1. Introduction
Development of a calibrated Cambrian timescale has resulted from precise U–Pb dating of volcanic ash zircons in fossiliferous marine successions (Landing et al. Reference Landing, Davidek, Westrop, Geyer and Heldmaier1998; Harvey et al. Reference Harvey, Williams, Condon, Wilby, Siveter, Rushton, Leng and Gabbott2011), combined with detailed carbon isotope chemostratigraphy (Kirschvink et al. Reference Kirschvink, Magaritz, Ripperdan, Zhuravlev and Rozanov1991; Magaritz et al. Reference Magaritz, Kirschvink, Latham, Zhuravlev and Rozanov1991; Maloof et al. Reference Maloof, Porter, More, Dudás, Bowring, Higgins, Fike and Eddy2010). This work has led to a progressive stepwise ‘younging’ of the system and a shortening of the chronostratigraphic divisions of the Cambrian (e.g. Bowring & Schmitz, Reference Bowring, Schmitz, Hanchae and Huskins2003, fig. 1; Peng et al. Reference Peng, Babcock, Cooper, Gradstein, Ogg, Schmitz and Ogg2012). Precise U–Pb zircon dates also allow better estimates of rates of faunal turnover and oceanic geochemical changes (Maloof et al. Reference Maloof, Schrag, Crowley and Bowring2005, Reference Maloof, Porter, More, Dudás, Bowring, Higgins, Fike and Eddy2010), the duration of biostratigraphic zones and the timing of geological events.
Radioisotopic ages and the marine record of carbon isotope excursions are particularly important for Cambrian correlation. Conventional biostratigraphic correlations are compromised by strong biogeographic, climate and lithofacies/habitat controls on the distributions of groups such as trilobites (which tend to be more offshore and unrestricted marine in distribution) and archaeocyaths (tropically restricted and which do not appear on cool-water palaeocontinents) (e.g. Debrenne & Debrenne, Reference Debrenne and Debrenne1995; Landing & Westrop, Reference Landing, Westrop, Lipps and Waggoner2004; Landing, Reference Landing2005; Landing et al. Reference Landing, Geyer, Brasier and Bowring2013). In addition, regional unconformities of often undetermined duration, the problematical biostratigraphic utility of groups such as lower Cambrian acritarchs, and correlations of the upper lower Cambrian that are often based on genus- rather than species-level comparisons of trilobite faunas all work to confound precise interregional correlation (e.g. Landing et al. Reference Landing, Geyer, Brasier and Bowring2013). For these reasons, improved inter-palaeocontinent correlations must increasingly rely on highly precise U–Pb zircon dates and detailed geochemical stratigraphy, both of which must be calibrated against local biostratigraphic zonations (e.g. Landing et al. Reference Landing, Davidek, Westrop, Geyer and Heldmaier1998; Maloof et al. Reference Maloof, Porter, More, Dudás, Bowring, Higgins, Fike and Eddy2010).
The fossil record of trilobites extends only as low as the upper lower Cambrian strata, c. 19 Ma after the beginning of the Cambrian Period (e.g. Landing et al. Reference Landing, Davidek, Westrop, Geyer and Heldmaier1998; Landing, Reference Landing1994; Peng & Babcock, Reference Peng and Babcock2005; Linnemann et al. Reference Linnemann, Ovtcharova, Schaltegger, Gärtner, Hautmann, Geyer, Vickers-Rich, Rich, Plessen, Hofmann, Ziegler, Krause, Kriesfeld and Smith2019). Among the oldest of these are olenelloids of the superfamily Fallotaspidoidea Hupé, Reference Hupé1953b, which were first described and put into a biostratigraphic succession based on collections from the Tiout Member of the upper Igoudine Formation and overlying Amouslek Formation in southern Morocco (Figs 1, 2). This synthesis featured work by Neltner (Reference Neltner1938), Neltner & Poctey (Reference Neltner and Poctey1950), Hupé & Abadie (Reference Hupé and Abadie1950) and, particularly, Hupé (Reference Hupé1950, Reference Hupé1952, Reference Hupé1953 a, b) (see reviews by Palmer & Repina, Reference Palmer and Repina1993; Geyer, Reference Geyer1996, Reference Geyer2019).

Fig. 1. Generalized geological map of southern Morocco showing Ediacaran–Cambrian rocks (medium grey), areas with Cambrian rocks (light grey) and outcrops of Neoproterozoic and Mesoproterozoic (Pan-African orogen, crosses). Trend of High Atlas and Anti-Atlas ranges shown and major basement massifs named (Adrar n’Dren, El Graara, Jbel Sirwa and Jbel Taïssa).

Fig. 2. Terminal Ediacaran–Cambrian lithostratigraphic units of the Moroccan Atlas regions; cross-section runs west (right-hand side) to east (left) and includes Lemdad Formation facies of the High Atlas; vertical lines indicate unconformity. Regional extent of sequence boundary at Igoudine–Amouslek Formation contact at Tiout unknown. Figure modified from Geyer & Landing (Reference Geyer, Landing, Geyer and Landing2006a, fig. 2), using traditional lower and middle Cambrian subdivisions. Lower–middle Cambrian boundary interval regressive, macrotidal sandstones of Tazlaft Formation distinguished from unconformably overlying, volcanic-rich, wave-dominated sandstones of Talelt Formation, which unconformably locally overlies units as low as the Issafen Formation (Landing et al. Reference Landing, Geyer and Heldmaier2006). The succession of the regressive Tazlaft–overlying transtensional Talelt depositional sequence is readily distinguished for c. 200 km in the Anti-Atlas and High Atlas ranges; the units must not be combined into a single unit and mapped as the traditional ‘regressive’ Asrir Formation (e.g. Álvaro et al. Reference Álvaro, Bellido, Gasquet, Pereira, Quesada and Sánchez-García2014).
Collecting in western Laurentia (White-Inyo and Esmeralda mountains) and SW Siberia led to the recognition that the oldest trilobite faunas in these areas include taxa that were earlier identified as Fallotaspis Hupé, Reference Hupé1953b, and referred to a ‘Fallotaspis Zone’ that was compared with the lowest known Moroccan trilobite interval. Subsequent taxonomic work has referred the Siberian taxa to similar archaespidid genera but affirmed the presence of Fallotaspis, or a genus very similar to Fallotaspis, in the White-Inyo Mountains of California and western Nevada (reviews in Geyer, Reference Geyer1996; Hollingsworth, Reference Hollingsworth2007; Landing et al. Reference Landing, Geyer, Brasier and Bowring2013).
With fallotaspidids regarded as keys to early trilobite evolution and global correlation, the well-exposed section at Tiout, southern Morocco (Fig. 1), has become particularly important. Sdzuy (Reference Sdzuy1978) described horizons with fallotaspidid and bigotinid trilobites at the Tiout section. A recent comprehensive study of these earliest trilobites from Tiout and comparable sections at Tazemmourt and Amouslek (Geyer, Reference Geyer2019) shows that numerous taxa of the Bigotinidae and Fallotaspididae occur in the Tiout Member of Geyer (Reference Geyer1990), down to a level with the lowest known identifiable Moroccan trilobites (Fig. 3, ‘T’-horizons). These trilobites are complemented by the oldest archaeocyath-bearing beds (Fig. 3, ‘A’-horizons) known in Morocco and West Gondwana (Debrenne & Debrenne, Reference Debrenne and Debrenne1978, Reference Debrenne and Debrenne1995; Debrenne et al. Reference Debrenne, Debrenne and Faure-Muret1992).
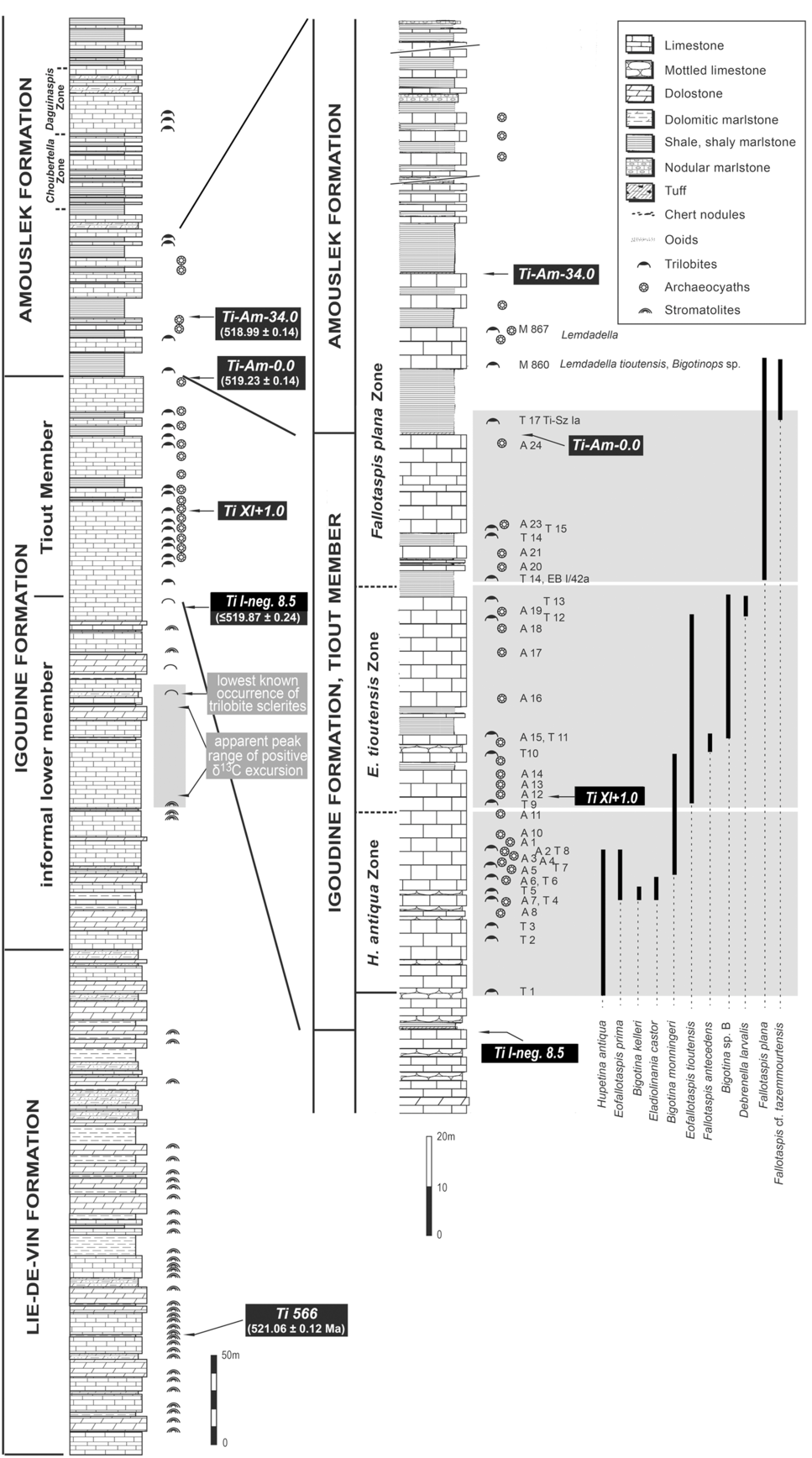
Fig. 3. Upper Igoudine (and Tiout Member) – lower Amouslek Formation at the Tiout section shows ashes and volcaniclastic sandstones examined in this study (Ti-I-neg8.5, Ti-XI-1.0, Ti-Am-0.0, Ti-Am-34.0). Horizons in Tiout Member with trilobites (Sdzuy, Reference Sdzuy1978) have the prefix ‘T’; archaeocyathan horizons (partly also with determinable trilobites) (Debrenne & Debrenne, Reference Debrenne and Debrenne1978, Reference Debrenne and Debrenne1995; Debrenne et al. Reference Debrenne, Debrenne and Faure-Muret1992) have the prefix ‘A’. Selected trilobite ranges from Geyer (Reference Geyer2019). Section modified from Sdzuy (Reference Sdzuy1978, fig. 2b) and Schmitt (Reference Schmitt1979, fig. 44).
