INTRODUCTION
Broechem-Nierlenders is part of a group of early medieval, pre-Christian burial sites in the Flemish (i.e. north-Belgian) part of the Meuse-Demer-Scheldt region (Figure 1; Annaert Reference Annaert, Kars, Van Oosten, Roxburgh and Verhoeven2018a) (note that in the study area the Early Middle Ages cover the period from the beginning of the 5th to the end of the 9th century AD: Slechten Reference Slechten2004). The site, containing both inhumation and cremation graves, was excavated by the Flanders Heritage Agency and its predecessors, during two campaigns (2001–2003 and 2007–2010). A full account of the excavation results and a catalog of the finds have been published by Annaert (Reference Annaert2018b). The cemetery at Broechem proves to be the oldest early medieval burial ground within the region and was in use from the first half of the 5th century until the mid-7th century AD, a date range based upon the study of the cultural artifacts. With its 513 graves, including three horse burials clearly associated with a human grave, it is also the largest early medieval cemetery known in the region, presenting a typical example of a central burial ground used by a community living in several farmsteads spread over the surrounding landscape (Annaert Reference Annaert, Kars, Van Oosten, Roxburgh and Verhoeven2018a). On the basis of the common grave goods (pottery, weapons) and exceptional finds such as (gold) coins, gold brooches with garnet inlay and the horse burials, it is evident that the people buried at Broechem knew a clear social stratification, with a regional higher class within this peasant society certainly being present (Annaert and Ervynck Reference Annaert, Ervynck and Ludowici2013).
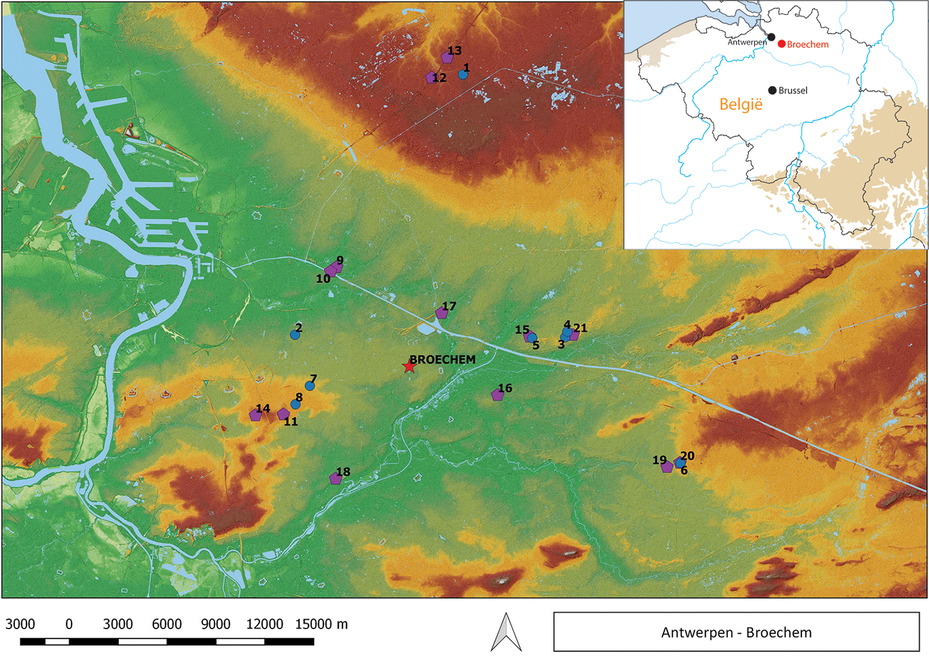
Figure 1 Early medieval cemetries and settlements in the region east of Antwerp (Belgium): 1. Brecht; 2. Borsbeek; 3. Grobbendonk; 4. Grobbendonk-Ouwen; 5. Pulle; 6. Herentals-Zandkapel; 7. Boechout-Mussenhoeve; 8. Boechout-St. Bavo; 9. Wijnegem-Steenakker; 10. Wijnegem-wachthaven Albertkanaal; 11. Hove-Cueteghem; 12. Brecht-Zoegweg; 13. Brecht-Hanenpad; 14. Edegem-Buizegem; 15. Pulle-Keulsebaan; 16. Nijlen-Mussenpad; 17. Oelegem-Zandhovensteenweg; 18. Lier-Hooglachenen; 19. Herentals-Morkhoven; 20. Herentals-Zandkapel; 21. Grobbendonk-Vorselaarsebaan (inset: location of Broechem in Belgium) (after Annaert Reference Annaert2018b: figs 1.1 and 3.12).
