Introduction
Many polyploid taxa produce larger and/or heavier seeds as compared to related diploids, a feature that may favour speciation, diversification and geographical expansion on the short term (Maceira et al., Reference Maceira, Jacquard and Lumaret1993; Bretagnolle et al., Reference Bretagnolle, Thompson and Lumaret1995; Hoya et al., Reference Hoya, Shibaike, Morita and Ito2007; Urwin et al., Reference Urwin, Horsnell and Moon2007; Eliásová and Münzbergová, Reference Eliásová and Münzbergová2017). Indeed, the gigas effect on seeds has been found to positively affect the germination capacity, both in final percentage and in rate (Bretagnolle et al., Reference Bretagnolle, Thompson and Lumaret1995; Hoya et al., Reference Hoya, Shibaike, Morita and Ito2007; Eliásová and Münzbergová, Reference Eliásová and Münzbergová2014) and the production of larger seedlings (Moles and Westoby, Reference Moles and Westoby2004), which in turn may enhance plant establishment.
Plant recruitment from seeds is usually studied to investigate the environmental drivers shaping the ecological niche in both seed germination and seedling establishment (Poschlod et al., Reference Poschlod, Mehdi, Bartelheimer, Drobnik, Rosbakh, Saatkamp, Van der Maarel and Franklin2013). For instance, these drivers include several physical and biotic cues (reviewed by Donohue et al., Reference Donohue, de Casas, Burghardt, Kovach and Willis2010; Baskin and Baskin, Reference Baskin and Baskin2014). On the contrary, there are only a few studies addressing the effect of ploidy level on germination (Bretagnolle et al., Reference Bretagnolle, Thompson and Lumaret1995; Hoya et al., Reference Hoya, Shibaike, Morita and Ito2007; Broadhurst et al., Reference Broadhurst, Murray, Forrester and Young2012; Carta et al., Reference Carta, Probert, Moretti, Peruzzi and Bedini2014; Eliásová and Münzbergová, Reference Eliásová and Münzbergová2014), and in none of them embryo growth was taken into account. In this context, the role of the relative embryo size (usually expressed as the ratio of the embryo to seed length) is crucial from the ecological point of view (see Nikolaeva, Reference Nikolaeva and Khan1977; Vandelook et al., Reference Vandelook, Janssens and Probert2012; Carta et al., Reference Carta, Probert, Moretti, Peruzzi and Bedini2014; Blandino et al., Reference Blandino, Fernández-Pascual, Marin, Vernet and Pritchard2019). The importance of embryo growth, for instance, makes sense especially in those species that produce underdeveloped embryos at dispersal, and which are only able to germinate when the embryo reaches a critical length (Nikolaeva, Reference Nikolaeva and Khan1977). This seed property has been documented in several phylogenetically unrelated angiosperm families, from Ranunculaceae to Apiaceae (Baskin and Baskin, Reference Baskin and Baskin2014), although the absence of growth of the embryo prior to germination is also reported (Vandelook et al., Reference Vandelook, Van de Vyver and Carta2019). For instance, many Eurasian geophytes possess underdeveloped embryos, including many Liliaceae (Carasso et al., Reference Carasso, Hay, Probert and Mucciarelli2011; Mondoni et al., Reference Mondoni, Rossi and Probert2012; Baskin and Baskin, Reference Baskin and Baskin2014), such as representatives of Tulipa (Martin, Reference Martin1946; Tang et al., Reference Tang, Tian and Long2009; Baskin and Baskin, Reference Baskin and Baskin2014). This genus includes several species inhabiting open habitats, among which the only ones native to western Europe, namely Tulipa pumila Moench and Tulipa sylvestris L.; the latter presumably originated from the former via autopolyploidy (Cesca, Reference Cesca1986). This species reproduces sexually, and populations with functionally male flowers have been reported (Peruzzi, Reference Peruzzi2012), sharing this feature with the diploid ancestor T. pumila (Astuti et al., Reference Astuti, Pratesi, Carta and Peruzzi2020).