As work much lower in the Tiout section recorded datable volcanic ashes in the Lie de vin Formation (Monninger, Reference Monninger1979; Compston et al. Reference Compston, Williams, Kirschvink, Zhang and Ma1992; Landing et al. Reference Landing, Davidek, Westrop, Geyer and Heldmaier1998; Fig. 2), it was our belief that higher volcanic ashes occurred and that U–Pb dating using modern chemical abrasion isotope dilution thermal ionization mass spectrometry (CA-ID-TIMS) protocols could yield refined geochronologic brackets on the oldest Moroccan trilobites and archaeocyaths. It is important to emphasize ‘identifiable trilobites’, as fragmentary sclerites occur c. 30 m below the Tiout Member of the Igoudine Formation at Tiout (Geyer & Landing, Reference Geyer and Landing1995, Reference Geyer, Landing, Geyer and Landing2006b ; Geyer, Reference Geyer2019). This report also includes a reanalysis of the U–Pb zircon age on a sample in the Lie de vin Formation (Tiout 566 of Landing et al. Reference Landing, Davidek, Westrop, Geyer and Heldmaier1998) below these lowest known trilobite fragments. The five new U–Pb ages reported here are combined within a Bayesian statistical framework to build a robust age model for these earliest trilobites and archaeocyaths, and the associated carbon isotope stratigraphy.
2. Geology
2.a. Generalized setting
The rock successions of the Atlas ranges include early Ediacaran andesites, rhyolites and ignimbrites with 565–560 Ma dates at their tops (e.g. Mifdal & Peucat, Reference Mifdal and Peucat1985; Walsh et al. Reference Walsh, Aleinikoff, Benziane, Yazidi and Armstrong2002; Maloof et al. Reference Maloof, Schrag, Crowley and Bowring2005) that overlie Meso- and Neoproterozoic rocks of the Pan-African orogen (Fig. 1). These older units are unconformably overlain by the marine-dominated cover sequence of the terminal Ediacaran–Silurian Souss Basin (Geyer, Reference Geyer1989), which roughly parallels the trend of the Anti-Atlas.
2.b. Adoudou Formation
The initial deposits of this cover sequence (Fig. 2) form the Adoudou Formation (Geyer, Reference Geyer1989). This unit was divided by Maloof et al. (Reference Maloof, Schrag, Crowley and Bowring2005) into a lower Tabia Member (0–250 m of lower coarse siliciclastics with massive conglomerate; middle evaporitic dolostone and limestone; and an upper shale-dominated, heterolithic interval with conglomerate, arkose, sandstone and carbonate) and an upper Tifnout Member (up to 1000 m of shallow-marine, massive carbonate and minor siliciclastic beds with desiccation and tepee structures and gypsum moulds; see Monninger, Reference Monninger1979). Fossils, except for stromatolites and poorly preserved ichnofossils, are unknown in the Adoudou Formation.
Correlation of the Adoudou Formation is based on a −6‰ δ13C nadir in the lower Tifnout Member that is regarded as marking the Ediacaran–Cambrian boundary (Maloof et al. Reference Maloof, Schrag, Crowley and Bowring2005, fig. 2, but also reported as at the ‘base of the Tifnout’ on p. 2206). The middle Tifnout Member at Oued Sdas, c. 5.0 km east of the Tiout section, has a tuff with a weighted mean zircon 206Pb/238U age of 525.23 ± 0.12 (0.38) [0.94] Ma (Maloof et al. Reference Maloof, Porter, More, Dudás, Bowring, Higgins, Fike and Eddy2010, fig. 2). This tuff is likely correlative, in part, with the Tommotian Stage of the Siberian Platform (Maloof et al. Reference Maloof, Porter, More, Dudás, Bowring, Higgins, Fike and Eddy2010) and upper Stage 2 of the global lowest Cambrian chronostratigraphic reference section (see Terreneuvian Series geochronology in Landing & Kouchinsky, Reference Landing and Kouchinsky2016).
2.c. Lie de vin Formation
Continued restricted marine deposition is recorded by cyclically bedded siltstone and carbonate of the Lie de vin Formation (Choubert, 1952; to 940 m thick at Tiout), with rare stromatolites, thrombolites and evaporite mineral pseudomorphs (Monninger, Reference Monninger1979). The Lie de vin has Cambrian-aspect trace fossils (e.g. a form identified as Diplocraterion in Latham & Riding, Reference Latham and Riding1990, fig. 2). An early Cambrian SHRIMP U–Pb zircon date of 521 ± 7 Ma was determined 730 m above the base of the Lie de vin Formation (Compston et al. Reference Compston, Williams, Kirschvink, Zhang and Ma1992), with a more refined ID-TIMS U–Pb zircon date of 522 ± 2 Ma at 763 m at the Tiout section (Landing et al. Reference Landing, Davidek, Westrop, Geyer and Heldmaier1998). Maloof et al. (Reference Maloof, Porter, More, Dudás, Bowring, Higgins, Fike and Eddy2010) reported a CA-ID-TIMS U–Pb zircon date of 520.93 ± 0.14 Ma for a tuff in the upper Lie de vin Formation at 1736 m in the Oued Sdas section, purportedly near the same stratigraphic level as the coeval date in the Tiout section (Fig. 1). These dates are broadly consistent with proposed δ13C correlations of the Lie de vin Formation with the Siberian Tommotian Stage (e.g. Brasier et al. Reference Brasier, Magaritz, Corfield, Luo, Wu, Lin, Jiang, Hamdi, He and Fraser1991; Magaritz et al. Reference Magaritz, Kirschvink, Latham, Zhuravlev and Rozanov1991; Maloof et al. Reference Maloof, Schrag, Crowley and Bowring2005).
Boudda & Choubert (Reference Boudda and Choubert1972) reported trilobite fragments from rocks assigned to the upper Lie de vin Formation in the High Atlas range. However, these undescribed fragments actually come from a red siliciclastic mudstone in the lowest Lemdad Formation (Fig. 2) that is now correlated with the lower Igoudine Formation (Sdzuy, Reference Sdzuy1978; Geyer & Landing, Reference Geyer, Landing, Geyer and Landing2006 b, p. 72). Unidentified trilobite fragments also occur in the lower Igoudine Formation at the Tiout section in the Anti-Atlas (Geyer & Landing, Reference Geyer and Landing1995, p. 35, Reference Geyer, Landing, Geyer and Landing2006 b), providing a compelling reason to refine the U–Pb zircon date on an ash (Tiout 566) 180 m below the top of the Lie de vin (Monninger, Reference Monninger1979; Landing et al. Reference Landing, Davidek, Westrop, Geyer and Heldmaier1998) in order to bracket the lowest local occurrence, and perhaps global appearance, of trilobites.
2.d. Igoudine and Amouslek formations
The highest units in this report are the Igoudine and Amouslek formations of Geyer (Reference Geyer1989; Fig. 2). Development of an extensive carbonate platform following regional marine deepening is recorded by the massive, dove-grey weathering, black limestone-dominated Igoudine Formation (up to 400 m thick, with c. 190 m at the Tiout section; Monninger, Reference Monninger1979).
Further deepening is recorded by the change from an unnamed lower member of the Igoudine Formation, with black laminated limestones and dolosiltites and unidentifiable trilobite sclerites (Geyer & Landing, Reference Geyer and Landing1995, Reference Geyer, Landing, Geyer and Landing2006a , b), into the fossiliferous Tiout Member (Geyer, Reference Geyer1989) of the upper Igoudine (Figs 2, 3). The overlying Amouslek Formation represents open-marine deposition with abundant trilobites and archaeocyaths. This unit (260 m at Tiout; Monninger, Reference Monninger1979) features a distinctive change from massive carbonates of the Igoudine Formation up into initially siliciclastic mudstone-dominated deposits of the Amouslek Formation. The Igoudine–Amouslek contact is an unconformity mantled with an ash (Geyer & Landing, Reference Geyer, Landing, Geyer and Landing2006 a, b; sample Ti-Am-0.0 here), which is best regarded as a sequence boundary in the Anti-Atlas (Figs 2, 4). The gradual upwards increase in carbonate beds through the formation makes the Amouslek comparable to a ‘Grand cycle’, as first described in west Laurentia (Geyer & Landing, Reference Geyer and Landing1995).

Fig. 4. Volcanic ash at base of Amouslek Formation mantles sequence boundary on the Tiout Member of the upper Igoudine Formation at Tiout.
2.e. Tiout Member
The Tiout Member has ooid pack- and wackestones and an abundant macrofauna with identifiable trilobites, archaeocyaths, hyoliths and chancelloriids. The stratigraphically abrupt appearances of trilobites and, 13 m higher, of the oldest Moroccan archaeocyaths at the Tiout section (Figs 1, 3) do not reflect evolutionary events but track the appearance of more normal marine facies (Monninger, Reference Monninger1979; Geyer, Reference Geyer1989; Geyer & Landing, Reference Geyer and Landing1995; Landing et al. Reference Landing, Geyer, Brasier and Bowring2013). Sdzuy’s (Reference Sdzuy1978) work, which established the Eofallotaspis Zone, documented the lowest known identifiable trilobites in Morocco, with rare trilobite fragments found low in the lower member of the Igoudine Formation (Fig. 2) (Geyer & Landing, Reference Geyer and Landing1995, Reference Geyer, Landing, Geyer and Landing2006b ).
