Introduction
Clathrodictyid stromatoporoids form the dominant stromatoporoid group; they became cosmopolitan during the Silurian and were abundant until the end of the Devonian (Nestor, Reference Nestor1997; Nestor and Stock, Reference Nestor and Stock2001), surviving the Frasnian–Famennian extinction (Webby et al., Reference Webby, Stearn, Nestor and Selden2015, fig. 363). This group is characterized by weakly differentiated and inflected laminae with short to superposed pillars (Nestor, Reference Nestor1997, Reference Nestor and Selden2015) and is regarded as one of the most important early Paleozoic stromatoporoids involved in the establishment of mid-Paleozoic coral–stromatoporoid metazoan reefs (Nestor, Reference Nestor1997; Copper, Reference Copper, Kiessling, Flügel and Golonka2002, Reference Copper2011; Stearn, Reference Stearn and Selden2015a). Their early appearance was recorded in the middle Katian Stage of the Late Ordovician, and by then they were already widely distributed (Bolton, Reference Bolton1988; Lin and Webby, Reference Lin and Webby1988; Nestor et al., Reference Nestor, Copper and Stock2010; Nestor and Webby, Reference Nestor and Webby2013; Stock et al., Reference Stock, Nestor, Webby and Selden2015; Webby, Reference Webby and Selden2015a), in accordance with the wide distribution of Ordovician metazoan reefs (Webby, Reference Webby, Kiessling, Flügel and Golonka2002; Stock et al., Reference Stock, Nestor, Webby and Selden2015). The early diversification of clathrodictyid stromatoporoids involved four genera (i.e., Clathrodictyon, Ecclimadictyon, Camptodictyon, and Labyrinthodictyon); it occurred in the middle Katian in central New South Wales and spread to the Tasmanian Shelf and South China (peri-Gondwanan terranes), as well as Laurentia and Baltica (Webby, Reference Webby, Webby, Paris, Droser and Percival2004, Reference Webby and Selden2015a; Stock et al., Reference Stock, Nestor, Webby and Selden2015; Webby et al., Reference Webby, Stearn, Nestor and Selden2015; Jeon et al., Reference Jeon, Liang, Park, Choh and Lee2020a).
In South China, clathrodictyids are commonly recorded as significant reef components on the northern margin of the Cathaysian landmass (e.g., Yu et al., Reference Yu, Bian, Huang, Chen, Fang, Zhou and Shi1992; Chen, Reference Chen1995, Reference Chen1996; Bian et al., Reference Bian, Fang, Huang and Fan1996; Li et al., Reference Li, Kershaw and Mu2004; Zhang et al., Reference Zhang, Chen, Yu, Goldman and Liu2007). The Jiangshan–Changshan–Yushan (JCY) triangle, on the border between Jiangxi and Zhejiang provinces of south-eastern China (Fig. 1), is particularly well known for the development of clathrodictyid-dominated reefs (Yu et al., Reference Yu, Bian, Huang, Chen, Fang, Zhou and Shi1992; Bian et al., Reference Bian, Fang, Huang and Fan1996; Li et al., Reference Li, Kershaw and Mu2004; Wang et al., Reference Wang, Deng, Wang and Li2012; Park et al., Reference Park, Lee, Liang and Choh2021). Recent investigations of Late Ordovician stromatoporoids from the Xiazhen and coeval Sanqushan formations of the JCY triangle (Jeon et al., Reference Jeon, Liang, Park, Choh and Lee2020a, Reference Jeon, Li, Na, Liang and Zhang2022a) revealed that clathrodictyids played a critical role in a relatively complex reef community. However, species-level taxonomic work on clathrodictyids has not been conducted. This study, therefore: (1) presents a new detailed taxonomic description of clathrodictyid stromatoporoids from the Upper Ordovician Xiazhen Formation at Zhuzhai, Jiangxi Province, China; (2) links taxonomy with paleoecological features; and (3) applies this information toward understanding the global development of stromatoporoids as part of the Great Ordovician Biodiversification Event (GOBE). The results emphasize the importance of early fossil records of clathrodictyids in the development of stromatoporoids in the Late Ordovician.

Figure 1. (1) Locality of the study area in China. (2) Location of the Zhuzhai section, Yushan County, Jiangxi Province, near the border with Zhejiang Province. (3) Geological map of the Xiazhen Formation at Zhuzhai section, which is divided into three partly coeval subsections, ZU1, ZU2, and ZU3. Modified after Lee et al. (Reference Lee2012).
Geological setting
The Upper Ordovician Xiazhen Formation at Zhuzhai, Yushan County, Jiangxi Province, is among the classic representatives of Upper Ordovician carbonate successions in South China (Fig. 1). The formation is characterized by mixed carbonate–clastic deposits and is situated on the Zhe-Gan Platform along the northern margin of the Cathaysian landmass (Li et al., Reference Li, Kershaw and Mu2004; Zhang et al., Reference Zhang, Chen, Yu, Goldman and Liu2007; Park et al., Reference Park, Lee, Liang and Choh2021). The depositional environment is interpreted to have been a mixed siliciclastic–carbonate ramp-type platform (Park et al., Reference Park, Lee, Liang and Choh2021). The Zhuzhai section of the formation can be divided into three partially correlated subsections, ZU1, ZU2, and ZU3 (Lee et al., Reference Lee2012; Figs. 1.3, 2), separated by Quaternary sedimentary deposits (Fig. 1.3). The stratigraphy of the Zhuzhai section was revised and divided into four members: a lower limestone member, a lower shale member, a middle mixed-lithology member, and an upper shale member in stratigraphic ascending order (Lee et al., Reference Lee2012; Fig. 2). These subdivisions are not formally defined and are used herein on an informal basis. Comprehensive paleontological studies on many fossil groups from the formation have covered algae (Bian and Zhou, Reference Bian and Zhou1990; Kwon et al., Reference Kwon, Park, Choh, Lee and Lee2012; Lee et al., Reference Lee, Park, Tien, Choh, Elias and Lee2016a, Reference Lee, Elias, Choh and Lee2019), brachiopods (Zhan et al., Reference Zhan, Rong, Jin and Cocks2002), bryozoans (Zhang et al., Reference Zhang, Xia, Taylor, Liang and Ma2018), corals (Dai et al., Reference Dai, Liu, Lee, Peng and Miao2015; Lee et al., Reference Lee, Elias, Choh and Lee2016b; Liang et al., Reference Liang, Elias, Choh, Lee and Lee2016; Sun et al., Reference Sun, Elias, Choh, Lee, Wang and Lee2016; Zhang, Reference Zhang2016), and trilobites (Lee, Reference Lee2013), but little information is available for clathrodictyid stromatoporoids (Chen et al., Reference Chen, Rong, Qiu, Han, Li and Li1987; Chen, Reference Chen1995, Reference Chen1996; Lee et al., Reference Lee2012). The Xiazhen Formation is roughly correlative to the Sanqushan and Changwu formations judging from the fossil components such as brachiopods and corals and was previously estimated to be of middle to late Katian age (e.g., Zhan et al., Reference Zhan, Rong, Jin and Cocks2002; Zhang et al., Reference Zhang, Chen, Yu, Goldman and Liu2007). The discovery of the graptolite Anticostia uniformis (Mu and Lin in Mu et al., Reference Mu, Li, Ge, Chen, Lin and Ni1993) in the base of the upper shale member confirmed that the upper part of the formation is within the Dicellograptus complanatus to Paraorthograptus pacificus Biozone (Diceratograptus mirus Subzone), indicating a late Katian age (Chen et al., Reference Chen, Kim, Choh, Lee and Chen2016).

