = 2.20, p = .12, η 2 p = .08) compared to the healthy control group (Table 3).
Early Oculomotor Response in Emotion Regulation is Associated with Behavior in Daily Life
Across all children, our eye-tracking emotion regulation score was positively correlated with the emotional control score on the BRIEF (r = .29, p = .045; Figure 4(b)), indicating that children who had difficultly overriding the attentional capture of emotional faces displayed the worst emotional control in daily life.
Greater microstructural organization of WM in the body and splenium of the corpus callosum is associated with difficulty regulating the attentional capture of emotional faces, in brain tumor patients.
In children treated for brain tumors, we identified a cluster of 308 voxels where RD was negatively correlated with the eye-tracking emotion regulation score (higher score = worse regulation) (t = 4.36, p = .037; Figure 5a), and a cluster of 1585 voxels, where FA was positively correlated with our eye-tracking emotion regulation score (t = 3.04, p = .03; Figure 5(b)), located mostly in the body and splenium of the corpus callosum. In healthy controls, there were no voxels where RD or FA correlated with the eye-tracking emotion regulation score (all p > .05).

Fig. 5. Greater organization of white matter microstructure in the body and splenium of the corpus callosum is associated with worse performance on the directed viewing task in brain tumor patients. (a)–(b). Controlling for age, voxels where (a) RD (red-yellow) was negatively correlated and (b) FA (dark blue-light blue) was positively correlated, with the eye-tracking emotion regulation score (higher scores = worse regulation). In children treated for brain tumors, those who had more organized WM microstructure in the body and splenium of the corpus callosum were less able to voluntarily override the attentional capture of emotional faces. No correlations were observed in healthy controls. Clusters of significant voxels are superimposed on the FMRIB FA template and brain regions were labeled with the ICBM-DTI-81 white-matter labels atlas. Images are shown in radiological convention. Numbers represent MNI z-coordinates. L = left; R = right.
DISCUSSION
We developed and implemented a novel eye-tracking measure to assess the attentional components of emotion regulation and evaluated relations between performance on this measure with emotional functioning and WM structure, in children treated for brain tumors. We began by demonstrating that all children experienced attentional capture by the emotional faces. Next, we demonstrated that children treated for brain tumors had difficultly regulating attentional capture, and that this early component process of emotion regulation was related to emotional behavior in daily life across all children. Finally, we identified WM in the body and splenium of the corpus callosum as a potential neuroanatomical substrate of our eye-tracking measure of emotion regulation, in children treated for brain tumors.
Previous eye-tracking studies have demonstrated that emotional stimuli capture attention more effectively than neutral stimuli (Nummenmaa, Hyona, & Calvo, Reference Nummenmaa, Hyona and Calvo2006). Accordingly, in our baseline condition, emotional faces capture automatic (i.e., exogenous) attention in typically developing children and in children treated for brain tumors. That children treated for brain tumors also exhibited automatic attentional capture suggests their bottom-up processing of emotional information is preserved. Once we determined that emotional faces captured attention, we assessed the attentional components of emotion regulation by evaluating participants’ ability to deliberately (i.e., endogenously) override this response in a top-down manner. We demonstrated that typically developing children were able to override the attentional capture of emotional faces, whereas children treated for brain tumors were not. The inability to override the attentional capture of emotional faces suggests top-down processing of emotional information may be compromised in children treated for brain tumors.
Some studies have suggested that healthy children and adults are unable to override attentional capture when instructed (Lagattuta & Kramer, Reference Lagattuta and Kramer2017; Nummenmaa et al., Reference Nummenmaa, Hyona and Calvo2006); in contrast, we demonstrated that typically developing children in our sample were capable of doing so. Some notable study design differences may explain this discrepancy. In Nummenmaa and colleagues (Reference Nummenmaa, Hyona and Calvo2006), the stimuli were scenes and not faces. Using two facial expressions from the same actor, we reduced the potential for differences in visual content from driving attentional orientation. Although Lagattauta and Kramer (Reference Lagattuta and Kramer2017) utilized pictures of faces, they paired two emotional faces together, whereas we presented an emotional face with a neutral face. That typically developing children in our study were able to regulate their initial orienting away from the emotional face suggests our task design contained the sensitivity to detect subtle differences in the regulation of attention to emotional stimuli.
We also found that the ability to override the attentional capture of emotional faces was modestly associated with parent-report emotional control across all children. This finding is encouraging as it suggests our novel eye-tracking task measured a process related to emotional behavior in daily life. It also raises the possibility that investigations into the attentional components of emotion regulation may yield valuable insights into our understanding of emotional behavior.