2.f. Tiout and Timoulaye Izder sections
The N-dipping (25–35°) Tiout section lies at the north rim of the Anti-Atlas and about 25 km SE of Taroudant (Fig. 1). This is certainly the best studied and referenced Cambrian succession in Morocco. It extends along a canyon from the poorly exposed Tabia Member of the Adoudou Formation (at a hamlet at c. 30° 20′ 30″ N, 8° 41′ 50″ W) through the lower 260 m of the Amouslek Formation (Geyer & Landing, Reference Geyer and Landing1995, Reference Geyer, Landing, Geyer and Landing2006b ; see Stop 1 of both field guides). The upper, most relevant part of the section lies at c. 30° 22′ 40″ N, 8° 41′ 20″ W. Monninger (Reference Monninger1979) measured the Tiout section beginning in the upper 200 m of the Adoudou Formation. He painted numbers on each of his described beds through the Igoudine Formation that are still visible at places as an aid in field work. The early Cambrian U–Pb zircon dates from the Lie de vin Formation described above (Section 2.c) come from his beds 563 and 566 on the east side of the canyon.
The upper part of the lower member of the Igoudine Formation and the Tiout Member are exposed high in the cliffs on the east side of the canyon mouth at Tiout. Some of the horizons that yielded trilobites and archaeocyaths from the Tiout Member in the 1970s are painted with a letter–number designation. Each of the fossiliferous horizons in Figure 3 can therefore be readily located (although the markings have decayed over the last decade). These fossils include Sdzuy’s (Reference Sdzuy1978) trilobite horizons T1–T17, and the oldest known Moroccan archaeocyath-bearing levels at horizons A1–A24 of Debrenne & Debrenne (Reference Debrenne and Debrenne1978, Reference Debrenne and Debrenne1995) and Debrenne et al. (Reference Debrenne, Debrenne and Faure-Muret1992). Some archaeocyath horizons also yield trilobites (Fig. 3).
The Timoulaye Izder section is c. 2 km NE of Timoulaye Izder village on the NW margin of the Anti-Atlas (Fig. 1; c. 20° 12’ 30″ N, 9° 35′ 50″ W). It exposes the upper Igoudine (Tiout Member) through middle Issafen formations in a lithofacies different from that in the Tiout section, but characteristic of the western Anti-Atlas (Geyer & Landing, Reference Geyer, Landing, Geyer and Landing2006 b). The succession progressively steepens in dip from 20–27° SE on the cliff-forming Tiout Member – Amouslek Formation to nearly vertical on the slope-forming Issafen Formation. A total of 27 m of brownish-weathering Tiout Member are exposed on the dip slope, and are succeeded by a thick (27–312 m), deepening–shoaling succession through the Amouslek. The lowest Amouslek is nodular, trilobite-bearing wackestones in green shale, and the lower Amouslek is dominated by massive, algal build-ups (Geyer & Landing, Reference Geyer, Landing, Geyer and Landing2006 b). The middle Amouslek (79.5–288 m) is dominantly deeper-water, trilobite-bearing, bluish green to green siltstone and shale with thin nodular and trilobite hash limestone. Shallowing is obvious in the uppermost Amouslek, with evidence for shallow-water, wave-dominated deposition of fine-quartz sand- and ooid/pisolite- dominated intervals, and increased rates of deposition suggested by ball-and-pillow structures (Geyer & Landing, Reference Geyer, Landing, Geyer and Landing2006 b). Sample Tim-269.5 comes from the upper Amouslek Formation at the Timoulaye Izder section, within the upper Choubertella, or possibly the lower Daguinaspis Zone (G. Geyer, unpublished data).
2.g. Tiout faunas and an emended biostratigraphy
All of the trilobite-bearing horizons in the Tiout Member at Tiout (Fig. 1) were referred to the ‘Eofallotaspis beds’ by Sdzuy (Reference Sdzuy1978) and subsequently termed the ‘Eofallotaspis Zone’ (Geyer, Reference Geyer1990). These trilobite assemblages were initially believed to show little difference in faunal composition through c. 110 m of the Tiout Member (Geyer & Landing, Reference Geyer and Landing1995, Reference Geyer, Landing, Geyer and Landing2006b ) and thought to reflect a relatively brief geological interval. A recent study by Geyer (Reference Geyer2019) shows a very rapid vertical change in trilobite faunas and their diversity in the Tiout Member and lower Amouslek Formation. This required division of the Eofallotaspis Zone of Sdzuy (Reference Sdzuy1978) into a lower Hupetina antiqua and an upper Eofallotaspis tioutensis Zone, as well as modification of the overlying, previously defined Fallotaspis tazemmourtensis Zone to a new Fallotaspis plana Zone with a slightly lower lower boundary. The lowest known Fallotaspis plana at horizon T14 is used to assign the upper range of E. tioutensis to the F. plana Zone. Hupé’s (Reference Hupé1952, Reference Hupé1953a ) and Geyer’s (Reference Geyer1990) original F. tazemmourtensis Zone was earlier recognized as a thin and likely temporally brief interval at other sections (e.g. Tazemmourt; Geyer, Reference Geyer1996) equivalent to the upper F. plana Zone.
Bigotinids dominate the trilobites through the Tiout Member, and Eofallotaspis persists through much of the Tiout (Geyer, Reference Geyer2019). All trilobites of these surprisingly diverse associations belong to the Bigotinidae and Fallotaspididae, of which only Hupetina antiqua, Eofallotaspis prima, E. tioutensis and Fallotaspis cf. F. tazemmourtensis were earlier identified by Sdzuy (Reference Sdzuy1978, Reference Sdzuy1981). Geyer (Reference Geyer2019) introduced the new genera and species Bigotina kelleri, B. monningeri, Issendalenia grandispina, Tioutella floccofavosa, Pseudobigotina antiatlasensis, Eladiolinania castor, E. pollux, Debrenella larvalis and Fallotaspis antecedens from the Tiout section, and Bigotinops chouberti from below the known range of F. tazemmourtensis in the Amouslek section.
The successive higher zones named by Hupé (Reference Hupé1953a) (Fallotaspis tazemmourtensis, Choubertella and Daguinaspis zones) in southern Morocco were based on the lowest occurrences of the eponymous fallotaspidoid genera and species. The recovery of F. cf. tazemmourtensis Hupé, Reference Hupé1953a and F. plana below the Amouslek Formation, and thus a range overlapping with that of Eofallotaspis spp., required a change of the biostratigraphical concepts and the zonal boundaries (Geyer, Reference Geyer2019; Fig. 3).
Fallotaspis tazemmourtensis and F. plana appear to be limited to shaly rocks (Geyer, Reference Geyer1996, Reference Geyer2019), with the lowest occurrence of the species in a shaly layer in the Tiout Member at Tiout (Fig. 3, horizon T14). However, an even earlier species of Fallotaspis, F. antecedens Geyer, Reference Geyer2019, also occurs in limestones (Fig. 3).
Biostratigraphic re-evaluation of the Tiout section also included the fact that sclerites tentatively attributed to Fallotaspis bondoni (Neltner & Poctey, Reference Neltner and Poctey1950) are now known near the base of the c. 260-m-thick overlying Amouslek Formation (Fig. 3). Other occurrences of F. bondoni are limited to the Amouslek Formation above the Fallotaspis plana Zone and are from the higher Choubertella and Daguinaspis zones in southern Morocco (Geyer, Reference Geyer1996). Probable recovery of F. bondoni at Tiout suggests that the lower Amouslek Formation probably belongs to the Choubertella Zone (Fig. 3). Daguinaspis (the index fossil of the zone above the Choubertella Zone) is a frequent faunal element in the upper–uppermost Amouslek and occurs only higher in the Amouslek at Tiout (Geyer, Reference Geyer1996; Geyer & Landing, Reference Geyer, Landing, Geyer and Landing2006 b, p. 53). It should be noted, however, that Lemdadella tioutensis and Bigotinops sp. occur in the Tiout section in the lowest limestone of the Amouslek Formation and above the shales with F. cf. bondoni. Lemdadella tioutensis was described by Sdzuy (in Liñán & Sdzuy, Reference Sdzuy1978) from Tiout and assigned to Hupé’s (Reference Hupé1953a) F. tazemmourtensis Zone, although neither F. tazemmourtensis (from sample TIOU-T14) nor F. cf. bondoni (from TI-Sz Ia) were known at that time from Tiout (samples in Geyer & Landing, Reference Geyer and Landing1995, p. 59).
The Hupetina antiqua – Eofallotaspis tioutensis succession is complemented by numerous horizons with archaeocyaths, with a lowest appearance of determinable archaeocyaths 13 m above the lowest identifiable trilobites (Debrenne & Debrenne, Reference Debrenne and Debrenne1978; Debrenne et al. Reference Debrenne, Debrenne and Faure-Muret1992) and above the lithofacies change from the lower Igoudine Formation into the Tiout Member. A lowest archaeocyath zone (Erismacoscinus fasciola – Retecoscinus minutus Zone) is recognized through the Tiout Member and equated with the Eofallotaspis beds and Zone of Sdzuy (Reference Sdzuy1978) and Geyer (Reference Geyer1990) (Debrenne & Debrenne, Reference Debrenne and Debrenne1978, Reference Debrenne and Debrenne1995). The two eponymous species of the oldest archaeocyath zone do not appear above the Tiout Member. The overlying Amouslek Formation brackets the long-ranging Erismacoscinus marocanus Zone and the traditional Fallotaspis tazemmourtensis (extended downwards in this report to horizon T14), Choubertella and Daguinaspis trilobite zones (e.g. Debrenne & Debrenne, Reference Debrenne and Debrenne1995, p. 122). It should be noted that, in contrast to the trilobite succession, no significant change in composition of the archaeocyath assemblages can be recognized in the Tiout Member.
The Hupetina antiqua through Daguinaspis zonal succession is significant as it defines the Issendalenian Stage low in the lower Cambrian strata of the West Gondwanan composite chronostratigraphic succession. It is equivalent to most of the traditional ‘Ovetian Stage’ of Iberia (Geyer & Landing, Reference Geyer and Landing2004).
3. Methods
3.a. U–Pb geochronology
The dove-grey weathering, black limestones of the upper Igoudine Formation (Tiout Member) at Tiout contain a small number of brown weathering, thin siliciclastic sandstones. Two of these with high feldspar content and potential volcanic lapilli fragments were sampled (Ti-I-neg8.5 and Ti-XI+1). Fine-grained volcanic tuffs were also sampled at the base of the Amouslek Formation (Ti-Am-0.0) and higher in the formation (Ti-Am-34.0). The tuff at 763 m (Section 2.c) in the Tiout section (Ti-566) was re-dated in this study from the same mineral separation prepared and reported by Landing et al. (Reference Landing, Davidek, Westrop, Geyer and Heldmaier1998).