Figure 2. Stratigraphic column of the Xiazhen Formation with the 18 stromatoporoid-bearing intervals. The red-colored intervals (S1–S5, S7, S9–S18, and lower and upper parts of subsection ZU3) indicate where clathrodictyid stromatoporoids were found. The black-colored intervals (S6 and S8) indicate where only labechiid stromatoporoids occur. C = claystone; M = mudstone or lime mudstone; W = wackestone; P = packstone; G = grainstone, F = floatstone or framestone; R = rudstone; LLM = lower limestone member; LSM = lower shale member; MMM = middle mixed-lithology member; USM = upper shale member. Modified after Lee et al. (Reference Lee2012) and Park et al. (Reference Park, Lee, Liang and Choh2021). A large size version of this figure is presented in Supplementary Data 1.
Materials and methods
Approximately 420 specimens were collected from the 18 stromatoporoid-bearing intervals (Figs. 2, 3), of which over 300 belong to clathrodictyid stromatoporoids. In the lower limestone member, only two clathrodictyid specimens were collected. No stromatoporoids were found in the lower shale member, interpreted to be deposited below wave base (Park, Reference Park2017) and beyond the threshold of stromatoporoid survival. Most specimens were collected from the S2 to S9 intervals of subsection ZU2 and the S9 to S18 intervals of subsection ZU1, while only a few fragmented clathrodictyid samples were collected from ZU3, which is correlated to the upper part of ZU 1 (Figs. 2, 3; see Lee et al., Reference Lee2012, figs. 8, 9). From this collection, 239 stromatoporoid samples were selected, and 420 thin sections were made for taxonomic studies. These include specimens used by Lee et al. (Reference Lee, Elias, Choh and Lee2016b) and Jeon et al. (Reference Jeon, Liang, Park, Choh and Lee2020a, Reference Jeon, Liang, Lee and Kershawb). The suprageneric taxonomic assignment and terminology used in this study follow those of Nestor (Reference Nestor and Selden2015) and Webby (Reference Webby and Selden2015b).

Figure 3. Lithology, interpreted depositional energy level, stratigraphic distributions of clathrodictyid stromatoporoids, and their growth forms from each stromatoporoid-bearing interval of the Xiazhen Formation; SBI = stromatoporoid-bearing interval; M = mudstone; W = wackestone; P = packstone; G = grainstone; L–S couplets = limestone–shale couplets in wackestone to packstone; F = floatstone; Fr = framestone; R = rudstone; L = low-energy depositional environment; M = medium-energy depositional environment; H = high-energy depositional environment; Cl. id. = Clathrodictyon idense; Cl. cf. mmll. = Clathrodictyon cf. Cl. mammillatum; Cl. cf. mc. = Clathrodictyon cf. Cl. microundulatum; Cl. mglm. = Clathrodictyon megalamellatum Jeon n. sp.; Cl. pl. = Clathrodictyon plicatum; E. nst. = Ecclimadictyon nestori; E. und. = Ecclimadictyon undatum; Cam. amz. = Camptodictyon amzassensis; L. csc. = Labyrinthodictyon cascum.
Network analysis is applied to evaluate the global paleobiogeographic affinity of Ordovician clathrodictyids, illustrated as a visible network diagram. The occurrences of clathrodictyid species are organized as a binary data set (i.e., terrane and clathrodictyid species). We used the software Gephi version 0.9.2 (Bastian et al., Reference Bastian, Heymann and Jacomy2009) to perform the network analysis. In the network analysis diagram, a source node (i.e., terrane) is connected to a target node (i.e., clathrodictyid species) by an edge (i.e., line). An endemic clathrodictyid species is represented by a target node linked to only a single source node. A cosmopolitan species is connected with more than one target node, and larger node size indicates a higher degree of cosmopolitanism. From the various display options within Gephi, Force Atlas 2 was chosen to display the clathrodictyid data as the most appropriate for the purposes of this study. The following parameters were involved in this study: scaling 10.0, gravity 1.0, edge weight influence 1.0, number of threads 3, tolerance 1.0, and approximation 1.2.
The data set of the Ordovician clathrodictyid stromatoporoids for the network analysis was collected from previously published data as well as data in this study, including 27 species from South China (Lin and Webby, Reference Lin and Webby1988; Jeon et al., Reference Jeon, Li, Na, Liang and Zhang2022a; this study), North China (Lin and Webby, Reference Lin and Webby1988), Qaidam (Lin and Webby, Reference Lin and Webby1988), Tarim (Dong and Wang, Reference Dong and Wang1984), New South Wales (Webby, Reference Webby1969; Webby and Morris, Reference Webby and Morris1976; Pickett and Percival, Reference Pickett and Percival2001), Tasmania (Webby and Banks, Reference Webby and Banks1976), Altai–Sayan Fold Belt (Khalfina, Reference Khalfina1960), Laurentia (Bolton, Reference Bolton1988; Nestor et al., Reference Nestor, Copper and Stock2010), and Baltica (Nestor, Reference Nestor1964; Bogoyavlenskaya, Reference Bogoyavlenskaya1973). A few previously reported clathrodictyid species are not included (e.g., Bol'shakova and Ulitina, Reference Bol'shakova and Ulitina1985; Khromych, Reference Khromykh2001; Jiang et al., Reference Jiang, Sun, Bao and Wu2011) because of their problematic taxonomic assignments or a lack of detailed description and illustration.
