1. Introduction
We study here the impact of deformation partitioning that occurs in response to oblique plate convergence in the sense of Fitch (Reference Fitch1972), and strain partitioning triggered by mechanical stratigraphy of the lithosphere (e.g. Boyer & Elliott, Reference Boyer and Elliott1982) enhanced by coupling between long-term deformation and surface processes. Our experiments combined with observations from the active Taiwan orogen allow exploration of the mechanisms of deformation partitioning in mountain belts. Firstly, the effects of common rheologic layering of the lithosphere (brittle and ductile behaviour of crustal layers, décollements, including structural inheritance). Horizontal layering and inherited structures play a major role in the vertical deformation plane, favouring strain localization and partitioning. During crustal deformation, strong coupling between tectonics (deformation and its kinematics) and surface processes (the way mass is transferred from reliefs to basins) induces specific mechanical behaviours characterized by deformation partitioning (e.g. Dahlstrom, Reference Dahlstrom1969; Boyer & Elliott, Reference Boyer and Elliott1982; Perez-Estaun et al. Reference Perez-Estaun, Martinez Catalan and Bastida1991; Malavieille, Reference Malavieille2010). Secondly, oblique subduction and map geometry of continental margins (shape of continental or oceanic indenters) induce strain partitioning. The kinematics of oblique convergence are resolved by subduction for the trench-normal component and by localized strike-slip faulting for the trench-parallel component (e.g. Fitch, Reference Fitch1972).
Fossil and presently active mountain belts have been extensively studied worldwide through different approaches, from which numerous sets of studies and data allow for 3D characterization of their general structure, deformation and tectonic evolution. Scientific literature is rich in publications where analogue or numerical models of accretionary wedges are compared to natural mountain chains (e.g., among many others, Davis et al. Reference Davis, Suppe and Dahlen1983; Lu & Malavieille, Reference Lu and Malavieille1994; Chen et al. Reference Chen, Chan, Lo, Malavieille, Lu, Tang and Lee2018 for the Taiwan orogen; Brandon, Reference Brandon, van der Pluijm and Marshak2004 for the Cascadia wedge; Beaumont et al. Reference Beaumont, Ellis, Hamilton and Fullsack1996; Pfiffner et al. Reference Pfiffner, Ellis and Beaumont2000; Bonnet et al. Reference Bonnet, Malavieille and Mosar2007 for the Alps; Harris, Reference Harris, Brown and Ryan2011 for the Banda Arc–continent collision; Stockmal et al. Reference Stockmal, Beaumont, Nguyen, Lee, Sears, Harms and Evenchick2007; Konstantinovskaya & Malavieille, Reference Konstantinovskaya and Malavieille2011 for the Canadian Rocky Mountains, etc.). Based on the results of previous studies as well as on the results of our own observations from the Taiwan orogenic belt, and of our analogue experiments, we examine and discuss the key relationships between deformation partitioning, tectonics, structural inheritance and surface processes at different time scales within the framework of the regional geodynamics of the Taiwan collision zone.
2. Deformation partitioning in mountain belts: causes and consequences?
2.a. Rheological layering of the lithosphere
During the subduction of a continental margin under a continental or oceanic upper-plate lithosphere, the crust of the downgoing plate is deformed and thickened, leading to the development of an orogenic wedge (e.g. Dewey & Bird, Reference Dewey and Bird1970). The deformation is largely controlled by the rheology and vertical organization of subducted lithosphere, crustal materials and burial conditions (e.g. Kusznir & Park, Reference Kusznir and Park1984; Kusznir et al. Reference Kusznir, Vita-Finzi, Whitmarsh, England, Bott, Govers, Cartwright and Murrell1991; Ranalli, Reference Ranalli, Burg and Ford1997). The continental crust presents variable subhorizontal layering characterized by rock composition and rheology (brittle and ductile behaviour of crustal layers, crust–mantle interface, basement–sediment interface). The effects of such layering are numerous and significantly control crustal deformation involving vertical variations in deformation regime and strain localization (décollements, heterogeneous deformation). Changes occur at different levels in relation to the mechanical structure of the continental lithosphere: strong upper mantle, low-viscosity lower crust, ductile middle crust and brittle upper crust. A similar control exists in the sedimentary cover generally composed of layered sequences of various lithologies and rheology (Willis, Reference Willis1894) coined ‘mechanical stratigraphy’ (e.g. Laubach et al. Reference Laubach, Olson and Gross2009; Ferrill et al. Reference Ferrill, Morris, McGinnis, Smart, Wigginton and Hill2017). This mechanical stratigraphy means that distinct compositional changes (e.g. from clay-supported to grain-supported clastic rocks) in vertically and laterally equivalent deposits result in highly partitioned deformation and strain (Cawood & Bond, Reference Cawood and Bond2018). As most mountain belts form owing to the subduction of a continental margin (Dilek & Moores, Reference Dilek and Moores1999; Dilek, Reference Dilek, Dilek and Pavlides2006), the extensional history of this margin is crucial (e.g. Manatschal & Bernoulli, Reference Manatschal and Bernoulli1999) because it determines its behaviour during subduction and orogenesis. Structures (faults, folds, previous tectonic fabrics, magmatic bodies, etc.) inherited from former extensional or contractional deformation events influence deformation, structural style and evolution of a mountain belt.
Deformation partitioning is a major consequence of crustal heterogeneity. Its structural expression is characterized by specific geometries and kinematics of the tectonic units involved in orogenic wedges. The critical wedge theory (Davis et al. Reference Davis, Suppe and Dahlen1983; Dahlen et al. Reference Dahlen, Suppe and Davis1984; Dahlen, Reference Dahlen1984) is useful to describe the geometry and the state of stress of a fold-and-thrust belt at a whole-wedge scale. According to this theory, the taper of a wedge is related to the mechanical properties of the materials constituting the wedge and to the shear stress on the basal décollement. It also accounts for wedge mechanics assuming that crustal materials behave homogeneously, which is generally not the case. If wedge material is homogeneous, the nature of deformation can be simple and characterized by a forward migration of faults, deformation and wedge growth. If heterogeneities are present in the incoming crustal sequences, deformation is more complex owing to strain partitioning at different scales.
2.b. Impact of surface processes on deformation partitioning
Surface processes represent a first-order parameter that controls deformation partitioning in orogens. Crustal thickening induces the development of mountainous topographies that are subsequently eroded owing to climatic forcing (Fig. 1a). Associated mass transfer has a significant impact on the evolution of tectonic structures. Three different aspects of mass transfer can be analysed in relation to deformation partitioning: (1) the response of topography to internal strain partitioning and feedback effects on crustal structure, exhumation and long-term evolution of the wedge; (2) development of syn-convergent normal faults in the wedge; (3) the impact on the location of major seismogenic faults in active mountain belts.

Fig. 1. (a) Simplified cross-section showing the main features and processes involved in mountain building during continental subduction. (b) Photos of analogue model (initial and final stage) depicting the impact of erosion, sedimentation and structural inheritance of a subducted continental margin on deformation partitioning. The structure of the model margin accounts for rheological layering of the sedimentary cover and for the presence of inherited normal faults (see details of experimental procedure in Bonnet et al. Reference Bonnet, Malavieille and Mosar2008). U.P. – upper plate; L. P. – lower plate; BDT – brittle/ductile transition.
Coupling between internal and external processes is fundamental to explain deformation partitioning and strain localization in orogenic wedges. Topographic slope angle is mostly controlled by the vertical component of deformation, which is directly related to internal deformation mechanisms. An orogenic wedge grows by successive accretion of crustal material (accretionary flux), and is moderated by erosion (erosional flux) that transports removed material far from the growing topography by rivers (Barr & Dahlen, Reference Barr and Dahlen1989; Dahlen & Barr, Reference Dahlen and Barr1989; Barr et al. Reference Barr, Dahlen and McPhail1991). This process of mass balancing controls the growth of the orogen through variation of climatic forcing, becoming wider or narrower depending on the ratio of accretion to erosion (e.g. Willett et al. Reference Willett, Schlunegger and Picotti2006). Slope angle is the first-order parameter that determines the efficiency of erosion. The higher the slope angle is, the higher the erosion efficiency becomes. This implies that strong erosion takes place in those domains of the wedge where basal accretion is active, because accretionary vertical growth produces high topographic slopes. The portions of the wedge overlying the underplated duplex units are uplifted with little shortening, since many structural horses are stacked below them (Silver et al. Reference Silver, Ellis, Breen and Shipley1985). As a direct consequence, exhumation is favoured in these high-slope domains, producing specific features such as exhumed antiformal stacks composed of structurally deeper tectonic units incorporated earlier into the wedge by basal accretion (e.g. Malavieille, Reference Malavieille2010). This accretion mechanism, which is favoured by deep décollement zones, completely changes wedge behaviour, fault development and exhumation during shortening.
The location and depth of décollements control the shape and thickness of the underplated sequences and consequently determine the hinterland structure, geometry, kinematics and tectonic evolution of the orogenic wedge. Thus, basal accretion determines the location of domains of underplating and subsequent exhumation. Analysis of model wedges has shown that exhumation conditions depend on the internal dynamics of thrust wedges and, conversely, on how this dynamic is modified by erosion and sedimentation (Fig. 1b). Different décollement levels located at different depths in an incoming sequence produce multiple domains of basal accretion localized in different places in a wedge (e.g. Konstantinovskaya & Malavieille, Reference Konstantinovskaya and Malavieille2011), as demonstrated from many mountain belts (e.g. Malavieille, Reference Malavieille2010).
2.c. Subduction kinematics, 3D geometry and structure of continental margins: effects of oblique convergence, shape of indenters and inherited structures
Deformation partitioning does not occur only in 2D (in a vertical plane) owing to mechanical layering of the continental crust. Subduction kinematics (e.g. Chemenda et al. Reference Chemenda, Lallemand and Bokun2000; Platt, Reference Platt2000), 3D geometry and the structure of continental margins (e.g. Marshak, Reference Marshak and McClay2004) strongly impact the deformation field at a regional scale. It has been shown that the shape of continental domains involved in subduction or collision controls the shape, deformation regime and evolution of orogens (e.g. for the Alps, Tapponnier, Reference Tapponnier1977; the Himalayas, Tibet, Tapponnier & Molnar, Reference Tapponier and Molnar1977; Taiwan, Lu & Malavieille, Reference Lu and Malavieille1994; Chang et al. Reference Chang, Angelier and Lu2007; Brown et al. Reference Brown, Alvarez-Marron, Biete, Hao, Camanni and Ho2017; the Banda Arc, Roosmawati & Harris, Reference Roosmawati and Harris2009; the Quebec Appalachians, Konstantinovskaya et al. Reference Konstantinovskaya, Rodriguez, Kirkwood, Harris and Thériault2009; or other wedges, Calassou et al. Reference Calassou, Larroque and Malavieille1993). Indeed, the continent–ocean boundary geometry and its orientation relative to the subduction zone define the timing, geometry and structural evolution of the resulting mountain belt.