Broechem is located on dry, sandy-loamy soils. Hydrogeographically, the site belongs to the basin of the river Scheldt, with the Kleine Nete being the closest river. Smaller brooks can be found near the site (Figure 1). The habitations associated with the cemetery, or with cemeteries from the same period and region, are poorly documented archaeologically (Eggermont et al. Reference Eggermont, Annaert, Bastiaens, Derese, Vandenberghe, Van Den Haute, Haneca and Van Strydonck2008; Annaert Reference Annaert, Stevens, Landuyt and Gielis2010, Reference Annaert2018b; Debruyne et al. Reference Debruyne, Annaert, Clerbaut, Haneca and Lentacker2015), implying that many aspects of the lives of the people buried at Broechem-Nierlenders are hardly known. Poor site visibility and bad preservation conditions explain why environmental archaeology has produced virtually no data on the local, early medieval subsistence economy and (probably socially stratified) consumption patterns. The poor preservation conditions also determined the composition of the find assemblages from the inhumation graves. Except for a few human tooth rows (with only enamel still present), a number of mineralized textile (Walton Rogers Reference Walton Rogers and Annaert2018) and small wood fragments preserved in the corrosion layer of metal objects (Haneca et al. Reference Haneca, Deforce, Boone, Van Loo, Dierick, Van Acker and Van den Bulcke2012; Haneca and Deforce Reference Haneca, Deforce and Annaert2018), organic material was not preserved. In the cremation graves, however, an additional category of burnt or charred remains was recovered comprising seeds and fruits, charcoal, and cremated human and animal bone. Among the bones, only fragments with a white, chalky appearance were present (or preserved), indicating cremation temperatures higher than 500 to 650 °C (Zazzo et al. Reference Zazzo, Saliège, Person and Boucher2009: p. 605, table 3). The food offerings (Mittermeier Reference Mittermeier1986) in the cremation graves, when identifiable, always represent domestic pig (Sus scrofa f. domestica). In fact, given the absence of fragments of (unidentified) smaller or larger animals, it can be assumed that all animal bone from the cremations derives from pig (Ervynck and Lentacker Reference Ervynck, Lentacker and Annaert2018). Charcoal is not abundant in the cremation graves. Birch (Betula sp.), alder (Alnus sp.) and hornbeam (Carpinus betulus) are the dominant taxa, while oak (Quercus sp.) is only present in some of the graves, never dominating the assemblages (Deforce Reference Deforce and Annaert2018). In all features sampled, the density of charred seeds and fruits is very low, with only scarce indications for consumption remains. The taphonomic nature (depositional history) of this find category remains uncertain, and the probability of the occurrence of residual or intrusive material is evaluated as high (Cooremans Reference Cooremans and Annaert2018).
The excavated area at Broechem contained 438 inhumation and 75 cremation graves. On the basis of the characteristics of the grave gifts, both burial rituals were employed contemporaneously, although the cremation graves remain a minority within the funeral complex. The general chronological range of the cremation graves can be defined as first half of 5th to mid-7th century, on the basis of the cultural artifacts they contain, a chronology that corresponds to the date range of the inhumation graves (Annaert Reference Annaert2018b). As the cultural dates from the Broechem cemetery remain rather broad, it was attempted to obtain absolute dates through an archaeometric approach. A radiocarbon (14C) dating program has been set up in order to check and refine the general chronology put forward, and to reveal a possible chronological stratigraphy within the cemetery.
The first results of the dating program (Haneca et al. Reference Haneca, Ervynck, Boudin and Annaert2018), that initially exclusively focused on the cremated human remains, showed a dating range from 400 to 650 AD. The oldest dates derive from a cluster of graves in the northern part of the cemetery. The cremation from an urn, the only example of this burial ritual from the site, also belongs to this group of oldest graves. The remaining dates do not show a chronological evolution in spatial organization, as dates from all time periods are scattered throughout the cemetery (Haneca et al. Reference Haneca, Ervynck, Boudin and Annaert2018). These observations, however, did not bring the interpretation to an end. Comparing the radiocarbon dates from the human bones with the date ranges associated with the cultural artifacts from the graves revealed a discrepancy between both, with the human bones often being “too old” (Annaert Reference Annaert2018b). Therefore, it was decided to subject a series of cremated animal bones to radiocarbon analysis, which resulted in dates that were most often younger than those from the human bone from the same feature and that better matched the cultural chronology. This phenomenon was mentioned in the initial report (Haneca et al. Reference Haneca, Ervynck, Boudin and Annaert2018) where it was suggested that an old wood effect, acting more strongly on the human than on the animal bones, could perhaps explain the differences observed. CO2 derived from (old) wood used for the funeral pyre is taken up by the bones during cremation (Hüls et al. Reference Hüls, Nadeau, Grootes, Erlenkeuser and Andersen2010; Van Strydonck et al. Reference Van Strydonck, Boudin and De Mulder2010; Olsen et al. Reference Olsen, Heinemeier, Hornstrup, Bennike and Thrane2013; Snoeck et al. Reference Snoeck, Brock and Schulting2014; see also further: Discussion). In this study, the phenomenon is further explored, including, as a new step in the analysis, charcoal samples from the cremation graves. Charcoal samples from short-lived taxa were selected in order to better date the cremation event, while charcoal from long-lived taxa was chosen to evaluate the range of the old wood effect. Given the taphonomic uncertainties observed, and their low availability, charred seeds and fruits were not included in the dating program.
MATERIAL
In total, 71 radiocarbon dates from the cemetery at Broechem are now available, from 32 cremation graves (Table S1 in supplementary material). The selection of samples was strongly guided by availability, differing widely among the graves. Ten features are dated through the analysis of a single human bone fragment, while from another grave (feature 386) (only) two human bones were dated, because of the anomalous nature of the first result. From one grave, only a single piece of charcoal (Quercus sp.) is dated. The human bones dated derive from adult individuals, the age group that dominates the group of cremation remains from Broechem. Unfortunately, the material did not allow more precise age of death estimations (Quintelier and Watzeels Reference Quintelier, Watzeels and Annaert2018).