As many other bulbous geophytes in the Mediterranean area (e.g. Doussi and Thanos, Reference Doussi and Thanos2002; Carta et al., 2016, Reference Carta, Skourti, Mattana, Vandelook and Thanos2017), tulips are reported to preferentially germinate at cool temperature and in the dark (Tang et al., Reference Tang, Tian and Long2009; Baskin and Baskin, Reference Baskin and Baskin2014), except the desert species Tulipa systola Stapf, which seems insensitive to light exposure (Boeken and Gutterman, Reference Boeken and Gutterman1990).
Considering the alleged gigas effect displayed by polyploids, we compared seed mass and size in T. pumila and T. sylvestris. Then, we monitored embryo growth and radicle protrusion of both species under laboratory conditions to evaluate putative differences in germinability in terms of both percentage and rate.
Materials and methods
We sampled seeds and bulbs of T. pumila from one population growing in open dry habitats at Doccino (43°23′54″N, 10°37′19″E) (Riparbella, Pisa; Tuscany, central Italy). Seeds and bulbs of T. sylvestris were collected from one population growing in olive groves at Le Rose (43°43′15″N, 11°13′40″E) (Impruneta, Firenze; Tuscany, central Italy). Fruits of both species were collected in the field on June 2012 from around 50 individuals per species and processed in the laboratory. Empty or not fully developed seeds were discarded. Seed sowing was started within 1 month after collection, to avoid conservation procedures that may affect dormancy (Tang et al., Reference Tang, Tian and Long2009) and longevity (Baskin and Baskin, Reference Baskin and Baskin2014).
For seed mass, we weighed five batches of 30 randomly selected seeds for each species (accuracy of 1 × 10−4 g). Then, we measured seed size (length, width and thickness of 30 randomly chosen seeds for each species (resolution of 5 × 10−5 m)). For seed mass and size, a t-Student was applied.
Seeds used for monitoring embryo growth and radicle protrusion were sown in Petri dishes with 1% agar placed under the best temperature (7°C) and light (in the dark) conditions of germination according to previous studies (Van Tuyl and Van Creij, Reference Van Tuyl, Van Creij and Anderson2006; Carta et al., Reference Carta, Skourti, Mattana, Vandelook and Thanos2017) and our preliminary results (Fig. 1). We measured, by means of a hand calliper, the embryo and seed length every week in ten seeds for each species (Fig. 2). Then, we calculated the embryo length to seed length ratio (E:S hereafter). For calculating the mean E:S at radicle protrusion, we measured the embryo length at radicle protrusion in each seed, and we calculated the mean critical E:S for each species.

Fig. 1. Germination proportion (±95% CI) at different temperatures in T. pumila and T. sylvestris.

Fig. 2. Embryo growth progress in seeds of T. pumila. From left to right: at incubation, after 30 days of incubation and after 80 days of incubation. Bar = 1 mm.
For seed germination, we performed tests using 100 seeds for each species. Seeds were considered germinated only when the radicle reached 1 mm in length. We calculated the mean germination time (MGT hereafter) according to the formula:

where n i is the number of seeds germinated in the specific interval of time, t i is the time elapsed since the start of the test to the end of the specific interval of measurement and N is the total number of germinated seeds. The number of germinated seeds was recorded approximately every week. At the end of the germination test, we performed a cut test on those seeds that did not germinate to evaluate the number of not viable seeds.
In order to check the ploidy level of the studied populations, root tips from potted bulbs were pretreated with 0.4% colchicine and stained with leuco-basic fuchsine after hydrolysis in HCl 1 N at 60°C for 7 min. For chromosome counting, stained root tips were squashed on a drop of acetic orcein and observed under a light microscope.