Zircon crystals were separated from hand samples by conventional density and magnetic methods (Table 1). The entire zircon separate was placed in a muffle furnace at 900°C for 60 hours in quartz beakers to anneal minor radiation damage; annealing enhances cathodoluminescence emission (Nasdala et al. Reference Nasdala, Christian, Lengauer, Hanchar, Kronz, Wirth, Philippe, Kennedy and Seydoux-Guillaume2002), promotes more reproducible inter-element fractionation during laser ablation inductively coupled plasma mass spectrometry (LA-ICP-MS) (Allen & Campbell, Reference Allen and Campbell2012), and prepares the crystals for subsequent chemical abrasion (Mattinson, Reference Mattinson2005). Following annealing, individual grains were hand-picked and mounted, polished and imaged by cathodoluminence (CL) on a scanning electron microscope. From these compiled images, grains with consistent and dominant CL patterns were selected for further isotopic analysis.
Table 1. Zircon chemical abrasion IDTIMS U-Pb isotopic data

(a) z1, z2 etc. are labels for zircon fragments annealed and chemically abraded after Mattinson (Reference Mattinson2005); data formatted in bold are included weighted mean calculation.
(b) Model Th/U ratio calculated from radiogenic 208Pb/206Pb ratio and 207Pb/235U date.
(c) Pb* and Pbc are radiogenic and common Pb, respectively. Mol % 206Pb* is with respect to radiogenic and blank Pb.
(d) Measured ratio corrected for spike and fractionation only. Samples were spiked with the ET535 tracer, with an internal U fractionation correction based on the measured 233U/235U, and an external Pb fractionation correction of 0.16 ± 0.02 (1-sigma) %/amu (atomic mass unit), based on analysis of double Pb ET2535 spiked samples over the same analytical period.
(e) Corrected for fractionation, spike, common Pb and initial disequilibrium in 230Th/238U. Up to 0.5 pg of common Pb is assigned to procedural blank with composition of 206Pb/204Pb = 18.042 ± 0.61%; 207Pb/204Pb = 15.537 ± 0.52%; 208Pb/204Pb = 37.686 ± 0.63% (1-sigma). Excess over blank was assigned to initial common Pb, using the Stacey & Kramers (Reference Stacey and Kramers1975) two-stage Pb isotope evolution model at 520 Ma.
(f) Errors are 2-sigma, propagated using algorithms of Schmitz & Schoene (Reference Schmitz and Schoene2007). Corr. coeff. – correlation coefficient.
(g) Calculations based on the decay constants of Jaffey et al. (Reference Jaffey, Flynn, Glendenin, Bentley and Essling1971). 206Pb/238U and 207Pb/206Pb ratios and dates corrected for initial disequilibrium in 230Th/238U using Th/U [magma] = 3.
(h) Uncertainties listed as ± analytical (analytical + tracer) [analytical + tracer + decay constant] error; MSWD = mean squared weighted deviation.
The methods for U–Pb geochronology by both LA-ICP-MS and CA-ID-TIMS follow those of Schmitz & Davydov (Reference Schmitz and Davydov2012) and MacDonald et al. (Reference MacDonald, Schmitz, Strauss, Halverson, Gibson, Eyster, Cox, Mamrol and Crowley2018). A full suite of trace-element concentrations in zircon, as well as low-resolution U-Pb dates, were obtained by LA-ICP-MS on 25 µm diameter spot domains on multiple zircon crystals for each sample (online Supplementary Tables S1–S3, available at http://journals.cambridge.org/geo). Blank-subtracted trace-element analyte signals were internally normalized to 29Si and calibrated to NIST-610 and -612 glasses. U–Pb and Pb–Pb dates were calibrated with respect to interspersed measurements of several zircon reference materials. The primary standard Plešovice zircon (Sláma et al. Reference Sláma, Jan, Condon, Crowley, Gerdes, Hanchar, Horstwood, Morris, Nasdala, Norberg, Schaltegger, Schoene, Tubrett and Whitehouse2008) was used to monitor time-dependent instrumental fractionation, and a secondary correction was subsequently applied to each analysis as a function of radiogenic Pb count rate compared with the returned age bias in reference materials including Seiland, Zirconia and Plešovice zircon.
Following LA-ICP-MS spot analysis, selected zircon crystals were subjected to a modified version of the chemical abrasion method of Mattinson (Reference Mattinson2005), whereby single crystal fragments plucked from grain mounts were individually abraded in a single step with concentrated hydrogen fluoride at 180°C for 12 hours. Residual crystals were rinsed, spiked with the ET535 mixed 205Pb–233U–235U tracer solution, and processed for ID-TIMS analysis. U–Pb dates and uncertainties for each CA-ID-TIMS analysis were calculated using the algorithms of Schmitz & Schoene (Reference Schmitz and Schoene2007) and the U decay constants of Jaffey et al. (Reference Jaffey, Flynn, Glendenin, Bentley and Essling1971). Uncertainties are based upon non-systematic analytical errors, including counting statistics, instrumental fractionation, tracer subtraction and blank subtraction. These error estimates should be considered when comparing our 206Pb/238U dates with those from other laboratories that used tracer solutions calibrated against the EARTHTIME gravimetric standards. When comparing our dates with those derived from other decay schemes (e.g. 40Ar/39Ar, 187Re–187Os), the uncertainties in tracer calibration (0.03%; Condon et al. Reference Condon, McLean, Bowring and Parrish2015; McLean et al. Reference McLean, Condon, Schoene and Bowring2015) and U decay constants (0.108%; Jaffey et al. Reference Jaffey, Flynn, Glendenin, Bentley and Essling1971) should be added to the internal error in quadrature. Quoted errors for calculated weighted means are therefore of the form ±X(Y)[Z], where X is solely analytical uncertainty, Y is the combined analytical and tracer uncertainty, and Z is the combined analytical, tracer and 238U decay constant uncertainty. The mean squared weighted deviation (MSWD; Wendt & Carl, Reference Wendt and Carl1991) was used to evaluate the goodness of fit of weighted mean model ages (online Supplementary Files S1–S4, available at http://journals.cambridge.org/geo).
3.b. Bayesian age modelling
Bayesian models attempt to estimate the probable values of unknown parameters (θ) based on prior information about these parameters, which can be conditioned by observed data, or likelihoods (x). In the case of age modelling, dated horizons at particular stratigraphic levels (x) condition the parameters of the age model (θ) in concert with prior knowledge of those parameters from constraints such as stratigraphic superposition and/or assumptions of sedimentation rate and its variability. We used the Bayesian age modelling software modifiedBchron (Trayler et al. Reference Trayler, Schmitz, Cuitiño, Kohn, Bargo, Kay, Strömberg and Vizcaíno2019), an open-source R package evolved from the package Bchron (Haslett & Parnell, Reference Haslett and Parnell2008) for deep time applications. These packages are identical in their use of a compound Poisson-gamma distribution of accumulation events under the constraint of stratigraphic superposition to describe the prior probability of an ensemble of piece-wise linear sedimentation paths, which are conditioned by likelihood functions describing dated horizons (Haslett & Parnell, Reference Haslett and Parnell2008). Additionally, modifiedBchron uses an adaptive proposal algorithm in its Markov Chain Monte Carlo engine to remove any necessity for user scaling of the space or time domains, and still ensure effective and efficient sampling of the posterior parameter distributions (Trayler et al. Reference Trayler, Schmitz, Cuitiño, Kohn, Bargo, Kay, Strömberg and Vizcaíno2019). For the likelihood functions, modifiedBchron can represent radioisotopic ages as a single Gaussian normal distribution (e.g. to represent a weighted mean age), but can also aggregate individual (normally distributed) crystal analyses of a sample at a given stratigraphic horizon to accurately reflect the complex probability function of dated crystals in some volcanic event beds. Similarly, uniform distributions can be input as likelihood functions (e.g. to represent magnetostratigraphic, chemostratigraphic or cyclostratigraphic signals), and aggregated at any stratigraphic horizon to approximate any arbitrarily complex distribution (e.g. arising from detrital zircon maximum depositional age constraints).
4. Results
4.a. Tiout 566
As part of this study, the age of the 10-cm-thick ash collected at 763 m in the Tiout section and reported as 522 ± 2 Ma (Landing et al. Reference Landing, Davidek, Westrop, Geyer and Heldmaier1998, their sample Tiout 566) was re-measured using chemical abrasion TIMS methods. This sample is c. 210 m below the top of the Lie de vin Formation and c. 310 m below the carbon excursion in the lower member of the Igoudine Formation that is equated with the Siberian IV excursion. Sample Tiout 566 is 500 m below the base of the Tiout Member with its trilobites and archaeocyaths (Monninger, Reference Monninger1979; Kirschvink et al. Reference Kirschvink, Magaritz, Ripperdan, Zhuravlev and Rozanov1991).
Cathodoluminescence imaging of mounted zircons revealed a bimodal distribution of CL-dark, oscillatory zoned crystals and CL-bright weakly zoned crystals (online Supplementary Fig. S1, available at http://journals.cambridge.org/geo). LA-ICP-MS spot analyses produced a majority of Cambrian and a minority of late Ediacaran 206Pb/238U dates, irrespective of CL pattern. Trace-element concentrations and ratios for these zircons are plotted in Figure 5; the Tiout 566 zircon compositions define two fields or branches in bivariate and ternary spaces. Seven crystals chosen from both subpopulations were selected for CA-ID-TIMS analysis; all seven crystals yielded concordant and equivalent U–Pb dates, with a weighted mean 206Pb/238U date of 521.06 ± 0.12 (0.28) [0.61] Ma (MSWD = 0.61; Fig. 6). The invariance in age as a function of zoning and composition is taken as indicating that any magmatic complexity in the crystal population is minor compared with the analytical variance; the weighted mean date is therefore interpreted as the depositional age of the tuff horizon.

Fig. 5. Ternary and bivariate diagrams illustrating variation in zircon geochemistry for four volcanic ash beds of the upper Lie de vin Formation and Amouslek Formation, southern Morocco. Zircon geochemistry was measured by spot LA-ICP-MS analysis.

Fig. 6. Condordia diagram and ranked 206Pb–238U age plots for zircons from volcanic ash beds of the upper Lie de vin Formation and Amouslek Formation, southern Morocco. Zircon ages were measured by CA-ID-TIMS.
4.b. Ti-I-neg8.5
This 1.5-cm-thick, brown-weathering, dolomitic feldspathic sandstone layer is 8.5 m below trilobite horizon T1 (base of Hupetina antiqua Zone) and in the uppermost part of the lower member of the Igoudine Formation. The sample has abundant, sub-rounded, white to pink feldspars and lapilli-sized, whitish ash fragments. A sparse sample of small zircon crystals of heterogeneous aspect, rounding, colour and clarity was obtained from this sample; this optical heterogeneity is affirmed in the variation in CL response and internal zoning (online Supplementary Fig. S1). LA-ICP-MS analysis of 18 crystals yielded 206Pb/238U dates ranging from the middle Cambrian Period to Palaeoproterozoic Era. Three of the youngest crystals, including two distinctive elongate prismatic zircons with bright CL response, were analysed by CA-ID-TIMS. The latter two crystals gave reproducible 206Pb/238U dates with a weighted mean of 519.87 ± 0.24 (0.35) [0.64] Ma (MSWD = 2.60), which is interpreted as a maximum depositional age constraint for this stratigraphic horizon.