3. The Taiwan orogenic belt as a case study
3.a. Geology of Taiwan
The Taiwan orogen is now considered as an archetype of arc–continent collision-induced mountain building. It has developed as a result of the convergence between the Eurasia (EP) and the Philippine Sea (PSP) plates in a complex 3D subduction setting during late Cenozoic time (Fig. 2). Around the island, this setting varies from south to north allowing a general overview of the major tectonic processes associated with the subduction of the EP from pure oceanic subduction to continental subduction, mountain belt formation and subsequent collapse (Teng, Reference Teng1996; Malavieille et al. Reference Malavieille, Lallemand, Dominguez, Deschamps, Lu, Liu, Schnuürle, Byrne and Liu2002; Chang et al. Reference Chang, Angelier, Lee and Huang2003). The mountain belt can be described as a doubly vergent orogenic wedge, formed by accretion of the Eurasian margin continental crust leading to the building up of the very high topography of the island (e.g. Suppe, Reference Suppe1981; Willett et al. Reference Willett, Beaumont and Fullsack1993). Global kinematics (Seno, Reference Seno1977; Seno et al. Reference Seno, Stein and Grip1993) indicate that the PSP is currently moving northwestward (N306° ± 1° azimuth) with respect to the EP at a convergence rate ranging between 80 and 83 mm yr−1 (GPS data, Yu et al. Reference Yu, Chen and Kuo1997; Lallemand & Liu, Reference Lallemand and Liu1998) or 90 mm yr−1 (REVEL model, Sella et al. Reference Sella, Dixon and Mao2002). This kinematics promotes a strong and fast deformation of the lithosphere of the two convergent plates, which in turn is strongly influenced by the major impact of climate-dependent surface processes controlling the balance between tectonic accretion and mass transfer via erosion (Willett et al. Reference Willett, Slingerland and Hovius2001; Willett & Brandon, Reference Willett and Brandon2002)
The Taiwan orogenic wedge is composed of four main tectono-stratigraphic units (Fig. 3). From west to east, they are (Ho, Reference Ho1986): (1) the Foreland Basin and Western Foothills (WF). The Western Foothills correspond to a fold-and-thrust belt deformation of Oligo-Miocene strata overlain by a 4 km thick sequence of Pliocene–Quaternary molasse deposits (Lu & Hsü, Reference Lu and Hsü1992). This foreland thrust belt is inactive north of 24° N, but it is still growing south of this latitude. (2) The Slate Belt (SB) includes the Hsuehshan Range units (HsR) and the Backbone Range slates (BR). (3) The Central Range (CR) is composed of metamorphosed Backbone Range strata dated to the Eocene and Miocene (but not Oligocene) and the Tananao Complex (TC) composed of pre-Tertiary metamorphic rocks of the Chinese continental margin incorporated early in the orogenic wedge (Beyssac et al. Reference Beyssac, Negro, Simoes, Chan and Chen2008). West of the Central Range, the Lishan–Laonung Fault is presently a high-angle W-dipping backthrust (Lee et al. Reference Lee, Angelier and Chu1997), which separates the western Backbone Range from the Eocene–Oligocene units of the Hsuehshan Range (Ho, Reference Ho1986; Angelier et al. Reference Angelier, Bergerat, Chu and Lee1990; Teng et al. Reference Teng, Wang, Tang, Huang, Huang, Yu and Ke1991; Clark et al. Reference Clark, Fisher, Lu and Chen1993; Tillman & Byrne, Reference Tillman and Byrne1995). The Tananao Complex has a long polymetamorphic history. By contrast, the Cenozoic units within the Slate Belt have only experienced very low-grade Cenozoic metamorphism (Ernst & Jahn, Reference Ernst and Jahn1987; Faure et al. Reference Faure, Lu and Chu1991; Beyssac et al. Reference Beyssac, Simoes, Avouac, Farley, Chen, Chan and Goffe2007). The Central Range is bounded to the east by the Longitudinal Valley (LV), which marks the present-day plate boundary and suture zone. (4) East of the Longitudinal Valley, the Coastal Range (CoR) represents remnants of the northernmost segment of the Luzon volcanic arc. The Coastal Range is mainly composed of volcanic rocks and melanges related to deformation and docking of part of the PSP (Page & Suppe, Reference Page and Suppe1981).

Fig. 3. Tectonic map of Taiwan and surroundings. Eurasia Plate: (1) Asian continental margin and foreland basin; (2) oceanic crust of the South China Sea; (3) Western Foothills (WF) and Kaoping Slope (Miocene–Pleistocene series of the syn-collision orogenic wedge); (4) pre-collision oceanic accretionary wedge; (5) underthrust Palaeogene sequences of the Eurasian continental margin; (6) extended continental crust (Okinawa back-arc basin); (7) uplifted core of the Miocene–Pliocene pre-collision oceanic accretionary wedge; (8) Eocene series of the Eurasian continental margin; (9) pre-Tertiary metamorphic rocks of the Eurasian continental margin; (10) exhumed HP rocks of the Yuli belt; (11) Pleistocene–Holocene sediments of the Southern Longitudinal Trough and Longitudinal Valley orogenic basins. Philippine Sea Plate: (12) fore-arc basins and deformed fore-arc sediments of the Huatung Ridge (HtR); (13) accreted volcanic edifices and fore-arc sediments of the Luzon arc; (14) Luzon volcanic arc; (15) oceanic crust of the Philippine Sea Plate; (16) Ryukyu accretionary wedge. Tectonic units: WF – Western Foothills; HsR – Hsuehshan Range; LF – Lishan–Laonung Fault; TC – Tananao Complex; CR – Central Range; CoR – Coastal Range; CP – Coastal Plain; BR – Backbone Range; SLT – Southern Longitudinal Trough; HP – Hengchun Peninsula; HtR – Huatung Ridge; TT – Taitung Trough; KS – Kaoping Slope; MAW – Manila Accretionary Wedge; OCT – ocean–continent transition.
Metamorphic petrology of rocks from the orogenic wedge characterizes a general eastward increase in metamorphic grade (e.g. Ernst & Jahn, Reference Ernst and Jahn1987), from diagenetic conditions in the Western Foothills to the upper-greenschist facies in the Tananao Complex. Significant lateral and vertical displacements along some of the major faults bounding these tectonic units have produced major gaps in the metamorphic grade and deformation intensity from west to east across the mountain belt.
3.b. Tectonic evolution of Taiwan during oblique convergence
Oblique convergence is responsible for the special shape and evolution of the orogen that, by moving from south to north, allows the study of the different orogenic processes linked with the transition from oceanic to continental subduction (e.g. Malavieille et al. Reference Malavieille, Lallemand, Dominguez, Deschamps, Lu, Liu, Schnuürle, Byrne and Liu2002; McIntosh et al. Reference McIntosh, Nakamura, Wang, Shih, Chen and Liu2005). The obliquity of the plate convergence (e.g. Suppe, Reference Suppe1984) involves the progressive subduction of the continental margin of Eurasia inducing the growth of the Taiwan mountain belt (Figs 2, 3). In addition, coupling between surface processes and tectonics are particularly well expressed, allowing us to study their 3D interactions at the scale of the whole orogenic system.
On the island, GPS data (Yu et al. Reference Yu, Chen and Kuo1997, Reference Yu, Kuo, Punongbayan and Ramos1999) show the impact of an oblique subduction setting on short-term active deformation partitioning. It outlines different domains with specific kinematics and significant rotations (Angelier et al. Reference Angelier, Chang, Hu, Chang, Siame, Lee, Deffontaines, Chu and Lu2009). In the long term (i.e. at the scale of several million years), different tectonic processes interact with surface processes. The evolution of the palaeostress (e.g. Angelier et al. Reference Angelier, Bergerat, Chu and Lee1990) suggests significant rotations of the stress field over time and subsequent deformation partitioning at map scale across the whole mountain belt. Experimental studies also illustrate the impact of the PSP indenter on wedge deformation showing different fault kinematics and block rotations (Lu & Malavieille, Reference Lu and Malavieille1994; Lu et al. Reference Lu, Chan, Lee, Chu and Malavieille2002).
At the scale of lithospheric plates (Fig. 2), deformation evolves from typical submarine accretion in the south, to continental subduction beneath the Luzon arc and wedge accretion, which collapses more to the north at the junction with the Ryukyu fore-arc. The transition from oceanic to continental subduction along the Manila Trench occurs near 22° N, but the impact on deformation of this major change in the nature and geometry of the two plates is observed as far south as 21° N.
The evolution of the Taiwan orogenic wedge is complex as the upper-plate backstop of the subduction evolves continuously from the south to the north. During oceanic subduction (south of Taiwan), accretion of trench fill sediments and pieces of the South China Sea oceanic basement occurs mainly against a relatively flat rigid backstop constituted by the fore-arc basement. The geodynamic setting becomes complex to the north owing to the subduction of the fore-arc lithosphere, which constituted the former backstop of the orogenic wedge during the stages of incipient continental subduction. The oceanic prism overrides the domain of accreted lower-plate units. Progressively, owing to the continuous shortening-induced crustal thickening and effects of erosion, the oceanic wedge materials are completely removed from the mountain belt. The deformation in the retrowedge changes as a result of the indentation of the hinterland by the arc lithosphere, inducing major backthrusting and backfolding. This phenomenon explains why no remnant nappes of the PSP fore-arc upper plate exist in the synformal part of the orogenic wedge, as, for instance, is observed at some places in the Banda orogen (e.g. Harris, Reference Harris, Brown and Ryan2011). The upper-plate geometry and behaviour can be different depending on the structure and evolution of the orogenic wedge. In the western Alps, for example, the Austro-Alpine nappe units represent remnants of African upper plate, suggesting that before the development of major backthrusting a relatively flat upper-plate lid covered the orogen (see Malavieille, Reference Malavieille2010, fig. C). Thinning of the upper-plate lid by erosion allowed such backthrusting and backfolding to develop, the Alpine belt evolving finally as a doubly vergent orogenic wedge.