Results
Mean weight, length, width and thickness of seeds are reported in Table 1. Seeds of both species are flattened but distinctly three-dimensional, light-coloured and smooth, with copious endosperm and a well-distinct underdeveloped embryo at the acute apex with an E:S value at the dispersal of ca. 0.25 in both species. Seeds of T. sylvestris were significantly heavier (t = 10.58, df = 4, P < 0.01) and larger (length: t = 2.92, df = 29, P < 0.01; thickness: t = 3.30 df = 29, P < 0.01; width: t = 0.95, df = 29, P > 0.05) than those of T. pumila.
Table 1. Seed mass and size comparison between T. pumila and T. sylvestris

For each feature the mean and the standard deviation are given.
Progress of embryo growth is shown in Fig. 3. Although the mean E:S at germination was similar and not significantly different (t = 1.995, df = 9, P > 0.05) in the two species (ca. 0.91 in T. pumila, 0.87 in T. sylvestris), the embryo growth of T. sylvestris showed an unimodal progress and its rate was faster (ca. 48 days) as compared to T. pumila (ca. 97 days). In the latter species, instead, the embryos grew at two different rates (bimodal progress), increasing rapidly in the first 28 days, after which they slowly reached their critical length.

Fig. 3. Embryo growth curves (±SE) and cumulative germination (±95% CI), in the diploid T. pumila and tetraploid T. sylvestris.
The radicle protrusion curves of both species are shown in Fig. 3. Radicle protrusion in T. sylvestris was completed in 76 days, with MGT = 46.03 days. On the contrary, T. pumila germination was much more lagged, with MGT = 74.20 days, and was completed after 104 days. Interestingly, while 50% of germination was reached much more earlier in T. sylvestris, E:S of 0.5 was reached roughly at the same time in both the species. Nevertheless, final germination was close to 100% in both the species.
From the karyological analysis, we counted 2n = 48 chromosomes for T. sylvestris (Fig. 4) and 2n = 24 chromosomes for T. pumila (not shown).
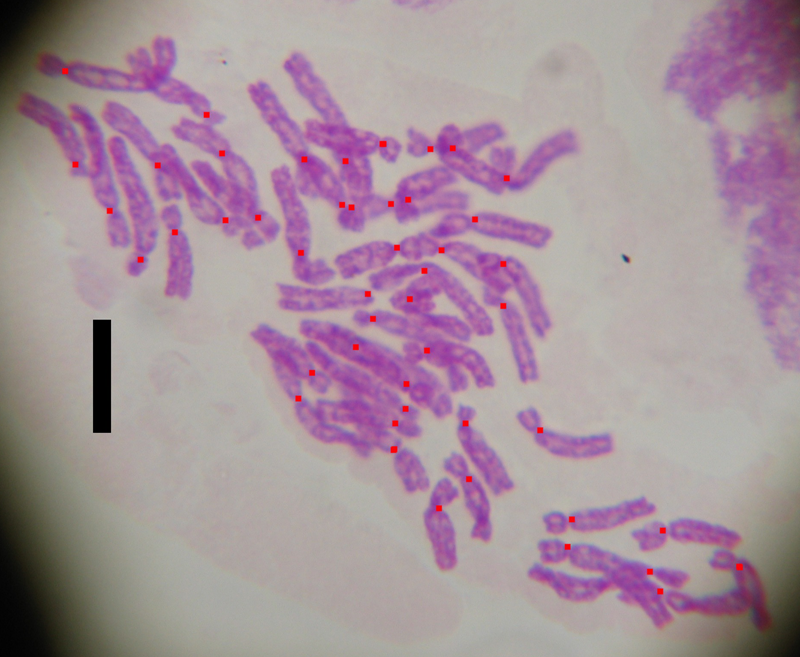
Fig. 4. Metaphasic plate of T. sylvestris from Le Rose (2n = 4x = 48). Red dots indicate the centromeres. Scale bar = 10 μm.
