An archetypal example of the influence of parenting style on a child's development is how the Spartans in ancient Greece created the most fierce and brave soldiers that ever existed by using extremely harsh parenting practices. Although the historical example of Spartan childrearing is anecdotal (Kennell, Reference Kennell, Bell, Grubbs and Parkin2013), empirical research in the last decades has confirmed the influence of caregiving styles on children's cognitive and social-emotional development (Gilbert et al., Reference Gilbert, Widom, Browne, Fergusson, Webb and Janson2009; Keyes et al., Reference Keyes, Eaton, Krueger, McLaughlin, Wall, Grant and Hasin2012; Morris, Cui, & Steinberg, Reference Morris, Cui, Steinberg, Larzelere, Morris and Harrist2013; Pechtel & Pizzagalli, Reference Pechtel and Pizzagalli2011). Children who experienced insensitive or harsh parenting practices, such as physical and emotional neglect or abuse, show impaired cognitive performance (Pechtel & Pizzagalli, Reference Pechtel and Pizzagalli2011), altered reward processing, stress reactivity, and impulsiveness (Andersen & Teicher, Reference Andersen and Teicher2009; Lovallo, Reference Lovallo2013). Growing up in unstable and emotionally cold families may result in deficits in emotional understanding, stronger emotional reactivity, and less effective emotion regulation and coping with stressful situations (Repetti, Taylor, & Seeman, Reference Repetti, Taylor and Seeman2002). During puberty and adolescence, these social–emotional problems can translate into risky sexual behavior, increased aggression (Repetti et al., Reference Repetti, Taylor and Seeman2002; Veenema, Reference Veenema2009), and substance abuse and suicidality (Andersen & Teicher, Reference Andersen and Teicher2009). Furthermore, experienced harsh caregiving constitutes a major risk factor for the development of psychopathology later in life such as anxiety and mood disorders (Heim & Nemeroff, Reference Heim and Nemeroff2001), schizophrenia (Morgan & Fisher, Reference Morgan and Fisher2007), borderline personality disorder (BPD; Ball & Links, Reference Ball and Links2009), and posttraumatic stress disorder (PTSD; Berntsen et al., Reference Berntsen, Johannessen, Thomsen, Bertelsen, Hoyle and Rubin2012).
In contrast, parenting styles characterized by high levels of parental warmth result in improved emotion regulation, self-esteem, and social–emotional functioning (Morris et al., Reference Morris, Cui, Steinberg, Larzelere, Morris and Harrist2013). Although not all children are equally sensitive to variation in caregiving practices (Ellis, Boyce, Belsky, Bakermans-Kranenburg, & van IJzendoorn, Reference Ellis, Boyce, Belsky, Bakermans-Kranenburg and van IJzendoorn2011), and genetic differences might play a role, these findings clearly demonstrate the importance of sensitive caregiving for the outcome of a child's social–emotional development. It is of critical importance that disturbances in children's social–emotional behavior due to insensitive and harsh parenting can also result in reduced parental responsivity later in life (Lanius, Vermetten, & Pain, Reference Lanius, Vermetten and Pain2010; Meaney, Reference Meaney2001) and increase the risk for developing a similar parenting style toward one's own children (Milner, Reference Milner2003). As such, a cyclic pattern may be established wherein insensitive and harsh parenting is transmitted over generations (Bailey, Hill, Oesterle, & Hawkins, Reference Bailey, Hill, Oesterle and Hawkins2009). Recent empirical work suggests that especially neglect and sexual abuse are subject to such intergenerational transmission (Widom, Czaja, & DuMont, Reference Widom, Czaja and DuMont2015). Yet already at an early stage in life the results of intergenerational transmission can be observed; attachment security in early infancy predicts attachment security later in life and, subsequently, attachment security in the next generation (Belsky, Reference Belsky, Carter, Ahnert, Grossmann, Hrdy, Lamb, Porges and Sachser2005; van IJzendoorn, Reference van IJzendoorn1995).
The long-term intergenerational effects of variation in parenting on social–emotional development, and the risk factor it constitutes for psychopathology, stress the importance of the parental qualities of human caregivers. Compared to other mammals, human infants are extremely demanding. To thrive as adults, infants not only require food, warmth, and protection like all other mammalian species (Farmer, Reference Farmer2000), but also need to learn the use of language, social rules, emotion regulation, and cognitive empathic abilities (De Haan & Gunnar, Reference De Haan and Gunnar2009; Heyes & Frith, Reference Heyes and Frith2014). In addition, human infants develop slowly, partly due to the metabolic demands of a protracted brain development to facilitate these cognitive and social capacities (Kuzawa et al., Reference Kuzawa, Chugani, Grossman, Lipovich, Muzik, Hof and Lange2014). The development of a child's cognitive and social–emotional abilities thus depends on intensive and long-term investment by caregivers. In evolutionary terms, this dependency most likely evolved in the context of group living, where paternal care and alloparenting both contributed to infant survival (Gray & Anderson, Reference Gray and Anderson2010; Hrdy, Reference Hrdy2009). Multiple caregivers facilitated the possibility of a protracted development by shared care, and because of shared care the risk of exposure to inadequate parenting spread. As such, shared care and social control in early human group living might have served as protective factors for childhood abuse and neglect (Gray & Anderson, Reference Gray and Anderson2010; Hrdy, Reference Hrdy, Carter, Ahnert, Grossmann, Hrdy, Lamb, Porges and Sachser2005). In current Western society, childrearing practices have radically changed. Group living has shifted to living in nuclear families, with shared care being mostly replaced by nuclear family care and also by institutional out of home care. This has made children increasingly dependent on the parental qualities of their primary caregivers, who might easily feel overburdened by the caregiving workload. This might be one of the reasons for the high prevalence rates of child maltreatment and neglect in Western countries, where conservative prevalence estimates are around 10% (Gilbert et al., Reference Gilbert, Widom, Browne, Fergusson, Webb and Janson2009; Lanius et al., Reference Lanius, Vermetten and Pain2010; Stoltenborgh, Bakermans-Kranenburg, & van IJzendoorn, Reference Bakermans-Kranenburg and van IJzendoorn2013). Considering the wide ranging effects of variation in parenting style on a child's developmental outcome as described above, such high prevalence rates pose a strong challenge to society. For this reason, and in order to provide avenues for intervention, understanding the underlying neurobiological mechanisms of variation in caregiving and the intergenerational transmission of caregiving is crucial (Anda, Butchart, Felitti, & Brown, Reference Anda, Butchart, Felitti and Brown2010; Shonkoff, Reference Shonkoff2012). Research on the intergenerational transmission of human caregiving has so far mostly focused on the role of observational learning and socialization (Bretherton & Munholland, Reference Bretherton, Munholland, Cassidy and Shaver2008; Milner, Reference Milner2003), but with increasing consilience of social and biological sciences there is a growing interest in the biological mechanisms that underlie intergenerational transmission.
In the current review I describe the recent literature investigating a critical biological factor underlying variation in caregiving: variability in endocrine systems. Bearing on decades of animal studies, the role of hormones and neuropeptides in human caregiving behavior is becoming increasingly acknowledged (Rilling, Reference Rilling2013; Rilling & Young, Reference Rilling and Young2014; Swain et al., Reference Swain, Kim, Spicer, Ho, Dayton, Elmadih and Abel2014; van Anders, Goldey, & Kuo, Reference van Anders, Goldey and Kuo2011). Especially the role of the neuropeptide oxytocin (OXT) in social bonding has received much attention (e.g., Bartz, Zaki, Bolger, & Ochsner, Reference Bartz, Zaki, Bolger and Ochsner2011; Galbally, Lewis, van IJzendoorn, & Permezel, Reference Galbally, Lewis, van IJzendoorn and Permezel2011; Riem, van IJzendoorn, Tops, et al., Reference Riem, van IJzendoorn, Tops, Boksem, Rombouts and Bakermans-Kranenburg2011; Ross & Young, Reference Ross and Young2009). However, an overview of the literature also shows that there is large variability in the effects of hormones and neuropeptides on behavior, and that effects are strongly dependent on environmental context and personal factors (Bakermans-Kranenburg & van IJzendoorn, Reference Bakermans-Kranenburg and van IJzendoorn2013; Bartz, Zaki, et al., Reference Bartz, Zaki, Bolger and Ochsner2011; Bos, Panksepp, Bluthe, & Honk, Reference Bos, Panksepp, Bluthe and Honk2012). This variability is of special interest, as not only basal levels of hormones and neuropeptides but also variation in sensitivity to these hormones and neuropeptides will add to the variability in the behavioral repertoire of caregiving. For example, equal basal levels of OXT in two individuals can affect their behavior differently depending on these individuals’ genetic makeup and early life experiences.
First, a formal introduction of these hormonal factors, their underling physiological effects, and their relevance to caregiving behavior as based on animal studies. Second, the role of the most important steroid hormones and neuropeptides in human caregiving are described. Third, an overview is provided of all the factors that throughout development contribute to variability in neural and behavioral sensitivity to the hormones and neuropeptides relevant for human caregiving. Fourth, based on the overview in the preceding sections, endocrine profiles are proposed leading to sensitive and insensitive caregiving practices. Fifth, the final section describes how the influence of experienced caregiving in childhood and adolescence can shape the endocrine profiles predictive of (in)sensitive caregiving, and as such provides a model for the intergenerational transmission of human caregiving, with a critical underlying role for endocrine sensitivity.
Hormonal Factors, Physiology, and Their Relevance to Caregiving Behavior
OXT and vasopressin (AVP)
OXT and AVP are two evolutionarily closely related peptides that derived from a single peptide more than 400 million years ago (Acher, Reference Acher1980). Both are synthesized in the supraoptic and paraventricular nucleus of the hypothalamus, from where they affect the central nervous system following different pathways. Peripheral targets are reached by release in the bloodstream via the pituitary, and OXT and AVP are projected to diverse regions in the brain via axonal transport. Local extracellular diffusion and transport by the cerebrospinal fluid are functionally relevant pathways (Landgraf & Neumann, Reference Landgraf and Neumann2004; Veening, de Jong, & Barendregt, Reference Veening, de Jong and Barendregt2010). In mammalian species, OXT is of critical importance for reproductive function, such as the onset of uterine contractions during labor and for milk letdown during suckling. It exerts its effects by acting on a single receptor (OXTR). AVP is known as an antidiuretic hormone and acts to retain water and increases vasoconstriction. It acts on a few receptors of which the AVP receptor 1a (AVPR1a) has shown most relevance to mammalian social behavior. Studies in several mammalian species have shown that OXT release during parturition is critical in the formation of the mother–infant bond (Neumann, Reference Neumann2009), and that OXT and AVP stimulate mutual recognition and maternal bonding after parturition (Curley & Keverne, Reference Curley and Keverne2005). AVP is also important in the regulation of maternal and paternal behavior after parturition, and both OXT and AVP have been implicated in maternal aggression (Bosch & Neumann, Reference Bosch and Neumann2012; Wang, Ferris, & De Vries, Reference Wang, Ferris and De Vries1994). Besides parental care behavior, receptor distributions of OXT and AVP have shown to be critical for monogamous pair bonding in voles, with higher densities of OXTR in the ventral striatum in female monogamous voles and higher densities of AVPR1a in the lateral septum in monogamous males (Insel & Young, Reference Insel and Young2001). These findings suggest a role for these neuropeptides in adult pair bonding and also show the sexually dimorphic effect these neuropeptides have on mammalian social behavior. Besides these effects of OXT and AVP on social–emotional behavior, both peptides are implicated in the endocrine responses to stress. Acute stressors in both sexes lead to pulses of OXT and AVP release, where in the short term OXT might increase arousal and activation of the sympathetic nervous system (Carter, Reference Carter2014), whereas AVP increases goal-directed behavior and anxiety (Engelmann, Landgraf, & Wotjak, Reference Engelmann, Landgraf and Wotjak2004), both facilitating adaptive responses to threat and challenge. Afterward OXT can have downregulating effects on endocrine stress responses by reducing anxiety and reinstating homeostasis (Carter, Reference Carter2014).
Estradiol
Estradiol acts on the estrogen receptor (ER), and its primary function is to act in concert with progesterone (PROG) to facilitate pregnancy. In several mammalian species, estradiol is also critical for the formation of maternal bonding after parturition by priming the maternal brain for synthesis of OXT and OXT receptors (Nelson, Reference Nelson2005). In primates, estradiol levels during pregnancy have been shown to predict maternal caregiving behavior after parturition, although the relation of estradiol levels after pregnancy with maternal caregiving are less clear (Saltzman & Maestripieri, Reference Saltzman and Maestripieri2011). Although the role of estrogens in paternal behavior are unknown, preliminary data from primates have further shown increased estrogen levels in fathers exposed to infant odors (Ziegler, Peterson, Sosa, & Barbard, Reference Ziegler, Peterson, Sosa and Barnard2011).
Testosterone/hypothalamus–pituitary–gonadal (HPG) axis
Testosterone acts on the androgen receptor (AR). It is the end product of the HPG axis and is critical for mammalian reproductive, sexual, and aggressive behavior. Testosterone is easily converted into estradiol and is functionally relevant in both males and females (Bos, Panksepp, et al., Reference Bos, Panksepp, Bluthe and Honk2012). With regard to mammalian caregiving behavior, studies on maternal behavior are scarce, but testosterone levels are generally negatively associated with paternal behavior. In primates, high caring fathers generally have lower levels of testosterone and show decreases in testosterone levels when interacting with their infants (Storey & Ziegler, Reference Storey and Ziegler2016). Nonetheless, in rodents testosterone has been related to maternal behavior (González-Mariscal, McNitt, & Lukefahr, Reference González-Mariscal, McNitt and Lukefahr2007) and indirectly might contribute to paternal behavior by conversion to estradiol (Trainor & Marler, Reference Trainor and Marler2002).
Cortisol (CORT)/hypothalamus–pituitary–adrenal (HPA) axis
CORT acts on the glucocorticoid receptor (GR) and mineralocorticoid receptor (MR) and is the end product of the HPA axis. CORT serves a broad function to recruit neural and peripheral resources to deal with threat and to restore homeostasis in the aftermath of stress (Nelson, Reference Nelson2005). In the central nervous system, CORT can facilitate shifts in brain networks to adaptively deal with stressful situations and to restore emotional reactivity (Hermans, Henckens, Joëls, & Fernández, Reference Hermans, Henckens, Joëls and Fernández2014). During pregnancy CORT levels rise in most mammalian species, including primates (Nelson, Reference Nelson2005; Saltzman & Maestripieri, Reference Saltzman and Maestripieri2011). Correlational studies in primates indicate that CORT is positively related to maternal and paternal responsiveness toward infants and can facilitate arousal-dependent initiation of caregiving behavior, especially in unexperienced parents (Saltzman & Maestripieri, Reference Saltzman and Maestripieri2011; Storey & Ziegler, Reference Storey and Ziegler2016). Studies in animals have further shown that action of corticotropin-releasing hormone (a component of the HPA axis preceding CORT) during parturition is critical for maternal responsiveness after birth (Broad, Keverne, & Kendrick, Reference Broad, Keverne and Kendrick1995), likely acting in concert with peptides such as AVP, OXT, and opioids (Olza-Fernández, Gabriel, Gil-Sanchez, Garcia-Segura, & Arevalo, Reference Olza-Fernández, Gabriel, Gil-Sanchez, Garcia-Segura and Arevalo2014). Thus, the relation between CORT and parenting behavior might be brought forth by the stress-induced upregulation of the HPA axis during parturition, which facilitates maternal recognition and bonding directly postpartum. An indication that such a role of CORT remains relevant later in life comes from a study that shows that in monogamous mammals, exposure to stress and CORT injections both induce partner preference (DeVries, DeVries, Taymans, & Carter, Reference DeVries, DeVries, Taymans and Carter1996).
Prolactin (PRL)
The physiological functions of PRL are diverse, but in mammalian species it is most known for its role in lactation. The function of this hormone might however extend to stress reduction, social learning, and maternal protection (Neumann, Reference Neumann2009). In rodents, PRL has convincingly been demonstrated to induce maternal behavior (Nelson, Reference Nelson2005), but its role in primate maternal behavior is unknown and has not received much attention (Saltzman & Maestripieri, Reference Saltzman and Maestripieri2011). PRL has been studied in relation to primate paternal behavior, and these studies show that PRL is most likely important to instigate caring behavior by motivating fathers for caregiving behavior (Storey, Noseworthy, Delahunty, Halfyard, & McKay, Reference Storey, Noseworthy, Delahunty, Halfyard and McKay2011), similar to the role of CORT described above.