Twenty other graves have been dated on more than one type of material and will form the dataset discussed in this study. From these 20 graves, 58 dates were obtained: 21 on human bone (in one case two bones from the same grave, as part of an older, intercomparison study [Naysmith et al. Reference Naysmith, Scott, Cook, Heinemeier, van der Plicht, Van Strydonck, Bronk Ramsey, Grootes and Freeman2007]), 10 on animal (pig) bone, 17 on charcoal from short-lived taxa, 10 on charcoal from long-lived taxa (Table 1). The samples from short-lived taxa comprise charcoal fragments from alder (Alnus sp.), birch (Betula sp.), hazel (Corylus avellana) and willow (Salix sp.), which are the trees and shrubs with the lowest potential maximal age present in the graves. These taxa all have an average maximum age of ca. 150 years or less (McVean Reference McVean1953; Bugmann Reference Bugmann1994; Hynynen et al. Reference Hynynen, Niemistö, Viherä-Aarnio, Brunner, Hein and Velling2009; Vakkari Reference Vakkari2009). Usually, fragments from twigs would have been preferred as samples but the material from Broechem did not allow such selection. The charcoal from long-lived taxa in all cases derives from oak (Quercus sp.), which has an average maximum age of 500 years and an exceptional maximum age of ca. 1000 years (Rackham Reference Rackham1980; Bugmann Reference Bugmann1994). It was attempted to select pieces of oak heartwood, based on the presence of high numbers of tyloses in the earlywood vessels of the charcoal fragments (Marguerie and Hunot Reference Marguerie and Hunot2007), as sapwood is generally characterised by the absence or a far lower frequency of tyloses in earlywood vessels (Dufraisse et al. Reference Dufraisse, Coubray, Girardclos, Dupin and Lemoine2018). It should be mentioned that some minute fragments of coal were also found among the macrobotanical remains but these are considered to be intrusive (Deforce Reference Deforce and Annaert2018) and thus without influence on the present study.
Table 1 Material from 20 cremation graves from Broechem dated by radiocarbon analysis (HB: human bone, AB: animal bone, CH_S: charcoal from short-lived taxa, CH_L: charcoal from long-lived taxa, p: present, pp: two samples dated, *: intrusive material).

METHODS
All charcoal samples were pretreated with the acid-alkali-acid (AAA) method. The human bone fragments selected derive from the cortex of long bones. In all cases except one (grave 373), adult bone was present. In the case of the animal bones, again pieces of compact bone were selected, most often shaft fragments of long bones, representing subadult or adult animals, certainly no young piglets. Only white, calcined bone fragments were used for the analysis, an evident choice because this was the only human or animal material present or preserved in the cremation graves. Bone that had not been fully cremated, showing dark brown, grey or black colors (Ellingham et al. Reference Ellingham, Thompson, Islam and Taylor2015), would have presented a risk of contamination (Van Strydonck et al. Reference Van Strydonck, Boudin and De Mulder2009). The preparation of the cremated bone samples was done at the Royal Institute for Cultural Heritage (KIK/IRPA, Brussels) taking extreme care to avoid contamination (following Van Strydonck et al. Reference Van Strydonck, Boudin and De Mulder2009). The bone fragments were first treated with hydrogen chloride (HCl, 8%), dissolving ca. 30% of the cremated bone, i.e. the outer part that could have been touched by contamination. The remaining pieces were then washed with demineralized water, dried and grinded. Then followed an immersion in acetic acid (CH3-COOH, 1%) for 24 hr, after which the residue was rinsed with demineralized water, and again dried. In a next step, phosphoric acid (H3PO4, 85%) was added under vacuum. The CO2 that was released was captured in a reactor with liquid nitrogen and transformed into graphite. The 14C/12C ratio in the graphite was measured using accelerator mass spectrometry (AMS) and converted into a radiocarbon age (expressed in years BP), after correction for isotope fractionation, using the δ13C AMS measurement. All measurements took place using the MICADAS of the Royal Institute for Cultural Heritage (RICH-codes in Table S1, supplementary material), except three that were measured at the Leibniz Laboratory of the Christian Albrechts University at Kiel, Germany (KIA-codes in Table S1), as part of an intercomparison study (Naysmith et al. Reference Naysmith, Scott, Cook, Heinemeier, van der Plicht, Van Strydonck, Bronk Ramsey, Grootes and Freeman2007). In these three cases, it is not sure but indeed most probable that fragments of long bones were used as samples.
Calibration of the conventional radiocarbon ages into calendar years (BC/AD) was performed using the software package OxCal (Bronk Ramsey Reference Bronk Ramsey2009: OxCal version 4.2.4, https://c14.arch.ox.ac.uk/oxcal.html) and the atmospheric calibration curve IntCal13 (Reimer et al. Reference Reimer, Bard, Bayliss, Beck, Blackwell, Ramsey, Buck, Cheng, Edwards Rl, Grootes, Guilderson, Haflidason, Hajdas, Hatté, Heaton, Hoffmann Dl, Hughen, Kaiser, Kromer, Manning, Niu, Reimer, Richards, Scott, Southon, Staff, Turney and van der Plicht2013). It should be noted that a major part of the calibrated dates in this study show a broad distribution, due to the irregular form of the calibration curve between ca. 400 and 550 AD. The possible contemporaneous nature of radiocarbon dates from the same feature was investigated using a χ2-test, as part of the OxCal function R_Combine (Ward and Wilson Reference Ward and Wilson1978).
RESULTS
General
All radiocarbon measurements are listed in Table S1, supplementary material, and presented graphically in Figure S1, supplementary material. In the presentation and discussion of the results, following abbreviations will be used: HB (human bone), AB (animal bone), CH_S (charcoal from short-lived taxa) and CH_L (charcoal from long-lived taxa). When a date is described as being “older” or “younger” than another date, this means that there is a significant statistical difference between the radiocarbon dates, on the basis of a χ²-test taking into account a 95% probability. When dates are described as being “contemporaneous”, this implies that the radiocarbon dates do not show a significant statistical difference, again using the χ²-test and 95% probability. Differences between dates are expressed as differences between the conventional radiocarbon ages (radiocarbon years BP) before calibration (Table 2).
Table 2 Conventional radiocarbon dates (R: radiocarbon years BP) from 20 cremation graves from Broechem, and differences (D: radiocarbon years) between them, per feature (HB: human bone, AB: animal bone, CH_S: charcoal from short-lived taxa, CH_L: charcoal from long-lived taxa, *: OxCal combination of two dates, (): statistically not significant difference). Three dates obtained from intrusive material have been left out: one CH_S (grave 272) and two CH_L (graves 83 and 510).