Discussion
Seeds of both the species germinated completely, without the need for a preliminary disruption of a physiological block by a cold stratification period. Hence, the cold period stimulating embryo growth suggests that seeds cannot be considered physiologically dormant, but rather as morphologically dormant according to the classification proposed by Baskin and Baskin (Reference Baskin and Baskin2004). Particularly, for our Tulipa species we could draw the same conclusion made by Carasso et al. (Reference Carasso, Hay, Probert and Mucciarelli2011) for a Fritillaria species (Liliaceae): seeds simply germinate slowly under a range of low temperatures without any lag between embryo growth, radicle protrusion and cotyledon emergence, without physiological and epicotyl dormancy.
Tulipa sylvestris and T. pumila were confirmed to be tetraploid and diploid, respectively, also in our studied populations. Tulipa sylvestris has larger and heavier seeds than T. pumila. Indeed, polyploids have a larger genome size, which is considered one of the main drivers in yielding divergence in seed size among angiosperms (Beaulieu et al., Reference Beaulieu, Leitch and Knight2007). Here, larger and heavier seeds are paralleled by faster embryo growth and germination rates in T. sylvestris, as found in other polyploids (Bretagnolle et al., Reference Bretagnolle, Thompson and Lumaret1995; Hoya et al., Reference Hoya, Shibaike, Morita and Ito2007; Broadhurst et al., Reference Broadhurst, Murray, Forrester and Young2012; Eliásová and Münzbergová, Reference Eliásová and Münzbergová2014). In T. sylvestris, indeed, the germination was significantly faster, with an anticipation of ca. 28 days as compared to T. pumila. Two main explanations have been provided for the faster germination of polyploids: (1) they are better in mobilizing seed reserves (von Well and Fossey, Reference Von Well and Fossey1998) or (2) larger seeds induce faster germination (Thompson, Reference Thompson1990). However, although the seeds of the two species are different in size and weight, they share similar starting E:S and mean E:S at germination, meaning that ploidy level likely does not affect the contribution of endosperm and/or integuments in controlling the whole seed structure. Furthermore, we neither found a higher proportion of germination in polyploids, as found in other genera such as Dactylis (Bretagnolle et al., Reference Bretagnolle, Thompson and Lumaret1995), Taraxacum (Hoya et al., Reference Hoya, Shibaike, Morita and Ito2007) and Vicia (Eliásová and Münzbergová, Reference Eliásová and Münzbergová2014), nor a significant germination decrease as reported by Cohen et al. (Reference Cohen, Fait and Tel-Zur2013) and Hosseini et al. (Reference Hosseini, Chehrazi, Sorestani and Ahmadi2013).
As seeds from both the species showed high viability, we did not find evidence of different performance in germination caused by polyploidy. Instead, we have shown that T. pumila and T. sylvestris show a different seed germination rate, and cytotype variation may be considered the reason for these germination differences as previously detected in other species (Hoya et al., Reference Hoya, Shibaike, Morita and Ito2007; Broadhurst et al., Reference Broadhurst, Murray, Forrester and Young2012; Eliásová and Münzbergová, Reference Eliásová and Münzbergová2014). It is possible, however, that the faster germination of the polyploid species represents a local adaptation to a new environment (Donohue et al., Reference Donohue, de Casas, Burghardt, Kovach and Willis2010) different from that inhabited by the diploid ancestors. Indeed, as also observed in our target populations, the two species show a peculiar ecological shift in habitat preference, which makes it difficult to disentangle to which extent habitat conditions rather than ploidy level contribute to the difference found here, especially concerning seed mass. It is possible that the poorer soils typically colonized by T. pumila negatively affect its seed size. In conclusion, it should be remarked that the germination at dispersal does not necessarily reflect the fitness potential of seeds in the natural environment, a discrepancy already highlighted in studies dealing with pollination and mating systems (Carta et al., Reference Carta, Bedini, Giannotti, Savio and Peruzzi2015; Baskin and Baskin, Reference Baskin and Baskin2019). Hence, we recommend a more cautionary approach when dealing with studies on ploidy level and germination to avoid misinterpretation of the results when tested on natural conditions.
Acknowledgements
We would like to thank Filip Vandelook (Meise Botanic Garden, Belgium) and two anonymous reviewers for their valuable comments.