Repository and institutional abbreviation
All the clathrodictyid stromatoporoid specimens from the Xiazhen Formation are deposited in the Nanjing Institute of Geology and Palaeontology (NIGP), Chinese Academy of Sciences, Nanjing, China.
Systematic paleontology
Phylum Porifera Grant, Reference Grant1836
Class Stromatoporoidea Nicholson and Murie, Reference Nicholson and Murie1878
Order Clathrodictyida Bogoyavlenskaya, Reference Bogoyavlenskaya1969
Family Clathrodictyidae Kühn, Reference Kühn1939
Clathrodictyon Nicholson and Murie, Reference Nicholson and Murie1878
Type species
Clathrodictyon vesiculosum Nicholson and Murie, Reference Nicholson and Murie1878.
Clathrodictyon idense Webby and Banks, Reference Webby and Banks1976
Figure 4
- Reference Webby and Banks1976
Clathrodictyon idense Webby and Banks, p. 130, pl. 1, figs. 1, 2.

Figure 4. Thin-section photographs of Clathrodictyon idense Webby and Banks, Reference Webby and Banks1976 from the S3 interval. (1) Longitudinal section of dendroid growth form of Clathrodictyon idense, NIGP 177104-1. (2, 3) Enlargements of the rectangular areas in (1), showing longitudinal section and axial part. Note sharply folded, crumpled laminae in the axial part of (3). (4) Tangential section, NIGP 177103. (5) Tangential section of single (left) and branching (right) dendroid forms of Clathrodictyon idense, NIGP 177106. Note that a thin laminar form encrusted on the branching dendroid form (right).
Type specimen
Holotype UTGD 58125 from the Gordon Limestone Subgroup, southwest of Ida Bay, Tasmania, precise stratigraphic horizon and location unknown (Webby and Banks, Reference Webby and Banks1976, p. 130, pl. 1, figs. 1, 2); deposited in University of Tasmania, Hobart, Australia.
Occurrence
The S2–4, S7, and S9 intervals of the Xiazhen Formation (Upper Ordovician, Katian) at Zhuzhai, Yushan County, Jiangxi Province, China (Figs. 2, 3).
Description
Columnar to dendroid forms of skeletons (up to 100 mm high and 7 mm wide) dominate; fragmented low domical and bulbous examples (up to 40 mm high and 50 mm wide) are also common. Skeletons exhibit a variety of orientations. Astrorhizae, latilaminae, and mamelons are not observed.
Laminae are continuous, moderately wavy, and regularly spaced, ranging from 0.05 to 0.23 mm thick (number of measured laminae = 121, average 0.13 mm); five to seven laminae per millimeter. Pillars are short, stout, and rod-like. Galleries are commonly elongated and round, ranging from 0.10 to 1.15 mm wide (number of measured galleries = 121, average 0.42 mm).
In the axial part of the columnar and dendroid growth forms, zigzag-shaped cassiculate skeletal structure is well developed. The structure is sharply folded and radially upward arranged, which resembles the Ecclimadictyon-like cassiculate structure. During growth, it gradually changed to the normally laminated skeletal phase (Fig. 4.1, 4.3).
Materials
Twenty-seven specimens: NIGP 159424, 159440, 159441, 177100–177113 from the S2–S3 interval, NIGP 177114 and 177115 from the S7 interval, NIGP 177116–177123 from the S9 interval of the Xiazhen Formation (Upper Ordovician, Katian) at Zhuzhai, Yushan County, Jiangxi Province, China.
Remarks
The present Xiazhen specimens show close morphological resemblance to those of Clathrodictyon idense Webby and Banks, Reference Webby and Banks1976 from the Gordon Limestone Subgroup, southwest of Ida Bay, Tasmania. The Tasmanian specimens are similar to the present specimens in features of laminae, exhibiting 10–12 laminae within two millimeters (Webby and Banks, Reference Webby and Banks1976). However, the Tasmanian specimens are restricted to sheet-like growth forms (Webby and Banks, Reference Webby and Banks1976), in contrast to the present specimens, which show a much wider spectrum of growth forms (Fig. 3).
Clathrodictyon megalamellatum Jeon new species
Figure 5
Type specimen
Holotype with three thin sections, NIGP 177124-1–3 from the S18 interval of the Xiazhen Formation at Zhuzhai, Yushan County, Jiangxi Province, China.

Figure 5. Holotype specimen of Clathrodictyon megalamellatum Jeon n. sp. from the S18 interval. (1, 3, 4) Longitudinal sections of Clathrodictyon megalamellatum Jeon n. sp. showing widely spaced laminae and well-developed pillars: (1) NIGP 177124-1; (3, 4) NIGP 177124-2. (2) Enlargement of the rectangular area in (1). (5–7) Tangential sections of Clathrodictyon megalamellatum Jeon n. sp. showing irregularly developed pillars with some circular structure, particularly in (5): (5, 6) NIGP 177124-1; (7) 177124-2. (8) Enlargement of the rectangular area in (4) showing astrorhizae-like structures in the mamelon-like up-growth areas.
Diagnosis
Clathrodictyon with laminae planar, continuous, laterally well developed and widely spaced, ranging from 1.05 to 4.21 mm thick (two to three per millimeter); pillars rod- to funnel-like and crumpled; galleries varying from slightly round to angular, ranging from 0.17 to 1.60 mm wide; microstructure compact.
Occurrence
The S18 interval of the Xiazhen Formation (Upper Ordovician, Katian) at Zhuzhai, Yushan County, Jiangxi Province, China (Figs. 2, 3).