The tectonic history of the Taiwan orogen can be summarized as follows. Five main morphostructural domains characterize the evolution of the mountain belt (Fig. 4):

Fig. 4. Geodynamic evolution and deformation partitioning at plate scale. Five sections across different domains of the orogen (see location on Fig. 2) reflect the complex 3D evolution of the Taiwan orogenic wedge during continental subduction. From south to north: (a) subduction of the South China Sea lithosphere and development of the Manila sedimentary accretionary wedge; (b) incipient subduction of the Eurasian continental margin and shortening of the fore-arc domain; (c) mature continental subduction and fore-arc subduction; (d) subduction reversal; (e) collapse of the orogenic wedge induced by back-arc extension and Philippine Sea Plate (PSP) slab rollback.
(1) South of 21° N, a domain of oceanic subduction is characterized by the subduction of the South China Sea oceanic lithosphere under the PSP (Fig. 4a). The submarine Manila accretionary wedge has developed by accretion of the sediments deposited in the Manila Trench against the Luzon arc basement (e.g. Lester et al. Reference Lester, McIntosh, Van Avendonk, Lavier, Liu and Wang2013). Deformation partitioning at this stage is mainly characterized by E-vergent backthrusting in the retroside of the wedge in response to backstop geometry (the low-dipping fore-arc basement surface). Whereas frontal accretion develops through imbricate oblique thrusting and folding in the forewedge, backthrusting controls the growth of the retrowedge and fore-arc basin (e.g. Silver & Reed, Reference Silver and Reed1988; Chi et al. Reference Chi, Reed, Moore, Nguyan, Liu and Lundberg2003). Submarine gravity-driven processes control surface mass transfer and the formation of tectono-sedimentary mélanges (Festa et al. Reference Festa, Pini, Dilek and Codegone2010, Reference Festa, Dilek and Pini2012, Reference Festa, Pini, Ogata and Dilek2019). A strong coupling between backthrusting of sediments and oceanic rocks offscraped from the subducting oceanic plate and submarine erosional processes allows exhumation and redeposition of ophiolite-bearing mélanges along the retroside of the wedge (e.g. Chang et al. Reference Chang, Angelier, Huang, Lallemand, Funiciello and Lallemand2009; Chi et al. Reference Chi, Chen, Liu and Brookfield2014; Malavieille et al. Reference Malavieille, Molli, Genti, Dominguez, Taboada, Beyssac, Vitale-Brovarone, Lu and Chen2016; Chen et al. Reference Chen, Huang, Yan, Dilek, Chen, Wang, Zhang, Lan and Yu2017).
(2) In the domain of incipient continental subduction, deformation is characterized by the transition between submarine processes acting to the south and aerial processes, which strongly impact the development of the orogenic wedge to the north. It has undergone a complex tectonic history (Fig. 4b). Owing to oblique subduction coupled with the nature and geometry of the continental margin, the wedge growth involves strong deformation partitioning mainly in the area located between the retroside of the growing wedge and the volcanic arc (e.g. Reed et al. Reference Reed, Lundberg, Liu and Kuo1992). Here, major deformation occurs in the sedimentary wedge owing to the shortening induced by the subduction (and/or strong deformation) of a fore-arc basement sliver beneath the PSP upper plate (e.g. Chemenda et al. Reference Chemenda, Yang, Hsieh and Groholsky1997). Offshore south of 22° N, as a result of the continental margin subduction, the fore-arc basin is shortened in between the retroside of the accretionary wedge and the volcanic edifice of the arc. Along an E–W transect, different morphotectonic units are present and characterize partitioning across the wedge. In the frontal part, a ‘lower-slope wedge’ with a very low dipping slope characterizes frontal accretion. This domain is bounded to the east by a slope break characterized by a steeply dipping upper slope. In the deep core of the wedge, basal accretion is likely to occur. Different mechanical interpretations have been proposed for these morphostructural features. Reed et al. (Reference Reed, Lundberg, Liu and Kuo1992) suggested significant shortening of the central domain of the wedge, resulting from out-of-sequence thrusting within the wedge, and/or underplating along the base of the wedge and major backthrusting in the fore-arc domain. Underplating of transitional crust units in the core of the thrust wedge (McIntosh et al. Reference McIntosh, Nakamura, Wang, Shih, Chen and Liu2005) represents an additional cause for changes in the slope dip, the domain of low-dip slope representing the zone of frontal accretion, the high-dip upper slope marking the area of underplating at depth (e.g. see also experimental studies of Gutscher et al. Reference Gutscher, Kukowski, Malavieille and Lallemand1998 or Kukowski et al. Reference Kukowski, Lallemand, Malavieille, Gutscher and Reston2002). In the frame of this kinematic setting, the Huatung ridge (HtR) that has developed east of the retrowedge (Fig. 3) has been interpreted as deformed sediments of the fore-arc basin mixed with various ophiolite-bearing mélanges (e.g. Malavieille et al. Reference Malavieille, Lallemand, Dominguez, Deschamps, Lu, Liu, Schnuürle, Byrne and Liu2002; Malavieille & Trullenque, Reference Malavieille and Trullenque2009; Malavieille et al. Reference Malavieille, Molli, Genti, Dominguez, Taboada, Beyssac, Vitale-Brovarone, Lu and Chen2016; Chen et al. Reference Chen, Huang, Yan, Dilek, Chen, Wang, Zhang, Lan and Yu2017). North of 22° N, the Hengchun Peninsula (HP) represents the emerged continuation of the submarine prism core (Fig. 4b). At this latitude, a large part of the slope sediments from the continental margin is involved in the accretionary wedge. Onland, the deformation structures of southern Taiwan characterize the main tectonic events that occurred during the growth of the orogenic wedge. Thick series of Miocene deposits in this area suffered low-grade metamorphism and polyphased ductile to brittle deformation during the late Cenozoic orogeny (Lu et al. Reference Lu, Lee, Angelier, Lee and Chu2001). Structural studies suggest that, during progressive deformation, important partitioning occurred in the form of a large counterclockwise rotation of this southern part of the emerged wedge (Chang et al. Reference Chang, Angelier, Lee and Huang2003, Reference Chang, Angelier, Huang, Lallemand, Funiciello and Lallemand2009). This Plio-Pleistocene partitioning of deformation is the result of the tectonic escape induced by the progression from south to north of the PSP indentor (e.g. Lu & Malavieille, Reference Lu and Malavieille1994). Such a phenomenon has similar consequences on wedge deformation structures as incoming asperities (ridges, seamounts, plateaus, area of rough basement with strong basal coupling) in a critical wedge (see for example the modelling of this process in Lallemand et al. Reference Lallemand, Malavieille and Calassou1992; Dominguez et al. Reference Dominguez, Lallemand, Malavieille and Schnurle1998 a,b, Reference Dominguez, Malavieille and Lallemand2000). As subduction is oblique transpressive, strain partitioning concentrates in this active area producing important rotations around the vertical axis (e.g. Chang et al. Reference Chang, Angelier, Huang, Lallemand, Funiciello and Lallemand2009). The special organization of ophiolite-bearing tectono-sedimentary mélanges in Taiwan (e.g. Malavieille et al. Reference Malavieille, Molli, Genti, Dominguez, Taboada, Beyssac, Vitale-Brovarone, Lu and Chen2016; Chen et al. Reference Chen, Huang, Yan, Dilek, Chen, Wang, Zhang, Lan and Yu2017) reflects the complex evolution of the orogen involving interactions between wedge growth, deformation partitioning and external processes (submarine gravity-driven erosion and aerial surface processes).
(3) The domain of mature continental subduction extends from 22° 40′ N to about 24° N. It represents the area where the extended continental margin of eastern Asia is largely involved in the subduction. The wedge grows again by frontal accretion to the west (e.g. Simoes et al. Reference Simoes, Avouac, Beyssac, Goffe, Farley and Chen2007), underplating in the central part of the belt (Beyssac et al. Reference Beyssac, Simoes, Avouac, Farley, Chen, Chan and Goffe2007) and accretion of parts of the arc–fore-arc domain, as well as backthrusting and tectonic wedging in the eastern side of the orogenic belt (Malavieille et al. Reference Malavieille, Lallemand, Dominguez, Deschamps, Lu, Liu, Schnuürle, Byrne and Liu2002). Figure 4c illustrates the deformation of the main tectonic units constituting the mature Taiwan belt. In this area, the slice of fore-arc basement is entirely subducted under the PSP lithosphere. East of the mountain belt, the volcanic arc edifice is completely detached from the PSP and accreted to the orogenic wedge. The fore-arc domain is drastically reduced giving place to the Longitudinal Valley structure, which marks the fossil plate boundary suture. The wide orogenic wedge constituted by all the various accreted units and growing foreland basin presently deforms against the basement of the residual volcanic arc.
(4) Orogenic collapse and subduction reversal are expected to take place beneath northern Taiwan (Fig. 4d) (Malavieille et al. Reference Malavieille, Lallemand, Dominguez, Deschamps, Lu, Liu, Schnuürle, Byrne and Liu2002). Possible driving mechanisms have been highlighted by physical and numerical modelling studies (e.g. Chemenda et al. Reference Chemenda, Yang, Konstantinovskaya and Ivanov2001; Faccenda et al. Reference Faccenda, Gerya and Chakraborty2008; Stern & Gerya, Reference Stern and Gerya2017). The development of a more symmetrical two-sided collision zone is caused by the indentation of the EP by the PSP lithosphere. As the Ryukyu subduction becomes dominant the PSP starts to tear the EP inverting subduction polarity and halting continental subduction and wedge growth (Fig. 5).