Analysis per Grave
The results can first be viewed per feature separately. The order of the graves follows Table 1.
grave 150: HB and CH_L are older than AB and CH_S, the latter two being contemporaneous. CH_L is contemporaneous with HB.
grave 377: HB and CH_S are older than AB and CH_L, the latter two being contemporaneous. CH_S is contemporaneous with HB.
grave 85: HB is older than AB and CH_S, the latter two being contemporaneous.
grave 173: HB and CH_S are older than AB, the former two being contemporaneous.
grave 369: HB and CH_S are older than AB, the former two being contemporaneous.
grave 373: HB is older than AB and CH_S, the latter two being contemporaneous.
grave 374: HB is older than AB and CH_S, the latter two being contemporaneous.
grave 88: HB is older than AB.
grave 229: Both HB samples are contemporaneous. The combined dates for HB and AB are contemporaneous.
grave 384: HB is older than AB.
grave 83: CH_S is older than HB. CH_L is intrusive.
grave 163: CH_L is older than HB and CH_S, the latter two being contemporaneous.
grave 272: HB is contemporaneous with CH_L. CH_S is intrusive.
grave 372: HB, CH_S and CH_L are all contemporaneous.
grave 379: HB, CH_S and CH_L are all contemporaneous.
grave 466: CH_L is older than HB and CH_S, the latter two being contemporaneous.
grave 492: HB, CH_S and CH_L are all contemporaneous.
grave 510: HB and CH_S are contemporaneous. CH_L is intrusive.
grave 75: HB and CH_S are contemporaneous.
grave 216: HB and CH_S are contemporaneous.
Two outliers are the results for HB in grave 374, a very old date, outside of the variation of the other HB dates, and for AB from grave 384, a young date outside of the variation of all other AB (and HB) dates. Three dates obtained from charcoal (one CH_S [grave 272] and two CH_L [graves 83 and 510]) are much younger than the rest of the dataset and these samples must be regarded as intrusive material.
Human versus Animal Bone
In 10 cases, both HB and AB are present (Table 2). In all but one, HB is older than AB, while in one case (grave 229) HB yielded a somewhat older date than AB without the difference being statistically significant. The difference between the BP radiocarbon date for HB and AB varies from 79 to 115 radiocarbon years, for 7 of the cases. In graves 374 and 384 this difference is much larger (266 and 281 radiocarbon years, respectively). One case (grave 229) showed a difference of only 41 radiocarbon years. The mean of the differences measured is 99 radiocarbon years for the 7 first cases, with a standard deviation of 13.
Charcoal from Short-Lived Taxa
From 17 graves a sample of CH_S could be recovered (Table 1). One of them yielded a date that clearly indicates intrusive material (grave 272) (omitted from Table 2). The remaining 16 dates can be compared with those from other find categories (Table 2). In 7 graves, AB was also present and in 4 of those cases CH_S proves to be contemporaneous with AB. In 3 cases, however, CH_S is significantly older than AB, with differences of 93 (grave 377), 111 (grave 369) and 185 radiocarbon years (grave 173).
When comparing the dates from CH_S and HB (16 cases), 11 combinations show no statistically significant difference. However, in 3 of those 11 cases an AB was also found that is around a century younger than the CH_S and HB (the 8 other graves contained no AB). In one case, CH_S proves to be older than HB (grave 83: a difference of 85 radiocarbon years) while in the 4 remaining cases HB is older than CH_S (differences of 81 (grave 85), 96 (grave 150), 117 (grave 373) and 339 radiocarbon years (grave 374) but in this last case the HB date is an outlier).
Charcoal from Long-Lived Taxa
Ten samples of charcoal of long-lived taxa were available but two of them yielded dates that imply that the material was intrusive (graves 83 and 510). When combining the dates from CH_S and CH_L, possible for 7 graves, in three cases CH_L is older than CH_S (differences of 77 [grave 163], 150 [grave 150] and 228 radiocarbon years [grave 466]), in three cases there is no statistically significant difference (graves 379, 492 and 372) and in one case (grave 377), CH_S is older than CH_L (difference of 88 radiocarbon years).
When comparing CH_L and HB dates, possible in 8 cases, CH_L is older than HB in two cases (102 [grave 163] and 228 radiocarbon years [grave 466]), CH_L is younger than HB in one case (74 radiocarbon years [grave 377]) and in 5 cases there is no significant difference between the dates for CH_L and HB. A comparison between the dates of CH_L and AB was only possible in two cases, one in which CH_L is older than AB (difference of 169 radiocarbon years [grave 150]), and one in which CH_L is contemporaneous with AB (grave 377).
DISCUSSION
General Remarks
The results of the dating program show that in a number of graves the CH_S produced dates that are not the youngest for that feature (or that are not contemporaneous with the youngest), in contrast to what was expected. In some cases, the time differences with the dates from other material can attain values up to a century and more. At least a part of the CH_S fragments must thus be residual, or represent recycled wood used as fuel for the pyre or derive from the heartwood of trees that had reached a considerable age. The latter explanation can most probably not hold for the CH_S samples showing the largest differences in dating since the taxa sampled usually do not attain ages of a century or more. The argument of recycling is also somewhat problematic because the wood of the selected short-lived taxa (i.e. Alnus sp., Betula sp., Corylus avellana and Salix sp.) is not frequently used as construction timber as these wood species are not durable (durability class 5: European Standard 350-2 1994), have no good mechanical properties and do not provide timber in large dimensions (Wiselius Reference Wiselius1990; Fennessy Reference Fennessy2004; Haneca et al. Reference Haneca, Cufar and Beeckman2009; Deforce Reference Deforce2017). An additional explanation could lie in the observation that a number of CH_S samples showed the traces of insect damage. This implies that wood was used from trees that were already dead for some time but that were still standing. Such dead wood, when protected by the forest canopy is dry and ideal for starting a fire (Deforce Reference Deforce and Annaert2018). However, as the wood from the selected short-lived taxa is not durable, it is characterised by a high decomposition rate and dead wood from these taxa will thus have had a short residential time in the woods.