Description
Skeleton is low domical, up to 98 mm wide and 45 mm high. Astrorhizae and latilaminae are not found, but mamelon-like upward-arching areas are common. Laminae are continuous, planar, and notably widely spaced, ranging from 1.05 to 4.21 mm thick (number of measured laminae = 92, average 2.62 mm), laminae spaced two to four per millimeter (mostly three). Wavy and slightly undulating laminae are rarely seen. In longitudinal section, pillars are well defined and commonly extend to interlaminar space, varying from rod- to funnel-like shapes. In tangential section, pillars range from round to elongate. Galleries vary from slightly round to angular shapes, ranging from 0.17 to 1.60 mm (number of measured galleries = 88, average 0.53 mm) wide.
Etymology
Combination of Greek mégas, meaning large, and Latin lamella, meaning thin plate or layer; derived from its morphological feature of widely spaced laminae with well-developed pillars, clearly distinguishable from other previously known Clathrodictyon species found in Late Ordovician strata.
Material
One specimen, NIGP 177124-1–3, from the S18 interval of the Xiazhen Formation (Upper Ordovician, Katian) at Zhuzhai, Yushan County, Jiangxi Province, China.
Remarks
The new species is most easily distinguished by having the most widely spaced laminae of any known Ordovician Clathrodictyon. One of the typical skeletal features of Clathrodictyon is irregularly wrinkled laminae (Nestor, Reference Nestor and Selden2015), but wavy laminae are less common in Clathrodictyon megalamellatum Jeon n. sp. Its planar laminae (Fig. 5.1–5.4, 5.8) are rather like those of the genus Petridiostroma, which is characterized by planar and continuous laminae (Nestor, Reference Nestor and Selden2015). However, in terms of pillars, the new species is closer to the genus Clathrodictyon in morphological features, possessing a variety of rod-like, funnel-like, and crumpled morphologies in both longitudinal and tangential sections (Fig. 5). Petridiostroma is first known in the early Silurian (middle Llandovery), much later than the appearance of Clathrodictyon in the Late Ordovician (middle Katian) (Stock et al., Reference Stock, Nestor, Webby and Selden2015; Webby et al., Reference Webby, Stearn, Nestor and Selden2015). Although this new species possesses similar morphological features to both Clathrodictyon and Petridiostroma, it is regarded here as a species of Clathrodictyon because of the similarity of pillars and stratigraphic occurrences.
Clathrodictyon cf. Cl. mammillatum (Schmidt, Reference Schmidt1858)
Figure 6
- cf. Reference Schmidt1858
Stromatopora mammillata Schmidt, p. 232.
- cf. Reference Rosen1867
Stromatopora mammillata; Rosen, p. 71, pl. 8, figs. 1–5.
- cf. Reference Nestor1964
Clathrodictyon mammillatum; Nestor, p. 42, pl. 13, figs. 7, 8.
- Reference Webby1969
Clathrodictyon aff. mammillatum; Webby, p. 657, pl. 126, figs. 3–5.
- non cf. Reference Yang and Dong1980
Clathrodictyon mammillatum; Yang and Dong, p. 397, pl. 1, figs. 5, 6.
- Reference Lin and Webby1988
Clathrodictyon cf. mammillatum; Lin and Webby, p. 242, fig. 5a–e.
- Reference Jeon, Li, Na, Liang and Zhang2022
Clathrodictyon cf. mammillatum; Jeon et al., p. 60, figs. 2a, b, 3a, b, 4a, b.

Figure 6. (1) Longitudinal to tangential view of Clathrodictyon cf. Cl. mammillatum from the S18 interval, NIGP 169634. (2) Longitudinal section from the S18 interval, NIGP 177134. (3) Enlargement of the rectangular area in (2). (4) Longitudinal section of dendroid form showing sharply folded, crumpled Ecclimadictyon-like laminae in the axial part from the S9 interval, NIGP 177127-1. (5) Tangential section from the S9 interval, NIGP 177130.
Occurrence
The S1, S3, S9, S11, S14–16, and S18 intervals of subsections ZU1 and ZU2 and the lower part of ZU3 of the Xiazhen Formation (Upper Ordovician, Katian) at Zhuzhai, Yushan County, Jiangxi Province, China (Figs. 2, 3).
Description
Growth form of skeletons varies from laminar, low domical, dendroid, to irregular, up to 80 mm wide and 50 mm high, and commonly fragmented. Mamelons range from 1.89 to 3.90 mm in diameter (number of measured mamelons = 8, average 2.94 mm), separated by around 3 mm and up to 5.35 mm. Astrorhizae and latilaminae are not found.
Laminae vary from slightly wrinkled, laterally continuous, and well developed, ranging from 0.07 to 0.45 mm thick (number of measured laminae = 113, average 0.14 mm) with a spacing of four to eight laminae per millimeter (normally five laminae). Pillars are dominantly simple rod-like forms and rarely branching, triangular, wedge-shaped forms. Galleries are round, elongated, and slightly angular, ranging from 0.12 to 0.95 mm (number of measured galleries = 0.96, average 0.34 mm) wide. In the axial part of the dendroid form, laminae are more widely spaced than the normal phases and radially arranged with sharply folded zigzag Ecclimadictyon-like phases (Fig. 6.4).
Material
Twenty specimens: NIGP 177125, 177126 from the S1 interval, NIGP 177127–177130 from the S9 interval, NIGP 177131 from S11 interval, NIGP 177132 from the S15 interval, NIGP 177133-1, 2 and 177313 from the S16 interval, NIGP 159423, 159430, 159433, 159442, 159445, 159447, 159448, 169634-1–20, 177134, 177135 from the S18 interval of the Xiazhen Formation (Upper Ordovician, Katian) at Zhuzhai, Yushan County, Jiangxi Province, China.
Remarks
The present specimens of Clathrodictyon cf. Cl. mammillatum from the Xiazhen Formation show close similarity of morphological features with those from Sanqushan, Changshan, Zhejiang Province, and New South Wales (Webby, Reference Webby1969; Lin and Webby, Reference Lin and Webby1988; Jeon et al., Reference Jeon, Li, Na, Liang and Zhang2022). Laminae in the latter specimens are spaced at six to nine laminae per two millimeters (Webby, Reference Webby1969), being thicker than those of the Xiazhen Formation specimens; this is considered to reflect intraspecific variation.
Clathrodictyon cf. Cl. microundulatum Nestor, Reference Nestor1964
Figure 7
- cf. Reference Nestor1964
Clathrodictyon microundulatum Nestor, p. 41, pl. 13, figs. 1–6.