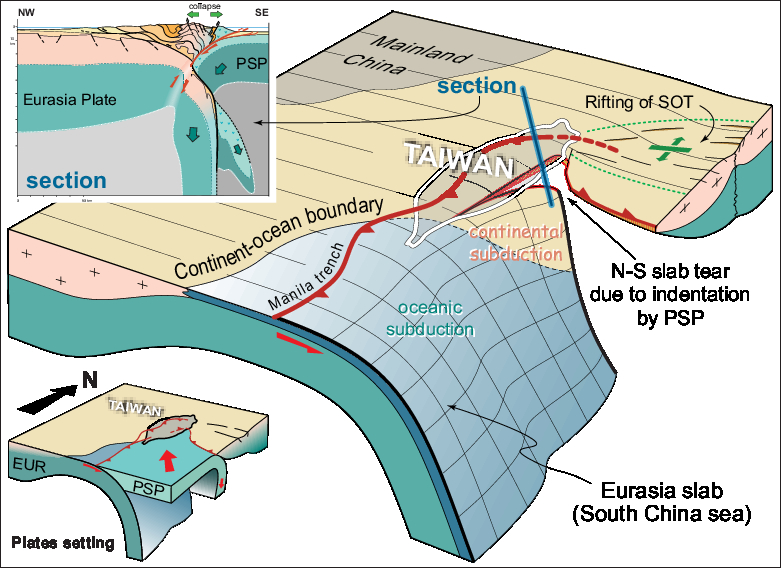
Fig. 5. Three-dimensional view of plate geometry showing the tear of the Eurasia Plate (EUR) indented by the edge of the Philippine Sea Plate (PSP). Inset shows indentation on a section across the Taiwan mountain belt. SOT – Southern Okinawa Trough.
(5) A strong curvature characterizes the northeastern part of the orogen. Following indentation of the EP and migration of the Ryukyu subduction to the southwest, the former northern tip of the orogenic wedge has undergone a clockwise rotation around a vertical axis during orogenic collapse facilitated by slab rollback and consequent westward spreading of the Okinawa back-arc basin (Fig. 4e).
4. Deformation partitioning in the Taiwan orogen: insights from analogue models
4.a. Response of topography to strain partitioning and its effects on the crustal structure, exhumation and long-term evolution of the orogenic wedge
The major switch from oceanic to continental subduction has played a major role in the deformation partitioning observed in Taiwan. Geodynamic and analogue models must, therefore, account for the inherited structural architecture of the tectonically extended Eurasian margin in better understanding the evolution of the orogenic wedge. Simple analogue or numerical models of accretionary wedges show that when décollement horizons are present within accreted sedimentary sequences, they strongly modify the behaviour of thrusting. This modification involves two competing mechanisms of deformation: frontal accretion and basal accretion (e.g. Gutscher et al. Reference Gutscher, Kukowski, Malavieille and Lallemand1998; Kukowski et al. Reference Kukowski, Lallemand, Malavieille, Gutscher and Reston2002; Konstantinovskaia & Malavieille, Reference Konstantinovskaia and Malavieille2005; Hoth et al. Reference Hoth, Adam, Kukowski and Oncken2006; Bonnet et al. Reference Bonnet, Malavieille and Mosar2007; Stockmal et al. Reference Stockmal, Beaumont, Nguyen, Lee, Sears, Harms and Evenchick2007; Feng et al. Reference Feng, Bartholomew and Choi2015). Both accretion mechanisms work synchronously in the growing wedge and change its internal structure, shape and time-progressive evolution. Insights from these models applied to Taiwan help develop an understanding of its complex structural evolution. In the 1990s, thermomechanical modelling of mountain building applied to the Taiwan case (Barr & Dahlen, Reference Barr and Dahlen1989; Dahlen & Barr, Reference Dahlen and Barr1989; Barr et al. Reference Barr, Dahlen and McPhail1991) proposed that underplating of footwall rocks by the assimilation of duplexes along the basal décollement would play a significant role in the development of fold-and-thrust belts. Owing to the observed balance between the accretionary influx at the toe and the erosive efflux off the top, these researchers did not consider this process as significant. Subsequently, it has been shown by other researchers (Hwang & Wang, Reference Hwang and Wang1993; Crespi et al. Reference Crespi, Chan and Swaim1996; Konstantinovskaia & Malavieille, Reference Konstantinovskaia and Malavieille2005; Fuller et al. Reference Fuller, Willett, Fisher and Lu2006; Simoes & Avouac, Reference Simoes and Avouac2006; Simoes et al. Reference Simoes, Avouac, Beyssac, Goffe, Farley and Chen2007; Beyssac et al. Reference Beyssac, Simoes, Avouac, Farley, Chen, Chan and Goffe2007; Chen et al. Reference Chen, Chan, Lo, Malavieille, Lu, Tang and Lee2018), using structural analysis, thermomechanical modelling, thermochronology and analogue modelling, that underplating processes have been largely underestimated. These studies have also shown that orogenic processes cannot be understood without considering the major role played by surface processes.
Insights from previously published experimental studies of thrust wedges (e.g. Malavieille, Reference Malavieille1984; Calassou et al. Reference Calassou, Larroque and Malavieille1993; Lallemand et al. Reference Lallemand, Schnurle and Malavieille1994; Lu & Malavieille, Reference Lu and Malavieille1994; Kukowski et al. Reference Kukowski, Von Huene, Malavieille and Lallemand1994; Chemenda et al. Reference Chemenda, Mattauer, Malavieille and Bokun1995; Larroque et al. Reference Larroque, Calassou, Malavieille and Chanier1995; Gutscher et al. Reference Gutscher, Kukowski, Malavieille and Lallemand1996, Reference Gutscher, Kukowski, Malavieille and Lallemand1998, Reference Gutscher, Klaeschen, Flueh and Malavieille2001; Dominguez et al. Reference Dominguez, Lallemand, Malavieille and Von Huene1998 b, Reference Dominguez, Malavieille and Lallemand2000; Lallemand et al. Reference Lallemand, Liu, Dominguez, Schnurle and Malavieille1999; Martinez et al. Reference Martinez, Malavieille, Lallemand and Collot2002; Kukowski et al. Reference Kukowski, Lallemand, Malavieille, Gutscher and Reston2002; Konstantinovskaia & Malavieille, Reference Konstantinovskaia and Malavieille2005; Bonnet et al. Reference Bonnet, Malavieille and Mosar2007, Reference Bonnet, Malavieille and Mosar2008; Malavieille & Trullenque, Reference Malavieille and Trullenque2009; Malavieille & Konstantinovskaya, Reference Malavieille and Konstantinovskaya2010; Konstantinovskaya & Malavieille, Reference Konstantinovskaya and Malavieille2011; Graveleau et al. Reference Graveleau, Hurtrez, Dominguez and Malavieille2011, Reference Graveleau, Malavieille and Dominguez2012, Reference Graveleau, Strak, Dominguez, Malavieille, Chatton, Manighetti and Petit2015; Perrin et al. Reference Perrin, Clemenzi, Malavieille, Molli, Taboada and Dominguez2013; Guerit et al. Reference Guerit, Dominguez, Malavieille and Castelltort2016; Malavieille et al. Reference Malavieille, Molli, Genti, Dominguez, Taboada, Beyssac, Vitale-Brovarone, Lu and Chen2016) combined with recent ones are analysed to investigate the effects of erosion on the active bivergent orogenic wedge of Taiwan. In this present study, we have used in most of the experiments granular materials (sand, silica, plastic powders) to simulate crustal deformation and associated coupling with surface processes. Analogue materials are well adapted to model deformation mechanisms in the brittle upper crust, including fracturing, faulting and even folding. No viscous materials, except for the one presented in Figure 10, were included in our experiments to keep them as simple as possible. However, granular materials can display a viscous-like behaviour when subjected to particular deformation conditions, such as large cumulated shear stress/strain and increased confinement pressure. For instance, to model underplating processes, granular materials undergo large strains because significant shortening is required to activate underthrusting and basal accretion mechanisms. Under such conditions, previously formed thrust units experience increasing confinement and most sand grains are involved in deformation. At a macroscopic scale, sand deforms in a more homogeneous way, and continuous deformation produces a sort of ductile behaviour.

Fig. 10. Still image of an experimental set-up showing normal faults developing in the hinterland of a subduction wedge during convergence (A. Hamon, unpub. M.Sc. thesis, Montpellier Univ., 2018). A low-viscosity material at the base of the sequence allows décollement, folding, thrusting and normal faulting. Thickening of the viscous material at the base of the model in the hinterland allows local extension to develop above the domain of basal accretion. More information on an equivalent experimental set-up, material and boundary conditions are presented in Graveleau et al. (Reference Graveleau, Strak, Dominguez, Malavieille, Chatton, Manighetti and Petit2015).
In former experiments (Konstantinovskaia & Malavieille, Reference Konstantinovskaia and Malavieille2005; Konstantinovskaya & Malavieille, Reference Konstantinovskaya and Malavieille2011), analogue models have been submitted to erosion under flux steady-state conditions to simulate an erosion pattern close to what is expected in Taiwan over long periods of time. Experimental results reveal that the presence of weak layers, simulating décollement levels, in the entering sequence promotes strain partitioning. The frontal part of the model wedges is characterized by imbricate accretion of the upper sequences located above the décollement, whereas basal accretion of underthrusted units characterizes the deformation in the hinterland. Duplex underplating at depth gives rise to the development of an antiformal nappe stack, whose growth and location is enhanced by erosion. With continued shortening and erosion a stable zone of fast exhumation is created, controlled by underplating at depth and by the major backthrusting and backfolding occurring in the retroside of the wedge. The whole material resting above the abandoned décollements is then passively uplifted, incurring only gentle internal deformation and limited horizontal shortening (Fig. 6). Thus, the main mechanisms of wedge growth can be described by frontal accretion in the foreland and basal accretion of tectonic units at depth in the hinterland (Malavieille, Reference Malavieille2010; Harris, Reference Harris, Brown and Ryan2011).