For three cremation graves the radiocarbon date of the CH_L is contemporaneous with that of the CH_S, HB or AB, and for three other graves the CH_L dates are significantly older. This is in line with what could be expected. The stem of an old-grown oak tree can be considered as a composite of successive annual growth layers, with the oldest wood near the pith (center) of the tree and the most recently formed layers of wood towards the outer circumference. Hence, when using an entire trunk of oak wood as fuel for a cremation a proportionally larger volume of younger wood (from the outermost part of the trunk) will be charred compared to the relatively small volume of old wood present near the center of the stem (see next section for more detailed calculations). A random sample of charcoal thus has a higher probability to have been part of the outermost (younger) volume of an old-grown oak tree. Charcoal samples from the more outward growth layers of an old tree, will therefore result in comparable radiocarbon ages of coeval short-lived trees. In this case three out of six CH_L samples have indeed comparable radiocarbon ages compared to CH_S samples from the same grave. In three other graves, the selected CH_L fragments are clearly older than the CH_S samples from the same grave, and therefore probably originate from the center of an old-grown oak tree. In one grave (377) however, the CH_L sample provided a date that was younger than the short-lived charcoal sample, and therefore contrasts the model described above. Possibly, this sample derives from the outer side of a stem or a branch and thus consists of young (sap)wood. Considering the overlap with the HB and AB dates, the CH_L samples that are coeval with the CH_S samples, are also coeval with the HB dates. In those cases where CH_L is significantly older that the CH_S, the oak charcoal is much older than HB, although the HB dates can certainly also be anomalous (see further). Additionally, for all CH_L samples an intrusive nature can never be excluded totally.
Given the uncertainties with the dates and the taphonomy of the charcoal samples, it is best to concentrate the discussion upon the data for HB and AB (finds that have less chance to be intrusive). They provide the most consistent pattern for the study collection, with HB being almost always older than AB. This is true for 7 out of 8 graves, excluding the two cases with outliers. The difference varies around ca. a century (of radiocarbon years). In one grave the date for HB is older than that for AB without the difference being statistically significant. Overall, the AB dates prove to be always the youngest or among the youngest per assemblage (including the charcoal samples). Where comparisons can be made between the AB dates and the date ranges of the cultural artifacts, there is never a conflict between the two, but it must be admitted that the ranges are broad (Table S1 in supplementary material).
Old Wood
The main challenge now is to explain the (rather consistent) difference in radiocarbon dates between the HB and the AB. First, the old wood hypothesis mentioned in the preliminary study (Haneca et al. Reference Haneca, Ervynck, Boudin and Annaert2018) must be considered, implying that part of the carbon in the cremated bone finally derived from CO2 escaping the funeral pyre. Experimental work with AB has indeed shown that it takes up a lot of CO2 from the pyre, replacing half to almost all of the carbon in the bio-apatite matrix (Hüls et al. Reference Hüls, Nadeau, Grootes, Erlenkeuser and Andersen2010; Zazzo et al. Reference Zazzo, Saliège, Lebon, Lepetz and Moreau2012; Snoeck et al. Reference Snoeck, Brock and Schulting2014). In these experiments the fire was maintained for a few hours, but real cremations must have lasted longer, suggesting that carbon replacement was even higher. Indeed, old wood is present within the assemblages (see section above) but it must be questioned whether its dates are sufficiently old to cause the bias of around a century in the dates of the HB. The argument could perhaps be valid for the two cases in which the CH_L is much older than the HB, but in five cases the dates of both samples are contemporaneous (the case in which CH_L is younger than HB is left out of this discussion). Furthermore, a (living) old tree also contains a lot of young wood.
To evaluate this, from dendrochronologically measured tree-ring data of early medieval construction timbers (from well linings: Haneca Reference Haneca2010, Reference Haneca2011, Reference Haneca2012), a general growth curve for oak trees (Quercus sp.) in medieval Flanders was calculated (Figure 2, top). From this growth curve, the surface of the successive annual growth layers can be calculated (annual basal area increment, BAI) (Figure 2, bottom), using the formula:

with i the cambial age of a certain growth ring, what corresponds to the age of the tree when this growth ring was produced (the ring at the center/pith of the tree has a cambial age i = 1), ∏ pi or 3.14, and R the distance from the outer side of a growth ring to the center/pith of the tree (this equals the sum of all ring widths starting from the center/pith of the tree up to the growth ring with a cambial age of i). Consequently, the relative contribution (%) of each successive annual growth ring to the overall surface of a section through the trunk of an oak tree of a given age is calculated, after which the age of each growth ring is multiplied by its own specific relative contribution factor. Adding up the values for all rings produces the average age of the wood of the whole tree (Haneca et al. Reference Haneca, Van Acker and Beeckman2005). From this calculation, it can be estimated that burning a stem of a 100-yr-old tree, releasing the CO2 that accumulated in the wood tissues during the entire lifespan of that tree, is equivalent to burning 34-yr-old wood (Figure 3). Burning a stem of a 200-yr-old tree is equivalent to burning 78-yr-old wood. The old-wood-effect in cremated bone can only reach 100 years when very old trees (>250–300 years) were used as fuel (and all carbon in the bone is replaced by carbon derived from the fuel). Whether such enormous stems would have been used to build a pyre seems unlikely. It is always possible that within the assemblages CH_L was present, much older than assessed by the radiocarbon dates, as per feature only one CH_L sample was analyzed. But it is not very probable. Alternatively, the extent of the bias between the HB and AB dates could point to the use of wood dead for centuries or—perhaps more likely—old construction wood recycled as fuel for the pyre. However, the use of recycled wood still implies that it must have been a century or more in use, before being used as fuel. Where this wood then must have come from remains an interesting historical question, given the scarcity of late Roman sites in Flanders (Van Thienen Reference Van Thienen2016). Finally, one would expect higher percentages of oak (the best suited wood for timber) in the charcoal assemblages from the cremation graves if mainly wood from old buildings had been reused as fuel.