Opioids
Opioids act on several receptor types to inhibit pain processing and are an important component in the neural reward circuitry by mediating affective responses to pleasurable stimuli (Nelson, Reference Nelson2005). During parturition, endogenous opioids may alleviate pain and together with OXT stimulate the onset of maternal behavior (Keverne & Kendrick, Reference Keverne and Kendrick1994). After parturition, opioids regulate attachment behaviors and mother–infant bonding by reducing separation anxiety and increasing motivation for social interaction (Nelson & Panksepp, Reference Nelson and Panksepp1998). Opioids also modulate primate maternal behavior, as variability in genes coding for the opioid receptor and pharmacological manipulations of the opioids system have demonstrated strong effects on maternal behavior (Curley, Reference Curley2011; Saltzman & Maestripieri, Reference Saltzman and Maestripieri2011).
PROG
In humans, during pregnancy PROG levels gradually increase, and this increase is important for the maintenance of the pregnancy. Although the role of PROG during pregnancy in relation to maternal behavior in most mammalian species is not entirely clear, in rats it is proposed to sensitize the brain for infant stimuli and to control the timing of this responsivity (Bridges, Reference Bridges2015). However, PROG levels during pregnancy follow a different profile in rats and humans and, thus, might serve a different function. In other mammals, such as in sheep, priming of OXT receptors by PROG (together with estradiol as described above) is essential for mother–infant bonding (Keverne & Kendrick, Reference Keverne and Kendrick1994). Data from primates has shown involvement of PROG in maternal caregiving, but no consistent pattern has emerged so far. Both positive and negative relations with maternal behavior have been found for PROG, depending on the species involved and the methodology used to measure endocrine levels (Saltzman & Maestripieri, Reference Saltzman and Maestripieri2011).
Endocrine interactions
The hormones and neuropeptides described in this review orchestrate complex social–emotional behavioral repertoires, which rely on neural networks that, although conserved throughout the mammalian lineage, have evolved over the course of millions of years. Hormonal factors can adaptively change the activation patterns of these neural networks, often in coordination with peripheral release, to deal with environmental cues. As such, variation in hormonal control allows for dynamic variation in response patterns between and within species (Goodson & Kabelik, Reference Goodson and Kabelik2009). Considering the coordinated fashion in which hormonal factors are involved in behavioral regulation, a single hormonal factor being responsible for a specific behavioral outcome is likely to be an exception. Although the role of the above hormones on behavior are generally described in isolation, it is important to stress that none of the reported endocrine factors function in isolation. Diverse interactive effects have been observed between steroid hormones, neuropeptides, and several neurotransmitter systems. For example, there is bidirectional control between the HPA and HPG axes, which might explain some of the sex-specific responses to stress (Viau, Reference Viau2002). As mentioned above, testosterone is easily converted into estradiol via aromatase in both males and females. Both estradiol and testosterone affect dopaminergic and serotonergic activity (de Souza Silva, Mattern, Topic, Buddenberg, & Huston, Reference de Souza Silva, Mattern, Topic, Buddenberg and Huston2009; McEwen & Alves, Reference McEwen and Alves1999) and have stimulating effects on the expression of OXT and AVP in several brain regions (de Vries, Reference de Vries2008). Estradiol further interacts with PROG and PRL function, as well as with the noradrenergic system (McEwen & Alves, Reference McEwen and Alves1999). In addition, several of the effects of OXT are established by interacting with the dopamine and opioid system (Rilling & Young, Reference Rilling and Young2014), and when released in high quantities, OXT and AVP can activate each other's receptors (Carter, Reference Carter2014). Because these examples are only a fraction of all possible interactions between the endocrine factors described in this review, it is important to realize that although the hormones and neuropeptides in the remainder of this review are discussed in relative isolation, this does not do justice to the complexity of the exact neuroendocrine mechanisms that mediate social–emotional behavior. Instead of representing approximations of the exact neuroendocrine mechanisms involved in human caregiving and its intergenerational transmission, the models proposed in this review are to be seen as heuristic models, guiding further research, based on which new hypotheses can be formulated.
Endocrinology of Human Caregiving
As described above, in addition to the basic needs of warmth, food, and protection that all mammals need (Farmer, Reference Farmer2000), a greater variety of caregiving behavior is required to ascertain a healthy outcome of a human child's social–emotional development. Caregivers need to be sensitive to a child's social signals, to show empathic responses to these signals, and to regulate emotional behavior such as inappropriate impulsive responses toward the child and its sometimes repulsive behavior (Joosen, Mesman, Bakermans-Kranenburg, & van IJzendoorn, Reference Joosen, Mesman, Bakermans-Kranenburg and van IJzendoorn2012; Milner, Reference Milner2003). This behavioral repertoire of social sensitivity, empathic responding, and emotion regulation is influenced by hormones. Correlational and administration studies have shown the effects of OXT, AVP, testosterone, estradiol, and CORT on these social–emotional behaviors; and these findings have been extensively reviewed elsewhere (Bos, Panksepp, et al., Reference Bos, Panksepp, Bluthe and Honk2012; McCall & Singer, Reference McCall and Singer2012; Montoya, Terburg, Bos, & Honk, Reference Montoya, Terburg, Bos and Honk2012; Putman & Roelofs, Reference Putman and Roelofs2011; van Anders et al., Reference van Anders, Goldey and Kuo2011). Of these endocrine factors, OXT has also been addressed in detail in relation to human caregiving behavior (Rilling, Reference Rilling2013; Rilling & Young, Reference Rilling and Young2014; Swain et al., Reference Swain, Kim, Spicer, Ho, Dayton, Elmadih and Abel2014). In the remainder of this section, I will focus on human studies and describe the proposed role of the currently known key endocrine factors in human caregiving, as well as highlight some possible endocrine candidates for future research. See the online-only supplementary materials for a table of all included studies and whether these studies were performed in humans or in other animals.
OXT and AVP
Of all of the hormonal factors, which effects on parenting are summarized in Figure 1, OXT has certainly received the most attention due to both the ease of administration (Guastella & MacLeod, Reference Guastella and MacLeod2012) and the strong link with parental and social bonding as evidenced by animal research as described above. Based on the considerable number of studies that employed OXT administration procedures in humans, it has convincingly been demonstrated that OXT increases emotion recognition in others (Shahrestani, Kemp, & Guastella, Reference Shahrestani, Kemp and Guastella2013), trust toward ingroup members (van IJzendoorn & Bakermans-Kranenburg, Reference van IJzendoorn and Bakermans-Kranenburg2012), and cognitive empathic behavior (Bos, Panksepp, et al., Reference Bos, Panksepp, Bluthe and Honk2012). OXT administration has further been shown to increase neural empathic responses to infant crying (Riem, Bakermans-Kranenburg, et al., Reference Riem, Bakermans-Kranenburg, Pieper, Tops, Boksem, Vermeiren and Rombouts2011) and functional connectivity of the amygdala with the orbitofrontal cortex (OFC; an important region critical for reward evaluation) in response to infant laughter (Riem, van IJzendoorn, et al., Reference van IJzendoorn, Huffmeijer, Alink, Bakermans-Kranenburg and Tops2011). This increased connectivity of the amygdala with frontal brain regions also indicates facilitation of emotion regulation (Kim et al., Reference Kim, Loucks, Palmer, Brown, Solomon, Marchante and Whalen2011). In addition, OXT responses in mothers after interaction with their child predicted neural reward responses in the ventral striatum toward their children's faces (Strathearn, Fonagy, Amico, & Montague, Reference Strathearn, Fonagy, Amico and Montague2009), indicating increased sensitivity for the rewarding properties of infant stimuli. Administration of OXT in fathers tended to result in less hostility (Naber, van IJzendoorn, Deschamps, van Engeland, & Bakermans-Kranenburg, Reference Naber, van IJzendoorn, Deschamps, van Engeland and Bakermans-Kranenburg2010) and increased touch and social reciprocity toward their children during interactive play (Weisman, Zagoory-Sharon, & Feldman, Reference Weisman, Zagoory-Sharon and Feldman2012). Outside the context of parent–infant interaction, OXT administration also leads to a reduction of physiological stress responses during social interaction (Heinrichs, Baumgartner, Kirschbaum, & Ehlert, Reference Heinrichs, Baumgartner, Kirschbaum and Ehlert2003) and to increased social bonding of adult partners during conflict (Ditzen et al., Reference Ditzen, Schaer, Gabriel, Bodenmann, Ehlert and Heinrichs2009). These effects might rely on the neurobiological mechanisms by which OXT exerts its soothing effects during mother–infant interaction after birth (Carter, Reference Carter1998; Panksepp, Reference Panksepp2009). For example, breastfeeding, which leads to upregulated OXT levels in both mother and infant, also results in reduced stress responses in mothers (Heinrichs, Neumann, & Ehlert, Reference Heinrichs, Neumann and Ehlert2002).
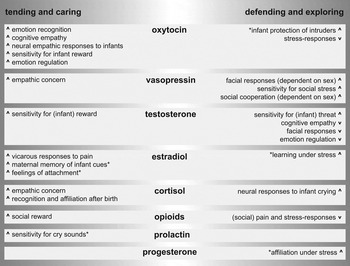
Figure 1. Model of the hormones and peptides relevant for human caregiving. The behavioral effects of the described hormones and neuropeptides are indexed depending on the relevant context, either in safe environments to elicit tending and caring behavior or in more unsafe or challenging environments supporting protecting and exploring behavior. Arrows next to the descriptions of the behavior are used to indicate whether the hormonal factors are expected to increase or decrease this type of behavior. *Behavioral effects observed in mammals, but not convincingly demonstrated in humans.
The OXT literature has also demonstrated the context dependency of the effects of OXT (Bakermans-Kranenburg & van IJzendoorn, Reference Bakermans-Kranenburg and van IJzendoorn2013; Bartz, Zaki, et al., Reference Bartz, Zaki, Bolger and Ochsner2011). A meta-analysis shows that the effects of OXT on trust are selective for ingroup members (van IJzendoorn & Bakermans-Kranenburg, Reference van IJzendoorn and Bakermans-Kranenburg2012), and OXT has also shown to increase protective decision making selectively for ingroup members (De Dreu et al., Reference De Dreu, Greer, Handgraaf, Shalvi, van Kleef, Baas and Feith2010). OXT has even been demonstrated to have antagonistic effects toward outgroup members (De Dreu, Greer, Vsn Kleef, Shalvi, & Handgraaf, Reference De Dreu, Greer, van Kleef, Shalvi and Handgraaf2011), effects that can all be interpreted as serving a protective function, whereby the prosocial effects of OXT are reserved for closely related others. Such a function of OXT bears similarities with increased maternal aggression toward intruders after OXT administration observed in rodents (Bosch, Meddle, Beiderbeck, Douglas, & Neumann, Reference Bosch, Meddle, Beiderbeck, Douglas and Neumann2005).
A neuropeptide related to OXT of which animal studies have shown considerable involvement in parenting behavior is AVP (e.g., Bosch & Neumann, Reference Bosch and Neumann2008; see Figure 1). Although studies on the role of AVP in human parenting are scarce, a recent study found a positive relation between AVP levels and parent–infant joint attention, as well as stimulatory touch (Apter-Levi, Zagoory-Sharon, & Feldman, Reference Apter-Levi, Zagoory-Sharon and Feldman2014). In addition, administration of AVP in expectant fathers increased their attention for infant stimuli compared to control fathers (Cohen-Bendahan, Beijers, van Doornen, & de Weerth, Reference Cohen-Bendahan, Beijers, van Doornen and de Weerth2015). Other studies on social behavior have shown that AVP administration increases sensitivity to social stress (Ebstein et al., Reference Ebstein, Israel, Lerer, Uzefovsky, Shalev, Gritsenko and Yirmiya2009) and aggressive facial responses to others (Thompson, George, Walton, Orr, & Benson, Reference Thompson, George, Walton, Orr and Benson2006), but also increased social cooperation (Brunnlieb et al., Reference Brunnlieb, Nave, Camerer, Schosser, Vogt, Münte and Heldmann2016; Rilling et al., Reference Rilling, DeMarco, Hackett, Chen, Gautam, Stair and Patel2014) and empathic concern for others (Tabak et al., Reference Tabak, Meyer, Castle, Dutcher, Irwin, Han and Eisenberger2015). Some of these effects are contrary to those of OXT, indicating that both peptides could have very different functions in relation to parenting behavior, relating to the strong sex differences that are observed after administration (Rilling et al., Reference Rilling, DeMarco, Hackett, Chen, Gautam, Stair and Patel2014; Thompson et al., Reference Thompson, George, Walton, Orr and Benson2006). Based on an overview of the animal and human literature, it is proposed that AVP and OXT are both important, yet serve different functions in challenging situations and in dealing with threat (see Figure 1). Especially OXT is likely to be more important in the reinstatement of homeostasis after stress and in coping with chronic stress by directing behavior more toward social engagement (Bos, Panksepp, et al., Reference Bos, Panksepp, Bluthe and Honk2012; Carter, Reference Carter2014; Neumann, Reference Neumann2009). The distinct roles these neuropeptides have in stress responding might translate to the proposed different roles they have in parental caregiving (Apter-Levi et al., Reference Apter-Levi, Zagoory-Sharon and Feldman2014). In this light it is important to consider the sex-specific effects that OXT and AVP can have on human social–emotional behavior. Although data on humans is scarce, research in other mammalian species show that sexes vary in the expression of OXT and AVP and in the location and density of their respective receptor distributions (Dumais & Veenema, Reference Dumais and Veenema2016). Such sex differences might contribute to the differences between maternal and paternal behavior and sex-specific dealing with stressful situations. However, due to paucity of data in humans, together with the often selective inclusion of only males or females in human clinical studies (Bos, Panksepp, et al., Reference Bos, Panksepp, Bluthe and Honk2012), such interpretations have to be considered with care.
Testosterone and estradiol
In contrast to the mostly beneficial effects of OXT on the behavioral repertoire of sensitive caregiving, the functions of testosterone are generally antagonistic to such parenting behavior. For example, testosterone reduces cognitive empathic behavior (van Honk et al., Reference van Honk, Schutter, Bos, Kruijt, Lentjes and Baron-Cohen2011), interpersonal trust (Bos, Terburg, & van Honk, Reference Bos, Terburg and van Honk2010), and automatic empathic facial responses toward others (Hermans, van Wingen, Bos, Putnam, & van Honk, Reference Hermans, van Wingen, Bos, Putman and van Honk2009). In addition, testosterone reduces neural connectivity of the amygdala with the prefrontal cortex (PFC; Bos, Hermans, Ramsey, & van Honk, Reference Bos, Hermans, Ramsey and van Honk2012; van Wingen, Mattern, Verkes, Buitelaar, & Fernandez, Reference van Wingen, Mattern, Verkes, Buitelaar and Fernandez2010), symptomatic for reduced emotion regulation (Kim et al., Reference Kim, Loucks, Palmer, Brown, Solomon, Marchante and Whalen2011). In fathers, basal testosterone levels are inversely related to the amount of caregiving they provide (Mascaro, Hackett, & Rilling, Reference Mascaro, Hackett and Rilling2013), and testosterone responses to erotic behavior in nonfathers are inversely related to interest in babies (Zilioli et al., Reference Zilioli, Ponzi, Henry, Kubicki, Nickels, Wilson and Maestripieri2016). Furthermore, longitudinal data has shown that parenthood results in reduced basal testosterone levels in both mothers and fathers, which in the light of the effects of testosterone indicates adaptive downregulation of testosterone function (Gettler, McDade, Feranil, & Kuzawa, Reference Gettler, McDade, Feranil and Kuzawa2011; Kuzawa, Gettler, Huang, & McDade, Reference Kuzawa, Gettler, Huang and McDade2010).