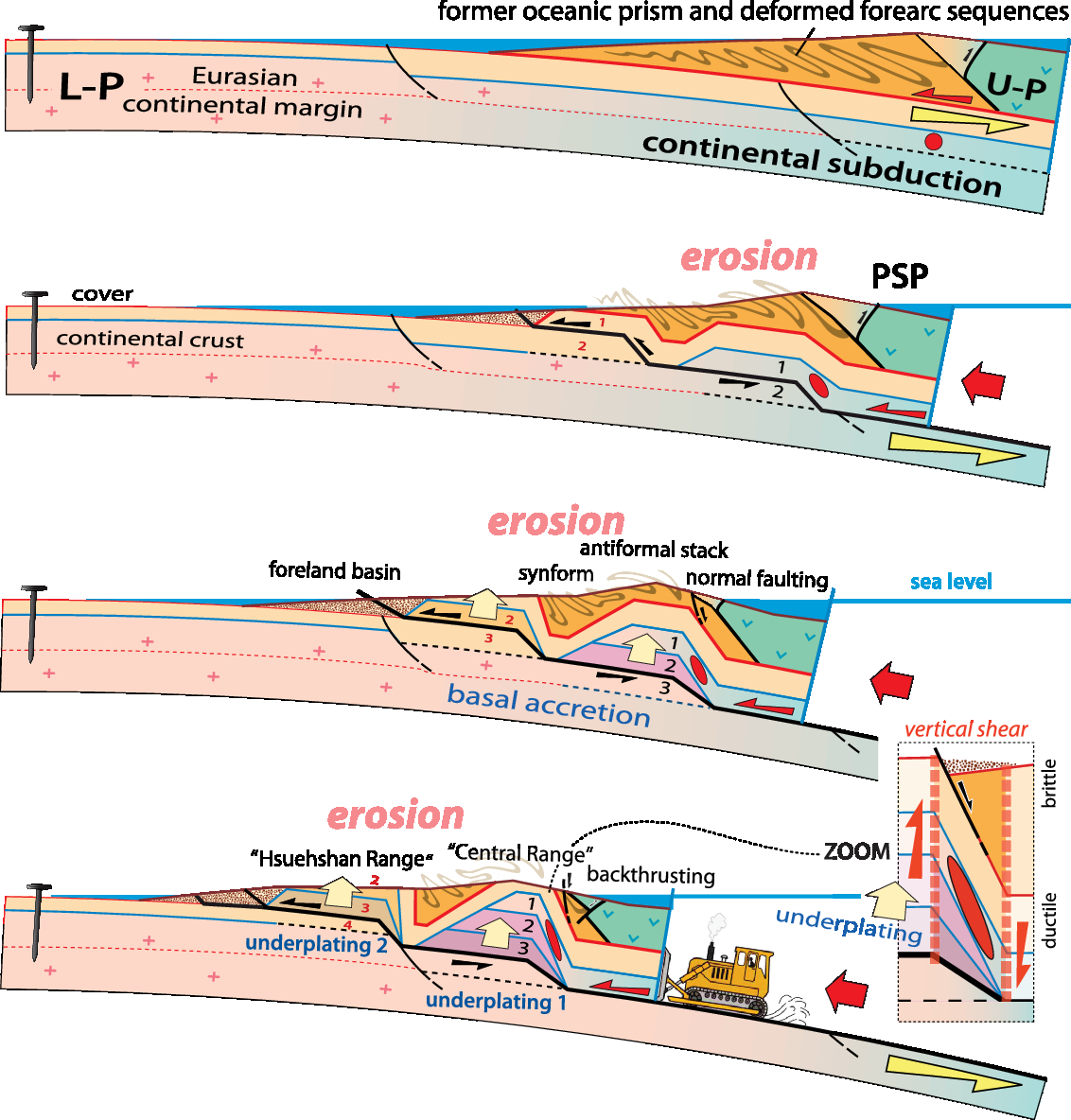
Fig. 6. Sequential tectonic diagrams depicting mechanisms involved in deformation partitioning during subduction of a continental margin. Four stages of convergence are shown for the growth of the entire orogenic wedge. This conceptual model is applied to Taiwan. Close-up diagram on the last panel shows the details of a vertical shear that develops at the back of an antiformal stack allowing syn-convergent normal faulting.
Within the Taiwanese wedge, intra-crustal décollements are influenced by flats and ramps, and inherited structures localized within the subducting continental margin of Eurasia. The continental margin of Eurasia favours the same style of deformation partitioning and wedge growth (e.g. Malavieille et al. Reference Malavieille, Lallemand, Dominguez, Deschamps, Lu, Liu, Schnuürle, Byrne and Liu2002; Malavieille & Trullenque, Reference Malavieille and Trullenque2009; Simoes et al. Reference Simoes, Beyssac and Chen2012). Underplating occurred in two domains of the Taiwan orogenic wedge: in the hinterland under the Central Range (e.g. Simoes & Avouac, Reference Simoes and Avouac2006) and under the Hsuehshan Range (e.g. Chen et al. Reference Chen, Chan, Lo, Malavieille, Lu, Tang and Lee2018). Basal accretion is at the origin of rapid uplift and exhumation of wedge material, and accounts for most of the vertical component of deformation. Early thrust faults and décollements are then passively uplifted and gently bent upwards during continuous underplating processes (Figs 1b, 6).
In such a framework, the strain and deformation patterns are expected to significantly evolve across the whole orogenic wedge. Regional variations from west to east in the orientation of maximum finite stretch across the wedge hinterland (with down-dip maximum stretching) and along-strike maximum elongation in its eastern part occur in the western Central Range, suggesting a complex three-dimensional deformation system. Deformation structures and patterns have been studied in Taiwan for a long time, resulting in various and still controversial hypotheses (e.g. Stanley et al. Reference Stanley, Hill, Chang and Hu1981; Ernst & Jahn, Reference Ernst and Jahn1987; Teng, Reference Teng1990; Faure et al. Reference Faure, Lu and Chu1991; Clark et al. Reference Clark, Fisher and Lu1992, Reference Clark, Fisher, Lu and Chen1993; Fisher & Byrne, Reference Fuller, Willett, Fisher and Lu1992; Tillman & Byrne, Reference Tillman and Byrne1995; Fisher, Reference Faccenda, Gerya and Chakraborty1999; Pulver et al. Reference Pulver, Crespi, Byrne, Byrne and Liu2002; Fisher et al. Reference Fisher, Lu, Chu, Byrne and Liu2002, Reference Fisher, Willett, En-Chao and Clark2007; Yamato et al. Reference Yamato, Mouthereau and Burov2009; Byrne et al. Reference Byrne, Chan, Rau, Lu, Lee, Wang, Brown and Ryan2011; Mondro et al. Reference Mondro, Fisher and Yeh2017). The map (Fig. 7) of the long-term structural data compiled by C.-Y. Lu (unpub. data) shows a ductile deformation pattern (orientation of foliation, stretching lineations and fold axis) in the metamorphic hinterland of the Taiwan orogen. It is widely accepted (e.g. Malavieille et al. Reference Malavieille, Lacassin and Mattauer1984; Ellis & Watkinson, Reference Ellis and Watkinson1987; Dias & Ribeiro, Reference Dias and Ribeiro1994) that stretching lineations develop subparallel to convergence and approach transport direction of geological structures (ductile shear zones, thrusts, nappes, etc.). In the Central Range, the main trend of stretching is surprising as it appears that the x axis of the strain ellipsoid is oblique to the convergence direction and parallel to the trend of the mountain chain. Pulver et al. (Reference Pulver, Crespi, Byrne, Byrne and Liu2002) considered that deformation has been partitioned between the Slate Belt and the Tananao Complex. They propose that in response to oblique collision, the pre-Tertiary metamorphic basement was deformed within a zone of left-lateral transpression and that the orogen-perpendicular component of oblique convergence was accommodated by lateral extrusion. Mondro et al. (Reference Mondro, Fisher and Yeh2017) suggested that owing to the obliquity of the convergence vector relative to the regional strike of the mountain range and owing to the orientation of the Luzon arc relative to the edge of the Eurasian continental margin, deformation could result in partitioning of strike-slip shearing into the interior of the collision and lateral extrusion of the ductile core of the range. They proposed that deformation partitioning reflects lateral advection through the strain field in response to accretionary and erosional fluxes. Only this last work considers the major complementary roles of subduction obliquity, subsequent strain partitioning and erosion.
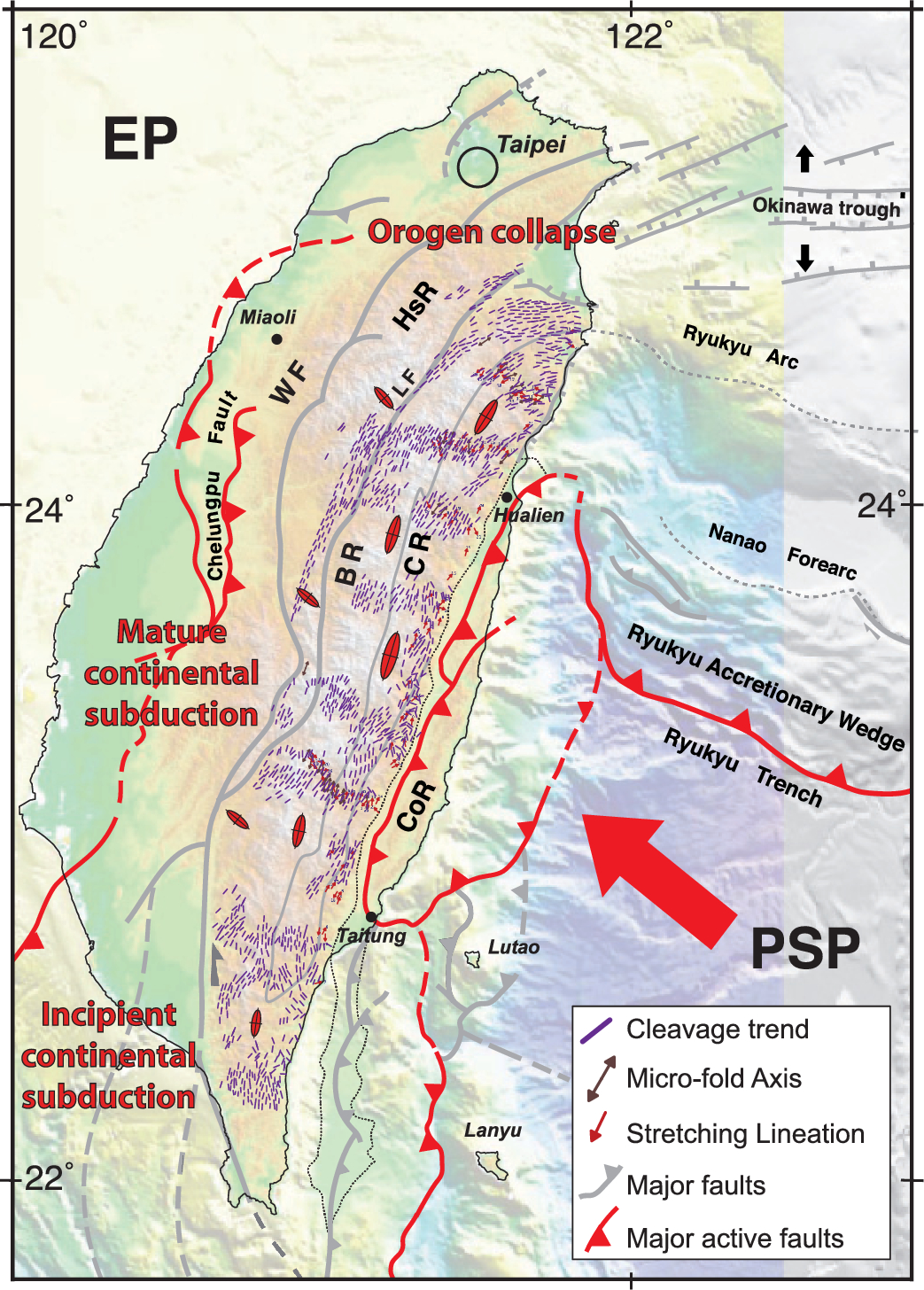
Fig. 7. Ductile strain patterns in the metamorphic part of the Taiwan mountain belt. Orientations of structures are shown on a simplified structural map (data from C.-Y. Lu, unpub. compilation). Approximate shape and orientation of the strain ellipsoid are marked by red ellipses. WF – Western Foothills; HsR – Hsuehshan Range; BR – Backbone Range; CR – Central Range; CoR – Coastal Range; PSP – Philippine Sea Plate; ER – Eurasia Plate.