- Reference Webby1969
Clathrodictyon cf. microundulatum; Webby, p. 657, pl. 126, fig. 6, pl. 127, figs. 1–4.
- Reference Lin and Webby1988
Clathrodictyon cf. microundulatum; Lin and Webby, p. 242, fig. 6a, b.
- Reference Jeon, Liang, Park, Kershaw and Zhang2022a
Clathrodictyon cf. microundulatum; Jeon et al., p. 62, figs. 2e, f, 4e, f.

Figure 7. (1) Longitudinal section of Clathrodictyon cf. Cl. microundulatum from the S18 interval, NIGP 177194-4. (2) Enlargement of the rectangular area in (1). (3) Enlargement of the rectangular area in (1) showing longitudinal section of a vertically developed skeletal structural tube. (4) Astrorhizal structure of Clathrodictyon cf. Cl. microundulatum from the S18 interval, NIGP 177194-13. (5) Tangential section of Clathrodictyon cf. Cl. microundulatum with intergrown Bajgolia from the S18 interval, NIGP 177194-4.
Occurrence
The S3, S13, and S15–18 intervals of subsections ZU1 and ZU2 and the upper part of ZU3 of the Xiazhen Formation (Upper Ordovician, Katian) at Zhuzhai, Yushan County, Jiangxi Province, China (Figs. 2, 3).
Description
Skeletons range from laminar, low to high domical, to irregular growth forms. Large domical forms are commonly found in the S15 interval, up to 100 cm wide. Latilaminae and mamelons are not observed. Astrorhizae are rarely found (Fig. 7.4). Laminae, which are similar to vesicular in morphology, are well undulating; thickness ranges from 0.16 to 0.43 mm (number of measured laminae = 96, average 0.30 mm) high, spaced three to five laminae per millimeter. Pillars are persistently crumpled and funnel-like shaped. Galleries are round and elongated, ranging from 0.19 to 1.58 mm (number of measured galleries = 88, average 0.67 mm) wide. Astrorhizae are approximately 0.3–0.4 mm in diameter and 2 mm high, with ragged marginal contacts with laminae.
Material
Sixty-six specimens: NIGP 177136 and 177137 from the S3 interval, NIGP 177138 from the S9 interval, NIGP 159427, 159428, 159437, 159438, and 177139–177179 from the S15 interval, NIGP 159421 and 177180–177185 from the S16 interval, NIGP 177186 and 177187 from the S17 interval, NIGP 159420 and 177188–177194 from the S18 interval, and NIGP 177195 and 177196 from the upper part of the subsection ZU3 of the Xiazhen Formation (Upper Ordovician, Katian) at Zhuzhai, Yushan County, Jiangxi Province, China.
Remarks
The Xiazhen specimens of Clathrodictyon cf. Cl. microundulatum show close skeletal resemblance to those from the Sanqushan Formation in Changshan, Zhejiang Province, China, and from New South Wales, Australia (Webby, Reference Webby1969; Percival et al., Reference Percival, Zhen and Pickett2006; Jeon et al., Reference Jeon, Li, Na, Liang and Zhang2022a). Astrorhizae have not been found in the Sanqushan specimens, but both the New South Wales and Xiazhen Formation specimens possess well-developed astrorhizae with ragged margins between laminae.
A vertical tube is developed in the present specimen (Fig. 7.3); the tube has the same compact microstructure as the stromatoporoid (similar to Kershaw et al., Reference Kershaw, Munnecke and Jarochowska2018, fig. 18). A part of the tube is in open continuation with the stromatoporoid gallery and may be a part of the stromatoporoid skeletal structure (Fig. 7.3). However, it remains possible that the tube is a bioclaustration (an intergrown organism lacking its own shell). This vertical element is distinguishable from astrorhizae (Fig. 7.4, right-hand side) and intergrown tubes (Fig. 7.1, 7.4, 7.5). Astrorhizae have ragged marginal contact with laminae (Fig. 7.4). Downward or upward laminae of the clathrodictyid stromatoporoid occur near the contact with intergrown corals (Fig. 7.1, 7.4). By contrast, the stromatoporoid laminae adjacent to the vertical tube in Fig. 7.3 do not exhibit any skeletal distortions. Overall, the nature of this tube is not resolved and may require more samples to verify.
Clathrodictyon plicatum Webby and Banks, Reference Webby and Banks1976
Figure 8
- Reference Webby and Banks1976
Clathrodictyon plicatum Webby and Banks, p. 131, pl. 2, figs. 1–5.
- Reference Jeon, Li, Na, Liang and Zhang2022a
Clathrodictyon plicatum; Jeon et al., p. 3, figs. 2c, d, 3c.

Figure 8. Thin-section photographs of Clathrodictyon plicatum from the S10 interval. (1) Longitudinal section of Clathrodictyon plicatum showing skeletal variation from the axial to lateral part, NIGP 177200-3. (2, 3) Enlargements of the rectangular areas in (1). Note the variation from the normal phase in the lateral phase of (2) and the sharply folded, crumpled Ecclimadictyon-like axial part of (3). (4, 5) Longitudinal and tangential sections of Clathrodictyon plicatum, respectively, NIGP 177204. (6) Sharply folded, crumpled Ecclimadictyon-like structure in the axial part in Clathrodictyon plicatum, NIGP 177205. (7) Tangential section of Clathrodictyon plicatum showing well-developed mamelons, NIGP 177213-1.
Type specimen
Holotype UTGD 94626 and paratypes UTGD 94623–94625, 94628–94629, and 94631–94632 from the Upper Ordovician Den Formation of the uppermost Gordon Limestone Subgroup, Tasmania (Webby and Banks, Reference Webby and Banks1976, p. 131, pl. 2, figs. 1–5; see Burrett et al., Reference Burrett, Banks, Clota and Seymour1989 for the stratigraphic information); deposited in University of Tasmania, Hobart, Australia.
Occurrence
The S10–S12 and S14–S18 intervals of subsections ZU1 and ZU2 and the upper part of ZU3 of the Xiazhen Formation (Upper Ordovician, Katian) at Zhuzhai, Yushan County, Jiangxi Province, China (Figs. 2, 3).
Description
Skeletons vary from laminar, low to high domical, bulbous, dendroid to irregular or even digitate, up to 16 cm wide and 15 cm high. Astrorhizae and latilaminae are not found. Mamelons are common, spaced at 2.83–5.43 mm (number of measured distances between two mamelons = 13, average 4.28 mm) and up to 9.66 mm apart from each other, generally 6 mm.