However, in some contexts, similar to the role of AVP in challenging situations, testosterone also has effects on social–emotional behavior that might be beneficial for caregiving behavior. Testosterone increases social vigilance (Hermans, Ramsey, & van Honk, Reference Hermans, Ramsey and van Honk2008), sensitivity to emotional facial expressions (Bos, Hermans, et al., Reference Bos, Hermans, Ramsey and van Honk2012; Bos, van Honk, Ramsey, Stein, & Hermans, Reference Bos, van Honk, Ramsey, Stein and Hermans2013), and sensitivity for reward (Hermans et al., Reference Hermans, Bos, Ossewaarde, Ramsey, Fernandez and van Honk2010). In fathers, basal testosterone levels correlate positively with neural activation in reward regions when observing their own children (Kuo, Carp, Light, & Grewen, Reference Kuo, Carp, Light and Grewen2012), and in nonmaternal women higher basal testosterone levels predict increased reward values for cuteness of infant faces (Hahn, DeBruine, Fisher, & Jones, Reference Hahn, DeBruine, Fisher and Jones2015). Administration of testosterone also increases neural activation in response to crying infants in the thalamocingulate circuit (Bos, Hermans, Montoya, Ramsey, & van Honk, Reference Bos, Hermans, Montoya, Ramsey and van Honk2010), a key circuit for parental responsiveness (MacLean, Reference MacLean1985). As such, testosterone might facilitate caregiving by serving a protective function when facing challenge or threat, but also in successfully dealing with infant distress. Tentative support for this notion comes from studies showing increases in testosterone levels in fathers listening to infant crying (Fleming, Corter, Stallings, & Steiner, Reference Fleming, Corter, Stallings and Steiner2002), whereas no such increase in testosterone is observed when listening to crying was combined with active nurturing behavior (van Anders, Tolman, & Volling, Reference van Anders, Tolman and Volling2012). Perhaps testosterone directly increases in response to crying, in order to motivate the parent for active caring behavior, followed by a fast decrease when care is provided. This view is not in conflict with the longitudinally observed decreases in basal testosterone levels, but merely shows that low baseline in combination with fast reactivity of the HPG axis could be optimal for caregiving behavior. Increased parental behavior was related to stronger declines in testosterone levels in fathers after the strange situation procedure (Kuo et al., Reference Kuo, Saini, Thomason, Schultheiss, Gonzalez and Volling2016). In addition, while baseline testosterone levels had no effect, stronger diurnal variability in testosterone levels in fathers was related to more sensitivity toward the child during play behavior (Endendijk et al., Reference Endendijk, Hallers-Haalboom, Groeneveld, van Berkel, van der Pol, Bakermans-Kranenburg and Mesman2016). Mothers however showed a different pattern, with higher baseline testosterone levels predicting more sensitivity. However, diurnal variability was inversely related to sensitivity toward the child, indicating a sex-specific role of testosterone in caregiving behavior. Perhaps similar to the context-dependent functions of OXT described above, the role of testosterone might be critical for “protective” parenting behavior, which is an indispensable behavioral repertoire in addition to more “tending” behavior, in order to guarantee reproductive success. As such, the role of testosterone might possibly be more important in paternal compared to maternal protective behavior, as maternal aggression is mediated partly by OXT. However, because testosterone administration studies have mostly been performed in females, and most baseline studies in males, more comparative studies between males and females such as Endendijk et al. (Reference Endendijk, Hallers-Haalboom, Groeneveld, van Berkel, van der Pol, Bakermans-Kranenburg and Mesman2016) should be performed to give more insight.
Another potential role of testosterone in parenting is indirectly, by conversion of testosterone to estradiol (see Endocrine interactions section). In humans, estradiol is known for its role in the development of women's secondary sexual characteristics and facilitation of ovulation and implantation during the menstrual cycle. It is surprising that only a few studies have been conducted investigating the role of estradiol in relation to human parenting. One study shows that mothers with higher levels of estradiol report higher postpartum feelings of attachment (Fleming, Ruble, Krieger, & Wong, Reference Fleming, Ruble, Krieger and Wong1997), whereas more indirect evidence for a role of estradiol in human parenting comes from a study in which variation of the ER polymorphism correlated with neural responses toward one's own child (Lahey et al., Reference Lahey, Michalska, Liu, Chen, Hipwell, Chronis-Tuscano and Decety2012). Research on the role of estradiol in other human social–emotional behavior is equally scarce, although a recent study in which estradiol was administered in males shows increased vicarious responses to pain observed in others (Olsson, Kopsida, Sorjonen, & Savic, Reference Olsson, Kopsida, Sorjonen and Savic2016), pointing at increased affective empathy after estradiol. In addition, there is evidence for a role of estradiol in emotional memory and fear learning in females (Zeidan et al., Reference Zeidan, Igoe, Linnman, Vitalo, Levine, Klibanski and Milad2011), which might relate to the role of estradiol in maternal memory for infant cues in other mammals (Banerjee & Liu, Reference Banerjee and Liu2013). Overall, this scarcity of studies on the relation between estradiol and human parenting, or other forms of social–emotional behavior, is surprising considering the number of women, including mothers, who take estradiol in the form of oral contraceptives on a daily basis. Comparative studies between naturally cycling women and those using oral contraceptives have shown differences in partner preference and selection (Little, Burriss, Petrie, Jones, & Roberts, Reference Little, Burriss, Petrie, Jones and Roberts2013), in emotional memory and stress responses (Nielsen, Segal, Worden, Kim, & Cahill, Reference Nielsen, Segal, Worden, Yim and Cahill2013), and in mood and neural responses to emotional stimuli (Gingnell et al., Reference Gingnell, Engman, Frick, Moby, Wikström, Fredrikson and Sundström-Poromaa2013). These effects might be due to the altered levels of estradiol in the oral contraception group, and therefore, more research on the role of estradiol in human (parenting) behavior is of utmost importance.
CORT
In the context of parenting, and similar to testosterone, CORT also increases in response to infant crying and predicts alertness to such crying (Fleming et al., Reference Fleming, Corter, Stallings and Steiner2002). Furthermore, CORT administration upregulates hippocampal responses to infant cry sounds (Bos, Montoya, Terburg, & van Honk, Reference Bos, Montoya, Terburg and van Honk2014). The hippocampus is also a critical region for social recognition (Hitti & Siegelbaum, Reference Hitti and Siegelbaum2014), and activation of the hippocampus has previously been related to levels of maternal bonding (Musser, Kaiser-Laurent, & Ablow, Reference Musser, Kaiser-Laurent and Ablow2012). In line with this, mothers with higher CORT levels are better able to distinguish infant cry sounds and respond more affectionately to these sounds (Stallings, Fleming, Corter, Worthman, & Steiner, Reference Stallings, Fleming, Corter, Worthman and Steiner2001). Similar effects are observed for different modalities, as higher CORT levels in mothers also predict increased sensitivity and recognition of olfactory infant cues (Fleming, Steiner, & Corter, Reference Fleming, Steiner and Corter1997). CORT might however also act on more deliberate and reflective behavior, as mothers with higher CORT also hold more positive attitudes toward their own children (Fleming, Ruble, et al., Reference Fleming, Ruble, Krieger and Wong1997). Thus, in line with studies in other primates, CORT in humans might similarly facilitate arousal-dependent initiation of caregiving behavior. Higher levels of CORT in males predicted stronger feelings of closeness toward a previously unknown interaction partner after social stress (Berger, Heinrichs, von Dawans, Way, & Chen, Reference Berger, Heinrichs, von Dawans, Way and Chen2016). Furthermore, a recent study showed that administration of a MR agonist results in more self-reported empathic concern for others (Wingenfeld et al., Reference Wingenfeld, Kuehl, Janke, Hinkelmann, Dziobek, Fleischer and Roepke2014), similar to effects of AVP and OXT. In contrast to these positive relations between CORT and human caregiving behavior, opposite relations have also been reported, which might be due to persistent high levels of CORT in the context of continued stress or depression, which can lead to suppression of maternal motivation (Saltzman & Maestripieri, Reference Saltzman and Maestripieri2011).
Other candidates: Opioids, PRL, and PROG
Despite the theoretical background on the role of these hormonal factors in shaping parental behavior, experimental studies in humans are scarce. The studies on human social behavior that have been performed so far show that opioids reduce social pain after rejection and facilitate sensitivity for social reward (Chelnokova et al., Reference Chelnokova, Laeng, Eikemo, Riegels, Løseth, Maurud and Leknes2014; Hsu et al., Reference Hsu, Sanford, Meyers, Love, Hazlett, Wang and Korycinski2013). Administration of naltrexone, an opioid antagonist, decreased feelings of social connection both in the laboratory and in daily life, which is a strong indicator of involvement of the opioid system in human social bonding (Inagaki, Ray, Irwin, Way, & Esenberger, Reference Inagaki, Ray, Irwin, Way and Eisenberger2016). PRL has been studied in relation to parenting, and in males, increased PRL levels have been observed in anticipation of fatherhood, as well as in fathers compared to nonfathers (Storey & Ziegler, Reference Storey and Ziegler2016). Other data from fathers shows that levels increase when listening to infant crying, and that higher levels predicted more alert and positive responses to the cry sounds (Fleming et al., Reference Fleming, Corter, Stallings and Steiner2002). In addition, baseline levels of PRL predicted exploratory play behavior in fathers when interacting with their 6-month-old infants (Gordon, Zagoory-Sharon, Leckman, & Feldman, Reference Gordon, Zagoory-Sharon, Leckman and Feldman2010). Overall, while these studies show that higher PRL levels predict increased paternal behavior, levels generally decline during father–infant interaction, which might indicate that PRL, comparable to the role of testosterone, is most important in initiation of paternal behavior rather than the actual caregiving behavior itself (Storey & Ziegler, Reference Storey and Ziegler2016). Nonetheless, these findings are promising and can hopefully spawn more research on the role of PRL in human parenting, although research in this direction is challenging due to the limited availability of methods to pharmacologically alter PRL levels in humans. Synthetic PRL, for example, is currently not available for administration purposes in humans. In contrast, PROG can be pharmacologically administered in humans, but effects of administration on human social behavior have not yet been investigated. Nonetheless, a few studies measured baseline PROG levels in response to social manipulations, and these studies suggest a role for PROG in facilitating affiliation during or after stress. More specifically, PROG increases after the observation of a movie clip showing social rejection, and correlated with the participants’ motivation to affiliate (Wirth & Schultheiss, Reference Wirth and Schultheiss2006). Another study measured increased PROG levels after social interaction with an unfamiliar other, which predicted altruistic responses to this person a week later (Brown et al., Reference Brown, Fredrickson, Wirth, Poulin, Meier, Heaphy, Cohen and Schultheiss2009). Finally, a recent study showed that after social rejection, PROG increased when given the opportunity to affiliate with others, specifically in individuals sensitive for social rejection, whereas socially anxious subjects showed a decrease in PROG after social rejection (Maner, Miller, Schmidt, & Eckel, Reference Maner, Miller, Schmidt and Eckel2010). These few studies suggest that, similar to opioids and PRL, future research on PROG hold great promise for increasing our understanding of the neurobiology underlying human social bonding and caregiving.
Causes of Endocrine Variability
Central to the topic of the current review is that the key endocrine factors that affect human caregiving behavior, as described in the overview above, do not affect all humans equally. As stated above, there is a tremendous variability in human caregiving styles, variation that has often been attributed to differences in socialization and learning, which leaves parents with different cognitive schemes or internal working models (Bretherton & Munholland, Reference Bretherton, Munholland, Cassidy and Shaver2008; Milner, Reference Milner2003). The claim made here, based on the literature described below, is that part of this variation observed in human caregiving depends on the variation in individuals’ endocrine systems. Individuals vary in their peripheral and central levels of the above described hormones and peptides (see Figure 1), in the sensitivity of their brain to these hormones and peptides, and also in sensitivity of peripheral nodes in their endocrine axis on which they exert part of their effects, such as the HPA and HPG axes. This variability in sensitivity to hormones and neuropeptides, together with variation in basal levels, might critically contribute to the variability in parenting practices observed later in life. Below, the essential sources of such endocrine variability will be described: genetic variation, fetal programming, parturition, and early postpartum conditions, as well as early life environmental factors, including experienced parenting.
Genetic variation of endocrine receptors
The primary ontogenetic factor that can explain variability in endocrine sensitivity is the variation in genes coding for the receptors of hormones and neuropeptides. Most research has been done on two single nucleotide polymorphisms (SNPs) of the OXTR gene, rs53576 and rs2254298, and these studies have generally attempted to investigate the direct effect of individual variation in SNPs on measures of social behavior. This approach has resulted in some promising findings. Individuals homozygous for the G allele of OXTR rs53576 polymorphism expressed more neural empathic responses to pain in others (Luo et al., Reference Luo, Li, Ma, Zhang, Rao and Han2015), showed stronger differentiation of neural responses to infant emotions in mothers (Peltola et al., Reference Peltola, Yrttiaho, Puura, Proverbio, Mononen, Lehtimäki and Leppänen2014), and showed higher levels of sensitive parenting (Bakermans-Kranenburg & van IJzendoorn, Reference Bakermans-Kranenburg and van IJzendoorn2008). Children homozygous for the G allele show stronger structural abnormalities in the ventral striatum after increasing early life trauma experiences (Dannlowski et al., Reference Dannlowski, Kugel, Grotegerd, Redlich, Opel, Dohm and Baune2015), pointing at increased vulnerability to early life stress in this group. In adults higher self-reported childhood attachment predicted alterations in neural structure and activation, in regions related to emotional processing, selectively in individuals homozygous for the G allele (Schneider-Hassloff et al., Reference Schneider-Hassloff, Straube, Jansen, Nuscheler, Wemken, Witt and Kircher2016). However, several inconsistent findings have been reported, such as reduced levels of positive parenting and accompanying neural empathic responses toward child stimuli for participants homozygous for the G allele (Michalska et al., Reference Michalska, Decety, Liu, Chen, Martz, Jacob and Lahey2014). In addition, a recent meta-analyses failed to find evidence for significant effects of the rs53576 and rs2254298 SNPs on social behavior (Bakermans-Kranenburg & van IJzendoorn, Reference Bakermans-Kranenburg and van IJzendoorn2014).
A difficulty in the approach of directly predicting behavior from variation in SNPs is that the underlying molecular function of these SNPs is currently unknown, which makes it difficult to compare the effects of these studies to endocrine administration and baseline studies. For example, the above-mentioned beneficial effects of a homozygous G allele on empathic responses (Luo et al., Reference Luo, Li, Ma, Zhang, Rao and Han2015) seem in contrast to a study in which administration of OXT results in reduced empathic neural responses to pain in others (Bos Montoya, Hermans, Keysers, & van Honk, Reference Bos, Montoya, Hermans, Keysers and van Honk2015). Thus, critical to further our understanding of how variation in OXTR SNPs contributes to variation in caregiving behavior is understanding the functional significance of these SNPs on the neurobiological level. A first step is a study in humans that shows that rs2254298 GG carriers have lower levels of peripheral OXT, which subsequently predict higher depression and risk of child pathology (Apter-Levy, Feldman, Vakart, Ebstein, & Feldman, Reference Apter-Levy, Feldman, Vakart, Ebstein and Feldman2013). A promising approach that can give critical insight in how SNPs alter neuroendocrine sensitivity is the combination of hormone administration paradigms with genetic analyses. Employing this approach, Marsh et al. (Reference Marsh, Henry, Pine, Gorodetsky, Goldman and Blair2012) show that rs53576 GG allele carriers are more sensitive to infant faces after OXT administration, pointing at increased sensitivity for OXT. Another recent study following a similar approach, yet focusing on several OXTR SNPs, shows that the rs53576 A allele was associated with increased emotion recognition performance under OXT versus placebo (Chen et al., Reference Chen, Kumsta, Dvorak, Domes, Yim, Ebstein and Heinrichs2015), which together with the study by Marsh et al. (Reference Marsh, Henry, Pine, Gorodetsky, Goldman and Blair2012) is in line with the studies described above by Luo et al. (Reference Luo, Li, Ma, Zhang, Rao and Han2015) and Bakermans-Kranenburg and van IJzendoorn (Reference Bakermans-Kranenburg and van IJzendoorn2008). In addition, a less well-studied SNP (rs401015) modulated activation of the amygdala to adult faces after OXT administration (Montag, Sauer, Reuter, & Kirsch, Reference Montag, Sauer, Reuter and Kirsch2013). Studies such as these are promising attempts to bridge the gap in the causal chain between genetic variation and variation in caregiving behavior.
