INTRODUCTION
Understanding how interactions among organisms affect the patterns observed in a community is key to comprehend its dynamics and functioning (Raffaelli & Hawkins, Reference Raffaelli and Hawkins1996; Bertness et al., Reference Bertness, Gaines and Hay2001). For example, in many rocky intertidal habitats, the high diversity of sessile organisms is allowed by the pressure exerted by starfish on competitively superior prey species that would otherwise monopolize the space (e.g. Paine, Reference Paine1974). In analogous way, subtidal barren areas with low diversity of sessile organisms and dominated by encrusting corallines can be maintained by sea urchins that graze all foliose macroalgae (Lawrence, Reference Lawrence1975; Vance, Reference Vance1979). These examples refer to keystone, single consumer species that can strongly impact prey abundance, controlling most community patterns and processes (Power et al., Reference Power, Tilman, Estes, Menge, Bond, Mills, Daily, Castilla, Lubchenco and Paine1996; Duffy, Reference Duffy2002). However, most systems are inhabited by more than one consumer species that can interact with each other, resulting in varying effects in the structure of the community (e.g. Duffy, Reference Duffy2002).
As already noted, the maintenance of barren areas has been generally attributed to intense grazing by sea urchins (e.g. Lawrence, Reference Lawrence1975; Vance, Reference Vance1979). However, the consequences of the individual foraging activities of the interacting species that inhabit the barren area may vary due to differences in their temporal and spatial patterns of distribution, feeding preferences and behaviour (Branch & Branch, Reference Branch and Branch1980; Schmitt, Reference Schmitt1996; Bulleri et al., Reference Bulleri, Benedetti-Cecchi and Cinelli1999). In general, coexisting gastropod grazers have subtle effects on the crustose barrens when compared with sea urchins, since the former cannot deal with the growth of large foliose macroalgae (Moreno & Sutherland, Reference Moreno and Sutherland1982; Fletcher, Reference Fletcher1987). In addition, gastropods may require the presence of sea urchins to impede large macroalgae from swamping the areas of crustose corallines creating unsuitable conditions (Underwood & Jernakoff, Reference Underwood and Jernakoff1981; Fletcher, Reference Fletcher1987).
In moderately protected rocky shores of central Peru (~11°S), the sea urchin Tetrapygus niger (Molina, 1782) and the gastropod Tegula atra (Lesson, 1830) are the most abundant grazers of the low intertidal zone (Paredes, Reference Paredes1974), where the substrate is covered mainly by crustose corallines. In the south-eastern Pacific, most research on the effect of these grazers on macroalgal assemblages have been conducted in shallow subtidal areas south of 18°S in Chile. For example, in northern and central Chile, Tetrapygus niger and T. tridentata maintain extensive barren grounds in the shallow subtidal zone by impeding the settling of macroalgal propagules (Vásquez, Reference Vásquez1993, Reference Vásquez and Lawrence2001). In the boundary between the intertidal and subtidal zones of central Chile, field experiments showed that T. niger can impair the colonization of the substrate by foliose macroalgae after the removal of the canopy forming, competitively dominant kelp Lessonia nigrescens (Ojeda & Santelices, Reference Ojeda and Santelices1984). In southern Chile, Tegula atra can remove ephemeral algae in shallow subtidal areas where sea urchins are not present, although this effect is temporary since the perennial kelp Macrocystis pyrifera cannot be controlled by T. atra and overgrow algae (Moreno & Sutherland, Reference Moreno and Sutherland1982). Thus, it seems that Tetrapygus niger is important in limiting the development of macroalgae in the low intertidal and shallow subtidal zones, while Tegula atra would have minor effects. However, no work has been conducted to explore and compare their effects when they coexist. Therefore, the main objective of this work was to evaluate and compare the grazing effects of Tetrapygus niger and Tegula atra in the low rocky intertidal zone of central Peru. In addition, we also analysed their distribution patterns and diet in order to account for differences in the use of habitat and prey resources.