4.c. Ti-XI+1
This sample comes from a lenticular, 10-cm-thick, reddish-brown weathering dolomitic feldspathic sandstone with large, sub-rounded, white feldspars. The sample horizon is located 1.0 m above trilobite horizon T9 in the upper Eofallotaspis tioutensis Zone of the Tiout Member. As for sample Ti-I-neg8.5, this sample yielded sparse, heterogeneous zircon crystals (online Supplementary Fig. S1). LA-ICP-MS analysis of 25 crystals yielded apparent 206Pb/238U dates ranging from 1947 to 409 Ma. Given the heterogeneity and discordance of these likely detrital crystals, no further CA-ID-TIMS work was undertaken.
4.d. Ti-Am-0.0
Ti-Am-0.0 is a 5–13-cm-thick, yellow-white weathering volcanic tuff with abundant euhedral to subhedral, large white feldspars and occasional grey, rounded, porous lapilli up to 5 mm in diameter. The bed has reworked clasts of lime mudstone and rests unconformably on locally truncated thrombolites at the top of the Tiout Member. This ash is the basal bed of the Amouslek Formation and is assigned here to the transition between the upper Fallotaspis plana Zone and lower Choubertella Zone.
This tuff sample yielded abundant, sharply facetted, prismatic zircon crystals with a uniform CL response and zoning characterized by brighter interiors and moderately darker margins, often with sector zoning (online Supplementary Fig. S1). LA-ICP-MS spot analyses produced late Ediacaran to dominantly Cambrian apparent ages. Trace-element concentrations and ratios for these zircons are plotted in Figure 5; these compositions define a field distinct from those of the underlying Tiout 566 ash bed in bivariate and ternary spaces. Seven crystals selected for CA-ID-TIMS analysis yielded six concordant and equivalent U–Pb dates, with a weighted mean 206Pb/238U date of 519.23 ± 0.14 (0.21) [0.58] Ma (MSWD = 0.29; Fig. 6). A single crystal gave an anomalously young date attributed to slight residual Pb loss. The weighted mean date of the six texturally, geochemically and geochronologically similar crystals is interpreted to estimate the eruption and deposition of the tuff.
4.e. Ti-Am-34.0
Sample Ti-Am-34.0 is identical in lithology to Ti-Am-0.0. The sample comes from an interval in the lower Amouslek Formation (33.75–34.1 m) not directly bracketed by index fossils. Lithostratigraphic correlation with the neighbouring section at Tazemmourt (e.g. Geyer & Landing, Reference Geyer, Landing, Geyer and Landing2006 b, p. 60–64, fig. 11) suggests that it belongs to the upper Choubertella Zone. The sample horizon lies within a succession of seven thin, current cross-bedded ashes.
This sample yielded abundant, sharply facetted, prismatic zircon crystals from elongate to mostly more equant morphology. CL response revealed a majority of crystals characterized by relatively subdued intensity and superimposed oscillatory zoning, while a handful of crystals were very bright and homogeneous (online Supplementary Fig. S1). LA-ICP-MS spot analyses produced discordant Precambrian results as well as concordant Cambrian apparent ages. Trace-element concentrations and ratios for these zircons are distinct from the underlying Tiout 566 and Ti-Am-0.0 ash beds in bivariate and ternary spaces, particularly in their high actinide concentrations (Fig. 5). Eight crystals selected for CA-ID-TIMS analysis yielded six concordant and equivalent U–Pb dates, with a weighted mean 206Pb/238U date of 518.99 ± 0.14 (0.20) [0.58] Ma (MSWD = 0.38; Fig. 6), which is interpreted to estimate the eruption and deposition of the tuff. A single crystal gave a resolvably young date attributed to slight residual Pb loss, unsurprising given the higher U concentrations in this crystal. A second crystal belonging to the minority bright CL intensity subpopulation gave a resolvably older age, consistent with crystal inheritance as the origin of these anomalous grains.
4.f. Tim-269.5
Sample Tim-269.5 from the Timoulaye Izder section is a fairly indurated, calcareous, light-yellowish-grey ash bed with unrounded, sand-sized ash fragments. This ash produced a modest sample of relatively small, facetted zircon crystals, which are admittedly somewhat heterogeneous in CL response. LA-ICP-MS spot analyses on 35 crystals recovered indications of a bimodal crystal population, including an inherited c. 2.0 Ga component comprising mostly CL-dark crystals, and a Cambrian component comprising mostly crystals with moderate to bright CL intensity. Eight crystals were selected from the latter group for CA-ID-TIMS analysis. Of these crystals, five of those with the brightest CL response yielded obvious inheritance ages of 522–603 Ma; the remaining three crystals are concordant and equivalent in isotope ratios, with a weighted mean 206Pb/238U date of 518.59 ± 0.20 (0.32) [0.63] Ma (MSWD = 0.22; Fig. 6), which is interpreted to estimate the eruption and deposition of the tuff and is a date within the transition between the Choubertella and Daguinaspis Zones.
4.g. Bayesian age model
We conditioned the modifiedBchron age model for the Tiout section with the four volcanic ash bed depositional ages from the upper Lie de vin Formation and Amouslek Formation, as well as the maximum depositional age constraint from the volcanic sandstone (sample Ti-I-neg8.5) near the base of the Tiout Member. The position of the ash bed at Timoulaye Idzer was projected into the Tiout section by proportional scaling of the thickness of the Amouslek formation and the appearance of Daguinaspis trilobites at each section, with an associated stratigraphic uncertainty. Similarly, the position of the base of the Choubertella Zone and top of the Daguinaspis Zone at Tiout were projected via proportional scaling from their better-constrained positions in the Timoulaye Idzer section, with secondary control from the nearby Tazemmourt section. Weighted mean ages and their normally distributed standard errors were used for the ash bed depositional ages. We constructed a probability density function for the detrital maximum depositional age by applying a uniform probability between the weighted mean age of the youngest detrital zircons from Ti-I-neg8.5 and that of the next overlying depositional age (ash Ti-Am-0.0; Fig. 7). This complex probability density function was approximated by a stack of uniform probability functions grouped at the sample horizon depth.

Fig. 7. Bayesian age model for the Tiout section, southern Morocco, constructed using the modifiedBchron R package of Trayler et al. (Reference Trayler, Schmitz, Cuitiño, Kohn, Bargo, Kay, Strömberg and Vizcaíno2019). Inset illustrates the conditioned posterior probability relative to the starting likelihood function of the detrital zircon maximum depositional age for the volcanic sandstone horizon Ti-I-neg8.5. Model suggests no significant duration of sub-Amouslek sequence boundary. DZ – detrital zircon.
Two age models are illustrated in Figure 7; the median (most likely) paths of each model are indicated by solid lines, with the 95% highest density interval shown as a shaded or dashed envelope. A model constructed solely with the volcanic ash beds is illustrated with the red solid and dashed lines. For comparison, the age model with the detrital zircon maximum depositional age constraint is shown by the black median line and surrounding grey envelope encompassing the 95% highest density interval. Although the addition of the detrital zircon constraint makes a minor difference in the median age model, its inclusion significantly shrinks the uncertainty envelope in its vicinity. The refinement to the older side of the age model is a simple consequence of superpositional constraints based on the maximum depositional age constraint at the sandstone horizon. The refinement on the younger side of the age model is related to the interaction of the sedimentation model prior and the full set of conditioning ages, namely the tendency of the prior to minimize changes in accumulation rate, thus lowering the probability of paths that seek the younger end of the maximum depositional age likelihood. This effect is further illustrated in the inset of Figure 7, which superposes the starting likelihood with the posterior probability for the age of the Ti-I-neg8.5 horizon.
Table 2 documents the results of the age model for specific horizons of interest in the Tiout section. Stratigraphic levels are reported with respect to a base at the position of the Tiout 566 volcanic ash bed in the upper Lie de vin Formation. The first occurrence of trilobite fragments in the upper portion of the informal lower member of the Igoudine Formation is dated as 519.95 + 0.43/− 0.40 Ma, while the first identifiable trilobite occurrence in the lower Tiout Member defines the base of the Hupetina antiqua Zone, dated as 519.78 + 0.26/− 0.37 Ma. The Hupetina antiqua through Daguinaspis zonal succession that defines the Issendalenian Stage is quantitatively calibrated with this age model to range up to 518.43 + 0.25/− 0.69 Ma. Individual zones span approximately 0.2 Ma, with the exception of the longer Fallotaspis plana Zone (0.7 Ma). The first appearance of archaeocyaths 15 m above the base of the Tiout Member of the Igoudine Formation is dated at 519.71 + 0.26/− 0.35 Ma.
Table 2. Bayesian age model results for the Tiout section, southern Morocco

5. Discussion
5.a. Interregional biostratigraphic correlation of the Tiout Member
The most certain, albeit tentative, correlation of the Tiout Member faunas is with the similar West Gondwanan assemblages of the Ovetian Stage in southern Spain (i.e. the Ossa-Morena zone). Here, the joint lowest occurrence of archaeocyaths (Zone I of the composite archaeocyath zonation of Perejón, Reference Perejón1986, Reference Perejón1994) and trilobites (bigotinids) has been used to define the base of the Spanish Ovetian Stage (Liñán et al. Reference Liñán, Perejón and Sdzuy1993). As at Tiout, the lowest occurrence of these macrofossils lies at a facies change into more carbonate-rich, shallow, higher energy shelf facies in Spain (Liñán et al. Reference Liñán, Perejón and Sdzuy1993). Perejón’s (Reference Perejón1986) succession of 11 lower Cambrian archaeocyath zones is more finely divided than the Moroccan archaeocyath succession.
However, the Spanish archaeocyath zonation is a composite succession developed from localities across Spain. The zonation shows little vertical change in taxonomic composition, with most taxa appearing low in the ‘succession’ and persisting to the top. Geyer & Landing (Reference Geyer and Landing2004, p. 183) suggested that key differences through the Spanish zonation reflect collecting bias with genera ‘appearing’ and ‘disappearing’ with changes in diversity of the successive assemblages. The lower six or so Spanish archaeocyath zones of the Ovetian have been broadly correlated with the West Gondwanan Issendalenian Stage as developed in Morocco (Debrenne & Debrenne, Reference Debrenne and Debrenne1995; Zhuravlev, Reference Zhuravlev1995; Geyer & Landing, Reference Geyer and Landing2004). Unfortunately, the relatively low-diversity Ovetian trilobite faunas feature shallow-water genera that do not allow a precise correlation into the Issendalenian of Morocco (Geyer & Landing, Reference Geyer and Landing2004). It is possible that the abrupt appearances of archaeocyaths and trilobites in Iberia and southern Morocco reflect deepening by a combination of roughly coeval epeirogenic/eustatic changes on the Moroccan–Iberian margin of the West Gondwanan shelf, which would be a development similar to the linked lithofacies and faunal changes in the lower–middle Cambrian boundary interval of southern Morocco and northern Iberia (Landing et al. Reference Landing, Geyer and Heldmaier2006).