A genetic polymorphism in which biochemical function is better understood is variation in the number of repeats of CAG trinucleotide in the first exon of the AR. The number of CAG repeats is inversely related to sensitivity of the AR, on which testosterone exerts most of its genomic effects (Chamberlain, Driver, & Miesfeld, Reference Chamberlain, Driver and Miesfeld1994). Consistent with this inverse relation is the finding that men with shorter CAG repeat lengths exhibited greater upper body strength, scored higher on self-report measures of dominance (Simmons & Roney, Reference Simmons and Roney2011; but see Hurd, Vaillancort, & Dinsdale, Reference Hurd, Vaillancourt and Dinsdale2011), and had increased activity of the amygdala to facial expressions of negative affect (Manuck et al., Reference Manuck, Marsland, Flory, Gorka, Ferrell and Hariri2010). These are all measures typically related to testosterone function (Bos, Panksepp, et al., Reference Bos, Panksepp, Bluthe and Honk2012). In addition, shorter CAG repeat lengths also predicted larger testosterone responses when males interacted with young women (Roney, Simmons, & Lukaszewski, Reference Roney, Simmons and Lukaszewski2010) and less empathic neural responses to infant crying in fathers (Mascaro, Hackett, Gouzoules, Lori, & Rilling, Reference Mascaro, Hackett, Gouzoules, Lori and Rilling2014). Furthermore, baseline salivary levels of testosterone have shown to correlate positively with CAG repeat lengths (Manuck et al., Reference Manuck, Marsland, Flory, Gorka, Ferrell and Hariri2010). This might indicate a compensation mechanism where higher sensitivity downregulates physiological levels of testosterone, which illustrates the difficulties of studying endocrine parameters in isolation.
This notion becomes even clearer when considering the diverse pathways by which testosterone can influence brain function. One of these pathways is the conversion of testosterone to estradiol by aromatase. This pathway is of great relevance, because aromatase can act quickly and estradiol has diverse effects on social behavior and the brain, both genomically and nongenomically. Because variation in SNPs for the ER predicted mother's neural responses to their own children, and indirectly predicted observed negative parenting, this pathway might account for some of the effects ascribed to testosterone. With regard to fast effects of aromatase, this gives infinite temporal flexibility (Balthazart, Taziaux, Holloway, Ball, & Cornil, Reference Balthazart, Taziaux, Holloway, Ball and Cornil2009; McEwen & Alves, Reference McEwen and Alves1999). Considering that aromatase is densely located in regions of the human brain such as the amygdala (Biegon et al., Reference Biegon, Kim, Alexoff, Jayne, Carter, Hubbard and Fowler2010), some of the effects seen after testosterone administration might be caused by local conversion to estradiol. A further step in scrutinizing this pathway is to find the genes that can explain variation in the functionality of this cascade. Such a gene is the recently identified transcription factor retinoic acid receptor-related orphan receptor alpha (RORA), which regulates expression of aromatase (Sarachana, Xu, Wu, & Hu, Reference Sarachana, Xu, Wu and Hu2011). In addition, the expression of RORA itself is controlled by steroid hormones, as it is downregulated by the androgen dihydrotestosterone and upregulated by estradiol, making RORA an additional interesting target to study individual variation in relation to caregiving or other steroid regulated social behavior.
Another candidate is the gene coding for the mu-opioid receptor M1 (OPRM1), which predicts variation in neural and dispositional sensitivity for social rejection in humans (Way, Taylor, & Eisenberger, Reference Way, Taylor and Eisenberger2009), as well as self-reported fearful attachment (Troisi et al., Reference Troisi, Frazzetto, Carola, Di Lorenzo, Coviello, Siracusano and Gross2012). Animal studies have shown a strong link between OPRM1 and mother–infant attachment (Curley, Reference Curley2011). Finally, variation in the genes coding for AVP receptor 1a (AVPR1a) and MR are of interest because the function of these receptors have both been related to empathy. Although it warrants replication, a single study reported that variation in the AVPR1a genes correlated with self-reported trait cognitive empathy (Uzefovsky et al., Reference Uzefovsky, Shalev, Israel, Edelman, Raz, Mankuta and Ebstein2015). The relevance of the MR is demonstrated by a study showing that administration of an MR agonist increased self-reported empathic concern (Wingenfeld et al., Reference Wingenfeld, Kuehl, Janke, Hinkelmann, Dziobek, Fleischer and Roepke2014).
Despite the importance of genetic variation as a first etiological factor for endocrine sensitivity, the vast complexity of the intermediate steps from genes to phenotypic outcomes limits the predictive values of the “candidate genes approach” (Chabris et al., Reference Chabris, Hebert, Benjamin, Beauchamp, Cesarini, van der Loos and Laibson2012). Regarding this complexity, a highly interesting and relatively new research area is the field of epigenetics, which looks at how genetic expression can be changed under the influence of environmental factors, such as early childhood or even prenatal experiences, without changing the genetic code itself (Zhang & Meaney, Reference Zhang and Meaney2010). Although the field of human epigenetics is young, the few studies that have been done in humans on epigenetic expression of endocrine receptors are promising. For example, increased methylation of the OXTR gene, which generally causes reduced expression of the receptor, resulted in stronger neural responses to negative emotional expressions and reduced connectivity of the PFC with the amygdala, which could suggest less efficient emotion regulation (Puglia, Lillard, Morris, & Connelly, Reference Puglia, Lillard, Morris and Connelly2015). This finding is in line with a study showing increased OXTR methylation in children that develop callous–unemotional traits (Cecil et al., Reference Cecil, Lysenko, Jaffee, Pingault, Smith, Relton and Barker2014) and in individuals with autism (Gregory et al., Reference Gregory, Connelly, Towers, Johnson, Biscocho, Markunas and Ellis2009). However, a first study that investigated depression found decreased methylation of the OXTR gene compared to healthy controls, and further showed that this effect depended on both the exact location of the methylation and on the polymorphism of the OXTR gene (Reiner et al., Reference Reiner, van IJzendoorn, Bakermans-Kranenburg, Bleich, Beutel and Frieling2015). This preliminary finding forewarns that the relation between epigenetic expression and phenotypic outcome is complex. In addition, another recent finding demonstrates the temporal dynamics of such methylation. Subjects exposed to social stress showed increased OXTR methylation directly after stress exposure, which normalized again in a follow-up the year after (Unternaehrer et al., Reference Unternaehrer, Luers, Mill, Dempster, Meyer, Staehli and Meinlschmidt2012).
Research on the epigenetic expression of the CORT receptors has revealed strong sensitivity of the GR and the FK506 binding protein 5 (FKBP5) gene methylation to early life stress in humans (Klengel et al., Reference Klengel, Mehta, Anacker, Rex-Haffner, Pruessner, Pariante and Bradley2013; Kundakovic & Champagne, Reference Kundakovic and Champagne2015). FKBP5 is an important regulator of the GR complex, and childhood trauma affects transcription of FKBP5, which results in long-term alteration of the stress system and hippocampal structure (Klengel et al., Reference Klengel, Mehta, Anacker, Rex-Haffner, Pruessner, Pariante and Bradley2013). Because epigenetic expression of the GR and FKBP5 polymorphisms have both been related to mood and anxiety disorders (e.g., Klengel et al., Reference Klengel, Mehta, Anacker, Rex-Haffner, Pruessner, Pariante and Bradley2013; Vukojevic et al., Reference Vukojevic, Kolassa, Fastenrath, Gschwind, Spalek, Milnik and Demougin2014), studies on epigenetic expression of endocrine receptors can contribute to increased understanding of the etiology of stress-related psychopathology. In addition, these studies also pave the way for an explanatory mechanism for the environmental effects of endocrine sensitivity and subsequent variation in caregiving behavior. Already starting before conception, the environment alters the epigenetic expression of individuals (Gapp et al., Reference Gapp, Jawaid, Sarkies, Bohacek, Pelczar, Prados and Mansuy2014). In the remainder of this review, the focus will be on the sensitive periods from fetal development to early childhood.
Fetal programming
The time spent in the womb is the first sensitive period after conception that is important in the regulation of endocrine sensitivity. During this period, testosterone levels have strong organizational effects on the brain. A line of studies has investigated the effect of fetal testosterone as measured from amniotic fluid in pregnant women on the development of children after birth. These studies show that fetal testosterone predicts the children's brain structure in a sexually dimorphic way (Lombardo, Ashwin, Auyeyng, Chakrabarti, Taylor, et al., Reference Lombardo, Ashwin, Auyeung, Chakrabarti, Lai, Taylor and Baron-Cohen2012). That is, in areas involved in cognitive empathy, such as the temporoparietal junction and the inferior frontal gyrus, gray matter volume was predicted by fetal testosterone consistent with observed sex differences in these children. Fetal testosterone further predicted sex-typical play behavior (Auyeung et al., Reference Auyeung, Baron-Cohen, Ashwin, Knickmeyer, Taylor, Hackett and Hines2009) and neural responses to socially rewarding stimuli (Lombardo, Ashwin, Auyeung, Chakrabarti, Lai, et al., Reference Lombardo, Ashwin, Auyeung, Chakrabarti, Lai, Taylor and Baron-Cohen2012), similar to the effects of testosterone described above. Finally, in a large longitudinal data sample, elevated levels of testosterone and PROG were found in amniotic fluid in individuals diagnosed with autism later in life (Baron-Cohen et al., Reference Baron-Cohen, Auyeung, Nørgaard-Pedersen, Hougaard, Abdallah, Melgaard and Lombardo2015). A different line of studies investigated effects in women exposed to elevated levels of androgens due to the rare genetic disease congenital adrenal hyperplasia. These women show masculinized behavior and interests, including reduced interest in infants (Leveroni & Berenbaum, Reference Leveroni and Berenbaum1998) and motherhood (Ehrhardt & Meyer-Bahlburg, Reference Ehrhardt and Meyer-Bahlburg1981). These findings show that fetal testosterone has organizational effects on the infants’ brain, which can lead to sex-typical differences in caregiving behavior later in life. In addition, fetal testosterone also seems to alter sensitivity to testosterone surges later in life. A study by van Honk et al. (Reference van Honk, Schutter, Bos, Kruijt, Lentjes and Baron-Cohen2011) showed that administration of testosterone reduced cognitive empathic behavior in women, and that the effect was dependent on those women with a low ratio of the second and fourth digit of the right hand (2D/4D ratio), which is a marker of fetal exposure to testosterone (Breedlove, Reference Breedlove2010). Thus, increased exposure to fetal testosterone can increase later life sensitivity to testosterone, resulting in subsequent alterations in caregiving behavior. Preliminary data shows that a low 2D/4D and low maternal warmth predicted self-reported anxious attachment later in life (Blanchard, Lyons, & Nelson, Reference Blanchard, Lyons and Nelson2014), whereas self-reported attachment is related to more positive parenting behavior (Jones, Cassidy, & Shaver, Reference Jones, Cassidy and Shaver2015).
Furthermore, a recent finding suggests that the effects of prenatal priming by testosterone interact with sensitivity for OXT (Weisman, Pelphrey, et al., Reference Weisman, Pelphrey, Leckman, Feldman, Lu, Chong and Ebstein2015). The study shows that cognitive empathy was predicted by the 2D/4D ratio selectively in carriers of the GG allele of the OXTR gene rs53576, whereas in carriers of the A allele, no such effect for this proxy of fetal testosterone was found. Whether exposure to OXT and estradiol in the womb during pregnancy can affect later life sensitivity to these endocrine factors has not yet been investigated, but a few studies have demonstrated that OXT levels in mothers during pregnancy predict maternal behavior postpartum, such as increased maternal bonding (Feldman, Weller, Zagoory-Sharon, & Levine, Reference Feldman, Weller, Zagoory-Sharon and Levine2007) and also a decreased risk of postpartum depression (Skrundz, Bolten, Nast, Hellhammer, & Meinlschmidt, Reference Skrundz, Bolten, Nast, Hellhammer and Meinlschmidt2011). These studies should be interpreted with caution, because the measurement procedure for OXT in these studies have been proven unreliable (McCullough, Churchland, & Mendez, Reference McCullough, Churchland and Mendez2013); therefore, replications with more sensitive OXT measures are needed. Nonetheless, because both OXT and testosterone can easily cross the placenta, the above findings give ground to speculate that testosterone and OXT prenatally affect both the child's and the mother's neuroendocrine system underlying social bonding behaviors. Although the relation between prenatal estradiol and caregiving behavior has not yet been studied, a recent study does show that epigenetic markers for postpartum depression are related to changes in estradiol during pregnancy (Osborne et al., Reference Osborne, Clive, Kimmel, Gispen, Guintivano, Brown and Braier2015). This indicates that epigenetic variation of these markers might contribute in the etiology of postpartum depression by mediating endocrine sensitivity.
The programming effects of prenatal stress on the HPA axis in children and adults are relatively well studied (Bowers & Yehuda, Reference Bowers and Yehuda2016). Extensive animal literature shows downregulating effects of prenatal stress on epigenetic MR and GR expression, which is most pronounced at the level of the hippocampus (Zhang, Labonte, Wen, Turecki, & Meaney, Reference Zhang, Labonte, Wen, Turecki and Meaney2013). This literature also shows increased HPA-stress responses, most likely caused by reduced downregulating effects of the hippocampus on the HPA axis (Lupien, McEwen, Gunnar, & Heim, Reference Lupien, McEwen, Gunnar and Heim2009). Studies in humans suggest similar increased HPA axis activation in infants after prenatal stress, and preliminary data indicate that maternal anxiety and CORT levels affect epigenetic expression of the infants’ GR, pointing at comparable mechanisms in humans to those described in rodents (Hompes et al., Reference Hompes, Izzi, Gellens, Morreels, Fieuws, Pexsters and Freson2013). In addition, CORT levels measured from amniotic fluid in mothers predicted low infant birth weight, which subsequently predicted infant distress at 3 months (Baibazarova et al., Reference Baibazarova, van de Beek, Cohen-Kettenis, Buitelaar, Shelton and van Goozen2013). Finally, animal work shows that some of the effects of prenatal stress can be countered by postnatal infusion of OXT (Lee, Brady, Shapiro, Dorsa, & Koenig, Reference Lee, Brady, Shapiro, Dorsa and Koenig2007), indicating a possible therapeutic role of OXT administration for altered function of the HPA axis after prenatal stress. Taken together, the above findings show that prenatal exposure to testosterone and CORT results in long-term endocrine alterations, which could change the developmental trajectory in which child–parent interactions shape the child's caregiving behavior later in life.
Parturition and early postpartum
Perhaps the least studied period in human development during which endocrine factors can mark human development is the timeframe of parturition and the early postpartum period. That this period is understudied is surprising considering the strong cultural and historical variation in caregiving behaviors associated with this period. For example, medical advances in Western societies have resulted in the widely applied practices of epidural anesthesia, synthetic OXT injections to facilitate contractions, caesarean section (CS), immediate mother–infant separation upon birth, bottle feeding, and reduced body contact due to separate sleeping. Some of these routines became common practice as recent as in the last few decades. Whereas the 1970 CS rate in the United States was only 5% (Sewell, Reference Sewell1993), today over 30% of deliveries in the United States are performed by CS, with numbers up to 48% for other Western countries (Olza-Fernández et al., Reference Olza-Fernández, Gabriel, Gil-Sanchez, Garcia-Segura and Arevalo2014). This strong rise in CS is concerning, because CS has been related to adverse health outcomes in children (Blustein & Liu, Reference Blustein and Liu2015). Preliminary data also suggest a link between CS and autism, as well as mood and anxiety disorders (Olza-Fernández et al., Reference Olza-Fernández, Gabriel, Gil-Sanchez, Garcia-Segura and Arevalo2014). Although such epidemiological data suffers from methodological difficulties, a critical difference between CS and normal delivery is the absence of a hormonal cascade resulting in high levels of OXT, AVP, CORT, and catecholamines such a noradrenaline in both mother and infant during CS. This hormonal cascade during natural birth has been brought forth as a critical period in the etiology of autism (Ben-Ari, Reference Ben-Ari2015). Studies in mammals have further shown that administration of OXT and AVP in infants directly after birth has long-lasting effects on social–emotional behavior, including parenting behavior (Hammock, Reference Hammock2015; Miller & Caldwell, Reference Miller and Caldwell2015), and that in some species OXT is essential for the formation of the mother–infant bond (Kendrick, Reference Kendrick2000). In humans, OXT levels in the infant directly after birth predicted infant social engagement at 3 and 6 months of age (Clark et al., Reference Clark, John, Pasca, Hyde, Hornbeak, Abramova and Penn2013). Postnatal CORT levels in mothers are related to maternal sensitivity and affection (Fleming, Ruble, et al., Reference Fleming, Ruble, Krieger and Wong1997; Stallings et al., Reference Stallings, Fleming, Corter, Worthman and Steiner2001) and to better olfactory recognition of their infants (Fleming, Steiner, et al., Reference Fleming, Steiner and Corter1997). Infants delivered by CS, but exposed to contractions before CS, show better olfactory recognition in the first days after birth compared to infants not exposed to contraction (Varendi, Porter, & Winberg, Reference Varendi, Porter and Winberg2002), suggesting that the endocrine cascade during childbearing facilitates social interaction and olfactory learning in both mother and infant. The absence of this hormonal cascade could also account for the reduced neural responses in mothers to their infants’ crying after CS compared to normal delivery (Swain et al., Reference Swain, Tasgin, Mayes, Feldman, Constable and Leckman2008).