Laminae are laterally continuous and regularly spaced, ranging from 0.08 to 0.25 mm (number of measured laminae = 86, average 0.15 mm), usually spaced from six to eight per millimeter (average seven). Galleries are round and elongate, and of variable width, ranging from 0.15 to 1.06 mm (number of measured galleries = 86, average 0.51 mm). In the axial part of columnar growth form, widely spaced laminae developed. These laminae are interlayered with crumpled laminae showing sharp, chevron-like folds, which are rather similar to the cassiculate network in actinodictyids such as Ecclimadictyon and Plexodictyon (see Fig. 8 for folded, chevron-like crumpled laminae in Clathrodictyon plicatum and Figs. 9, 10 for skeletal features of Ecclimadictyon species).

Figure 9. Thin-section photographs of Ecclimadictyon nestori from the S18 interval. (1) Longitudinal section, NIGP 177286. (2) Longitudinal section, NIGP 177287. (3) Tangential section, NIGP 177288.

Figure 10. (1, 2) Longitudinal sections of Ecclimadictyon undatum from the S15 interval: (1) NIGP 177302-1; (2) 177305. (3, 4) Tangential sections from the S15 interval: (3) NIGP 177302-7; (4) 177302-8. (5) Intergrown “Eofletcheria-like tubules” in Ecclimadictyon undatum, NIGP 177299. (6) Enlargement of the rectangular area in (5). Note that the endobionts are vertically grown, while free-living grown tubules have varying orientations in the matrix under the Ecclimadictyon skeleton.
Materials
Fifty-six specimens: NIGP 177197–177224 from the S10 interval, NIGP 177225–177228 from the S11 interval, NIGP 177229 from the S12 interval, NIGP 159429 and 177230–177232 from the S14 interval, NIGP 177233–177239 from the S15 interval, NIGP 177240 from the S16 interval, NIGP 168768 and 177241–177246 from the S17 interval, and NIGP 177247–177250 from the S18 interval of the Xiazhen Formation (Upper Ordovician, Katian) at Zhuzhai, Yushan County, Jiangxi Province, China.
Remarks
The specimens of Clathrodictyon plicatum Webby and Banks, Reference Webby and Banks1976 from the Upper Ordovician Den Formation of Tasmania (Webby and Banks, Reference Webby and Banks1976) and from South China (Jeon et al., Reference Jeon, Li, Na, Liang and Zhang2022a; the present study) exhibit an abnormal skeletal phase represented by folded, chevron-like crumpled laminae (“a Plexodictyon-type structure” in Webby and Banks Reference Webby and Banks1976, p. 131; “Plexodictyon-like abnormal phase” in Jeon et al., Reference Jeon, Li, Na, Liang and Zhang2022a, fig. 3). This skeletal variation has been interpreted as an advanced growth strategy affected by environmental conditions (Jeon et al., Reference Jeon, Li, Na, Liang and Zhang2022), and this species correspondingly shows the longest stratigraphic range in the Xiazhen Formation, representing a wide range of depositional environments.
Family Actinodictyidae Khalfina and Yavorsky, Reference Khalfina and Yavorsky1973
Ecclimadictyon Nestor, Reference Nestor1964
Type species
Clathrodictyon fastigiatum Nicholson, Reference Nicholson1887.
Ecclimadictyon nestori Webby, Reference Webby1969
Figure 9
- Reference Webby1969
Ecclimadictyon nestori Webby, p. 660, pl. 128, fig.1, pl. 129, figs. 1–6.
- Reference Lin and Webby1988
Ecclimadictyon sp. A Lin and Webby, p. 239, fig. 3a–d.
Type specimen
Holotype, SUP 28203, from the Upper Ordovician Vandon Limestone between Belubula River and Large Flat (Webby, Reference Webby1969). One paratype, SUP 28256, from the upper part of the Upper Ordovician Belubula Limestone, and the others (SUP 26199–26202, 26204, 26209) from the Upper Ordovician Vandon Limestone of New South Wales (see Webby, Reference Webby1969, p. 660) in the Cliefden Caves Limestone Subgroup, New South Wales (Webby, Reference Webby1969 p. 660, pl. 128, fig.1, pl. 129, figs. 1–6; see Percival et al., Reference Percival, Popov, Zhan, Ghobadi Pour, Gutiérrez-Marco, Rábano and García-Bellido2011 for the regional stratigraphic information); deposited in Australian Museum, Sydney, Australia.
Occurrence
The S2–4, 10, 16, 18 intervals of subsections ZU1 and ZU2 and the upper part of ZU3 of the Xiazhen Formation (Upper Ordovician, Katian) at Zhuzhai, Yushan County, Jiangxi Province, China (Figs. 2, 3).
Description
Skeletons are mostly laminar, and rarely low domical and irregular in growth forms, up to 10 cm wide and 4 cm high. Astrorhizae, mamelons, and latilaminae are not found.
Skeletons consist of sharply folded chevron-like crumpled laminae, which are laterally continuous, forming regular cassiculate networks. The thickness of laminae ranges from 0.08 to 0.20 mm (number of measured laminae = 92, average 0.12 mm), spaced six to eight laminae per millimeter (average seven laminae). Pillars are poorly developed and confined to interlaminar spaces. Galleries are mostly rhomboid with sharp marginal areas. Sharply crumpled laminae exhibit isolated dots or appear to be linked with others.
Materials
Fifty specimens: NIGP 177251–177253 from the S2–S3 interval, NIGP 177254–177259 from the S4 interval, NIGP 177260–177263 from the S10 interval, NIGP177264–177267 from the S15 interval, NIGP 177268–177271 from the S16 interval, NIGP 177272, 177273 from the S17 interval, NIGP 159431, 159436, 159443, 159444, 159449, 168769, 177274–177294 from the S18 interval of the Xiazhen Formation (Upper Ordovician, Katian) at Zhuzhai, Yushan County, Jiangxi Province, China.