One of the anchors claimed to supply a confident correlation between the Spanish Ovetian horizons with the Moroccan Anti-Atlas and a claimed very early age of the lower Ovetian is the occurrence of Lemdadella in both regions (i.e. Liñán & Sdzuy, Reference Sdzuy1978). The latter authors assigned Lemdadella tioutensis to the F. tazemmourtensis Zone and used the species for a correlation with the Lemdadella linaresae-bearing strata in the Pedroche Formation of the Córdoba area, southern Spain. Another taxon assigned to Lemdadella (i.e. L. perejoni) was later described from strata overlying those with L. linaresae (Liñán et al. Reference Liñán, Gámez Vintaned and Gozalo2008a ).
Lemdadella may be very geographically widespread in Gondwana, and another species, L. antarctica, is recorded from the Shackleton Limestone in the central Transantarctic Mountains (Palmer & Rowell, Reference Palmer and Rowell1995). However, careful restudy is needed to confirm this generic assignment.
Arguments for using Lemdadella tioutensis, or Lemdadella in general, for relatively precise correlation of the Iberian archaeocyath zone III into the F. tazemmourtensis (now F. plana) Zone (e.g. Liñán & Sdzuy, Reference Sdzuy1978; Liñán et al. Reference Liñán, Gozalo, Dies Álvarez, Gámez Vintaned and Zamora2008b ) remain unconvincing as these forms are lithofacies-associated taxa and include named species that are very restricted palaeogeographically. In addition, the locally abundant species L. linaresae, as well as L. perejoni, from the Iberian Ossa–Morena zone show distinct differences from Moroccan L. tioutensis and probably represent a different genus (G. Geyer, unpublished data). Later collection of bigotinid trilobites below the Lemdadella linaresae Zone in Iberia and the resultant suggestion of three more trilobite zones below it are based on monospecific occurrences of trilobites in Iberian archaeocyath Zones I–III. A recent description of the monospecific fauna with Lunolenus antiquus (Sdzuy, Reference Sdzuy1961) in the La Herrería Formation at Barrios de Luna in the Cantabrian Mountains and its tentative correlation with the lowest Ovetian archaeocyath zones (Liñán et al. Reference Liñán, Gozalo, Dies Álvarez, Gámez Vintaned and Zamora2008b ) is based on arguments that may be questioned.
Intercontinental correlations of the lowest faunas of the West Gondwanan Issendalenian Stage have emphasized archaeocyath-based correlations that have led to contradictory correlation into the lower Cambrian strata of SE Siberia. By one proposal (e.g. Debrenne & Debrenne, Reference Debrenne and Debrenne1995; Zhuravlev, Reference Zhuravlev1995), the Moroccan lower archaeocyath interval (Erismacoscinus fasciola – Retecoscinus minutus Zone) and the entire Tiout Member and lower Issendalenian Stage are equated with the lowest archaeocyaths (Retecoscinus zegebarti Zone) that define the base of the Siberian Atdabanian Stage. Alternatively, the E. fasciola – R. minutus Zone has been claimed to correlate with the upper Atdabanian Stage (Rozanov & Debrenne, Reference Rozanov and Debrenne1974; Magaritz et al. Reference Magaritz, Kirschvink, Latham, Zhuravlev and Rozanov1991).
Confusingly, the overlying Moroccan Erismacoscinus marocanus Zone, as well as the upper Issendalenian Stage, Amouslek Formation and traditional Fallotaspis tazemmourtensis – Choubertella – Daguinaspis zones have also been generally equated with the middle–upper Atdabanian Stage (Debrenne & Debrenne, Reference Debrenne and Debrenne1995; Zhuravlev, Reference Zhuravlev1995). A reason for this may be seen in the relatively small changes in the composition of the assemblages that preclude definition of biostratigraphically distinct zones. The problem with correlation of the lowest Moroccan archaeocyaths with those that define the base of the Atdabanian Stage is that the lowest appearances of Siberian Retecoscinus zegebarti Zone archaeocyaths and the oldest Atdabanian trilobites (Profallotaspis jakutensis Zone) are similar to the Moroccan and Spanish lowest trilobites and archaeocyaths in that all these taxa appear at a distinct lithofacies change.
As discussed by Landing et al. (Reference Landing, Geyer, Brasier and Bowring2013), 35 m of reddish mudstones and carbonates on the Lena River are assigned in many reports (e.g. Varlamov et al. Reference Varlamov, Rozanov, Khomentovsky, Shabanov, Abaimova, Demidenko, Karlova, Korovnikov, Luchinina, Malakhovskaya, Parkhaev, Pegel, Skorlotova, Sundukov, Sukhov, Fedorov and Kipriyanova2008) to the upper Tommotian Stage and Dokidocyathus lenaicus Zone, even though no archaeocyaths or other macrofossils are present. This interval is abruptly succeeded by strata with thin planar thrombolites with the reappearance of archaeocyaths (R. zegebarti Zone) and lowest known trilobites (Profallotaspis jakutensis Zone) 2.6 m higher (e.g. Varlamov et al. Reference Varlamov, Rozanov, Khomentovsky, Shabanov, Abaimova, Demidenko, Karlova, Korovnikov, Luchinina, Malakhovskaya, Parkhaev, Pegel, Skorlotova, Sundukov, Sukhov, Fedorov and Kipriyanova2008, figs 30, 34). The Retecoscinus zones of southern Morocco and SE Siberia can therefore only be considered to be similar in taxonomic (generic) composition, but a confident geochronologic correlation is not possible as the earliest record of archaeocyaths and trilobites of both regions corresponds to the lithofacies changes in the Moroccan and Siberian successions, and the prior record of both biotic groups is absent.
5.b. Carbon isotope-based global correlation of upper lower Cambrian System
A resolution of the interregional correlation of the oldest Moroccan archaeocyaths and identifiable trilobites seems possible with δ13C chemostratigraphy. Early reconnaissance work at Tiout by Tucker (Reference Tucker1986) and Kirschvink et al. (Reference Kirschvink, Magaritz, Ripperdan, Zhuravlev and Rozanov1991) demonstrated a positive δ13C excursion that peaks slightly below the middle of the traditional ‘Calcaire supérieur’ (middle of the lower member of the Igoudine Formation) and c. 130 m below the base of the Tiout Member (Kirschvink et al. Reference Kirschvink, Magaritz, Ripperdan, Zhuravlev and Rozanov1991, fig. 3). This positive shift in the lower Igoudine Formation seems to be present in the more closely spaced samples at the nearby Oued Sdas section (Fig. 1) as indicated by increasing δ13C values to the top of the sampled section (Maloof et al. Reference Maloof, Schrag, Crowley and Bowring2005, fig. 2). Correlation of the δ13C chemostratigraphy between the Tiout and Oued Sdas sections is confirmed in this study by ash beds in the two sections dated to the same age within the margin of error (Tiout 566 at 521.06 ± 0.12 Ma and M236 at 520.93 ± 0.14 Ma in the Lie de vin Formation). The calculated age of the strong, positive δ13C excursion higher in the Tiout succession in the lower Igoudine Formation is 520.27 + 0.59/− 0.57 Ma (Fig. 7).
The distinct δ13C positive excursion at Tiout and Oued Sdas has been correlated with the cycle IV excursion in the lower but not lowermost Atdabanian Stage (i.e. within the Repinaella Zone) in Siberia (Brasier & Cowie, Reference Brasier, Cowie, Cowie and Brasier1989; Kirschvink et al. Reference Kirschvink, Magaritz, Ripperdan, Zhuravlev and Rozanov1991; Maloof et al. Reference Maloof, Schrag, Crowley and Bowring2005, Reference Maloof, Porter, More, Dudás, Bowring, Higgins, Fike and Eddy2010; Landing et al. Reference Landing, Geyer, Brasier and Bowring2013). This correlation, along with equation of the strong negative δ13C excursion in the upper Adoudou Formation with the lowest Tommotian Stage of Siberia (Brasier et al. Reference Brasier, Magaritz, Corfield, Luo, Wu, Lin, Jiang, Hamdi, He and Fraser1991; Magaritz et al. Reference Magaritz, Kirschvink, Latham, Zhuravlev and Rozanov1991; Maloof et al. Reference Maloof, Schrag, Crowley and Bowring2005, Reference Maloof, Porter, More, Dudás, Bowring, Higgins, Fike and Eddy2010), would mean that the oldest archaeocyaths and identifiable trilobites in the Tiout Member are younger than the Siberian lower Atdabanian IV carbon excursion. In that case, the two ‘Retecoscinus zones’ in Morocco and Siberia are only similar in generic composition but cannot be considered to be coeval, while the Profallotaspis jakutensis Zone would be older than the Hupetina antiqua Zone.
5.c. Appearance of the oldest trilobites
As noted above (Section 2.e), the oldest trilobites and archaeocyaths have abrupt lowest occurrences in the lower Cambrian strata of southern Morocco at lithofacies changes with appearance of higher-energy, less-restricted marine conditions. The known lowest occurrence of identifiable trilobites such as Hupetina, Bigotina and Eofallotaspis may therefore reflect a preservational artefact and not an evolutionary event. More generally, Landing et al. (Reference Landing, Geyer, Brasier and Bowring2013, also Geyer & Landing, Reference Geyer and Landing2018) argued that the lowest occurrence of early Cambrian trilobites should not be equated with a correlatable evolutionary first appearance datum (FAD) and used to define the base of a global chronostratigraphic Series 2 of the Cambrian (fide Peng & Babcock, Reference Peng and Babcock2005, Reference Peng and Babcock2011; i.e. their ‘FAD of trilobites’). Indeed, the lowest local occurrences of the earliest trilobites overlie unconformities (sometimes cryptic) or may be controlled by the development of favourable habitats/lithofacies for colonization (e.g. southern Morocco, southern Spain, SE Siberian Platform), preservational windows or thoroughness of collecting (Landing et al. Reference Landing, Geyer, Brasier and Bowring2013). In addition, unidentifiable trilobite fragments occur in the lower part of the underlying lower member of the Igoudine Member, which means the simple presence of trilobite fossils may not be a biostratigraphic aid. The biostratigraphic utility of trilobites in sub-Tiout Member strata is therefore limited by preservational artefacts.