MATERIALS AND METHODS
Study site
The study was conducted from October 2005 to December 2007 in the low intertidal zone of a moderately protected rocky shore south of the Ancón bay (central Peru, 11°46′S, 77°12′W). The site is located on the eastern side of an island located ~70 m from the coast, and despite the fact that it is sheltered from severe wave action, currents can be intense and waves can reach up to 2 m high on stormy days. Tides are semidiurnal with a maximum range from −0.17 to 1.21 m with respect to Chart Datum (Dirección de Hidrografía y Navegación—Perú, 2006). The low intertidal zone is delimited by the upper limit of distribution of the barnacle Megabalanus psittacus and the lower limit of spring low tides (i.e. the infralittoral fringe; sensu Paredes, Reference Paredes1974). At the study site, the substrate is mostly occupied by encrusting coralline algae (probably Mesophyllum sp. and other calcareous crusts; Meneses, Reference Meneses1993), while other sessile invertebrates and algae are scarce (F. Hidalgo, personal observation).
Organism cover in the low intertidal zone
General descriptive data of organisms' cover in the study area were obtained by sampling the low intertidal zone with randomly placed quadrats (0.25 × 0.25 m; N = 30) in October 2005 and May 2007. Samplings were conducted during low tide and the cover of sessile organisms was estimated by the point intercept method (81 points).
Distribution patterns of Tetrapygus niger and Tegula atra
We used two procedures to evaluate the distribution patterns of Tetrapygus niger and Tegula atra in the low intertidal zone. The first one was conducted by recording the number of individuals present in permanent 50 × 50 cm quadrats during low tide. Quadrats were marked with epoxy putty approximately in the centre of eight bedrock areas, ~2 m2 each, separated from each other by cracks or pools. Four of these areas had an average slope <30% and were referred to as ‘horizontal’ surfaces, the other four had an average slope >60% and were referred to as ‘vertical’ surfaces. These contrasting slope surfaces were selected to account for possible inter-specific differences in microhabitat distribution that may result from the interaction among competition, food preferences or different vulnerability to predation or wave dislodgement (e.g. Hahn & Denny, Reference Hahn and Denny1989; Bulleri et al., Reference Bulleri, Benedetti-Cecchi and Cinelli1999; Rochette & Grand, Reference Rochette and Grand2004; Cobb & Lawrence, Reference Cobb and Lawrence2005; Espinosa et al., Reference Espinosa, Guerra-García, Fa and García-Gómez2006). To account for between-days variations in the abundance of T. atra and Tetrapygus niger, counts were repeated seven times at intervals of 5–7 days. The number of individuals of each species was log-transformed to meet parametric assumptions and was compared among days of sampling and surface inclinations with two-way ANOVAs (Zar, Reference Zar1999). Here and thereafter, when interactions between factors were found, the multiple comparisons Tukey HSD test was used to identify how factors interacted (Zar, Reference Zar1999).
The second sampling procedure accounted for differences in the abundance of T. niger and Tegula atra in the low intertidal zone in relation to the tidal cycle. For this, the tidal cycle was divided in to three intervals: ‘low tide, from 1 h before to 1 h after low tide (i.e. the low intertidal zone exposed, but washed by waves and splash); ‘high tide’, from 1 h before to 1 h after high tide (i.e. the low intertidal zone completely underwater); and ‘mid tide’, from 2 h before to 4 h after both low and high tides (i.e. the low intertidal zone partially submersed during flooding and ebbing, respectively). Samplings were done with quadrats (25 × 25 cm) randomly placed on ‘vertical’ (slope >60%) and ‘horizontal’ (slope <30%) rock surfaces. Because counts were always conducted in the low intertidal zone and some areas were not accessible during high and mid tide, a variable number of replicates (30–100) were used depending on the tide interval and substrate slope considered. The size of individuals sampled was also recorded (T. atra: shell diameter; Tetrapygus niger: test diameter). Data were compared among surface inclinations and tide intervals with two-way ANOVAs (Zar, Reference Zar1999). Counts data were log-transformed to meet parametric assumptions (Zar, Reference Zar1999). To determine the type of distribution of each species at each tide interval and on each surface inclination, we calculated the variance/mean quotient (Margalef, Reference Margalef1974; see Espinosa et al. Reference Espinosa, Guerra-García, Fa and García-Gómez2006). With the distribution data obtained in the quadrats of 25 × 25 cm during low tide, we evaluated if there was any relationship between the abundance of T. niger and Tegula atra using a Spearman's rank correlation analysis (Zar, Reference Zar1999). In addition, the spatial segregation between T. atra and Tetrapygus niger within the quadrats was analysed with nearest neighbour contingency tables (Pielou, Reference Pielou1961; see Branch & Branch, Reference Branch and Branch1980), by measuring the distances between each individual and the closest neighbour in each quadrat. Segregation between species was evaluated by comparing the observed and expected distributions with chi-square statistics (Pielou, Reference Pielou1961). To determine the degree of segregation, the coefficient of segregation (S) was calculated (Pielou, Reference Pielou1961).