Besides CS, another recent practice with possible longer lasting endocrine effects is the common use of administration of synthetic OXT (pitocin) to facilitate delivery. Use of pitocin has been related to an increased risk for autism (Weisman, Agerbo, et al., Reference Weisman, Agerbo, Carter, Harris, Uldbjerg, Henriksen and Dalsgaard2015) and has been implicated in problems with initiation of breastfeeding, with subsequent effects on OXT function (Odent, Reference Odent2013; Olza-Fernández et al., Reference Olza-Fernández, Gabriel, Gil-Sanchez, Garcia-Segura and Arevalo2014). Although conclusive studies are not available, similar problems with breastfeeding have been related to the use of epidural anesthesia (French, Cong, & Chung, Reference French, Cong and Chung2016). Thus, pitocin administration, CS (but also reduced body contact between mother and infant), and bottle-feeding compared to breastfeeding strongly reduce maternal and infant exposure to OXT after birth, which can interfere with the synchronous neurobiological mechanisms in mother and infant and can thereby hamper the establishment of mother–infant attachment (Feldman, Reference Feldman2015). For example, longer duration of breastfeeding is positively associated with sensitive responsiveness and attachment security (Tharner et al., Reference Tharner, Luijk, Raat, IJzendoorn, Bakermans-Kranenburg, Moll and Tiemeier2012), effects that might be related to upregulation of OXT function. In addition, mother–infant separation in the first 2 hr after birth compared to skin to skin contact and suckling resulted in reduced maternal sensitivity, infant self-regulation, and mother–infant interaction 1 year later (Bystrova et al., Reference Bystrova, Ivanova, Edhborg, Matthiesen, Ransjö-Arvidson, Mukhamedrakhimov and Widström2009). Findings such as these suggest a considerable impact of the early postpartum period on development and stresses the need for more research on this sensitive period.
Early life environment
One of the strongest environmental factors during early development that affect neuroendocrine sensitivity is the experience of stressful situations. Although stress can be caused by various sources, most detrimental is stress caused, or accompanied by, inadequate caregiving behavior. Because the primary caregivers are considered a pivotal source of social support and stress buffering (Hostinar & Gunnar, Reference Hostinar and Gunnar2013), parenting practices such as physical and emotional abuse and neglect not only induce stress but also deprive the child of a valuable protective factor against stress: the safety and security provided by caregivers. Although it is difficult to experimentally study in humans, the effects of such harsh caregiving practices on the neuroendocrine outcomes of child development have been substantially studied, mostly due to the strong effects this behavior has on social–emotional behavior and the risk it brings for psychopathology (e.g., Andersen & Teicher, Reference Andersen and Teicher2009; Cicchetti & Toth, Reference Cicchetti and Toth2009; Heim & Nemeroff, Reference Heim and Nemeroff2001).
Effects on the HPA axis
Activation of the HPA axis in response to stressful situations is adaptive as a means to minimize the impact of a stressor, but a prolonged increase of CORT levels can have neurotoxic effects (Lupien et al., Reference Lupien, McEwen, Gunnar and Heim2009; McEwen, Reference McEwen2010). In humans, especially the effects of early life stress on the HPA axis have been investigated, and these studies are comprehensively described elsewhere (Lanius et al., Reference Lanius, Vermetten and Pain2010; Lupien et al., Reference Lupien, McEwen, Gunnar and Heim2009; Struber, Struber, & Roth, Reference Struber, Struber and Roth2014). Overall, these studies show that early life stress can have robust enduring effects on the HPA axis, resulting in altered levels of, and sensitivity to, CORT. This body of research also shows that the effects of early life stress are dependent on the severity and timing of the experienced stress. Whereas severe early life adversity such as trauma and parental separation has been shown to result in lowered CORT baseline levels and stress reactivity, both hypo- and hyperactivation of the HPA axis have been observed after moderate stress induced by harsh caregiving (Lovallo, Reference Lovallo2013; Lupien et al., Reference Lupien, McEwen, Gunnar and Heim2009; Struber et al., Reference Struber, Struber and Roth2014).
An explanation for these diverse findings is that, in contrast to moderate or intermittent stress, sustained exposure to stressful experiences will dampen sensitivity to activation of the HPA axis due to the neurotoxic effects of long-term increases of CORT levels (McEwen, Reference McEwen2010). Animal studies show that especially at the level of the hippocampus, which has a downregulating effect on the HPA axis (Jacobson & Sapolsky, Reference Jacobson and Sapolsky1991), expression of CORT receptors is strongly affected by variation in maternal care (Curley, Jensen, Mashoodh, & Champagne, Reference Curley, Jensen, Mashoodh and Champagne2011; Meaney, Reference Meaney2001). Maternal separation in rats results in reduced GR and MR expression in the hippocampus and also in increased basal and stress-induced CORT levels later in life, whereas a moderate stressor in the form of short daily separation from the mother resulted in an opposite pattern of CORT receptor expression and behavioral stress responses (Meaney, Reference Meaney2001; Oitzl, Champagne, van der Veen, & de Kloet, Reference Oitzl, Champagne, van der Veen and de Kloet2010; but see Struber et al., Reference Struber, Struber and Roth2014). These studies also show that the alterations in stress responses induced by exposure to variation in parenting translated to altered parenting behavior later in life. Thus, variation in exposure to caregiving early in life can (depending on severity) either up- or downregulate hippocampal responses to CORT and thereby change the negative feedback loop of the HPA axis that prevents the adverse effects of CORT on the central nervous system. As such, depending on the exact developmental trajectory, harsh caregiving can result in both higher and lower CORT levels. This is reflected in the sometimes inconsistent findings from studies that investigate baseline levels of CORT in children exposed to institutionalized care and social deprivation. Whereas lower baselines and flattened diurnal patterns of CORT have been repeatedly found (Gunnar & Vazquez, Reference Gunnar and Vazquez2001), other studies have reported higher levels of CORT in children under poor caregiving conditions (Dobrova-Krol, van IJzendoorn, Bakermans-Kranenburg, Cyr, & Juffer, Reference Dobrova-Krol, van IJzendoorn, Bakermans-Kranenburg, Cyr and Juffer2008; Gunnar, Morison, Chisholm, Schuder, Reference Gunnar, Morison, Chisholm and Schuder2001). Longitudinal studies can provide more insight into what brings forth such alterations in CORT levels. A recent study by Laurent, Harold, Leve, Shelton, and van Goozen (Reference Laurent, Harold, Leve, Shelton and van Goozen2016) in a low-risk sample shows that in children of less sensitive and more intrusive mothers CORT responses to stress increase throughout the first 3 years of life, pointing at increased sensitivity to stress in children exposed to such a parenting style. A study by Jaffe et al. (Reference Jaffee, McFarquhar, Stevens, Ouellet-Morin, Melhuish and Belsky2015) shows that children subjected to more severe, harsh parenting at the age of 3 have reduced CORT responses to the stressor after recent exposure to traumatic events at around 10 years old, which corroborates with other findings of reduced CORT responses after direct stress (e.g., Trickett, Gordis, Peckins, & Susman, Reference Trickett, Gordis, Peckins and Susman2014). However, in children who had not been subjected to harsh parenting, increased CORT responses were observed, similar to the increased CORT responses observed in children exposed to less sensitive and more intrusive parenting (Laurent et al., Reference Laurent, Harold, Leve, Shelton and van Goozen2016). These findings might indicate altered sensitivity to CORT, due to adaptive up- or downregulation of the HPA axis, depending on the severity of experienced harsh caregiving. However, only few studies have attempted to directly investigate variation in human sensitivity to CORT in relation to caregiving behavior.
Although the relation between experienced stress and trauma on epigenetic variation of the CORT receptor has been investigated (Bowers & Yehuda, Reference Bowers and Yehuda2016), only few studies have directly investigated the effect of harsh parenting on GR expression. These studies show that maltreatment and parental loss decreased GR expression in healthy adults, as indexed by heightened methylation of the promotor region nuclear receptor subfamily 3, group C, member 1 (NR3C1) of the GR (Tyrka, Price, Marsit, Walters, & Carpenter, Reference Tyrka, Price, Marsit, Walters and Carpenter2012) and also decreased GR expression on the level of the hippocampus in suicide victims exposed to abuse (McGowan et al., Reference McGowan, Sasaki, D'Alessio, Dymov, Labonté, Szyf, Turecki and Meaney2009). Furthermore, a study by Schechter et al. (Reference Schechter, Moser, Paoloni-Giacobino, Stenz, Gex-Fabry, Aue and Serpa2015) shows that in mothers with PTSD who were exposed to interpersonal violence, methylation of NR3C1 was negatively related to PTSD symptoms and current maternal stress, and positively with medial PFC responses to videos of mother–infant interaction. Applying a different approach, Bos et al. (Reference Bos, Montoya, Terburg and van Honk2014) administered CORT in healthy young adults and used functional magnetic resonance imaging to investigate hippocampal responses to infant crying. In this study, increased self-reported parental neglect predicted stronger hippocampal sensitivity to CORT administration in response to infant crying. This upregulation of the hippocampus by CORT might relate to the increased CORT levels in fathers when listening to infant crying (Fleming et al., Reference Fleming, Corter, Stallings and Steiner2002). Such a physiological stress response to cry sounds might be relevant for care behavior. Although CORT responses to cry sounds have not been related to caregiving behavior, higher levels of alpha-amylase, a marker for activation of the sympathetic nervous system, has been related to intended harsh caregiving in response to infant crying (Out, Pieper, Bakermans-Kranenburg, Zeskind, & van IJzendoorn, Reference Out, Pieper, Bakermans-Kranenburg, Zeskind and van IJzendoorn2010). Thus, if increased hippocampal sensitivity to CORT can result in attenuated physiological stress responses to infant crying, this might facilitate sensitive caregiving. Nonetheless, increased hippocampal sensitivity to CORT seems in contrast to the reduced GR expression in the epigenetic studies. There was however a considerable difference in severity of exposure to harsh caregiving in these studies, with maltreatment in individuals that committed suicide by McGowan et al. (Reference McGowan, Sasaki, D'Alessio, Dymov, Labonté, Szyf, Turecki and Meaney2009), parental loss by Tyrka et al. (Reference Tyrka, Price, Marsit, Walters and Carpenter2012), and interpersonal violence leading to PTSD in Schechter et al. (Reference Schechter, Moser, Paoloni-Giacobino, Stenz, Gex-Fabry, Aue and Serpa2015), yet only low to moderate levels of exposure to neglect in the study by Bos et al. (Reference Bos, Montoya, Terburg and van Honk2014). Perhaps comparable to the animal studies described above, minor exposure to neglect may result in increased hippocampal sensitivity to CORT, whereas more severe neglect and maltreatment may reduce sensitivity to CORT (Curley et al., Reference Curley, Jensen, Mashoodh and Champagne2011; Oitzl et al., Reference Oitzl, Champagne, van der Veen and de Kloet2010). The effects of CORT administration on responses to infant crying may also be MR dependent, relating to the role of MR in empathic responses to adults (Wingenfeld et al., Reference Wingenfeld, Kuehl, Janke, Hinkelmann, Dziobek, Fleischer and Roepke2014). In addition, it is known from other studies that harsh caregiving also acts on other nodes of the HPA axis, such as basal levels of and sensitivity for corticotropin-releasing hormone, with subsequent effects on CORT levels (Heim, Newport, Mletzko, Miller, & Nemeroff, Reference Heim, Newport, Mletzko, Miller and Nemeroff2008; Tyrka et al., Reference Tyrka, Wier, Price, Ross, Anderson, Wilkinson and Carpenter2008). These cross-sectional studies in which only offspring are tested do however suffer from the difficulty that because the parents were not investigated, one cannot easily dissociate between endocrine effects specifically caused by parenting behavior or inherited factors that act on both endocrinology and parenting behavior. Another important factor that is not always taken into account in such studies is the influence of current psychopathology on the HPA axis, which can be confounded with HPA alterations due to experienced caregiving. For example, higher self-reported exposure to early childhood adversity in healthy subjects results in blunted CORT responses to social stress (Elzinga et al., Reference Elzinga, Roelofs, Tollenaar, Bakvis, van Pelt and Spinhoven2008), whereas in patients with social anxiety disorder, higher levels on the same self-report measure resulted in increased CORT responses (Elzinga, Spinhoven, Berretty, de Jong, & Roelofs, Reference Elzinga, Spinhoven, Berretty, de Jong and Roelofs2010). In the opposite direction, many endocrine abnormalities that have previously been related to psychopathology, such as depression, might actually be due to experienced childhood adversity, such as harsh parenting. A recent study showed reduced baseline CORT levels in response to harsh parenting unrelated to current depression (Hinkelmann et al., Reference Hinkelmann, Muhtz, Dettenborn, Agorastos, Wingenfeld, Spitzer and Otte2013). In addition, reduced hippocampal size, which has often been related to major depressive disorder, has recently been shown to depend on exposure to harsh parenting, while major depressive disorder diagnoses did not explain additional variance in this effect (Opel et al., Reference Opel, Redlich, Zwanzger, Grotegerd, Arolt, Heindel and Sams2014).
In light of these difficulties, it is of critical importance to unravel the developmental pathway by which caregiving alters the HPA axis. As a step in this direction, it was recently demonstrated that maternal stress in the first year of life predicted cortisol responses in infants at the age of 4.5, which subsequently predicted decreased amygdala–PFC connectivity later in life, which was then related to increased levels of anxiety (Burghy et al., Reference Burghy, Stodola, Ruttle, Molloy, Armstrong, Oler and Birn2012). In addition, in mothers exposed to maltreatment and parental loss, stronger CORT responses predicted less sensitive parenting to their own children (Gonzalez, Jenkins, Steiner, & Fleming, Reference Gonzalez, Jenkins, Steiner and Fleming2012). Thus, although the exact mechanisms are still unknown, these findings make clear that the quality of caregiving has strong effects on later life social behavior, extending to caregiving behavior, and that alteration of the HPA axis plays an important role in the intergenerational transmission of this behavior.
Effects on OXT
Besides the effects of caregiving on the HPA axis, effects on OXT sensitivity also contribute to variation in later life caregiving behavior, as evidenced by rodent studies and preliminary studies in humans. In infants of rodent mothers who provided more care, there is increased expression of (and stronger binding to) OXTR in limbic regions such as the amygdala and hypothalamus, and these infants also show increased maternal care later in life (Curley et al., Reference Curley, Jensen, Mashoodh and Champagne2011; Meaney, Reference Meaney2001). Decreased care, maternal separation, and a poor social environment have opposite effects on receptor expression and maternal behavior (Curley et al., Reference Curley, Jensen, Mashoodh and Champagne2011; Meaney, Reference Meaney2001). Although not yet systematically studied in humans, there are several recent studies showing that harsh circumstances in early life result in altered sensitivity to OXT. First, several studies show altered OXT levels and responses in people subjected to harsh caregiving and neglect. Second, OXT administration studies have revealed that experienced early life caregiving important modulates the effects of OXT on social–emotional behavior.