Figure 2 Average growth curve for oak (top), based on ring-width measurement on archaeological timbers from early medieval sites in Flanders (n = 30, data from Haneca Reference Haneca2010, Reference Haneca2011, Reference Haneca2012). The annual basal area increment (BAI) displays the surface covered by the successive growth rings according to the age of the tree (bottom).

Figure 3 Cumulative age of the wood contained in a tree trunk according to the age of that tree (calculated from the data presented in Figure 2).
A further complication, voluntarily ignored until now, is that the old wood hypothesis implies that the phenomenon must have acted more strongly upon the HB than on the AB (assuming that both were cremated on the same pyre, the most likely scenario). This would mean that the AB did not pick up CO2 from the pyre as easily as the HB did. The data even do not exclude that the AB underwent no bias at all, but this would contradict the experimental studies (Hüls et al. Reference Hüls, Nadeau, Grootes, Erlenkeuser and Andersen2010; Zazzo et al. Reference Zazzo, Saliège, Lebon, Lepetz and Moreau2012; Snoeck et al. Reference Snoeck, Brock and Schulting2014). An explanation for the pattern can perhaps be found in the differing morphology and histology of HB versus AB. Nor et al. (Reference Nor, Pastor and Schutkowski2015) summarize the phenomenon as follows: “Owsley et al. (Reference Owsley, Mires and Keith1985) have shown that humans have fewer osteons with larger Haversian canals compared to animals, which have more osteons and with smaller Haversian canals. Thus, diameter of osteon and Haversian canal may be used to form the basis for determining human or animal bone origin (Hillier and Bell, Reference Hillier and Bell2007). Absence of plexiform pattern in human has been also used to differentiate between human and non-human bones, where it is mostly found in non-human bones. Finally, cortical bone thickness may be used to identify human and animal. When viewed in cross-section, the cortex of an adult human bone is one-fourth the total diameter, whereas in large mammals such as dog or bear, the cortex is much thicker that is, one-third the total diameter” (see for further, extensive discussions of the bone structure of humans versus other mammals: Urbanová and Novotný Reference Urbanová and Novotný2005; Martiniaková et al. 2006; Reference Martiniaková, Grosskopf, Omelka, Dammers, Vondráková and Bauerová2007; Hillier and Bell Reference Hillier and Bell2007; Brits et al. Reference Brits, Steyn and L’Abbé2014). That there are differences in structure between HB and AB is thus clear, but whether they explain a possible difference in uptake of CO2 from the pyre is not certain at all. As mentioned, experiments have shown that AB, even when burnt with surrounding flesh, fat and skin, does take up a lot of carbon from the fuel and in fact, regarding this process, no difference could be noted between AB and HB (Zazzo et al. Reference Zazzo, Saliège, Lebon, Lepetz and Moreau2012).
Reservoir Effect
Another possible explanation for the bias on the HB dates can be found in the reservoir effect that makes that, through a restricted exchange in CO2 between water and the atmosphere, there is a depletion of 14C in water bodies compared to the atmosphere (Lanting and van der Plicht Reference Lanting and van der Plicht1998). This effect operates in both marine and freshwater environments and explains why aquatic organisms yield radiocarbon dates that are “too old”, thus showing a bias compared with contemporaneous terrestrial organisms. The marine reservoir effect is roughly similar throughout the world’s surface oceans, amounting to about 400 years (Stuiver and Braziunas Reference Stuiver and Braziunas1993). The freshwater reservoir effect is more complicated and varies widely between water bodies and river basins. It has been studied for the Scheldt basin through a dating program on archaeological fish bones of known age, showing that the freshwater reservoir effect can attain high values, up to more than 1800 radiocarbon years, depending on the fish species and size (Ervynck et al. Reference Ervynck, Boudin and Van Neer2018). Reservoir effects have a marked impact on radiocarbon dates obtained on the archaeological remains of humans (or other organisms) having consumed aquatic organisms as part of their diet (e.g., Cook et al. Reference Cook, Bonsall, Hedges, McSweeney, Boroneant, Bartosiewicz and Pettitt2002, for freshwater organisms as part of the human diet). In the case of inhumated human remains, the reservoir effect can be visible when dating the collagen fraction of the bone, while in the case of cremations one has to assume that the bio-apatite of the bone (after having expelled its “own” carbon) takes up the CO2 of the burning collagen and surrounding flesh and fat (Olsen et al. Reference Olsen, Heinemeier, Hornstrup, Bennike and Thrane2013). Experimental work sheds doubt upon the contribution this scenario can make to the final carbon content of the cremated bone (Snoeck et al. Reference Snoeck, Brock and Schulting2014) and indeed virtually all flesh and collagen must have disappeared when the recrystallisation of the bio-apatite takes place. Still, when only a small portion of the carbon in the cremated bone derives from the collagen, could this not be meaningful given the high values of the freshwater reservoir effect?