Diet analysis
Gut content analyses were conducted to assess the diet of Tegula atra and Tetrapygus niger. To analyse gut content, 30 average-size individuals of each species were collected in the low intertidal zone and fixed in the field with 10% formaldehyde diluted in seawater. In the laboratory, guts were dissected and food items consumed were identified under a dissection microscope. Dietary composition was assessed by mean occurrence percentage (%O) of each prey taxon (Hyslop, Reference Hyslop1980). As a complement of gut content analyses, isotopic analyses of each consumer were conducted. Stable isotopic signatures reflect long-term (weeks–years) diet composition (Hobson, Reference Hobson1999), whereas stomach contents analysis indicates intake over the previous few hours. For the analysis, 20 individuals of either Tetrapygus niger and Tegula atra were collected in the low intertidal zone and kept alive until processing within 12 h after collection. Each sample (T. niger, N = 4; T. atra, N = 5) was a pool of muscle tissue of 4–5 individuals. Muscle was extracted from the Aristotle's lantern in Tetrapygus niger and from the foot in Tegula atra. Samples were then dried at 60°C for 48 h, milled to a fine powder and packed into tin capsules for isotope analysis. All samples were analysed for δ13C and δ15N at the Stable Isotope Facility of the University of California (Davis), using a PDZ Europa ANCA-GLS elemental analyser interfaced to a PDZ Europa 20-20 isotope ratio mass spectrometer. Differences in δ13C and δ15N between Tetrapygus niger and Tegula atra were compared with t-tests (Zar, Reference Zar1999).
Grazing effects
The grazing effects of Tetrapygus niger and Tegula atra were evaluated using caging field experiments. Experiments were conducted from November 2005 to March 2006, and repeated from April to October 2006. During the first period, the experimental design encompassed five treatments (N = eight replicates per treatment), in order to analyse overall and individual effects: (1) inclusions of T. atra; (2) inclusions of Tetrapygus niger; (3) exclusion cages without herbivores to control for grazing effects; (4) control cages to controlling caging artefacts; and (5) total controls. Cages (length × width × height = 20 × 20 × 5 cm) were built with stainless steel wire mesh (mesh size = 5 mm) and were fixed to the substratum with a central bolt; control cages were of the same size but with two opposite sides open to allow access to grazers; and total controls were areas (20 × 20 cm) marked in the substratum with epoxy putty in the corners. In the inclusions, we used two individuals of the average field sizes per cage (sea urchins, 20 mm in test diameter; snails, 18 mm in shell diameter) to match average natural densities. Cages were inspected regularly to maintain experimental levels of grazers in the respective treatments. No animals were found dead or missing in the inclusions throughout the experiment. In the first experimental period, we measured the percentage cover of the main sessile organisms with the point intercept method (100 points) at the end of the experimental period (i.e. after 4 months). One-way ANOVAs (Zar, Reference Zar1999) were then used to compare the cover of sessile organisms among treatments. During the second experimental period we also evaluated grazing effects at early successional stages by measuring the percentage cover of sessile organisms at days 10, 20, 35, 80 and 180 since the start of the experiment. Given that no cage artefacts were observed during the first experimental period, and that the cover within inclusions of T. niger resemble that of the controls (see Results), control cages and total controls were not used in the second period. Repeated measures ANOVAs (Crowder & Hand, Reference Crowder and Hand1990) were then used to compare the cover of sessile organisms among treatments (i.e. inclusions of Tegula atra, inclusions of Tetrapygus niger and exclusions) and dates. Percentage cover data were square root transformed to meet parametric assumptions (Zar, Reference Zar1999).