Fallotaspidids and bigotinids are elements of early trilobite assemblages, but their species are provincial; the mere presence of one of their genera (as ‘Fallotaspis’ in the White-Inyo Mountains, SW Laurentia) does not provide a basis for a highly precise correlation. Indeed, Fallotaspis has a long stratigraphic range in Morocco where it appears as high as the Issafen Formation (Geyer, Reference Geyer1996; Fig. 2). There has been a desire to apply the early fallotaspidid-bearing zonal nomenclature of Morocco to sections worldwide, which led to erroneous identification of archaeaspidids as fallotaspidids (e.g. Nelson & Hupé, Reference Nelson and Hupé1964; Khomentovsky & Repina, Reference Khomentovsky and Repina1965; Nelson, Reference Nelson1978; Lieberman, Reference Lieberman2001). An unfortunate example of this inappropriate use of the Moroccan trilobite zonation in successions where it should not be applied is Rushton et al.’s (Reference Rushton, Brück, Molyneux, Williams and Woodcock2011) recognition of the Eofallotaspis Zone and a so-called ‘Fallotaspis Zone’ as the oldest trilobite zones in Avalonian Britain, even ‘though no trilobites definitive of these [endemic] zones are reliably known in British rocks’ (Rushton et al. Reference Rushton, Brück, Molyneux, Williams and Woodcock2011, p. 6).
Our new age model provides a high-resolution calibration of the trilobite zones of the Issendalenian Stage. As noted above (sections 2.e, 2.g), the lowest Fallotaspis plana (horizon T14) is used to assign the upper range of Eofallotaspis tioutensis to the F. plana Zone. Volcanic ashes at and above the base of the Amouslek with ages of 519.23 ± 0.14 Ma and 518.99 ± 0.14 Ma lie within this F. plana Zone and approximate the marine transgression across the Tiout–Amouslek hiatus. A volcanic ash dated at 518.59 ± 0.20 Ma just below the Daguinaspis Zone at Timoulaye Izder can be confidently correlated to the Tiout section, and thus constrains the timing and duration of the Choubertella to Daguinaspis Zones to between 518.73 and 518.43 Ma.
This age model assists in global correlation of the highly provincial early Cambrian trilobite faunas. These Choubertella to Daguinaspis Zone ages are significantly older than the 517.22 ± 0.31 Ma zircon date on a thin ash on the inner platform of Avalon (Landing, Reference Landing, Nance and Thompson1996) from the lower Callavia Zone at Nuneaton, Warwickshire (Williams et al. Reference Williams, Rushton, Cook, Zalasiewicz, Martin, Condon and Winrow2013). The Warwickshire Callavia Zone date is arithmetically identical to a legacy date of 517 ± 1.5 Ma from the Antatlasia guttapluviae Zone in the Lemdad Formation in the High Atlas (Landing et al. Reference Landing, Davidek, Westrop, Geyer and Heldmaier1998; Fig. 2). The perspective here is that Callavia had a surprisingly long range in Avalonia from 517.22 ± 0.31 Ma (Williams et al. Reference Williams, Rushton, Cook, Zalasiewicz, Martin, Condon and Winrow2013) up to a date of 514.45 ± 0.36 Ma on the Green Callavia Sandstone at Comley, Shropshire (Harvey et al. Reference Harvey, Williams, Condon, Wilby, Siveter, Rushton, Leng and Gabbott2011).
The unidentified trilobite remains in the basal Caerfai Bay Formation at Caerfai Bay, South Wales (Harkless & Hicks, Reference Harkless and Hicks1871; Landing et al. Reference Landing, Geyer, Brasier and Bowring2013) have an age of c. 519 ± 1 Ma (Landing et al. Reference Landing, Davidek, Westrop, Geyer and Heldmaier1998, Reference Landing, Geyer, Brasier and Bowring2013). This is comparable to a 519.30 ± 0.23 Ma zircon age from a fault-isolated block of the lowest Caerfai Bay at nearby Cwm Bach (Harvey et al. Reference Harvey, Williams, Condon, Wilby, Siveter, Rushton, Leng and Gabbott2011). These c. 519 Ma ages in South Wales are on trilobite remains that may be the oldest in Avalonia. The ages correspond closely to the 519.95 + 0.45/− 0.39 Ma age for the oldest sclerites in Morocco, and the 520.28 + 0.51/− 0.44 Ma carbon isotope excursion peak in the lower Igoudine Formation (Fig. 7) that is equated with the peak of the IV carbon isotope excursion in the lower, but not lowermost, trilobite-bearing strata of Siberia (Landing et al. Reference Landing, Geyer, Brasier and Bowring2013; Landing & Kouchinsky, Reference Landing and Kouchinsky2016). If the IV excursion peak at Zhurinsky Mys on the Lena River was used as the base of the upper lower Cambrian global Series 2/Lenaldanian Series, as proposed by Landing et al. (Reference Landing, Geyer, Brasier and Bowring2013), then the lithofacies-change-associated oldest trilobites in Siberia (Profallotaspis jakutensis Zone) would likely only be somewhat older, perhaps c. 520 Ma. This definition of the base of Series 2/Lenaldanian Series would allow interregional correlation of the IV excursion of Siberia, into South China (ZHUCE excursion) and, on the basis of this strong carbon excursion, into Avalonia and West Gondwana (lower Igoudine Formation).
Interregional comparison of the oldest identifiable trilobites (based on mineralized sclerites) through radioisotopic geochronology and carbon isotope stratigraphy serves to emphasize that quite distinct taxa comprise the oldest trilobite assemblages on Cambrian palaeocontinents. This means that an unrecorded interval of trilobite origination and diversification preceded the local appearance of the ‘oldest’ mineralized trilobites, with local assemblages comprising de facto trilobite faunal provinces (e.g. Fortey et al. Reference Fortey, Briggs and Wills1996; Landing et al. Reference Landing, Geyer, Brasier and Bowring2013). Taxonomic differences between the lowest-occurring forms include archaeaspidids and bigotinids on the Siberian Platform and western Laurentia, fallotaspidids s.s. and bigotinids in West Gondwana (Morocco and Iberia), seemingly significantly younger olenelloids representing endemic genera in southern Baltica and Avalonia, and redlichioids in East Gondwana (South China and Australia).
Very different dates have been proposed for the origin of trilobites as a crown group of euarthropods based on palaeobiogeographical analyses of differences between the oldest local trilobite assemblages. A Cryogenian origin of c. 750 Ma was proposed by Fortey et al. (Reference Fortey, Briggs and Wills1996), while Meert & Lieberman (Reference Meert and Lieberman2004, Reference Meert and Lieberman2008; also Álvaro et al. Reference Álvaro, Alhberg, Babcock, Bordonaro, Choi, Cooper, Ergaliev, Gapp, Pour, Hughes, Jago, Korovnikov, Laurie, Liebetrman, Patterson, Pegel, Popov, Rushton, Sukhov, Tortello, Zhou, Źyłinska, Harper and Servais2013) suggested an early–late, but not latest, Ediacaran age bracket of c. 600–550 Ma. These dates are far older and cannot be reconciled with the body or trace fossil record of trilobites. The oldest arthropod-produced, trace fossils include Rusophycus and Cruziana in the Avalonian Rusophycus avalonensis Ichnofossil Zone and in coeval intervals globally (e.g. review and references in Geyer & Landing, Reference Geyer, Landing, Brasier, McIlroy and McLoughlin2017). The upper Rusophycus avalonensis Zone has a c. 530 Ma U–Pb legacy age determined in Avalonian New Brunswick (Landing et al. Reference Landing, Davidek, Westrop, Geyer and Heldmaier1998). Ichnofossils from the R. avalonensis Zone precede the oldest known mineralized trilobite remains at c. 520 Ma as suggested here on the basis of interregional correlation of the Siberian IV carbon isotope excursion. There is an obvious morphologic similarity of traces from the R. avalonensis Zone and coeval intervals to later, likely trilobite-produced, rusophyciform and cruzianaform traces in marine Phanerozoic rocks. This similarity suggests their formation by arthropods that, but for their apparent skeletal nonmineralization, would be trilobites. If so, then the possible 530 Ma or somewhat earlier initial appearance and diversification of trilobites would fall in the later part of the Cambrian Evolutionary Radiation, and not the Ediacaran Evolutionary Radiation (i.e. Landing et al. Reference Landing, Antcliffe, Geyer, Kouchinsky, Andreas and Bowser2018).
5.d. Trilobites and lower Cambrian chronostratigraphy
As discussed above, a ‘FAD of trilobites’ should not be considered as a biostratigraphic criterion to define the base of a global upper lower Cambrian Series 2 (e.g. Peng & Babcock, Reference Peng and Babcock2005, Reference Peng and Babcock2011), as all known trilobite first occurrences in the lower Cambrian strata appear above stratigraphic discontinuities defined by unconformities or lithofacies changes. This problem led Landing et al. (Reference Landing, Geyer, Brasier and Bowring2013) to propose that the base of Cambrian Series 2, or their proposed Lenaldanian Series, should be defined at the Zhurinsky Mys section on the Lena River in Siberia. The proposed level is somewhat above the lowest local trilobite occurrence (Profallotaspis jakutensis Zone) and at the peak of the IV carbon isotope excursion within the Repinaella Zone. This would allow interregional correlation of the base of a Series 2/Lenaldanian Series by the IV excursion of Siberia, into South China (ZHUCE excursion) and, on the basis of this strong carbon excursion, into Avalonia and West Gondwana (lower Igoudine Formation). The calculated age of the peak of this excursion in the lower Igoudine Formation is c. 520.27 + 0.59/− 0.57 Ma, and this age would apply to the Siberian IV excursion (Maloof et al. Reference Maloof, Porter, More, Dudás, Bowring, Higgins, Fike and Eddy2010). In turn, this c. 520.3 Ma date would only be slightly younger than that of the oldest mineralized trilobites of the Profallotaspis jakutensis Zone that appear c. 22 m lower than the IV peak and 2.6 m above a lithofacies break in Siberia (Varlamov et al. Reference Varlamov, Rozanov, Khomentovsky, Shabanov, Abaimova, Demidenko, Karlova, Korovnikov, Luchinina, Malakhovskaya, Parkhaev, Pegel, Skorlotova, Sundukov, Sukhov, Fedorov and Kipriyanova2008). In lieu of a Siberian ash date, a c. 521 Ma date might be estimated for the oldest Siberian trilobites.
Landing et al. (Reference Landing, Geyer, Brasier and Bowring2013) discussed how this proposed definition for the informal Series 2 base would put the oldest known mineralized trilobite remains into the Terreneuvian Series. However, as discussed above, the lowest records of mineralized trilobites lie globally at a stratigraphic discontinuity and do not preserve the early origin and diversification of the group. Definition of a global stratigraphic section and point (GSSP) for the base of Cambrian Series 2 based on a taxon purportedly corresponding to the ‘FAD of trilobites’ means that subsequent recovery of this taxon or another older than the GSSP, which is likely given the undefined confidence interval of fossil taxa (e.g. Marshall, Reference Marshall1990), would demonstrate the limitations of the GSSP. Indeed, the presence of Rusophycus and Cruziana traces earlier than c. 530 Ma that suggests an origin of nonmineralized trilobites during early Terreneuvian time further means that the recovery of mineralized remains is possible in the c. 530–520 Ma interval.