The possibility that carbon “with a reservoir effect” is present in the bio-apatite before cremation (Zazzo et al. Reference Zazzo, Saliège, Person and Boucher2009) and that part of that carbon survives the process (even taking into account that the overall carbon content is reduced markedly after burning), even when a lot of CO2 is taken up from the fuel, is not fully exploited by experimental work. Still, with an almost complete carbon replacement in the bio-apatite, this possibility could be of some importance. Of course, it has to be remembered that the origin of the calciumcarbonates in the bio-apatite has to be sought in the whole food spectrum, a markedly less selective pathway than the direct uptake of proteins from the food into the collagen. The reservoir effect will thus always be more pronounced in the organic part of the bone than in the inorganic part.
The bias observed in the dates of the HB from Broechem is considerably smaller than the marine and maximum freshwater reservoir effects. When it is assumed that the people buried at the cemetery consumed considerable quantities of fish, crustaceans or aquatic molluscs, while the pigs, most probably herded in the woods, could not reach these food items (through eating human consumption refuse), could this pattern then be responsible for the anomalous dates of HB in contrast to AB, in contrast to the theoretical objections made above? The human in grave 229 would then have been a non-fish eater. Unfortunately, a major drawback for the Broechem case study remains that it is impossible to prove that the people at the site indeed incorporated aquatic organisms into their diet. Stable isotope analysis (i.e. 13C/12C and 15N/14N ratios) could provide this information, taking advantage from isotope data obtained from archaeological fish samples from the Scheldt basin, the estuary and the North Sea (showing high 15N/14N ratios: Fuller et al. Reference Fuller, Müldner, Van Neer, Ervynck and Richards2012) (Figure 4), but this cannot be done on cremated bone. Additionally, as mentioned before, archaeozoological data from habitation sites are lacking for the region and period considered, making it impossible to check whether fish bones or shells are present among the human consumption refuse.

Figure 4 Carbon and nitrogen stable isotope ratio results from fish taxa found in archaeological sites from Belgium, grouped by ecological type (n = 63, after Fuller et al. Reference Fuller, Müldner, Van Neer, Ervynck and Richards2012).
From early medieval Dutch archaeological sites, it is known that fish was regularly consumed, including both freshwater and marine species (see, e.g., Beerenhout Reference Beerenhout, Dijkstra, Verhoeven and van Straten2016: table 27.30). Also, for Broechem, it can thus be assumed (but not proven) that such dietary patterns occurred. More comparative data comes from stable isotope studies done on two Merovingian populations from Torgny and Ciply, two sites in the southern part of Belgium (Polet and Katzenberg Reference Polet and Katzenberg2002). Surprisingly, these data do not indicate any significant consumption of aquatic resources. When compared with the stable isotope data compiled for archaeological human bones from inhumations from the historical periods, the low values for the 15N/14N ratios at Torgny and Ciply are striking (Figure 5). Of course, this information has possibly no relevance for the interpretation of the dates from Broechem. Moreover, it remains an interesting observation that while the general stable isotope data for humans from the historical periods without doubt indicate (some) fish consumption (an interpretation that is confirmed through archaeozoological research), archaeological experience with Belgian material almost never leads to the impression that the radiocarbon dates obtained are “too old” (Ervynck et al. Reference Ervynck, Boudin, Van den Brande and Van Strydonck2014). Of course, smaller dating biases are perhaps not easily noted in human remains from the historical periods because, after the early Middle Ages, graves mostly no longer contain datable finds that could be used as a check for the radiocarbon dates. A few notable exceptions to this pattern have been found but they remain rare and represent inhumated remains, e.g. the radiocarbon analyses of the relic of Saint Waldetrudis (Van Strydonck et al. Reference Van Strydonck, Boudin and De Mulder2009) and of the skeletons of two historically known bishops buried in the cathedral of Tournai (Boudin et al. Reference Boudin, Boeckx, Vandenabeele and Van Strydonck2014), which in both cases could only be synchronized with the historical context by assuming a freshwater fish reservoir effect (Ervynck et al. Reference Ervynck, Boudin, Van den Brande and Van Strydonck2014).

Figure 5 Carbon and nitrogen stable isotope ratio results from radiocarbon dated human skeletons from the historical periods from Belgium (n = 234, after Ervynck et al. Reference Ervynck, Boudin, Van den Brande and Van Strydonck2014). Added are the averages (± 1σ) for the Merovingian sites of Ciply and Torgny (dark grey dots, after Polet and Katzenberg Reference Polet and Katzenberg2002).
Age of Death
An additional factor that has to be mentioned is the different age of death of the humans and the pigs. Because the former generally live longer, their skeleton could contain a higher frequency of “older” radiocarbon. However, bone continually undergoes remodelling, making that the collagen in a mature bone can only be between a few years old and at most around 30 years old (Price et al. Reference Price, Burton and Bentley2002). The former is the case for very young individuals, the latter for people of considerable age. Unfortunately, as no precise age of death estimations could be made on the basis of the cremated, heavily fragmented human remains from Broechem, this factor cannot further be evaluated. In any case, it cannot fully explain the age difference of a century between the HB and the AB.
Multiple Causes and Equifinality?
From the foregoing discussion it must be concluded that reservoir effects could explain the bias observed on the dates of HB but it cannot be proven that they have been a phenomenon of importance for the Broechem population. Another explanation could be one of the forms of the old wood effect but, except for the scenario of the use of recycled old construction wood (difficult to maintain historically) or old dead wood from the forest, this factor can hardly be responsible for the total range the bias reaches (around a century). Of course, both reservoir and old wood effects can have acted together, an interpretation that, unfortunately, again cannot be proven. Similarly, the younger dates of the AB can perhaps partly be explained by the structure of the material, making it less susceptible to the old wood effect, by the fact that the pigs did not eat fish, or by a combination of both factors. Since it can be assumed that more of the carbon in the cremated bone derives from the fuel rather than from the surrounding collagen, the old wood effect (lower offset) will in a way temper any reservoir effect (much higher offsets). However, this argument does not take into consideration the possibility of some “original” carbon with a reservoir effect remaining within the bio-apatite during the whole cremation process. Finally, the age of death differences between the AB and HB are possibly a further biasing factor. This complexity makes the century difference between the AB and HB dates from Broechem even more intriguing.