Remarks
The single specimen of Ecclimadictyon from the Sanqushan Formation, which was described by Lin and Webby (Reference Lin and Webby1988), was designated as an independent species of Ecclimadictyon in open nomenclature (Ecclimadictyon sp. A, Lin and Webby, Reference Lin and Webby1988, p. 239) on the basis of its more conspicuous latilaminae and larger astrorhizal canals than those of Ecclimadictyon nestori (Lin and Webby, Reference Lin and Webby1988). However, those differences cannot serve as critical features to separate two independent stromatoporoid species and should be counted as skeletal variations of a single species. Both show identical sharply folded, zigzag-shaped crumpled laminae, forming angular galleries, as mostly spacing 7–8 laminae per millimeter. Thus, in this study, Ecclimadictyon sp. A in Lin and Webby, Reference Lin and Webby1988 is synonymized with Ecclimadictyon nestori Webby, Reference Webby1969, judging from their close skeletal morphological resemblance.
Ecclimadictyon undatum Webby and Banks, Reference Webby and Banks1976
Figure 10
- Reference Webby and Banks1976
Ecclimadictyon undatum Webby and Banks, p. 132, pl. 2, figs. 1–3.
Type specimen
Holotype (UTGD 94636) and three paratypes (UTGD 90917, 94637, 94638) from the Upper Ordovician Den Formation of the uppermost Gordon Limestone Subgroup, Tasmania (Webby and Banks, Reference Webby and Banks1976, p. 132, pl. 2, figs. 1–3; see Burrett et al., Reference Burrett, Banks, Clota and Seymour1989 for the regional stratigraphic information).
Occurrence
The S11, S12, and S15 intervals of the Xiazhen Formation (Upper Ordovician, Katian) at Zhuzhai, Yushan County, Jiangxi Province, China (Figs. 2, 3).
Description
Skeleton is laminar in growth form, up to 14 cm wide and 3 cm high. Mamelons, latilaminae, and astrorhizae are not found. An unidentified endobiont (referred to as “Eofletcheria-like tubules” by Lee et al., Reference Lee, Elias, Choh and Lee2016b) occurs only in the current species (Fig. 10.5, 10.6).
The internal structure is variable, ranging from coarsely to finely structured laminae. In the coarser skeletal phase, laminae are moderately crumpled, forming elongated and round galleries (Fig. 10.1). In more finely structured portions of the skeleton, laminae are rather sharply folded, and galleries are more angular rhomboid (Fig. 10.2). The thickness of laminae ranges from 0.13 to 0.39 mm (number of measured laminae = 86, average 0.21), spaced three to five laminae per millimeter (average four laminae). Pillars are mostly indistinct.
Materials
Thirteen specimens: NIGP 177295, 177296 from the S11 interval, NIGP 177297 from the S12 interval, NIGP 159425, 159426, 177298–177307 from the S15 interval of the Xiazhen Formation (Upper Ordovician, Katian) at Zhuzhai, Yushan County, Jiangxi Province, China.
Remarks
The specimens of Ecclimadictyon undatum from Tasmania show that laminae range from 12 to 14 per two millimeters (Webby and Banks, Reference Webby and Banks1976), slightly finer than those in the Xiazhen Formation specimens. These differences in laminae dimensions are considered to be intraspecific variation. Astrorhizae are not found in either collection of specimens. E. undatum Webby and Banks, Reference Webby and Banks1976 exhibits widely spaced, less sharply folded laminae and coarser cassiculate structures than E. nestori Webby, Reference Webby1969.
Camptodictyon Nestor, Copper, and Stock, Reference Nestor, Copper and Stock2010
Type species
Camptodictyon penefastigiatum Nestor, Copper, and Stock, Reference Nestor, Copper and Stock2010.
Camptodictyon amzassensis (Khalfina, Reference Khalfina1960)
Figure 11
- Reference Khalfina1960
Clathrodictyon(?) kirgisicum amzassensis Khalfina, p. 370, pl. O–1, figs. 1–3.
- Reference Webby1969
Ecclimadictyon amzassensis (Khalfina); Webby, p. 659, pl. 127, figs. 5–7, pl. 128, figs. 1–5.
- Reference Dong and Wang1984
Ecclimadictyon crassilamellatum Dong and Wang, p. 260, pl. 14, fig. 2a, b.
- Reference Dong and Wang1984
Ecclimadictyon xinjiangense Dong and Wang, p. 261, pl. 14, fig. 3a, b.

Figure 11. (1–3) Longitudinal sections of Camptodictyon amzassensis from the S15 interval: (1, 2) NIGP177308-1; (3) NIGP 177308-2. (4) Tangential section of Camptodictyon amzassensis from the S15 interval, NIGP 177308-1.
Type specimen
Holotype no. 537 with three thin sections from the Amzass Formation of Gornaya Shoriya, Russia (Khalfina, Reference Khalfina1960, p. 370, pl. O–1, figs. 1–3); deposited in Trofimuk Institute of Petroleum Geology and Geophysics, Russian Academy of Sciences, Novosibirsk, Russia.
Occurrence
The S15, S17 intervals of the Xiazhen Formation (Upper Ordovician, Katian) at Zhuzhai, Yushan County, Jiangxi Province, China (Figs. 2, 3).
Description
Skeleton is laminar, about 20 mm high and 60 mm wide. Latilaminae and mamelon columns are not found.
Laminae range from zigzag-shaped (also commonly referred to as “chevron-like”; see Nestor et al., Reference Nestor, Copper and Stock2010, p. 83 for the diagnosis of genus Camptodictyon), smoothly downward-folded to undulating structures, spaced five to seven per millimeter (average 6). Thickness of laminae is 0.06–0.22 mm (number of measured laminae = 55, average 0.13 mm). Pillars are short and commonly indistinct, but may also reach the other laminae, 0.03–0.17 mm high (number of measured laminae = 70, average 0.08 mm). Galleries are round and elliptical in longitudinal section, varying from 0.32 to 1.61 mm wide (number of measured galleries = 52, average 0.75 mm). Astrorhizae are sporadically developed, represented by short and curved astrorhizal galleries (Fig. 11.2).
Materials
Three specimens: NIGP 177308 from the S15 interval and NIGP 177309, 177310 from the S17 interval of the Xiazhen Formation (Upper Ordovician, Katian) at Zhuzhai, Yushan County, Jiangxi Province, China.