Children treated for brain tumors experience considerable WM damage (Liu et al., Reference Liu, Scantlebury, Tabori, Bouffet, Laughlin, Strother and Mabbott2015; Mabbott et al., Reference Mabbott, Noseworthy, Bouffet, Rockel and Laughlin2006; Moxon-Emre et al., Reference Moxon-Emre, Bouffet, Taylor, Laperriere, Sharpe, Laughlin and Mabbott2016; Moxon-Emre et al., Reference Moxon-Emre, Farb, Oyefiade, Bouffet, Laughlin, Skocic and Mabbott2019), including to the corpus callosum (Palmer et al., Reference Palmer, Glass, Li, Ogg, Qaddoumi, Armstrong and Reddick2012). It is notable that microstructural organization of WM in the body and splenium of the corpus callosum was associated with our eye-tracking measure of emotion regulation in children treated for brain tumors only. The splenium facilitates interhemispheric communication between the visual cortices (Putnam, Steven, Doron, Riggall, & Gazzaniga, Reference Putnam, Steven, Doron, Riggall and Gazzaniga2010) and has been associated with visual processing (Davis & Cabeza, Reference Davis and Cabeza2015; Schulte, Muller-Oehring, Rohlfing, Pfefferbaum, & Sullivan, Reference Schulte, Muller-Oehring, Rohlfing, Pfefferbaum and Sullivan2010). Moreover, networks of top-down attention control have been shown to modulate activity in the visual cortex (Hopfinger, Buonocore, & Mangun, Reference Hopfinger, Buonocore and Mangun2000). Altered WM within the splenium has also been identified as a shared feature between children with neurodevelopmental disorders that are characterized in part by attentional challenges: autism spectrum disorder, attention deficit hyperactivity disorder, and obsessive compulsive disorder (Ameis et al., Reference Ameis, Lerch, Taylor, Lee, Viviano, Pipitone and Anagnostou2016).
Although reduced microstructural organization has typically been associated with functional impairments in children treated for brain tumors (Mabbott et al., Reference Mabbott, Noseworthy, Bouffet, Rockel and Laughlin2006; Palmer et al., Reference Palmer, Glass, Li, Ogg, Qaddoumi, Armstrong and Reddick2012; Rueckriegel, Bruhn, Thomale, & Hernaiz Driever, Reference Rueckriegel, Bruhn, Thomale and Hernaiz Driever2015), we demonstrate the opposite: greater microstructural organization of WM of the body and splenium of the corpus callosum (i.e., higher FA/lower RD), typically considered “healthier”, was associated with worse emotion regulation in children treated for brain tumors. Others have also reported that higher FA in the corpus callosum is associated with worse performance on cognitive control tasks; higher FA in the splenium was associated with worse task-switching ability in adolescents (Seghete, Herting, & Nagel, Reference Seghete, Herting and Nagel2013) and higher FA in the frontal projections of the corpus callosum was associated with worse inhibitory control in children (Treit, Chen, Rasmussen, & Beaulieu, Reference Treit, Chen, Rasmussen and Beaulieu2014). Broadly, the corpus callosum is thought to contribute to the integration of perception and actions and to affect attentional processing by facilitating interactions between hemispheres (Schulte & Muller-Oehring, Reference Schulte and Muller-Oehring2010). It is possible that in children treated for brain tumors, within the context of widespread WM compromise, higher FA and lower RD in the body and splenium of the corpus callosum are not functionally advantageous. Although speculative, greater WM organization in this context may contribute to more effective sustained attention that comes at a time cost for disengaging attention; if true, it could explain why no comparable association was observed between WM and our eye-tracking task in healthy controls.
This study was limited by small, albeit relatively equal, sample sizes within each of the three (healthy control, surgery and radiation) groups; when patients were considered together however, the healthy control sample size was comparatively smaller, which may have contributed to the absence of an association between WM and our eye-tracking task in this group. The present study was also limited by reliance on the parent-report BRIEF to evaluate emotional control in daily life; future studies could include assessment with the Difficulties in Emotion Regulation Scale (Neumann, van Lier, Gratz, & Koot, Reference Neumann, van Lier, Gratz and Koot2010), a measure developed to assess processes that contribute to emotion regulation problems, or with measures designed to assess cognitive emotion regulation strategies, such as the Emotion Regulation Questionnaire for Children and Adolescents (Gullone & Taffe, Reference Gullone and Taffe2012).
Despite these limitations, findings from this study raise interesting theoretical questions regarding the intersection of attention and emotion in children treated for brain tumors that may warrant further investigation; for instance, would improving the ability to override attentional capture by emotional faces transfer to improvements in emotional control? Interestingly, evidence suggests that active transcranial direct current stimulation (tDCS) over the left dorsolateral prefrontal cortex (DLPFC) results in faster disengagement from emotional faces in healthy individuals (Sanchez-Lopez, Vanderhasselt, Allaert, Baeken, & De Raedt, Reference Sanchez-Lopez, Vanderhasselt, Allaert, Baeken and De Raedt2018). As such, tDCS over the left DLPFC may represent a future area for investigation in patients.
We are the first to combine an objective eye-tracking measure with a standardized measure of emotional control to characterize the processes that contribute to emotional functioning in children treated for brain tumors, and our findings provide mechanistic clues into the processes that contribute to emotional functioning in children treated for brain tumors.
Acknowledgments
The authors would also like to thank all the children and families who participated in this study.
Funding
The authors acknowledge research support from the Canadian Institutes of Health Research (D.J.M., grant number MOP-123537); and the Pediatric Oncology Group of Ontario (I.M.-E., doctoral research fellowship).
Conflicts of Interest
The authors have nothing to disclose.
Supplementary material
To view supplementary material for this article, please visit https://doi.org/10.1017/S1355617720000491