The relation between basal levels and central and peripheral release of OXT and AVP is unclear, especially because these neuropeptides cannot easily cross the blood–brain barrier, and central and peripheral levels are not always correlated (Landgraf & Neumann, Reference Landgraf and Neumann2004). In this light, studies investigating OXT levels taken from saliva and blood need to be interpreted with caution. One of the first investigations in the relation between harsh childhood and OXT levels measured from the cerebrospinal fluid showed that adult women subjected to increased exposure to childhood abuse and neglect had lower levels of OXT in the central nervous system (Heim et al., Reference Heim, Young, Newport, Mletzko, Miller and Nemeroff2009). In young children (~4 years of age) who were exposed to severe neglect by being subjected to institutionalized care as orphans, lowered basal AVP, but no lower OXT, levels were found. However, while OXT levels of control children increased relative to baseline after physical interaction with their mother, for children exposed to neglect, no such increase was observed after interaction with their adoptive mother (Fries, Ziegler, Kurian, Jacoris, & Pollak, Reference Fries, Ziegler, Kurian, Jacoris and Pollak2005). This alteration in OXT might result in later life psychopathology, as demonstrated by a study in which infants of depressed mothers were investigated (Apter-Levy et al., Reference Apter-Levy, Feldman, Vakart, Ebstein and Feldman2013). Both mothers and infant had lower levels of OXT, and these children also displayed impaired social behavior and had a higher incidence of psychopathology at 6 years of age. In healthy mothers, attachment security is related to higher levels of OXT, and this correlates with reward-related neural activation when exposed to images of their own children (Strathearn et al., Reference Strathearn, Fonagy, Amico and Montague2009). A study that investigated stress-elicited levels of OXT in adults showed that after social stress, the highest levels of OXT were observed in those securely attached compared to insecurely attached adults (Pierrehumbert, Torrisi, Ansermet, Borghini, & Halfon, Reference Pierrehumbert, Torrisi, Ansermet, Borghini and Halfon2012). Seemingly in contrast to these studies, which all point in the direction of lower basal and elicited OXT levels in maltreated in insecurely attached people, opposite effects have been reported.
In a study by Bhandari, Bakermans-Kranenburg, et al. (Reference Bhandari, Bakermans-Kranenburg, van der Veen, Parsons, Young, Grewen and van IJzendoorn2014) lower levels of self-reported harsh parenting were correlated with higher OXT levels, which further predicted more positive evaluations of happy infant expression. Higher levels of OXT were also observed during acute social stress in victims of sexual abuse compared to controls, although this level dropped to a level below that of the controls after the stress induction (Pierrehumbert et al., Reference Pierrehumbert, Torrisi, Laufer, Halfon, Ansermet and Popovic2010). Finally, one study that investigated the effect of social stress in maltreated young girls showed increased OXT after the stress induction, an effect that was not seen in boys or control children (Seltzer, Ziegler, Connolly, Prososki, & Pollak, Reference Seltzer, Ziegler, Connolly, Prososki and Pollak2014). These girls however also showed flattened CORT levels in response to stress compared controls, and this effect might thus demonstrate adaptive regulation of the stress response after repeated early life stress, comparable to the effects on the HPA axis described above. Although the few studies on stress-elicited OXT are far from conclusive, OXT responses after stress likely serve a different function than OXT responses elicited by social interaction, and therefore stress-induced OXT levels might also be differently affected by inadequate parenting.
A different series of studies investigated effects of OXT administration in relation to harsh caregiving and attachment, in line with the current view that administration effects are highly dependent on personal and contextual factors (Bakermans-Kranenburg & van IJzendoorn, Reference Bakermans-Kranenburg and van IJzendoorn2013; Bartz, Zaki, et al., Reference Bartz, Zaki, Bolger and Ochsner2011). In a first pilot study that indicated reduced sensitivity to OXT after neglect, adult males exposed to early parental separation had attenuated decreases in CORT after OXT administration (Meinlschmidt & Heim, Reference Meinlschmidt and Heim2007). A similar attenuated downregulation of CORT after OXT administration was seen in subjects with high scores on self-reported early life neglect and maltreatment compared to controls, although in this study CORT was measured in response to social stress (Grimm et al., Reference Grimm, Pestke, Feeser, Aust, Weigand, Wang and Bajbouj2014). Accompanied functional magnetic resonance imaging measurements in this study also showed opposite neural effects during a stressful task in subjects exposed to harsh conditions after OXT; whereas OXT attenuated task-related effects in controls, it strengthened these effects in the subjects with self-reported early life neglect and maltreatment. Additional analyses showed that the level of emotional abuse was related to increased amygdala–hippocampal connectivity during the stressful task, which was attenuated after OXT (Fan et al., Reference Fan, Pestke, Feeser, Aust, Pruessner, Boker and Grimm2015). In the OXT condition, amygdala–hippocampal connectivity was negatively associated with CORT responses, providing a possible pathway by which altered OXT sensitivity at the levels of the amygdala could lead to up- or downregulation of the HPA axis by acting on the hippocampus. Another imaging study also revealed altered resting-state connectivity of the amygdala to the anterior cingulate cortex (ACC; part of the PFC) in subjects scoring high on self-reported early life neglect and maltreatment (Fan et al., Reference Fan, Herrera-Melendez, Pestke, Feeser, Aust, Otte and Grimm2014). In controls, this amygdala–ACC connectivity predicted ACC responses to stress, and this relation was attenuated after OXT administration, but in high scoring subjects, no such relation was observed. Thus, subjects exposed to harsh caregiving might be less sensitive to the positive effects that OXT has on amygdala–PFC connectivity (Sripada et al., Reference Sripada, Phan, Labuschagne, Welsh, Nathan and Wood2013), which is a critical circuitry in the regulation of emotional responses (Kim et al., Reference Kim, Loucks, Palmer, Brown, Solomon, Marchante and Whalen2011). High levels of self-reported maternal love withdrawal also reduced the effect of OXT on resting-state functional connectivity in the default mode network (Riem, van IJzendoorn, et al., Reference Riem, van IJzendoorn, Tops, Boksem, Rombouts and Bakermans-Kranenburg2013). This same measure of self-reported love withdrawal also attenuated OXT-induced increases in EEG responses to emotional faces (Huffmeijer et al., Reference Huffmeijer, Alink, Tops, Grewen, Light, Bakermans-Kranenburg and van IJzendoorn2013).
Altered responses after OXT administration dependent on harsh caregiving have also been observed in social–emotional behavioral paradigms. In a study testing the effect of emotion recognition, OXT improved recognition of avoidant emotional expression in subjects scoring low on experienced harsh caregiving, but not in high scoring subjects (Feeser et al., Reference Feeser, Fan, Weigand, Hahn, Gärtner, Aust and Grimm2014). Higher levels of maternal love withdrawal also attenuated the effects of OXT administration on charitable donations (van IJzendoorn, Huffmeijer, Alink, Bakermans-Kranenburg, & Tops, Reference van IJzendoorn, Huffmeijer, Alink, Bakermans-Kranenburg and Tops2011) and on compensatory behavior for ostracism of an excluded other player in the cyberball task (Riem, Bakermans-Kranenburg, Huffmeijer, & van IJzendoorn, Reference Riem, Bakermans-Kranenburg, Huffmeijer and van IJzendoorn2013). Whereas OXT led to higher donations to a charity and to increased ball throwing to an excluded other, these effects were only observed in those individuals with low levels of maternal love withdrawal. Directly investigating the effect on caregiving behavior, another study found that OXT reduced negative physical responses (hand squeezing) to infant cries, but this effects was not found in women subjected to harsh caregiving, again pointing at reduced sensitivity to OXT (Bakermans-Kranenburg, van IJzendoorn, Riem, Tops, & Alink, Reference Bakermans-Kranenburg, van IJzendoorn, Riem, Tops and Alink2012). A comparable effect was found in a baby reward task in which participants learn to dissociate happy and unhappy baby pictures based on previous information about the babies’ temperament. The data showed that after OXT administration, participants reporting more childhood emotional maltreatment performed less accurately (Bhandari, van der Veen, et al., Reference Bhandari, van der Veen, Parsons, Young, Voorthuis, Bakermans-Kranenburg and van IJzendoorn2014).
Besides effects of experienced harsh caregiving, also adult attachment style is a predictive factor for the behavioral effects of OXT administration. Whereas individuals scoring low on self-reported anxious attachment reported their mother as being more close and caring after OXT administration, individuals high on anxious attachment reported their mother as less caring after OXT (Bartz et al., Reference Bartz, Zaki, Ochsner, Bolger, Kolevzon, Ludwig and Lydon2010). In individuals with BPD, OXT administration has opposite effects on trust compared to effects observed in healthy subjects, effects that seemed driven by self-reported anxious attachment (Bartz, Simeon, et al., Reference Bartz, Simeon, Hamilton, Kim, Crystal, Braun and Hollander2011). A recent study replicated this trust-reducing effect, whereas in this study the effect was predicted by childhood neglect (Ebert et al., Reference Ebert, Kolb, Heller, Edel, Roser and Brüne2013), indicating that in BPD both childhood neglect and anxious attachment can contribute to impairment of social behavior after OXT. However, the modulating role of attachment in OXT effects is not straightforward, as shown by a study on healthy subjects by De Dreu (Reference De Dreu2012) in which specifically those subjects scoring high on attachment avoidance show increased trust and cooperation after OXT administration. Adding to the complexity of the relation between self-report measures of attachment and effects induced by OXT is a study in which OXT was administered to individuals with social anxiety disorder (Fang, Hoge, Heinrichs, & Hofmann, Reference Fang, Hoge, Heinrichs and Hofmann2014). These data show that in these diagnosed individuals, high attachment avoidance predicted faster detection of neutral and disgust faces after OXT, while OXT increased social interaction after exclusion only in low attachment avoidant individuals. Thus, from these studies it appears that attachment anxiety and avoidance can have different effects on OXT sensitivity, which depend on the task demands. However, a difficulty in the interpretation of these data is that in these studies attachment was measured from self-report as compared to the highly validated Adult Attachment Interview (AAI), which is a highly reliable predictor for insensitive parenting (Belsky, Reference Belsky, Carter, Ahnert, Grossmann, Hrdy, Lamb, Porges and Sachser2005; van IJzendoorn, Reference van IJzendoorn1995). Meta-analytic data show that outcomes of the two measures show only trivial to small overlap and thus measure different underlying constructs (Roisman et al., Reference Roisman, Holland, Fortuna, Fraley, Clausell and Clarke2007). It is suggested that whereas the AAI measures attachment as rooted in early parent–infant interaction, self-reported measures reflect more current romantic attachment and personality type (Roisman et al., Reference Roisman, Holland, Fortuna, Fraley, Clausell and Clarke2007; van IJzendoorn & Bakermans-Kranenburg, Reference van IJzendoorn and Bakermans-Kranenburg2010). A possible explanation for the differential effects of OXT on self-reported attachment might thus be that these effects reflect modulating effects of personality in addition to early life parenting experiences, which is in line with other conceptualizations on the role of OXT in social–emotional behavior (Bakermans-Kranenburg & van IJzendoorn, Reference Bakermans-Kranenburg and van IJzendoorn2013; Bartz, Zaki, et al., Reference Bartz, Zaki, Bolger and Ochsner2011). Even in this light, the data on self-reported attachment are of great relevance. Although less thoroughly investigated compared to the AAI, self-reported attachment questionnaires have proven predictive for diverse parental thoughts, emotions, and behaviors (Jones et al., Reference Jones, Cassidy and Shaver2015). Thus, whether the modulating effect of self-reported attachment on OXT administration truly reflects variation in attachment as derived from the individuals’ early life experience with their parents, or reflects the modulating effect of personality factors, needs to be tested in future studies. Because only a few studies have tested the modulatory effect of adult attachment so far, and because several of the studies were performed in patients groups not easily compared to healthy adults, it is too early to make generalizations regarding these effects.
In sum, although the literature investigating effects of experienced harsh caregiving on sensitivity to OXT consist of only a modest number of studies, so far it reveals a more consistent picture of reduced neural sensitivity after experienced harsh parenting and reduced sensitivity for the effects of OXT on behavior relating to sensitive parenting.
Effects on other candidate hormones and neuropeptides
Work in animals revealed several other endocrine factors involved in parenting of which sensitivity is shaped by experienced caregiving, such as AVP, testosterone, estradiol, and endogenous opioids (Curley, Reference Curley2011; Curley et al., Reference Curley, Jensen, Mashoodh and Champagne2011). For example, variation in maternal care or maternal separation can alter ER, AR, and AVP receptor expression in regions such as the amygdala, hypothalamus, and hippocampus (Curley et al., Reference Curley, Jensen, Mashoodh and Champagne2011; Kaiser, Kruijver, Swaab, & Sachser, Reference Kaiser, Kruijver, Swaab and Sachser2003), affecting caregiving behavior later in life. Although these data warrant investigations on the role of AVP, estradiol, and opioids in humans, especially in relation to experienced caregiving, data is scarce if not absent. One study that was described above showed decreased AVP levels in severely neglected children (Fries et al., Reference Fries, Ziegler, Kurian, Jacoris and Pollak2005), yet to my knowledge, that is the only study investigating this relation so far. I am also unaware of data showing relations of estradiol and testosterone function and early life caregiving in humans. However, there is some preliminary evidence suggesting a link between sensitivity to opioids and human caregiving. A study investigating genetic variation of the OPRM1 shows that only in subjects homozygous for the A allele of the A118G polymorphism are recollections of maternal care predictive for fearful attachment (Troisi et al., Reference Troisi, Frazzetto, Carola, Di Lorenzo, Coviello, Siracusano and Gross2012). Furthermore, of two studies using positron emission tomography, one demonstrated that in people with more attachment avoidance, there is less availability of opioid receptors in the amygdala, insula, ACC, and OFC (Nummenmaa et al., Reference Nummenmaa, Manninen, Tuominen, Hirvonen, Kalliokoski, Nuutila and Sams2015), regions critically involved in empathic behavior and sensitivity to infant stimuli (Rilling, Reference Rilling2013). A second study showed that in people exposed to stressful experiences, there is lower receptor availability in most of the regions listed above, yet more receptor availability in the OFC (Liberzon et al., Reference Liberzon, Taylor, Phan, Britton, Fig, Bueller and Zubieta2007). This indicates that opioid receptor availability changes dynamically depending on environmental factors, and that this altered availability relates to social bonding in adulthood. Thus, harsh caregiving could potentially alter neural availability of opioid receptors, thereby affecting later life caregiving behavior similar to the above described effects of harsh caregiving on OXT and CORT. Moreover, based on the study by Troisi et al. (Reference Troisi, Frazzetto, Carola, Di Lorenzo, Coviello, Siracusano and Gross2012) described above, these proposed effects might depend on the OPRM1 polymorphism. Testing this proposed mechanisms whereby altered opioid function can affect caregiving later in life is only one of the ample number of targets for future studies that can be derived from the promising body of research presented in this review.
Puberty, adolescence, and young adulthood
Of final importance is the period between early childhood and adulthood, with early adulthood being the phase when most individuals make the transition to parenthood. With respect to endocrine variability, during puberty and adolescence hormonal factors have strong organizational effects on the human brain (Crone & Dahl, Reference Crone and Dahl2012; Peper, Rol, Crone, & van Hook, Reference Peper, Pol, Crone and van Honk2011). Work in other animals has brought forth adolescence as an additional sensitive period in which behavioral profiles are shaped, because environmental circumstances during this period can alter neuroendocrine functions and later life social–emotional behavior, such as adult social bonding (Sachser, Kaiser, & Hennessy, Reference Sachser, Kaiser and Hennessy2013). In humans, where adolescence is considered critical for the development of cognitive control, emotion regulation, and social competence, this developmental phase is characterized by changes in endocrine function, including vast increases in levels of steroid hormones such as estradiol and testosterone (Crone & Dahl, Reference Crone and Dahl2012). Neuroimaging studies have attempted to shed light on the functional consequences of these endocrine changes for neural development and for social–emotional behavior. Data of these studies show that testosterone levels in both adolescent boys and girls are positively related to neural responses in reward processing (de Macks et al., Reference de Macks, Moor, Overgaauw, Güroğlu, Dahl and Crone2011) and that adolescents’ testosterone levels are also positively associated with increased risk taking (Peper, Koolschijn, & Crone, Reference Peper, Koolschijn and Crone2013). In boys this association between testosterone and risk taking was potentiated by smaller OFC volumes, which might explain impaired emotion regulation. Testosterone levels in both adolescent boys and girls were also related to lower quality of the structural connections between subcortical regions and the frontal and temporal cortices (Peper, de Reus, van den Heuvel, & Schutter, Reference Peper, de Reus, van den Heuvel and Schutter2015). The quality of these connections was further inversely related to increased expressive forms of aggression, indicating the relevance of increases of steroid hormones, and especially testosterone, for social–emotional behavior in this developmental phase. In addition, in young adults, quality of the structural connectivity between the frontal cortex and the striatum predicted lower levels of impulse control and higher levels of testosterone (Peper, Mandl, et al., Reference Peper, Mandl, Braams, de Water, Heijboer, Koolschijn and Crone2013). Taken together, these studies indicate that higher levels of especially testosterone during adolescence and early adulthood might predispose to more reward-seeking behavioral profiles characterized by less impulse control and higher levels of aggression, which could have negative effects on caregiving behavior. However, the direct relation between endocrine variation in adolescence and early adulthood and later life caregiving is currently unexplored.