We propose a complementary model for deformation partitioning in Taiwan based on geological observations and results of the experimental models coupling deformation and surface processes as summarized above. Strain partitioning occurs in the Taiwan orogenic wedge owing to oblique convergence, basal accretion, subsequent fast uplift and erosion in the hinterland. Thin-skinned décollement tectonics and imbrication of thrust units characterize the growth of the foreland thrust-and-fold belt. Deformation in the hinterland of the orogenic wedge is more complex. The first stage of deformation initiates during subduction within the obliquely subducting continental margin. It is characterized in the early accreted tectonic units of the wedge by a primary foliation associated with zones of high shear strain, regionally consistent stretching lineations that develop oblique to the trend of the belt, and isoclinal and sheath folds. It corresponds to the early deformation acquired during basal accretion of tectonic slices through subhorizontal tectonic contacts. Then, continuous underplating allows the development of an anticlinal stack of crustal units. Low-angle shear zone décollements activated at depth are progressively uplifted, deactivated and passively refolded. Combined uplift and erosion allow exhumation of the deep metamorphic units that constitute an antiformal structure (e.g. Platt, Reference Platt1986).
The continuous accretion of these tectonic units into the core of the orogenic wedge allows the progressive deformation to overprint former fabrics. The mainly horizontal compressional deformation applied perpendicular to the belt induces refolding of early flat structures and develops a penetrative strain characterized by new foliations and lineations overprinting former ones. Significant stretching and associated lineations develop parallel to the main trend of the orogen. Second phase structures reflect emergence and exhumation of these tectonic units progressively accreted to the upper plate and evolve independently of oblique plate convergence kinematics. Erosion of the rapidly uplifted rocks constituting the antiformal stack favours a complex 3D behaviour which involves lateral and vertical extrusion of ductile crustal material. This progressive deformation in the hinterland characterizes the strain partitioning which constrains the tectono-metamorphic evolution of the hinterland.
Accretion at depth through underplating of tectonic units has been shown from models to be cyclical (e.g. Gutscher et al. Reference Gutscher, Kukowski, Malavieille and Lallemand1998; L. Clemenzi, unpub. M.Sc. thesis, Univ. di Pisa, 2010). Thus, several cycles of basal accretion can occur during wedge growth. When the antiformal stack reaches a certain size, duplexes of lower units are no longer accreted to this structure, allowing a new duplex to develop commonly in a more forelandward position (L. Clemenzi, unpub. M.Sc. thesis, Univ. di Pisa, 2010; Perrin et al. Reference Perrin, Clemenzi, Malavieille, Molli, Taboada and Dominguez2013). Since the overall shape of the accretionary wedge is generally characterized by a basal dip and a slope angle, the presence of two adjacent duplexes leads to some interesting features. The area of maximum exhumation is located in correspondence with the more external antiformal stack, where the wedge is thinner and a small duplex may crop out. The maximum metamorphic peak conditions, on the contrary, are reached in correspondence with the more internal duplex, where the wedge is thicker and units can be deeply buried and then exhumed from a greater depth. Complex geometric relationships may develop between units underplated in the first duplex, and units underplated in the second one (Fig. 8a–c). In Taiwan, thermometry and thermochronology data obtained along transects through the orogen confirm such a complex behaviour and timing of underplating processes (e.g. Beyssac et al. Reference Beyssac, Simoes, Avouac, Farley, Chen, Chan and Goffe2007 for the hinterland and Chen et al. Reference Chen, Chan, Lo, Malavieille, Lu, Tang and Lee2018 for the Hsuehshan Range).

Fig. 8. (a) Coupling between tectonics and surface processes in Taiwan. High erosion rates are located above the two domains of underplating (Hsuehshan Range and Central Range). The curve at the top shows qualitatively variations in metamorphic grade across the orogenic wedge. Abbreviations from left to right: CP – Coastal Plain; WF – Western Foothills; HR – Hsuehshan Range; SB – Slate Belt; CR – Central Range; LV – Longitudinal Valley; CR – Coastal Range; PSP – Philippine Sea Plate. (b) Photo of an analogue model wedge involving a décollement layer and erosion. Two domains of basal accretion, characterized by underplated duplexes, are demonstrated. (c) Line drawing showing the main elements of the orogenic wedge, main faults and the folded décollement bounding underplated duplexes.
The Figure 9 block diagram illustrates the partitioning of deformation in an idealized doubly vergent orogenic wedge submitted to oblique convergence and to the action of surface processes locally enhanced above the domain of basal accretion. It shows that deformation partitioning and flow of ductile material at depth (e.g. Platt, Reference Platt1993, Reference Platt2000) involving stretching, refolding, and lateral and vertical extrusion is characterized by complex 3D kinematics. Accretion and crustal thickening occur in two different ways. The frontal part of the wedge is mainly characterized by imbricated thrust units and thrust kinematics in a direction sub-perpendicular to the axis of the belt. In the hinterland, major strain partitioning develops. Oblique compressional deformation induces ductile flow and stretching of deep rocks in a direction parallel to the belt trend. Lateral and vertical extrusion combined with erosion of uprising units favoured by underplating at depth allow a strong exhumation to develop in this domain of the orogenic wedge.
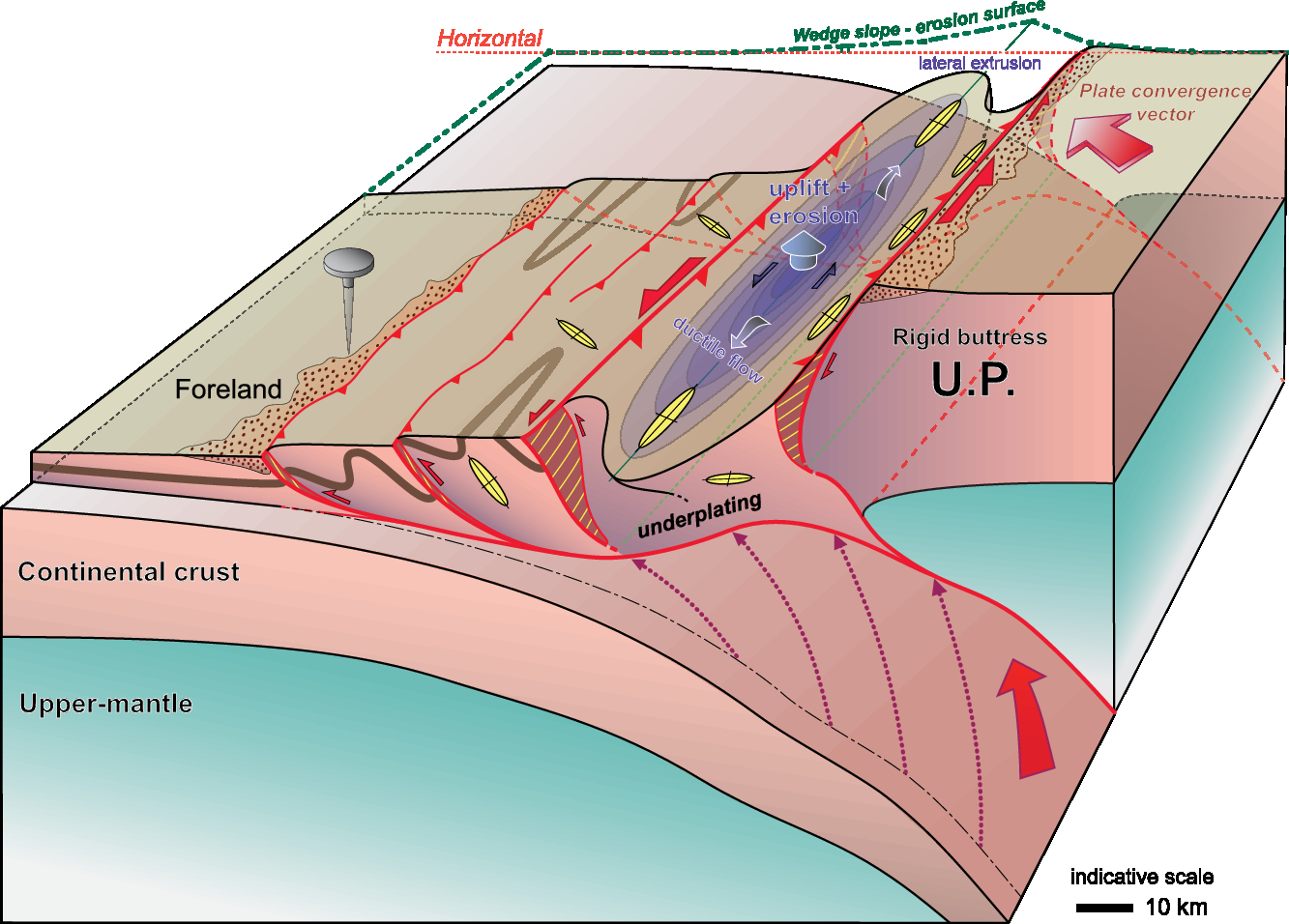
Fig. 9. Three-dimensional geodynamic diagram of a subduction–accretion system, showing the impact of deformation partitioning in erosional orogenic wedges developed in an oblique convergence setting. Ductile flow, lateral extrusion and exhumation in the hinterland are favoured by strain partitioning and erosion. Fault kinematics is suggested by the yellow lines drawn on the fault planes. U.P. – upper plate.