RESULTS
Organisms cover in the low intertidal zone
The encrusting coralline algae occupied >80% of the substrate in the low intertidal zone, while the percentage cover of other sessile organisms was <5%, the barnacle Megabalanus psittacus and the anemone Phymactics clematis being the most common (Figure 1).

Fig. 1. Percentage cover (mean + SE) of sessile organisms and bare rock in the low intertidal zone. No corticated algae, Ulva sp. and Semimytilus algosus were found in the sampling of October 2005, indicated by a zero.
Distribution patterns of Tetrapygus niger and Tegula atra
Samplings in permanent 50 × 50 cm quadrats during low tide showed that both Tegula atra and Tetrapygus niger were more abundant on vertical than horizontal rock surfaces (T. atra: F 6, 42 = 4.27, P = 0.045; T. niger: F 6, 42 = 8.23, P = 0.006; Figure 2), and that their abundance varied along the days of sampling (T. atra: F 6, 42 = 5.12, P = 0.0005; T. niger: F 6, 42 = 3.38, P = 0.008; Figure 2). The samplings with 25 × 25 cm quadrats revealed that the higher abundance on vertical surfaces was consistent throughout the tidal cycle for T. atra (F 1, 404 = 9.72, P = 0.002; Figure 3), which was also more abundant during high and mid tide than at low tide (F 2, 404 = 8.70, P < 0.001; Tukey HSD test, P < 0.001 for each contrast; Figure 3). In contrast, this sampling showed no statistical differences in the abundance of T. niger between surface inclinations (F 1, 404 = 0.03, P = 0.87; Figure 3) or among tide intervals (F 2, 404 = 2.20, P = 0.11; Figure 3). Sizes of T. niger individuals were not different between surface inclinations or tide intervals (mean, SD = 19.74, 5.86 cm; two-way ANOVA; surface: F 1, 411 = 0.48, P = 0.49; tide: F 2, 411 = 2.21, P = 0.11). For T. atra, slightly larger individuals were present at high (mean, SD = 17.11, 4.14 cm) and mid tide (mean, SD = 16.81, 4.03 cm) than at low tide (mean, SD = 15.91, 4.56 cm), without differences between vertical (mean, SD = 16.62, 4.26 cm) and horizontal surfaces (mean, SD = 16.61, 4.31 cm; two-way ANOVA; surface: F 1, 558 = 0.001, P = 0.97; tide: F 2, 558 = 3.17, P = 0.04). In all cases, both species showed an aggregated pattern of distribution (variance/mean quotient ranging from 2.04 to 17.10, P < 0.05 in all cases; according to the scattergraph of Margalef (Reference Margalef1974)).
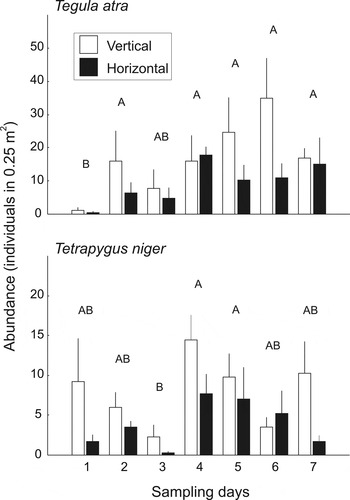
Fig. 2. Abundance (mean + SE) of Tegula atra and Tetrapygus niger in the permanent marked plots of 50 × 50 cm on vertical and horizontal rock surfaces at different days of sampling. Different upper case letters indicate differences in the abundance of each species among days of sampling. Individuals were always more abundant on vertical surfaces (for the sake of clarity, this is not presented with letters).