Research on animals has also demonstrated that social environmental factors interact with endocrine function during adolescence. For example, the number of competitive interactions in male rodents leads to changes in reproduction patterns under the influence of altered testosterone levels and function of the HPA axis (Sachser, Reference Sachser, Carter, Ahnert, Grossmann, Hrdy, Lamb, Porges and Sachser2005; Sachser et al., Reference Sachser, Kaiser and Hennessy2013). In humans, a comparable mechanism has been proposed, in that environmental factors that indicate instability interact with the endocrine stress system to accelerate the onset of puberty in girls (Ellis, Reference Ellis2004). As such, in a stressful environment, evolutionary pressure might stimulate higher fecundity at the cost of less investment in offspring (Trivers, Reference Trivers1972). Early life stress in humans has been shown to result in more adultlike hormonal patterns earlier in life compared to controls, and this effect was specific for girls (Ruttle, Shirtcliff, Armstrong, Klein, & Essex, Reference Ruttle, Shirtcliff, Armstrong, Klein and Essex2015). Effects of early life stress on the adolescent endocrine system were further demonstrated in a study that found flattened CORT levels, indicative of reduced sensitivity to CORT, in girls who were exposed to inconsistent discipline by their mother (Martin, Kim, & Fisher, Reference Martin, Kim and Fisher2016). Thus, during adolescence, characterized by the organizational effects of steroid hormones on the neuroendocrine systems involved in caregiving behavior, environmental factors can alter the developmental trajectories, which makes this developmental phase a fruitful direction for further research.
Endocrine Profiles of Caregiving Behavior
Although research on the endocrinology of human caregiving is still in its infancy, the studies reviewed above show that endocrine factors can play an important role in human caregiving, and that genetic makeup, together with the fetal and early life environment, contribute to variability in endocrine sensitivity. This variability can lead to endocrine profiles that are, depending on the environment, more or less optimal for providing infants with care. In addition, the effects of experienced parenting on endocrine sensitivity provide an endocrine model for the intergenerational transmission of human caregiving, similar to the rodent models for transmission of maternal caregiving (Champagne, Reference Champagne2011; Curley et al., Reference Curley, Jensen, Mashoodh and Champagne2011; Meaney, Reference Meaney2001). In the current section, these more and less optimal endocrine profiles are described. Based on these profiles, an endocrine model for the intergenerational transmission of human caregiving is described in the next section, which can serve as a framework to aid future research.
Of all the sources of variability in endocrine sensitivity, genetic variation of endocrine receptors constitutes the primary ontogenetic factor. In relation to human caregiving, the relevant steroid and neuropeptide receptors of which studies have shown functional significance are listed in Figure 2. However, because the explanatory value of genetic polymorphisms for direct behavioral outcomes is particularly small (Bakermans-Kranenburg & van IJzendoorn, Reference Bakermans-Kranenburg and van IJzendoorn2014; Chabris et al., Reference Chabris, Hebert, Benjamin, Beauchamp, Cesarini, van der Loos and Laibson2012), most promising are approaches that do not investigate the polymorphisms in isolation but include intermediate endophenotypical expression of genetic variability, such as neural sensitivity to hormonal administration (Marsh et al., Reference Marsh, Henry, Pine, Gorodetsky, Goldman and Blair2012). Of equal interest is research that focuses on the interaction between genetic and environmental variation with regard to caregiving behavior (Mileva-Seitz, Bakermans-Kranenburg, & van IJzendoorn, Reference Mileva-Seitz, Bakermans-Kranenburg and van IJzendoorn2016). Together, knowledge of the functional role of polymorphic variation, and how this variation results in different effects depending on environmental circumstances, can yield critical insight into how and under which conditions genetic variability results in altered caregiving.

Figure 2. (Color online) Overview of the sources of variability in endocrine systems related to parenting, and a model for the intergenerational transmission of sensitive or harsh caregiving. Whereas sensitive caregiving will alter neural sensitivity to oxytocin and cortisol leading to more sensitive caregiving practices, harsh caregiving will have an opposite effect. Adolescence can add additional variation to caregiving behavior by altering levels of testosterone.
The first environmental effects on an individuals’ endocrine development are established in the womb, as during pregnancy both fetal testosterone and maternal OXT have proven to be relevant for the quality of later life caregiving. Based on currently available literature, exposure to more fetal testosterone can be expected to result in increased sensitivity to testosterone later in life (van Honk et al., Reference van Honk, Schutter, Bos, Kruijt, Lentjes and Baron-Cohen2011) and more male-typical brain and behavior (Lombardo, Ashwin, Auyueng, Chakrabarti, Lai, et al., Reference Lombardo, Ashwin, Auyeung, Chakrabarti, Lai, Taylor and Baron-Cohen2012; Lombardo, Ashwin, Auyueng, Chakrabarti, Taylor, et al., Reference Lombardo, Ashwin, Auyeung, Chakrabarti, Taylor, Hackett and Baron-Cohen2012), as well as a higher incidence of autism (Baron-Cohen et al., Reference Baron-Cohen, Auyeung, Nørgaard-Pedersen, Hougaard, Abdallah, Melgaard and Lombardo2015). Considering the downregulating effects of testosterone on empathic abilities and regulation of emotionally reactive behavior (Bos, Panksepp, et al., Reference Bos, Panksepp, Bluthe and Honk2012), high fetal testosterone might negatively affect sensitive parenting later in life. Unfortunately, studies directly investigating the relation between fetal testosterone or testosterone levels in mothers during pregnancy and parent–infant interaction have not been performed so far. However, studies on maternal OXT show positive effects of higher basal levels during pregnancy on caregiving behavior after birth, such as increased maternal bonding (Feldman et al., Reference Feldman, Weller, Zagoory-Sharon and Levine2007). Thus, based on the currently available studies in humans, especially testosterone and OXT are important for caregiving behavior: OXT by facilitating the bond between parent and infant directly after parturition and testosterone by increasing sensitivity to the effects of testosterone on empathic abilities later in life.
Although a lack of experimental work in humans warrants caution; the behavioral variation around parturition is likely to have long-term effects on caregiving by either directly or indirectly interfering with release of OXT, CORT, AVP, and noradrenaline (Olza-Fernández et al., Reference Olza-Fernández, Gabriel, Gil-Sanchez, Garcia-Segura and Arevalo2014). Mode of delivery, mother–infant separation after birth, and bottle-feeding all have substantial impact on maternal and infant OXT and CORT levels, while infant OXT has been shown to predict social engagement (Clark et al., Reference Clark, John, Pasca, Hyde, Hornbeak, Abramova and Penn2013), and maternal CORT predicts maternal sensitivity, affection, and recognition (Fleming, Ruble, et al., Reference Fleming, Ruble, Krieger and Wong1997; Fleming, Steiner, et al., Reference Fleming, Steiner and Corter1997; Stallings et al., Reference Stallings, Fleming, Corter, Worthman and Steiner2001). Thus, after only a few days, many important neuroendocrine prerequisites for the establishment of the bond between mother and infant, but also for the infants’ social bonds later in life, are laid down. In addition, although some of these effects might prove rather subtle, infants exposed to high fetal testosterone and low maternal OXT, who after CS are immediately separated from their mother and refrained from breastfeeding, will not profit from the stress-reducing effects of mutually increasing levels of OXT, and possibly opioids. Such children might follow a different trajectory compared to others, in that the altered endocrine and behavioral synchronicity of parent and infant in this sensitive developmental period could hamper the onset of parent–infant attachment directly after birth (Feldman, Reference Feldman2015; Olza-Fernández et al., Reference Olza-Fernández, Gabriel, Gil-Sanchez, Garcia-Segura and Arevalo2014).
During early childhood, experienced caregiving impacts neuroendocrine development, as evidenced by several studies in which harsh parenting was shown to result in endocrine abnormalities. Overall, these studies indicate that harsh parenting such as abuse and neglect reduces OXT levels, as well sensitivity to OXT (see above, e.g., Bakermans-Kranenburg et al., Reference Bakermans-Kranenburg, van IJzendoorn, Riem, Tops and Alink2012; Fries et al., Reference Fries, Ziegler, Kurian, Jacoris and Pollak2005). Results for the HPA axis indicate a more complex interaction with harsh caregiving, but most studies are consistent with the idea that moderate levels of harsh caregiving increase sensitivity to CORT, whereas enduring neglect or abuse result in blunted sensitivity to CORT. The relation between harsh caregiving and CORT sensitivity can be proposed as an inverted U-shape, where moderate levels of harsh caregiving sensitize the hippocampus by epigenetic upregulation of CORT receptors, leading to adaptive downregulation of the HPA axis after stress (Bos et al., Reference Bos, Montoya, Terburg and van Honk2014). Chronic and severe neglect and abuse might then result in insensitivity of the hippocampus to CORT due to the neurotoxic effects of chronically high CORT (Lupien et al., Reference Lupien, McEwen, Gunnar and Heim2009; McEwen, Reference McEwen2010).
In relation to the role of hormones and neuropeptides in human caregiving, and based on the factors determining endocrine sensitivity (summarized in Figure 2), endocrine profiles for variation in caregiving behavior can be proposed. Overall, high levels of sensitivity to OXT, opioids, and PRL, together with moderate levels of sensitivity to CORT and testosterone, will serve sensitive caregiving practices, as high sensitivity to OXT, opioids, and PRL will generally result in high sensitivity and empathic responding to infant stimuli, as well as high levels of emotional regulation and initiation of caregiving behavior. Moreover, higher sensitivity to these hormonal factors can facilitate biobehavioral synchrony between caregiver and infant, the mechanism by which mutually dependent attachment relations are formed by synchronizing social interaction (Feldman, Reference Feldman2015). Moderate levels of sensitivity to testosterone will aid rewarding responses to own-infant stimuli, and also adaptive responding to the infants’ signals of stress such as crying. It will furthermore facilitate protective behavior in case of threat and emotionally reactive behavior in challenging situations. Against a background of declining levels of testosterone in the transition to parenthood, moderate but not low sensitivity might be especially relevant. Similarly, moderate levels of sensitivity to CORT might prove optimal for caregiving behavior, as responsiveness to CORT can facilitate arousal-dependent initiation of caregiving behavior.
In contrast, low sensitivity to OXT, opioids, and PRL, combined with high sensitivity to testosterone and either low or high levels of sensitivity to CORT, can predispose to insensitive caregiving practices. Low sensitivity to OXT, opioids, and PRL will decrease the likelihood of individuals benefiting from the positive effects of these hormones and will additionally hamper the mutually rewarding effect of synchrony in social interaction, which is important for attachment formation in both caregiver and infant (Feldman, Reference Feldman2015). Furthermore, high levels of sensitivity to testosterone will result in a behavioral profile which conflicts with sensitive caregiving. High sensitivity to testosterone will result in more dominant behavior, increased reward seeking, reduced emotion regulation, and reduced cognitive empathic abilities (Baron-Cohen, Reference Baron-Cohen2009; Dabbs & Dabbs, Reference Dabbs and Dabbs2000). For CORT, both low and high levels of sensitivity might reduce sensitive caregiving, as low sensitivity to CORT not only reduces responsiveness to initiate care but also prohibits the restoration of emotional reactivity in the aftermath of stress. In addition, low sensitivity to CORT of brain regions that are involved in feedback to the HPA axis, such as the hippocampus, can lead to chronically high baselines of CORT with potential neurotoxic effects. Conversely, high sensitivity to CORT might serve reactive stress regulation, but will also reduce reward sensitivity (Montoya, Bos, Terburg, Rosenberger, & van Hook, Reference Montoya, Bos, Terburg, Rosenberger and van Honk2014). As such, depending on the environmental circumstances, high or low endocrine sensitivity can be more or less optimal. Whereas high sensitivity to CORT, as well as to testosterone, might be beneficial in an unsafe and threatening environment, in a safer environment such high sensitivity to CORT can lead to downregulation of the HPA axis, perhaps similar to the flat hormonal curves and low baselines observed in children after experienced harsh parenting.
Thus, whereas some aspects of caregiving are important under all conditions, different caregiving practices might be required in unstable and unsafe versus stable and safe environments, and so are the role of endocrine factors underlying such behavior. An organism whose endocrine profile is adaptive in unsafe and threatening situations, but who lives under relatively safe conditions will experience a mismatch between environment and internal physiology, as can be the case in children who have undergone adaptive endocrine changes in early life that persist in adulthood. Overall, the idea that the above described endocrine profiles are important in caregiving behavior, and that these profiles are affected by experienced caregiving, forms the basis of the model for intergenerational transmission of caregiving behavior described in the following section.
Endocrine Model for Intergenerational Transmission of Caregiving
The notion of transmission of caregiving behavior and attachment bonds has received ample support (Bailey et al., Reference Bailey, Hill, Oesterle and Hawkins2009; Belsky, Reference Belsky, Carter, Ahnert, Grossmann, Hrdy, Lamb, Porges and Sachser2005; Milner, Reference Milner2003; van IJzendoorn, Reference van IJzendoorn1995), but the mechanisms underlying this transmission are still unclear. Learning by interaction and observation, and the transition of beliefs about caregiving from parents to children, undoubtedly plays a major role, as already described by Bowlby when discussing the intergenerational transmission of internal working models (Bowlby, Reference Bowlby1983; Bretherton & Munholland, Reference Bretherton, Munholland, Cassidy and Shaver2008). Based on the idea that children develop different cognitive models depending on the type of caregiving they receive, investigations on the mechanisms underlying intergenerational transmission of caregiving have described the heterogeneity in the types of maltreatment, and its underlying factors, that are transmitted from parents to children (Berzenski, Yates, & Egeland, Reference Berzenski, Yates, Egeland, Korbin and Krugman2014). As also described by Bowlby (Reference Bowlby1983), the internal working models of attachment behavior are embedded in a brain of which the prime function is to form predictions about the environment. This process depends on a rich set of brain structures, such as the amygdala and hippocampus, involved in the detection of salient information and memory formation, and which are important in caregiving behavior (Rilling, Reference Rilling2013). These structures are furthermore densely distributed with endocrine receptors, and altered endocrine sensitivity of these structures depending on experienced caregiving can thus lead to changes in caregiving behavior, and alter the formation of cognitive models and beliefs about interpersonal relations. Animal models show that such altered neuroendocrine sensitivity underlies the transmission of maternal care. Research in rodents shows that altered expression of OXTR in limbic regions, including the amygdala and hypothalamus, and of CORT receptors in the hippocampus are critical for intergenerational transmission of maternal behavior (Curley et al., Reference Curley, Jensen, Mashoodh and Champagne2011; Meaney, Reference Meaney2001). Thus, in rodents, being subjected to poor maternal care results in altered sensitivity to OXT and CORT, leading to poor maternal behavior in offspring. The data on humans discussed in this review suggest a similar role of altered neuroendocrine sensitivity in the transmission of caregiving behavior and specifically altered sensitivity to OXT and CORT.