4.b. Normal faulting
Normal faults and extensional shear zones have been observed in numerous hinterlands of active or ancient orogenic wedges (Dalmayrac & Molnar, Reference Dalmayrac and Molnar1981; Norton, Reference Norton1986; Malavieille, Reference Malavieille1987; Selverstone, Reference Selverstone1988; Dewey, Reference Dewey1988; Jolivet et al. Reference Jolivet, Dubois, Fournier, Michard and Jourdan1990; Malavieille et al. Reference Malavieille, Guilhot, Costa, Lardeaux and Gardien1990; Burchfiel et al. Reference Burchfiel, Chen, Hodges, Liu, Royden, Deng and Xu1992; Malavieille, Reference Malavieille1993; Burg et al. Reference Burg, Van Den Driessche and Brun1994; Chemenda et al. Reference Chemenda, Mattauer, Malavieille and Bokun1995). Similar normal faults are described in many places along the eastern part of the hinterland of the Taiwan wedge. As suggested by Crespi et al. (Reference Crespi, Chan and Swaim1996), ‘normal faulting appears to be an integral part of the orogenic process and is the response at upper crustal levels to uplift driven by underplating at depth’. Results of analogue models validate the mechanical aspect of this statement (Fig. 10). In addition, they show that surface processes represent a major factor for the development of normal faulting during convergence in compressional orogens. In the case of Taiwan, normal faults are localized in the eastern part of the Central Range, along the boundary between the accreted wedge derived from the lower plate and the upper plate (PSP basement and Coastal Range volcanic arc) of the subduction zone. The domain affected by normal faulting is broad and represents the shallow expression of the differential motion between the rapidly uplifted and eroded materials of the Central Range and the oceanic rocks of the less deformed upper-plate lithosphere (Fig. 6). Thus, underplating processes at depth induce the development of a subvertical zone of shear deformation and faulting. This brings into contact the rapidly exhumed metamorphic rocks of the eastern Central Range and less deformed non-metamorphic rocks of the Coastal Range (accreted part of subduction upper plate) along the Longitudinal Valley.
This normal shear deformation and faulting affect the eastern side of the uplifted and exhumed antiformal stack. Owing to the action of erosion and progressive deformation, resulting structures evolve from deep-seated ductile shear zones to brittle normal faults formed in the shallow levels of the upper crust (see zoom in Fig. 6).
Looking at experiments in more detail, we notice that this differential motion induces a secondary deformation. A large component of backthrusting and backfolding increasingly correlates with the evolution of the subvertical zone of crustal normal shear in the retrowedge. It locally reworks or overprints syn-convergent normal faults. This comparison reveals that the Longitudinal Valley is a complex structure (e.g. Shyu et al. Reference Shyu, Sieh, Chen, Chuang, Wang and Chung2008), whose deformation history is marked by alternating periods of normal faulting and backthrusting along the eastern side of the Central Range, combined with northwestward thrusting along the western flank of the Coastal Range.
4.c. Impact of deformation partitioning and erosion on the location of major seismogenic faults
In Taiwan, owing to the high convergence rate (8 ~ 9 cm yr−1) between Eurasia and the PSP, deformation rates are extreme (horizontal shortening >2 cm yr−1 on seismogenic faults with vertical motions up to 3 cm yr−1). Similarly, owing to extreme climatic conditions such as frequent typhoons and earthquake-induced landslides, catastrophic erosion has sculpted the sharp relief of the island within a span of a few million years. Present-day active deformation is marked by an array of seismogenic faults (see map of fig. 3 in Shyu et al. Reference Shyu, Sieh, Chen and Liu2005), mainly located at the boundaries of the orogenic wedge. Most of the big earthquakes (M >7) occur in the frontal part of the orogen and along the arc–continent plate boundary (yellow stars, Fig. 11). If we consider the short- to middle-term deformation kinematics, we realize that most of the horizontal shortening is accommodated by just a few major faults on the western foreland side of the wedge and along its retroside against the Philippine Sea upper plate. Around 4 cm yr−1 of shortening occurs across the frontal faults (e.g. Dominguez et al. Reference Dominguez, Avouac and Michel2003; Simoes et al. Reference Simoes, Avouac, Beyssac, Goffe, Farley and Chen2007), whereas about 3 cm yr−1 are consumed on the backside along the onshore Longitudinal Valley faults (e.g. Shyu et al. Reference Shyu, Sieh, Chen and Chung2006) and a few centimetres per year offshore within the PSP along the submerged flank of the Coastal Range (e.g. Malavieille et al. Reference Malavieille, Lallemand, Dominguez, Deschamps, Lu, Liu, Schnuürle, Byrne and Liu2002; Simoes & Avouac, Reference Simoes and Avouac2006). In contrast, little horizontal shortening occurs within the Central Range in the hinterland of the wedge owing to a strong partitioning of deformation modes between crustal depths and the surface.
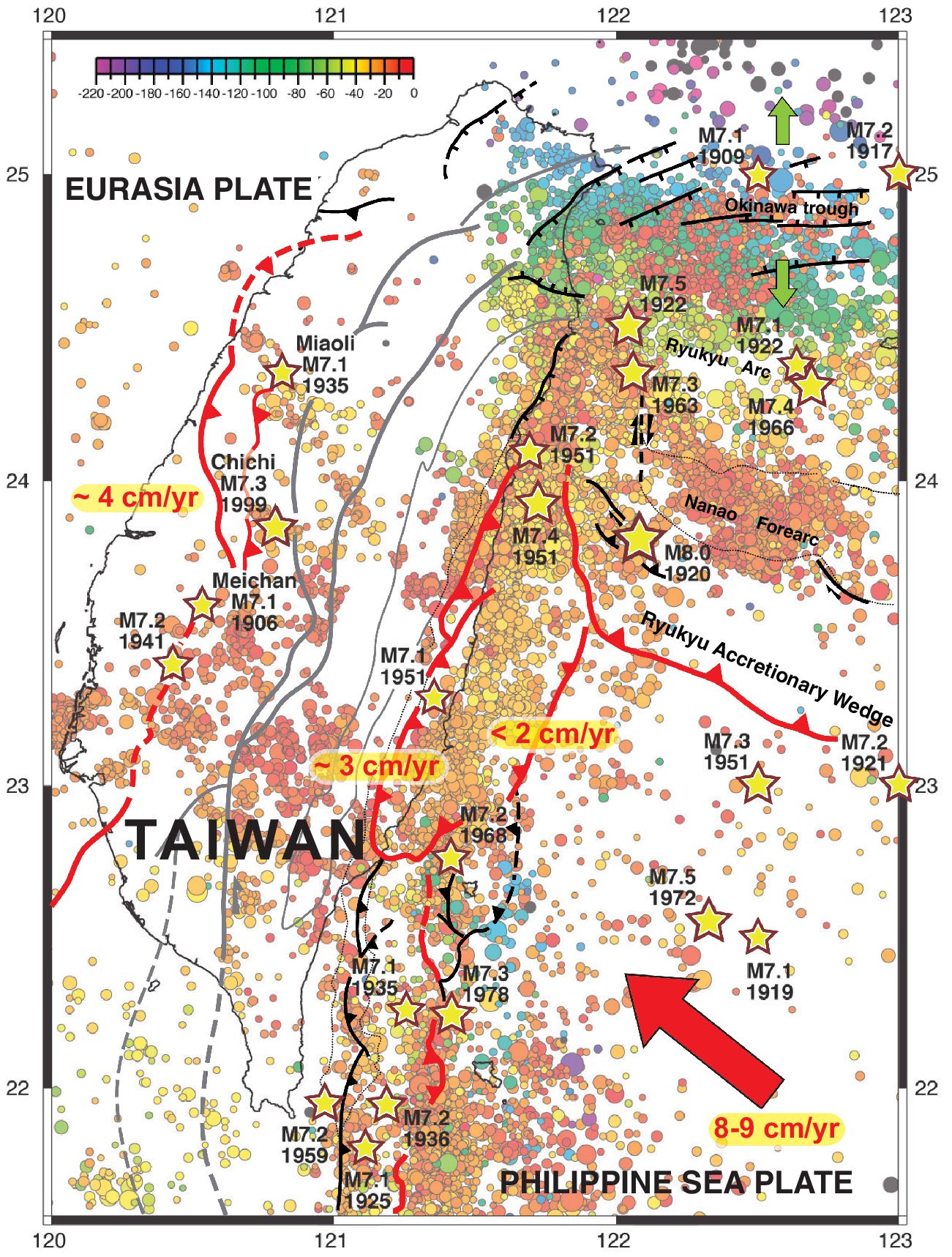
Fig. 11. Tectonic map outlining the main active faults (in red) and seismicity in the Taiwan orogenic belt and its oceanic surroundings from global (Engdahl et al. Reference Engdahl, Van Der Hilst and Buland1998) and regional networks (Yu et al. Reference Yu, Chen and Kuo1997; Cheng et al. Reference Cheng, Wang, Shyu and Shin1998). Locations of large (M >7) historical earthquakes are marked by yellow stars. Long-term estimates of horizontal shortening rates are given (red values in the yellow frame).
Such a kinematic pattern closely matches the behaviour of experimental erosional wedges involving décollements (see Fig. 1b). Here again the structural inheritance and the presence of décollements in the continental margin involved in subduction favour underplating mechanisms at depth and passive vertical unroofing of tectonic units in the hinterland. Two simple experiments (Fig. 12) characterize this unexpected behaviour. The evolution of an asymmetric doubly vergent thrust wedge is simulated using a simple sandbox that mimics subduction kinematics with a fixed upper plate and a mobile lower plate. The set-up (Fig. 12a) is constituted by a horizontal basement bounded by two sidewall glasses. A 0.1 m wide Mylar sheet, lying on the horizontal plate, is pulled beneath a flat upper plate, allowing more than 1.5 m of convergence for each experiment. In our experiment 10 mm is roughly equivalent to 1 km in nature. The material converges towards the S-point. A double-slope proto-wedge is built including coloured horizontal passive markers to enable a direct observation and analysis of deformation and fault kinematics (Fig. 12b, initial stage). The materials and boundary conditions of the two models are identical. Both involve a thin décollement level located in the middle part of the sand layers (weak layer of glass microbeads). The only difference is that one is run with no erosion (Fig. 12c), and in the other erosion is applied and all the incoming material is mechanically removed during shortening, maintaining a regular flux steady-state topography (Fig. 12d, e). That is, the volume of eroded material remains equal to the volume of newly accreted material, maintaining constant surface slopes during shortening.