5.e. Palaeogeographic consequences of new U–Pb dates
The revised and new U–Pb zircon dates from the Moroccan lower Cambrian strata provide additional information pertinent to reconstructing the palaeogeography of Avalonia and West Gondwana. The most common palaeogeographic reconstruction shows Avalonia very close to or coterminous with the tropical NW African (Moroccan) margin of West Gondwana through the Cambrian Period (e.g. Álvaro et al. Reference Álvaro, Alhberg, Babcock, Bordonaro, Choi, Cooper, Ergaliev, Gapp, Pour, Hughes, Jago, Korovnikov, Laurie, Liebetrman, Patterson, Pegel, Popov, Rushton, Sukhov, Tortello, Zhou, Źyłinska, Harper and Servais2013, fig. 19.1; Murphy et al. Reference Murphy, Nance, Keppie, Dostal, Wilson, Houseman, McCaffrey, Dore and Buiter2018, fig. 5B). Alternatively, Avalonia was an insular microcontinent separate from Gondwana (with early Cambrian fallotaspidid s.s.- and archaeocyath-bearing faunas in a tropical carbonate platform succession) by the end of the Ediacaran Period (e.g. Landing, Reference Landing, Nance and Thompson1996, Reference Landing2005; Keppie & Keppie, Reference Keppie and Keppie2014). Coeval successions in Avalonia, by contrast, have endemic Lower Cambrian faunas in a cool-water shale-platform sequence (with olenelloids, but without archaeocyaths) (Landing, Reference Landing, Nance and Thompson1996, Reference Landing2005; Landing & Westrop, Reference Landing, Westrop, Lipps and Waggoner2004; Landing et al. Reference Landing, Geyer, Brasier and Bowring2013, Reference Landing, Myrow, Narbonne, Geyer, Bautois, Mángano, Kaufman, Westrop, Kröger, Liang and Gougain2017).
The 519–517 Ma U–Pb zircon dates are useful to emphasize conclusions that coeval successions in Avalonia and Moroccan West Gondwana are lithologically and biotically distinctive (see also Landing et al. Reference Landing, Geyer, Brasier and Bowring2013). The two regions cannot be placed in a palaeogeographic reconstruction in which the two areas are relatively adjacent and only separated by several hundred kilometres) (e.g. Álvaro et al. Reference Álvaro, Alhberg, Babcock, Bordonaro, Choi, Cooper, Ergaliev, Gapp, Pour, Hughes, Jago, Korovnikov, Laurie, Liebetrman, Patterson, Pegel, Popov, Rushton, Sukhov, Tortello, Zhou, Źyłinska, Harper and Servais2013; Murphy et al. Reference Murphy, Nance, Keppie, Dostal, Wilson, Houseman, McCaffrey, Dore and Buiter2018), and lie in a comparable palaeolatitude (and climatic) setting, but do not have common faunas or biotas through the early Cambrian Period. Álvaro et al. (Reference Álvaro, Alhberg, Babcock, Bordonaro, Choi, Cooper, Ergaliev, Gapp, Pour, Hughes, Jago, Korovnikov, Laurie, Liebetrman, Patterson, Pegel, Popov, Rushton, Sukhov, Tortello, Zhou, Źyłinska, Harper and Servais2013, p. 282, fig. 19.1) stated that Avalonia was too far south to have tropical archaeocyaths like Morocco, but showed an ‘adjacent’ Morocco and Avalonia in their reconstruction at a ‘non-tropical’ 60° S. They also seem to deny existence of a regional carbonate platform in early Cambrian Morocco (contra Geyer & Landing, Reference Geyer and Landing1995, Reference Geyer, Landing, Geyer and Landing2006a , b) by noting a local carbonate-shale interval in a small transtensional basin in the western Atlas. They also noted that ‘fenestral fabrics’ in biological build-ups show a tropical location of Avalonia. However, it must be emphasized that fenestral fabrics are not documented in Avalonian Cambrian successions (e.g. Monninger, Reference Monninger1979). Furthermore, the purported palaeolatitudinal significance of fenestrae claimed by Álvaro et al. (Reference Álvaro, Alhberg, Babcock, Bordonaro, Choi, Cooper, Ergaliev, Gapp, Pour, Hughes, Jago, Korovnikov, Laurie, Liebetrman, Patterson, Pegel, Popov, Rushton, Sukhov, Tortello, Zhou, Źyłinska, Harper and Servais2013) in microbial build-ups is incorrect, as fenestrae occur even in lacustrine build-ups in modern, high-temperate New York (e.g. Eggleston & Dean, Reference Eggleston and Dean1976). A simple picture of coeval mudstone-dominated and carbonate platform successions in Avalonia and Morocco hopefully emphasizes that Avalonia and West Gondwana were not adjacent during the early Cambrian Period (Landing et al. Reference Landing, Geyer, Brasier and Bowring2013, fig. 3).
The new and revised U–Pb dates and Bayesian model for lower Cambrian Morocco also help reconstruct the region’s palaeogeographic setting. One implication of the dates is the very rapid early Cambrian subsidence of the Souss Basin. Sample Tiout 566 from the upper Lie de vin Formation and sample Ti-Am-0.0 from an ash at the sequence boundary defined by the base of the Amouslek Formation bracket a carbonate-dominated, 600-m-thick platform succession. The rate of accumulation of compacted carbonate rock (c. 220 m Ma–1) shown by the Tiout section is among the highest for carbonate platforms through the Phanerozoic Eon (e.g. Bosscher & Schlager, Reference Bosscher and Schlager1993). This indicates that carbonate sediment aggradation kept up with rapid, likely transtensional rift subsidence (Landing et al. Reference Landing, Geyer and Heldmaier2006) to maintain shallow-marine facies.
A likely change of epeirogenic subsidence and sea-level change rates may have led to the transition to less restricted marine-shelf conditions and the appearance of the oldest archaeocyaths and identifiable trilobite assemblages at about the base of the Tiout Member. Changes in either rates of subsidence or sea-level rise or both led to formation of the sequence boundary at the top of the carbonate platform (top of Tiout Member) and seemingly the end of the carbonate platform and its replacement by the mixed siliciclastic-carbonate shelf of the Amouslek Formation. An alternative explanation of this carbonate–mixed-siliciclastic-carbonate shelf transition may be the onset of a southwards movement of the West Gondwanan margin into cooler latitudes during the late early Cambrian Period (Landing & Westrop, Reference Landing, Westrop, Lipps and Waggoner2004; Landing et al. Reference Landing, Geyer, Brasier and Bowring2013).
6. Conclusions
New precise U–Pb zircon dates from the Tiout and Timoulaye Izder sections in southern Morocco allow a number of conclusions about the early history of trilobites and archaeocyaths, as well as early Cambrian palaeogeography. Bayesian age modelling constrains the age of the peak of a strong positive carbon isotope excursion in the lower member of the Igoudine Formation to 520.27 + 0.59/− 0.57 Ma. This date on the lower Igoudine Formation excursion is very important as the Moroccan excursion is correlated with the IV excursion in the lower Atdabanian Stage (Repinaella Zone).
Our age model (Fig. 7) shows that the lowest occurrence of trilobite fragments in the lower member of the Igoudine Formation can be dated as 519.95 + 0.43/− 0.40 Ma. This is younger than an estimated c. 521 Ma age of the oldest Siberian trilobites, while the first identifiable trilobite occurrence defining the base of the Hupetina antiqua Zone is even younger at 519.78 + 0.26/− 0.37 Ma.
This report demonstrates not only that the lowest occurrence of identifiable West Gondwanan (Moroccan) trilobites lies at a stratigraphic discontinuity (i.e. establishment of less restricted marine facies), but also shows that the global diachrony of the oldest trilobites argued by Landing et al. (Reference Landing, Geyer, Brasier and Bowring2013) can now be numerically calculated; the lowest fragmentary Moroccan fauna is at least c. 0.5 Ma younger than the estimated age of the lowest Siberian assemblage. This diachrony also extends to other clades, as the estimated maximum age on the oldest Tommotian Stage archaeocyaths in Siberia is c. 525.5 Ma (Maloof et al. Reference Maloof, Schrag, Crowley and Bowring2005, Reference Maloof, Porter, More, Dudás, Bowring, Higgins, Fike and Eddy2010), while new U–Pb dates demonstrate a much younger appearance of the lowest archaeocyaths in the Tiout Member at 519.71 + 0.26/− 0.35 Ma.
After the appearance of mineralized trilobites at an estimated c. 521 Ma, quite rapid changes in traditional (mineralized) trilobites took place. U–Pb dates from southern Morocco quantify those rapid changes through time of the oldest trilobite faunas. The Hupetina antique – Eofallotaspis tioutensis – Choubertella – Daguinaspis zones succeeded each other in the relatively brief time interval of < 1.5 Ma during c. 519.78 to 518.43 Ma (Fig. 7), with a distinct change of faunal composition.
U–Pb dates from the upper Lie de vin Formation (sample Tiout 566, c. 521.06 Ma) to the lower Amouslek Formation (sample Ti-Am-34, c. 518.99 Ma) demonstrate that c. 634 m of rock was deposited in c. 3 Ma, yielding an average rate of accumulation of compacted rock of slightly more than 220 m Ma–1. Finally, c. 519–517 Ma dates from Morocco and Avalonia further emphasize the distinctiveness of coeval biotas and lithofacies of the West Gondwanan (Moroccan) carbonate platform and Avalonian siliciclastic shelf. Although commonly illustrated as adjacent or even coterminous, Avalonia existed as an insular continent by the late Ediacaran Period and was latitudinally separate from the West Gondwanan margin through the early Cambrian Period as shown by the distinctive lithofacies and biotas of the two regions.
Acknowledgments
Funding for the analytical infrastructure of the Boise State Isotope Geology Laboratory was provided by the NSF Major Research Instrumentation grants EAR-0521221 and EAR-1337887, and NSF EAR Instrumentation and Facilities Program grant EAR-0824974. Funds from the New York State Museum assisted in sample analysis. Much of EL’s field and laboratory work was carried out under National Science Foundation grant support while at the New York State Museum. GG’s field work was supported by research grant GE549/13-1 of the Deutsche Forschungsgemeinschaft, andthe preparation of the manuscript was made possible by research grant GE549/22-1. Two anonymous reviewers and Fred Sundberg are thanked for helpful critiques.
Supplementary material
To view supplementary material for this article, please visit https://doi.org/10.1017/S0016756820000369