Fig. 3. Abundance (mean + SE) of Tegula atra and Tetrapygus niger during high, mid and low tide on vertical and horizontal rock surfaces. Different lower case and upper case letters indicate differences in the abundance of each species between surface inclinations and among tide intervals, respectively.
The abundance of Tegula atra and Tetrapygus niger was positively correlated on horizontal surfaces (r = 0.44, P = 0.02), but no relationship was observed on vertical surfaces (r = 0.17, P = 0.42). However, nearest neighbour analysis showed that the two species were positively segregated within the sampling units (i.e. 25 × 25 cm) on both surface inclinations (horizontal: χ 2 = 67.33, P < 0.0001, S = 0.429; vertical: χ 2 = 91.62, P < 0.0001, S = 0.450), with interspecific nearest neighbours occurring less often than expected under conditions of random occupancy.
Diet analysis
Both gut content and isotopic analyses revealed striking differences between the diet of Tetrapygus niger and Tegula atra. Gut content analysis showed that all Tetrapygus niger stomachs contained microalgae, and most had also macroalgae (mainly Gelidium spp., Ulva spp. and red filamentous macroalgae). Conversely, while most Tegula atra stomachs had microalgae, just a small proportion contained macroalgal remains. Mussel recruits were also found in stomachs of both Tetrapygus niger (53.33%O) and Tegula atra (6.67%O, Table 1). In Tetrapygus niger, shell fragments and crustaceans remains were also present in a large proportion of the stomachs analysed; one crab carapace was also found in a Tegula atra stomach (Table 1). Isotopic signature of N (δ15N‰) was higher in Tetrapygus niger (mean, SD = 13.85, 0.36) than in Tegula atra (mean, SD = 13.05, 0.19; t = 4.29, df = 7, P = 0.003) and the isotopic signature of C (δ13C‰) was smaller in Tetrapygus niger (mean, SD = −16.10, 0.19) than in Tegula atra (mean, SD = −15.25, 0.13; t = −7.88, df 7, P = 0.0001).
Table 1. Occurrence percentage (%O) of alimentary items in Tetrapygus niger and Tegula atra stomachs. %O is the number of stomachs containing that alimentary item divided by the total number of stomachs containing food × 100.

a, including diatoms and macroalgae sporelings.
Grazing effects
The experiment to evaluate grazing effects showed that Tetrapygus niger was able to keep the crustose surface free of other sessile organisms, while Tegula atra could not impede the colonization of the substrate by the mussel Semimytilus algosus. At the end of the first experimental period, the crustose coralline surface remained uncovered by other sessile organisms in the inclusions of Tetrapygus niger and in controls (both control cages and total controls), while it was completely covered by S. algosus in the inclusions of Tegula atra and in the exclusions (one-way ANOVAs; for Semimytilus algosus: F 4, 25 = 749.73, P < 0.001; for crustose corallines: F 4, 25 = 183.08, P < 0.001; Figure 4).

Fig. 4. Percentage cover (mean + SE) of the mussel Semimytilus algosus and calcareous crustose macroalgae within inclusions of Tegula atra (Ta), inclusions of Tetrapygus niger (Tn), exclusions (E), control cages (CC) and total controls (TC) at the end of the first experimental period. Lower case and upper case letters indicate differences in cover of mussel and crustose algae, respectively, among treatments.