The end result of this evaluation must be that virtually all radiocarbon dates put forward for the cremation graves remain uncertain. Taking into account the difficulties interpreting the dates of the charcoal samples with respect to the dated human and animal bones, the HB dates that cannot be checked against AB dates remain unreliable, even when they are contemporaneous with a CH_S date. Upon both HB and CH_S dates a bias can be present. Similarly, when HB and CH_L show contemporaneous dates within a grave, this does not mean that the date of CH_L has determined the date of the HB. The first can be the result of an old wood effect, the latter of a reservoir effect (and possibly an old wood effect too). This problem of equifinality renders all interpretations difficult.
Comparative Studies
Next to a number of laboratory or outdoor experiments (Hüls et al. Reference Hüls, Nadeau, Grootes, Erlenkeuser and Andersen2010; Van Strydonck et al. Reference Van Strydonck, Boudin and De Mulder2010, Zazzo et al. Reference Zazzo, Saliège, Lebon, Lepetz and Moreau2012; Snoeck et al. Reference Snoeck, Brock and Schulting2014), the archaeological case study that first drew attention to the practical implications of a possible old wood effect biasing the dates of cremated human bone, was that of the cremation remains of a child found in a Bronze Age wooden coffin from Egtved, Denmark. The coffin has been dated dendrochronologically to 1370 BC, which is consistent with the cultural artifacts from the grave, but two combined radiocarbon data from the cremation remains prove that they are 73 ± 26 radiocarbon years older (a statistically significant result) (Olsen et al. Reference Olsen, Heinemeier, Hornstrup, Bennike and Thrane2013). A reservoir effect was discarded as a possible explanation in this study.
A radiocarbon dating program for the Late Iron Age cremation cemetery of Westhampnett (southern England, GB) included the comparison between the dates of 8 HB with those of plant remains (in 7 cases) and a cremated sheep bone (one case) from the same features. The interpretation of the plant dates proved to be problematic, while there was no statistically significant difference between the dates of the HB and the sheep bone (Fitzpatrick et al. Reference Fitzpatrick, Hamilton and Haselgrove2017). It should be noted that it is hypothesized that during the Iron Age fish was hardly consumed in the area (Dobney and Ervynck Reference Dobney, Ervynck, Haselgrove and Moore2007). Other studies that compare radiocarbon dates from cremated HB and AB, deriving from the same features, do not seem to exist.
A review of radiocarbon dates on cremated HB from early medieval cemeteries from northwestern Europe, comparing them with the cultural chronologies of associated cultural artifacts, has not been made. Still, it has to be mentioned that from an early medieval funeral site at Borsbeek, located near to Broechem (Figure 1, n° 2), two radiocarbon dates on cremated HB were obtained, that were around a century older than the supposed chronological range of the burials, estimated on the basis of the cultural finds (De Mulder et al. Reference De Mulder, Van Strydonck, Annaert and Boudin2012).
CONCLUSION
The dating program presented has shown that biases can occur on the radiocarbon dates obtained from different find categories from cremation graves, that are large enough to have an impact upon the cultural interpretation of such features (which is certainly important for the historical periods). The causes of these biases are still poorly understood although some influencing factors have been discussed. As a working hypothesis, it could be put forward that both human and animal bone take up CO2 from the pyre, inducing a (mild) old wood effect (leaving out whether this acts differently between the two groups of bone), while uptake from the surrounding flesh and collagen is probably of little importance. A reservoir effect could then still present itself through “original” carbon in the bio-apatite, surviving the cremation process. This explanation assumes fish consumption by the people from Broechem, but not by their pigs.
Without doubt, in the future, experimental work will shed more light upon the dating problems presented. Meanwhile, it is advised that when animal remains are present within a cremation grave, this material should always be dated next to the human remains themselves. This does not mean, however, that the dates obtained from animal bone can be treated without caution. Although no disagreements could be found between the radiocarbon dates from the animal bones and the cultural dates of the grave goods, it remains possible that the first group of dates is still somewhat older than the actual cremation event, as it is probable that also the animal bones incorporated CO2 from the pyre (old wood effect). Given the broad range of the cultural dates, and the uncertainties around the charcoal samples, this remains difficult to evaluate. It has also to be taken into account that a possible small bias on the dates obtained from the animal bones can fall in a range that is difficult to pick up statistically.
The results from Broechem certainly suggest that single dates from cremation contexts can represent misleading results. Samples from human bone, at least in this case, show a bias, while this seems less the case for the cremated animal bone. The interpretation of the dated charcoal samples can suffer from problems with the taphonomy (intrusive or residual material), or their interpretation as representing material much older (CH_L) or almost contemporaneous to the cremation event (CH_S). For cremation contexts in which only human bone is present, and no datable cultural artifacts, these dating problems especially need attention.
ACKNOWLEDGMENTS
The radiocarbon dates have been funded by Flanders Heritage Agency (Belgium) and the Royal Institute for Cultural Heritage (Belgium). The authors are thankful for the valuable comments made on an earlier version of this paper by Dr Antoine Zazzo (associate editor of Radiocarbon) and by two anonymous reviewers.
Supplementary material
To view supplementary material for this article, please visit https://doi.org/10.1017/RDC.2019.159