The endocrine profiles leading to sensitive or insensitive caregiving behavior as described above are in part shaped by experienced caregiving during early childhood. As depicted in the lower panel of Figure 2, insensitive caregiving can reduce neural and behavioral sensitivity to OXT and result in both increased and reduced sensitivity to CORT. This altered sensitivity may render individuals less responsive to OXT surges when providing care later in life, possibly leading to lower levels of empathy, social sensitivity, and emotional regulation. Either heightened or reduced sensitivity to CORT can result in disturbed responsiveness to initiate care and maladaptive responses to stress. Thus, experienced caregiving alters neuroendocrine sensitivity to OXT and CORT, leading to less sensitive caregiving practices and thereby exposing the next generation to insensitive caregiving. A possible biological mechanism that underlies altered neuroendocrine sensitivity is epigenetic change in the genes promoting the expression of OXT and CORT receptors at the level of the brain (Curley et al., Reference Curley, Jensen, Mashoodh and Champagne2011). Target brain regions for epigenetic alterations of OXT are the amygdala, the ventral striatum, and the ACC, whereas for CORT the amygdala and hippocampus are most relevant. Increased sensitivity of the ventral striatum and amygdala to OXT can facilitate sensitive caregiving behavior by increasing reward sensitivity of the striatum and by shifting connectivity of the amygdala from the brainstem toward the PFC and ACC (Riem, Bakermans-Kranenburg, et al., Reference Riem, Bakermans-Kranenburg, Pieper, Tops, Boksem, Vermeiren and Rombouts2011; Sripada et al., Reference Sripada, Phan, Labuschagne, Welsh, Nathan and Wood2013; Strathearn et al., Reference Strathearn, Fonagy, Amico and Montague2009), suppressing impulsive and aggressive responses and enabling empathic behavior and emotion regulation (Bos, Panksepp, et al., Reference Bos, Panksepp, Bluthe and Honk2012; Kirsch et al., Reference Kirsch, Esslinger, Chen, Mier, Lis, Siddhanti and Meyer-Lindenberg2005). Sensitivity of the amygdala and hippocampus to CORT can support emotional memory formation, reactive responding to infant distress, and adaptive regulation of the HPA axis (Bos et al., Reference Bos, Montoya, Terburg and van Honk2014; Joëls, Pu, Wiegert, Oitzl, & Krugers, Reference Joëls, Pu, Wiegert, Oitzl and Krugers2006). In sum, insensitive caregiving can thus lead to epigenetic alterations of the genes promoting expression of OXT and CORT receptors in brain regions critical for caregiving behavior in children, thereby altering the child's sensitivity of these brain regions to OXT and CORT, resulting in impairments in social–emotional behavior relevant to caregiving. After early childhood, the period of adolescence can further shape the neuroendocrine profiles predictive of (in)sensitive caregiving, as harsh environmental circumstances can accelerate the onset of puberty exposing the brain to earlier surges of testosterone, which in adolescence are related to impaired inhibition and impulse control. In adulthood, these impairments can increase the chance that these individuals will show insensitive caregiving when they have made the transition to parenthood (see Figure 2).
Although the data discussed in this review support the view that neural and behavioral sensitivity to endocrine factors relevant for caregiving behavior are under influence of caregiving experiences, whether this exact mechanism underlies the intergenerational transmission of caregiving behavior has not yet been empirically tested in humans. Preliminary studies do however show promising results. Besides the study discussed above, which shows that OXT only reduced negative physical responses to infants in people that were not subjected to harsh caregiving (Bakermans-Kranenburg et al., Reference Bakermans-Kranenburg, van IJzendoorn, Riem, Tops and Alink2012), another study also showed that women who were insecurely attached have lower levels of maternal OXT during pregnancy (Samuel et al., Reference Samuel, Hayton, Gold, Feeley, Carter and Zelkowitz2015). In addition, a study that focused on intergenerational transmission of depression showed that subjects exposed to maternal depression in their youth and who carry an A allele of the OXTR rs53576 polymorphism are more likely to have depressive symptoms in adolescence compared to GG carriers (Thompson, Hammen, Starr, & Najman, Reference Thompson, Hammen, Starr and Najman2014), the variant whose carriers seem to be more sensitive to OXT (Marsh et al., Reference Marsh, Henry, Pine, Gorodetsky, Goldman and Blair2012). With regard to CORT, stronger diurnal CORT responses (indicating blunted sensitivity) in mothers exposed to maltreatment predict less sensitive parenting behavior toward their own children (Gonzalez et al., Reference Gonzalez, Jenkins, Steiner and Fleming2012). Furthermore, high levels of maternal depression during pregnancy predict higher CORT responses in 3-month-old babies during a challenging interaction with their mother (Luecken, MacKinnon, Jewell, Crnic, & Gonzalez, Reference Luecken, MacKinnon, Jewell, Crnic and Gonzales2015), an effect that was specific to babies who were categorized by their mothers as having a difficult temperament. This effect might be due to lower synchronous levels of OXT in mothers and infants exposed to maternal depression, leading to less efficient downregulation of the CORT response (Pratt et al., Reference Pratt, Apter-Levi, Vakart, Feldman, Fishman, Feldman and Feldman2015). Although more conclusive evidence is needed, these findings do point at involvement of OXT and CORT in the intergenerational transmission of caregiving. Conclusive evidence should come from future longitudinal studies that investigate how neural and behavioral sensitivity to OXT and CORT in children and young adults translate to parenting behavior later in their lives.
The relative simplicity of the currently presented model stands in stark contrast to the true complexity of the neurobiological systems that bring forth human caregiving behavior and how these neurobiological systems dynamically interact with environmental factors throughout development. Despite these complexities, recent advances in the application of structural equation modeling has led to a broad array of statistical models in which increasingly complex developmental questions can be addressed, such as panel analyses (Selig & Little, Reference Selig, Little, Laursen, Little and Card2012) and latent profile and developmental cascade models (Martin et al., Reference Martin, Kim and Fisher2016; Masten & Cicchetti, Reference Masten and Cicchetti2010). Models such as these can give unique insight into the mechanisms by which genetic variability can result in different endocrine profiles under different environmental circumstances, taking into account the dynamic interactions between these profiles and the environment throughout development and how these profiles ultimately lead to different types of caregiving behavior. A promising example is a study that investigated how phenotypes of maternal stress relate to, and interact with, parenting behaviors and diurnal cortisol rhythms in the children of these mothers (Martin et al., Reference Martin, Kim and Fisher2016). For now, a first step would be to empirically validate the model for intergenerational transmission as depicted in Figure 2. Critical for this validation would be to investigate whether experienced insensitive caregiving results in reduced sensitivity to OXT and altered sensitivity to CORT in young adults without children in brain regions relevant for caregiving. Combining hormone administration paradigms with brain imaging techniques provides an opportunity to test such relations (e.g., Bos et al., Reference Bos, Montoya, Terburg and van Honk2014; Grimm et al., Reference Grimm, Pestke, Feeser, Aust, Weigand, Wang and Bajbouj2014). Follow-up studies should investigate whether the altered endocrine sensitivities observed in the neuroimaging studies predict caregiving practices in these same individuals when they become parents and how these possible relations differ between males and females. Another research question of great relevance is how endocrine variation in adolescence interacts with environmental factors to predict caregiving behavior. For example, it is unknown whether earlier or higher testosterone surges in adolescence lead to higher incidence of insensitive caregiving in young parents. Because younger parenting has been implicated in the intergenerational transmission of maltreatment (Berzenski et al., Reference Berzenski, Yates, Egeland, Korbin and Krugman2014), higher testosterone levels or earlier onset of puberty could play a role and serve as a predictive marker. Studies on this topic have however not been performed so far and provide an exciting avenue for future research.
In addition, the proposed neuroendocrine transmission of caregiving may not be restricted to parenting behavior, but may also be reflected in adult attachment and partner bonding. The neuroendocrine circuitry that underlies human parenting behavior may also underlie other forms of social bonding, as throughout mammalian evolution this circuitry has adapted to facilitate increasingly diverse forms of social bonding (Nelson & Panksepp, Reference Nelson and Panksepp1998; Panksepp, Nelson, & Bekkedal, Reference Panksepp, Nelson and Bekkedal1997). Thus, our model for the intergenerational transmission of caregiving could extend to other forms of social bonding and for this reason can also be relevant to the literature on adolescent and adult attachment (Cassidy & Shaver, Reference Cassidy and Shaver2008).
Conclusion
The claim made in this review is that human caregiving behavior depends on hormones and neuropeptides (Figure 1) and that individual variation in sensitivity to these hormones and neuropeptides facilitates the intergenerational transmission of caregiving (Figure 2). The aim of the heuristic model is to aid future research by presenting a framework based on which new hypotheses can be formulated and tested. As such, it holds fundamental relevance by increasing our understanding of the neurobiological mechanisms that bring forth the human repertoire of caregiving behavior. The current model can further add to existing models of intergenerational transmission of caregiving. For example, Rilling and Young (Reference Rilling and Young2014) propose a model wherein higher levels of OXT contribute to transmission of secure attachment. The model presented here extends Rilling and Young's (Reference Rilling and Young2014) model in that not only baseline levels of hormones such as OXT are relevant but also that sensitivity of the relevant neural structures to these hormones might even be more important. In addition, the current model also highlights the role of the HPA axis in early childhood and the role of testosterone in puberty in transmission of caregiving. In addition to its theoretical value, the model might also have practical implications. By combining measures from genetics, neuroendocrinology, and clinical psychology, it will be possible to compose individually tailored biopsychosocial profiles that predict the risk for not only development of parental insensitivity but also later life psychopathology. This would allow the development of individually tailored interventions, altering the trajectory of the intergenerational transmission of caregiving toward more sensitive behavior.
There are several protective factors against intergenerational transmission of harsh caregiving, such as psychotherapy, the availability of a supportive adult other than the primary caregiver, and a supportive spouse (Belsky, Youngblade, & Pensky, Reference Belsky, Youngblade and Pensky1989). Especially when these factors are not available, interventions directed at increasing attachment and caregiving practices could be a valuable approach. Psychoeducational training and child–parent psychotherapy have been shown to increase attachment security (Berzenski et al., Reference Berzenski, Yates, Egeland, Korbin and Krugman2014; Cicchetti, Rogosch, & Toth, Reference Cicchetti, Rogosch and Toth2006). However, such approaches cannot prevent transmission in all cases, because some individuals are not responsive to therapy or training programs, perhaps due to endocrine insensitivity. Individuals less sensitive to natural fluctuations of OXT could be helped by the therapeutic use of intranasal OXT combined with psychotherapy or parent–infant training (van IJzendoorn & Bakermans-Kranenburg, Reference van IJzendoorn and Bakermans-Kranenburg2015). Besides intranasal OXT, other nonpharmaceutical methods to elicit OXT responses, such as physical contact and massage, might prove beneficial (Carter et al., Reference Carter, Pournajafi-Nazarloo, Kramer, Ziegler, White-Traut, Bello and Schwertz2007). Obviously, such interventions can also aid the therapeutic effects of individuals who are responsive to training programs and therapy. Recent studies investigating the effect of intranasal administration combined with behavioral therapy in social anxiety disorder and autism have unfortunately not been very promising (Guastella & Hickie, Reference Guastella and Hickie2016; Guastella, Howard, Dadds, Mitchell, & Carson, Reference Guastella, Howard, Dadds, Mitchell and Carson2009). In addition, studies testing therapeutic effect of OXT in maternal depression have yielded no clear beneficial effects so far (Mah, Bakermans-Kranenburg, van IJzendoorn, & Smith, Reference Mah, van IJzendoorn, Smith and Bakermans-Kranenburg2013; Mah, van IJzendoorn, Smith, & Bakermans-Kranenburg, Reference Mah, Bakermans-Kranenburg, van IJzendoorn and Smith2015), and these findings have tempered the expectations of OXT as therapeutic agent (Shen, Reference Shen2015). These few studies have however been done in mostly small samples and have not taken into account the modulating factors on OXT sensitivity as discussed in this review. Neither have these studies targeted a context in which the effects of OXT might prove most fruitful: in the therapeutic setting with both caregiver and child. Testing the effect of OXT in such a setting, with larger samples in which developmental factors such as genetics and early life environmental factors are taken into account, might therefore yield more promising findings. Besides bringing forth fundamental knowledge on the endocrinology of caregiving, the current model can thus also aid the development of new methods of altering and administrating steroid hormones and neuropeptides, as well as bring to light novel endocrine targets for intervention.
A few limitations of this review should also be mentioned. By definition, a selective review is incomplete, and by focusing on hormones and neuropeptides, a number of neuromodulators have not been considered. For example, both dopamine and serotonin are not included in this review, but both have proven relevant for caregiving behavior: dopamine for its strong link with the reward system and its interactions with PRL, OXT, and testosterone (Briffaud, Williams, Courty, & Broberger, Reference Briffaud, Williams, Courty and Broberger2015; Packard, Schroeder, & Alexander, Reference Packard, Schroeder and Alexander1998). Because dopamine responses to stress in humans depend on experienced parental care, dopamine could play a role in intergenerational transmission (Pruessner, Champagne, Meaney, & Dagher, Reference Pruessner, Champagne, Meaney and Dagher2004). The link between serotonin and caregiving behavior is less clear in humans, but polymorphisms of the serotonin transporter gene have been related to sensitive parenting (Bakermans-Kranenburg & van IJzendoorn, Reference Bakermans-Kranenburg and van IJzendoorn2008). In addition, a primate model of intergenerational transmission of maternal rejection and maltreatment has ascribed an important role to serotonin (Saltzman & Maestripieri, Reference Saltzman and Maestripieri2011). A marker for lower levels of serotonin in the mothers’ central nervous system predicted higher levels of maternal rejection, and exposure to maternal rejection altered serotonin function in offspring. In addition, abused mothers who became abusive themselves had lower levels of the same proxy for serotonin compared to abused mothers who did not become abusive to their own offspring. Low serotonin levels in humans has also been related to impaired impulse control and aggressive behavior (Montoya et al., Reference Montoya, Terburg, Bos and Honk2012), and a similar mechanism as proposed in primates could thus apply to humans as well. Although the focus of the current review is on endocrine factors leaving serotonin and dopamine beyond its scope, the role of these neurotransmitters should be incorporated in future extensions of the model. Because research in humans has further shown that effects of serotonin on aggression and impulsive behavior can depend on levels of testosterone and CORT (Montoya et al., Reference Montoya, Terburg, Bos and Honk2012), integrating serotonin in the model can also give more insight into the interactive effects of the different endocrine markers in predicting caregiving behavior.
Another limitation of the model presented here is that it is based upon a limited number of studies, and many of these studies lack the ideal experimental setup, often depending on “natural experiments.” As a result of this limitation, a diverse spectrum of caregiving behaviors are described in relation to endocrinology, whereas different types of caregiving behavior might have specific effects. For example, effects on neuroendocrine systems might vary for moderate insensitive parenting versus physical abuse and maltreatment. These differences can relate to the variability that has been observed in the types of intergenerational transmission of maltreatment, which depends on the type and severity of abuse (Berzenski et al., Reference Berzenski, Yates, Egeland, Korbin and Krugman2014). Unfortunately, there are not enough data currently available to describe the different trajectories of interactions between experienced caregiving and endocrine alterations depending on type and severity, and as a consequence the presented model is coarse, and might not apply similarly to all types of intergenerational transmission. Nonetheless, whenever possible an attempt has been made to explicitly address the question of how variation in severity relates to endocrine function, such as where the effect of early life environment on the HPA axis is discussed. Besides the lack of studies investigating the specificity of subtypes of maltreatment and insensitive caregiving, comparative studies on differences between sexes in relation to caregiving and endocrinology are also scarce. Although these differences undoubtedly exist due to fundamental differences in male and female neuroendocrinology and the role both sexes have in caregiving behavior, they have not received much attention, similar to the topic of caregiving behavior in fathers. Recent studies that have however specifically targeted these topics are promising, and this focus is about to shift (e.g., Endendijk et al., Reference Endendijk, Hallers-Haalboom, Groeneveld, van Berkel, van der Pol, Bakermans-Kranenburg and Mesman2016; Kuo et al., Reference Kuo, Saini, Thomason, Schultheiss, Gonzalez and Volling2016; Rilling & Young, Reference Rilling and Young2014).
Overall, it is not surprising that studies on the topic of this review are scarce considering the difficulties in studying the endocrinology of caregiving, especially insensitive or harsh caregiving. Fortunately, there is strong interest currently in the research on developmental social neuroscience and psychopathology and the impact of inadequate caregiving, which reflects in funding opportunities and an increasing number of publications on this topic (De Haan & Gunnar, Reference De Haan and Gunnar2009; Lanius et al., Reference Lanius, Vermetten and Pain2010). This interest is not only fortunate but also of vital importance when considering the high prevalence of harsh caregiving (Gilbert et al., Reference Gilbert, Widom, Browne, Fergusson, Webb and Janson2009; Stoltenborgh et al., Reference Stoltenborgh, Bakermans-Kranenburg and van IJzendoorn2013), the impact it has on a child's social–emotional development (Heim & Nemeroff, Reference Heim and Nemeroff2001), and the risk it bears for the development of psychopathology (Cicchetti & Toth, Reference Cicchetti and Toth2009; Keyes et al., Reference Keyes, Eaton, Krueger, McLaughlin, Wall, Grant and Hasin2012; Pechtel & Pizzagalli, Reference Pechtel and Pizzagalli2011). As a consequence, the impact of harsh caregiving cannot be underestimated, but what neither can be underestimated are the possible benefits from a better understanding, and ultimately prevention, of this behavior (Anda et al., Reference Anda, Butchart, Felitti and Brown2010; Shonkoff, Reference Shonkoff2012).
Supplementary Material
To view the supplementary material for this article, please visit https://doi.org/10.1017/S0954579416000973.