The samplings during the second experimental period revealed an increased cover of microalgae and Ulva spp. in the exclusions at day 20 since experiment starting (repeated measures ANOVA, date × treatment interaction; microalgae: F 8, 60 = 2.25, P < 0.05; Ulva spp.: F 8, 60 = 2.49, P < 0.05, Figure 5), while the cover of the sessile polychaete Phragmatopoma moerchi was higher in the inclusions of T. atra than in the other treatments at days 20 and 35 (repeated measures ANOVA, date × treatment interaction, F 6, 45 = 3.26, P < 0.01, Figure 5). After day 35 since experiment starting, the cover of microalgae, Ulva spp. and P. moerchi in the exclusions and inclusions of T. atra was swamped by the increasing cover of S. algosus, which reached ~100% cover in these treatments at day 80, remaining ~0% in the inclusions of Tetrapygus niger (repeated measures ANOVA, date × treatment interaction: F 8, 60 = 7.26, P < 0.0001; Figure 5). The cover of crustose corallines decreased through time in the exclusions and inclusions of Tegula atra, remaining ~80–90% in the inclusions of Tetrapygus niger (repeated measures ANOVA, date × treatment interaction, F 8, 60 = 10.66, P < 0.001, Figure 5).

Fig. 5. Percentage cover (mean ± SE) of Semimytilus algosus, microalgae, green ephemeral macroalgae, the sessile polychaete Phragmatopoma moerchi, and crustose coralline within exclusions, inclusions of Tegula atra and inclusions of Tetrapygus niger at days 10, 20, 35, 80 and 180 after beginning the second experimental period.
DISCUSSION
Tetrapygus niger and Tegula atra are the most abundant grazers in the low intertidal zone of protected rocky coasts of central Peru, and our results showed that at these areas Tetrapygus niger prevents the fast colonization of the crustose surface by the mussel Semimytilus algosus, while Tegula atra is unable to do so, according with most previous works reporting strong effects of sea urchins and more subtle effects of gastropod grazers in barren grounds (e.g. Fletcher, Reference Fletcher1987).
Semimytilus algosus is the dominant space holder at mid intertidal areas of central Peru (Paredes, Reference Paredes1974; Tokeshi & Romero, Reference Tokeshi and Romero1995). This is a fast recruiting, fast growing mussel whose abundance is controlled by predation of the starfish Heliaster helianthus, though it has little effect on mussels' colonization of bare rock during succession, probably because grazers negatively affect recruitment due to bulldozing or crushing the young settlers (Hidalgo et al., Reference Hidalgo, Firstater, Lomovasky and Iribarne2011). In the low intertidal, S. algosus was never found directly on the primary substratum, but on refuges such as fronds of macroalgae growing as epiphyte of the barnacle Megaloalanus psittacus (F. Hidalgo, personal observation), probably because there they escape grazing. Previous studies in Chile showed that grazing by Tetrapygus niger can be intense, generating halos in beds of intertidal pool benthic algae (Contreras & Castilla, Reference Contreras and Castilla1987) and impeding the colonization of the substrate by foliose and corticated macroalgae in the intertidal–subtidal boundary (Ojeda & Santelices, Reference Ojeda and Santelices1984). Our comparison of the effects of the most abundant grazers indicates that unlike what occurs in Chile regarding the interaction between sea urchins and algae (e.g. Ojeda & Santelices, Reference Ojeda and Santelices1984; Vásquez, Reference Vásquez1993, Reference Vásquez and Lawrence2001), S. algosus in Peru has the potential to fast colonize the substrata in the low intertidal zone and overgrowth macroalgae when T. niger is absent.