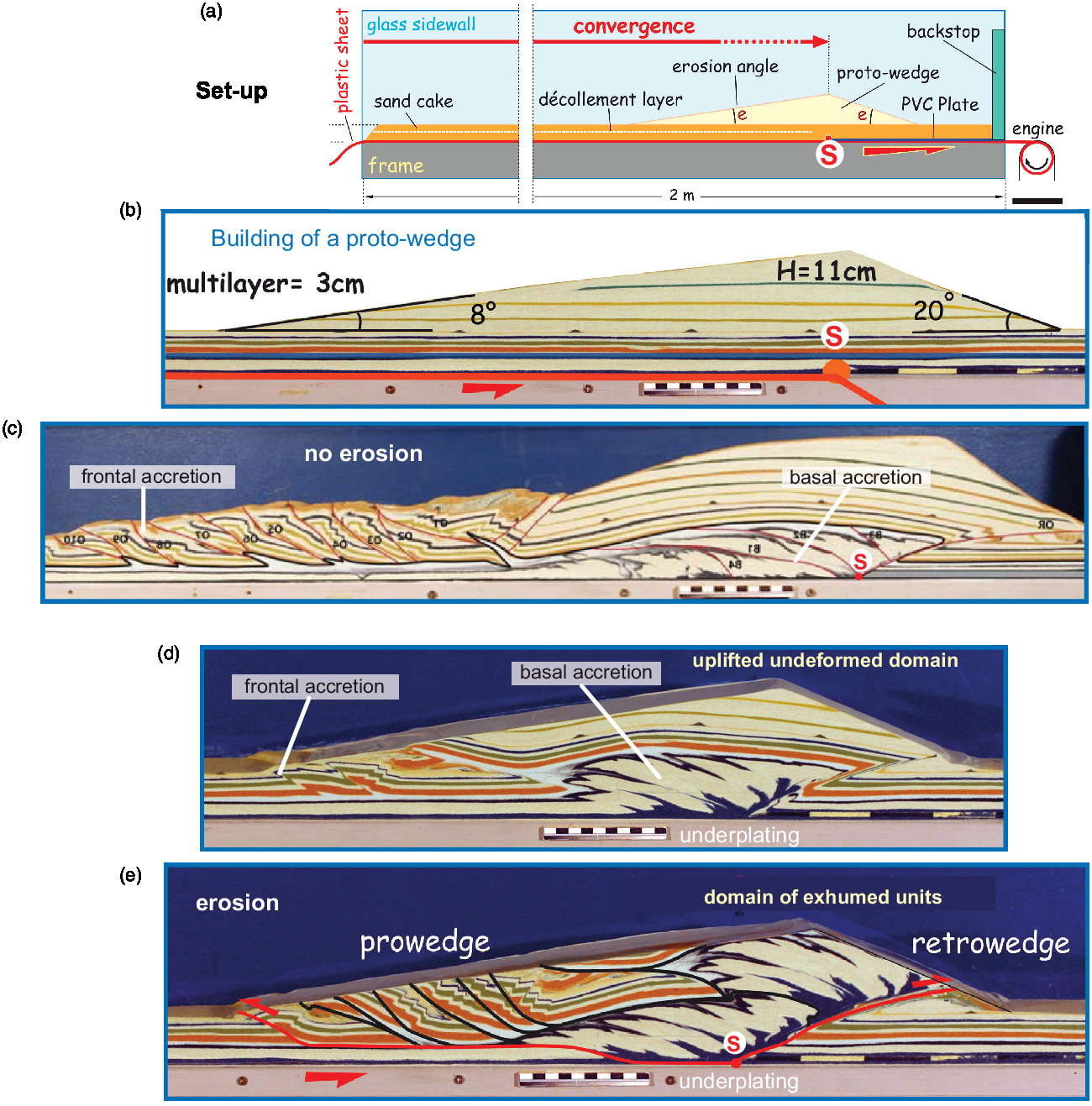
Fig. 12. Experiments showing the impact of deformation partitioning and erosion on the kinematics of main faults (M. Limoncelli, unpub. M.Sc. thesis, Univ. di Pisa, 2008). (a) Experimental set-up. (b) Initial stage of experiment showing the geometry of the two-sided proto-wedge. (c) Final result of an experiment with no erosion. (d) Experiment with erosion, intermediate stage showing the passive uplift of the undeformed proto-wedge above the domain of basal accretion. (d) Final geometry of the same experiment.
The results of these experiments clearly show the kinematics of deformation partitioning in the wedge. In the first model without erosion, partitioning involves a well-developed frontal accretion in the forewedge and a purely basal accretion in the hinterland part of the wedge (Fig. 12c). In the inner wedge, contractional deformation barely affects the upper layers, as shown by the passively uplifted horizontal markers of the proto-wedge. The second model (Fig. 12d, e) is exactly the same; it only differs because erosion removes a large part of the proto-wedge material, resulting in exhumation of underplated tectonic duplexes. Formerly accreted wedge material and underplated units are passively uplifted and continuously eroded owing to active basal accretion (see video in the online Supplementary Material).
Extrapolating our experimental results to the morphostructural evolution of Taiwan leads to the following interpretations. Continental material from the subducting Eurasian margin is accreted at depth by underplating processes. It develops a regional-scale antiformal nappe stack (the Central Range), where metamorphic rocks are rapidly exhumed from depth owing to combined effects of basal accretion and erosion (Fig. 13a). Tectonic units formerly formed in the frontal part of the orogen are then passively uplifted. Sliding on active faults at depth occurs along ductile shear zones by aseismic distributed slip. Thus, a direct relationship exists between tectonics (shortening inducing the partitioning between horizontal and vertical displacements on faults) and surface processes contributing to huge material transfer. Such a coupling has important consequences; the main seismogenic faults are located at the boundaries of the wedge both in front (Western Foothills) and along its backpart (in the Longitudinal Valley and offshore) where horizontal shortening rates are high. Most of the deformation that is responsible for the fast uplift of the hinterland is taken into account by aseismic ductile strain at depth (Fig. 13b). This unexpected behaviour clearly shows that the wedge does not grow as a simple homogeneous Coulomb wedge in which a punctuated activity of imbricate thrusts characterizes deformation mechanisms. Both, the high heat flow and the low seismicity observed today beneath the Central Range are consistent with this behaviour at the scale of the orogen (Chi & Reed, Reference Chi and Reed2008; Mesalles et al. Reference Mesalles, Mouthereau, Bernet, Chang, Lin, Fillon and Sengelen2014; Avouac, Reference Avouac and Schubert2015; Dal Zilio et al. Reference Dal Zilio, Van Dinther, Gerya and Pranger2018).

Fig. 13. Impact of deformation partitioning on the location of seismogenic faults prone to generating large earthquakes. (a) Long-term horizontal shortening rates estimated on major active faults reported on a general section across the Taiwan orogen. (b) Relationships between deformation partitioning in the Taiwan orogenic wedge and seismic behaviour of main active faults that generate large earthquakes. U.P. – upper plate; L.P. – lower plate.
5. Conclusions
Results of experiments involving erosion, sedimentation and structural inheritance compared to the Taiwan active mountain belt allow a better understanding of the coupling between tectonics and surface processes as well as the resulting mechanisms of deformation partitioning. Many of the first-order parameters that control deformation partitioning at all scales in orogenic wedges during continental subduction are highlighted in this study and its findings. These first-order parameters are summarized as the following: Deformation partitioning occurs during all stages of mountain building from the subduction of the continental margin to mountain collapse. Coupling between surface processes and tectonic processes plays a major role. At a large scale, the nature and geometry of the converging plates, obliquity of convergence and the accretionary mechanisms determine the shape and evolution of the growing orogen. Underplating processes coupled with surface erosion control the evolution of the internal structure of orogenic wedges and significantly impact the exhumation of deep rocks. They also impact the location of faults prone to generating large earthquakes across the wedge. Syn-convergent normal faulting in the retroside of orogenic wedges could be a consequence of deformation partitioning in the wedge (horizontal versus vertical differential motions of material).
In Taiwan, the nature of the underplating Eurasian continental margin has played a significant role in deformation partitioning. It provides insights into how margin structure contributes to changes in a fold-and-thrust belt along-strike and how deformation partitioning plays a major role at all scales during continental subduction. The inversion of a hyper-extended rifted margin is responsible for the structures and tectono-thermal evolution of the Central Range (McIntosh et al. Reference McIntosh, Van Avendonk, Lavier, Lester, Eakin, Wu, Liu and Lee2013; Mesalles et al. Reference Mesalles, Mouthereau, Bernet, Chang, Lin, Fillon and Sengelen2014). Structural inheritance of basement faults (reactivation of normal faults) is a key component in producing changes in structure, seismicity and topography along-strike, depending on which morphological part of the margin is involved in the deformation (Suppe, Reference Suppe1986; Mouthereau et al. Reference Mouthereau, Deffontaines, Lacombe, Angelier, Byrne and Liu2002; Byrne et al. Reference Byrne, Chan, Rau, Lu, Lee, Wang, Brown and Ryan2011; Brown et al. Reference Brown, Alvarez-Marron, Biete, Hao, Camanni and Ho2017).
Author ORCIDs
Jacques Malavieille 0000-0003-2423-9932, Chih-Tung Chen 0000-0002-4498-5513
Acknowledgements
This work has benefitted from a 30-year-long collaboration between Taiwan and France, and since 2005, from research projects developed in the framework of the LIA D3E, CNRS-MOST France–Taiwan International Laboratory. Discussions and fieldwork with numerous Taiwanese colleagues allowed us to propose this study. The manuscript benefitted from constructive reviews and comments by Mark Brandon, Ron Harris and Yildirim Dilek, the Editor. We thank Christian Romano for his strong support for the design of the various experimental setups and for help during modelling.
Supplementary material
To view supplementary material for this article, please visit https://doi.org/10.1017/S0016756819000645.