Different feeding capabilities of benthic herbivores may play a central role in determining the different patterns of distribution and abundances of their prey resources (see Branch & Branch, Reference Branch and Branch1980; Steneck & Watling, Reference Steneck and Watling1982; Schmitt, Reference Schmitt1996). In our case, the strong effect of urchins may be related to their coarse-grained method of grazing over the substratum, with which they can bulldoze the small underlying invertebrates (see Day & Branch, Reference Day and Branch2002). In contrast, the effects of Tegula atra were comparatively negligible, since it just reduced the abundance of microalgae and green ephemeral macroalgae during early succession, being unable to impede the fast colonization of the substrate by S. algosus. The presence of S. algosus recruits in the gut content of both grazers indicates that they can potentially affect mussels directly by consumption; however, the feeding apparatus of T. atra would impede them to ingest mussels once they have attained a size beyond which they can escape grazing by snails (see Wahl & Hoppe, Reference Wahl and Hoppe2002), and this is reflected in the comparatively lower number of T. atra stomachs containing mussels. Furthermore, the presence of shell fragments of mussels in the stomachs of Tetrapygus niger indicates that urchins can also break the valve of larger individuals with the teeth of the lantern, or passively swallow valve pieces when feeding on macroalgae. It should be noted here that we are assuming that the effects of T. niger and Tegula atra on community structure act in additive manner, with T. atra having negligible effects. The positive effects of T. atra on Phragmatopoma moerchi may be related to the selective elimination of microalgae and green macroalgae that may compete with P. moerchi settlers for substrate colonization.
The differential use of resources such as habitat and food can allow the coexistence of species sharing the same habitat (e.g. Branch & Branch, Reference Branch and Branch1980; Vásquez et al., Reference Vásquez, Castilla and Santelices1984; Cobb & Lawrence, Reference Cobb and Lawrence2005). In this regard, we did not find strong evidence of habitat segregation between Tetrapygus niger and Tegula atra at the temporal and spatial scales investigated here. However, gut content analysis did reveal differences in their diets. Microalgae were the main food item present in T. atra stomachs, while most Tetrapygus niger stomachs had also filamentous, foliose and corticated macroalgae. The rhipidoglossan radula of Tegula atra is mainly adapted to graze filamentous and microscopic algal forms (see Reyes et al., Reference Reyes, Córdova, Romero and Paredes2001), while the Aristotle's lantern of Tetrapygus niger makes it able to consume benthic foliose and corticated macroalgae (see Contreras & Castilla, Reference Contreras and Castilla1987), supporting our results. While it seems from this analysis that Tegula atra and Tetrapygus niger are functionally redundant in terms of consuming microalgae, both species have different feeding mechanisms and this may affect the mode the food is taken. Tegula atra would actually feed on microalgae, while Tetrapygus niger would ingest them accidentally as epiphytes growing on other benthic algae. The differences in the gut contents were also sustained by the carbon and nitrogen isotopes signatures for T. niger and Tegula atra. The difference in carbon isotope signature (~1‰) might reflect the different energy sources of both consumers, while a similar difference in nitrogen isotope signature (~1‰) is insufficient to separate both species in different trophic positions (consumers from different trophic levels tend to be nitrogen enriched by ~3‰ per trophic level; Lajtha & Michener, Reference Lajtha and Michener2007).
In summary, we can argue that Tetrapygus niger plays a key role in this low intertidal community. Through directly limiting S. algosus to monopolize the substratum, T. niger can indirectly reduce the diversity of mussel associated species, including recruits of commercially valuable species such as Fissurella spp., which cannot persist outside mussel matrices (Tokeshi & Romero, Reference Tokeshi and Romero1995). Conversely, through controlling primary space holders, sea urchins can also benefit other grazers, including Tegula atra, and filter-feeders that live on bare substrate (see Tokeshi & Romero, Reference Tokeshi and Romero1995), by creating a suitable area for feeding or living (e.g. Branch & Branch, Reference Branch and Branch1980; Ayling, Reference Ayling1981; Steneck & Watling, Reference Steneck and Watling1982).
ACKNOWLEDGEMENTS
We want to express our gratitude to the colleagues and friends of the Universidad Nacional Mayor de San Marcos, especially to J. Tarazona, E. Ramos, P. Gallegos and A. Gamarra, for providing us with their laboratory and logistics and field assistance during our work in Peru. We also thank Marina de Guerra del Perú for allowing us access to the study sites, and G. Alvarez and F. Botto for their help with the isotopic analysis. The manuscript has been improved with the comments of one anonymous referee.
FINANCIAL SUPPORT
This study was conducted and financed in the frame of the EU-project CENSOR (Climate variability and El Niño Southern Oscillation: impacts for natural resources and management, Contract 511071).