Remarks
The Xiazhen Formation specimens closely resemble specimens from Altai–Sayan (Khalfina, Reference Khalfina1960) and New South Wales (Webby, Reference Webby1969), with three to six laminae per millimeter. Ecclimadictyon xinjiangense (NIGP 70420) (Dong and Wang, Reference Dong and Wang1984, pl. 14, fig. 2a, b) and E. crassilamellatum (NIGP 70421) (Dong and Wang, Reference Dong and Wang1984, pl. 14, fig. 3a, b) from Xinjiang, China, develop cassiculate networks from downward folded to undulating laminae. These skeletal characteristics are much closer to the generic concept of Camptodictyon (Nestor et al., Reference Nestor, Copper and Stock2010, p. 83; Nestor, Reference Nestor and Selden2015, p. 758) than to Ecclimadictyon (Nestor, Reference Nestor and Selden2015, p. 758). The skeletal differences in these two species (i.e., slightly thicker laminae, space of astrorhizal canals) are not considered to possess sufficient taxonomic value to justify them as independent species in Dong and Wang (Reference Dong and Wang1984) (Lin and Webby, Reference Lin and Webby1988). The specimens from Xinjiang, China, show similar morphological features to those in the current material and are therefore regarded as junior synonyms of Camptodictyon amzassensis (Khalfina, Reference Khalfina1960).
Labyrinthodictyon Nestor, Copper, and Stock, Reference Nestor, Copper and Stock2010
Type species
Labyrinthodictyon angulosum Nestor, Copper, and Stock, Reference Nestor, Copper and Stock2010.
Labyrinthodictyon cascum (Webby and Morris, Reference Webby and Morris1976)
Figure 12
- Reference Webby and Morris1976
Plexodictyon? cascum Webby and Morris, p. 132, fig. 5a–c, e.
- Reference Jeon, Li, Na, Liang and Zhang2022a
Labyrinthodictyon cascum (Webby and Morris, Reference Webby and Morris1976); Jeon et al., p. 7, figs. 2g, h, 3d, e.

Figure 12. (1–4) Longitudinal sections of Labyrinthodictyon cascum from the S15 interval: (1–3) NIGP 177311-1; (4) 177312-1. (5) Tangential section of Labyrinthodictyon cascum from the S15 interval, NIGP 177312-2.
Type specimen
Holotype (SUP 78258) and three paratypes (SUP 77277–77279) from the Upper Ordovician Ballingoole Limestone in the upper part of the Bowan Park Limestone Subgroup, New South Wales (Webby and Morris, Reference Webby and Morris1976, p. 132, fig. 5a–c, e; see Percival et al., Reference Percival, Popov, Zhan, Ghobadi Pour, Gutiérrez-Marco, Rábano and García-Bellido2011 for the regional stratigraphic information); deposited in the Australian Museum, Sydney, Australia.
Occurrence
The S15 interval of the Xiazhen Formation (Upper Ordovician, Katian) at Zhuzhai, Yushan County, Jiangxi Province, China (Figs. 2, 3).
Description
Skeletons are fragmented but indicate a laminar growth form. Mamelons and astrorhizae are not found. Skeletons consist of planar paralaminae, which are laterally continuous. Thickness of paralaminae is 0.21–0.78 mm (number of measured paralaminae = 67, average 0.45 mm), regularly spaced two per millimeter (exceptionally, three paralaminae are also seen). The interparalaminae exhibit cassiculate laminae, varying from irregularly crumpled to triangular-wedge shapes, at a spacing of two to three crumpled laminae within one paralamina. Galleries are angular and variable in both shape and size.
Materials
Three specimens: NIGP 168770, 177311, 177312 from the S15 interval of the Xiazhen Formation (Upper Ordovician, Katian) at Zhuzhai, Yushan County, Jiangxi Province, China.
Remarks
The Xiazhen specimens have regularly spaced planar paralaminae, closely resembling the specimens from New South Wales (Webby and Morris, Reference Webby and Morris1976) and those from the coeval Sanqushan Formation of South China (Jeon et al., Reference Jeon, Li, Na, Liang and Zhang2022a). All the specimens from China and Australia show about two paralaminae per millimeter (Webby and Morris, Reference Webby and Morris1976; Jeon et al., Reference Jeon, Li, Na, Liang and Zhang2022a). No abnormal skeletal phases have been observed in the Xiazhen Formation specimens, while abnormal Ecclimadictyon-like phases occur in the Sanqushan Formation specimens (see Jeon et al., Reference Jeon, Li, Na, Liang and Zhang2022, fig. 3d, e).
Intergrowth association between clathrodictyid stromatoporoids and other sessile organisms
In the Xiazhen Formation, intergrowth associations between clathrodictyid stromatoporoids and other sessile organisms are commonly found (Figs. 13–17, Table 1). The intergrowth associations occur in both reef and nonreef environments but are much more common in reefs due to the high diversity and density of reef-building organisms. Lee et al. (Reference Lee, Elias, Choh and Lee2016b) found that only Clathrodictyon and Ecclimadictyon acted as host stromatoporoids. No intergrown organisms were found within skeletons of Camptodictyon and Labyrinthodictyon. The stratigraphic distribution of intergrowth associations between clathrodictyid stromatoporoids and other sessile organisms in the Xiazhen Formation is listed in Table 1. The intergrowth associations include seven host clathrodictyid species and various intergrown benthic organisms. Among those intergrown benthic organisms, tabulate corals include Agetolites, Bajgolia, Catenipora, and Heliolites (Figs. 13–17), and the auloporid Bajgolia is the most common intergrown coral (Figs. 14–16; see figures in Lee et al., Reference Lee, Elias, Choh and Lee2016b). Species of Agetolites are found to envelop dendroid forms of Clathrodictyon idense particularly in the S3 interval (Fig. 13.5, 13.6). Solitary-form rugose corals Streptelasma and Tryplasma are also found as endobionts. Other fossil groups, including Rhabdotetradium (see Lee et al., Reference Lee, Elias, Choh and Lee2016b, fig. 2b), unidentified Eofletcheria-like tubules (Figs. 10.6, 14.3; see Lee et al., Reference Lee, Elias, Choh and Lee2016b, fig. 2f), labechiid stromatoporoids (Fig. 14.6; see figures of Jeon et al., Reference Jeon, Liang, Lee and Kershaw2020b), and bryozoans (Fig. 14.2) also intergrew within clathrodictyid stromatoporoids.
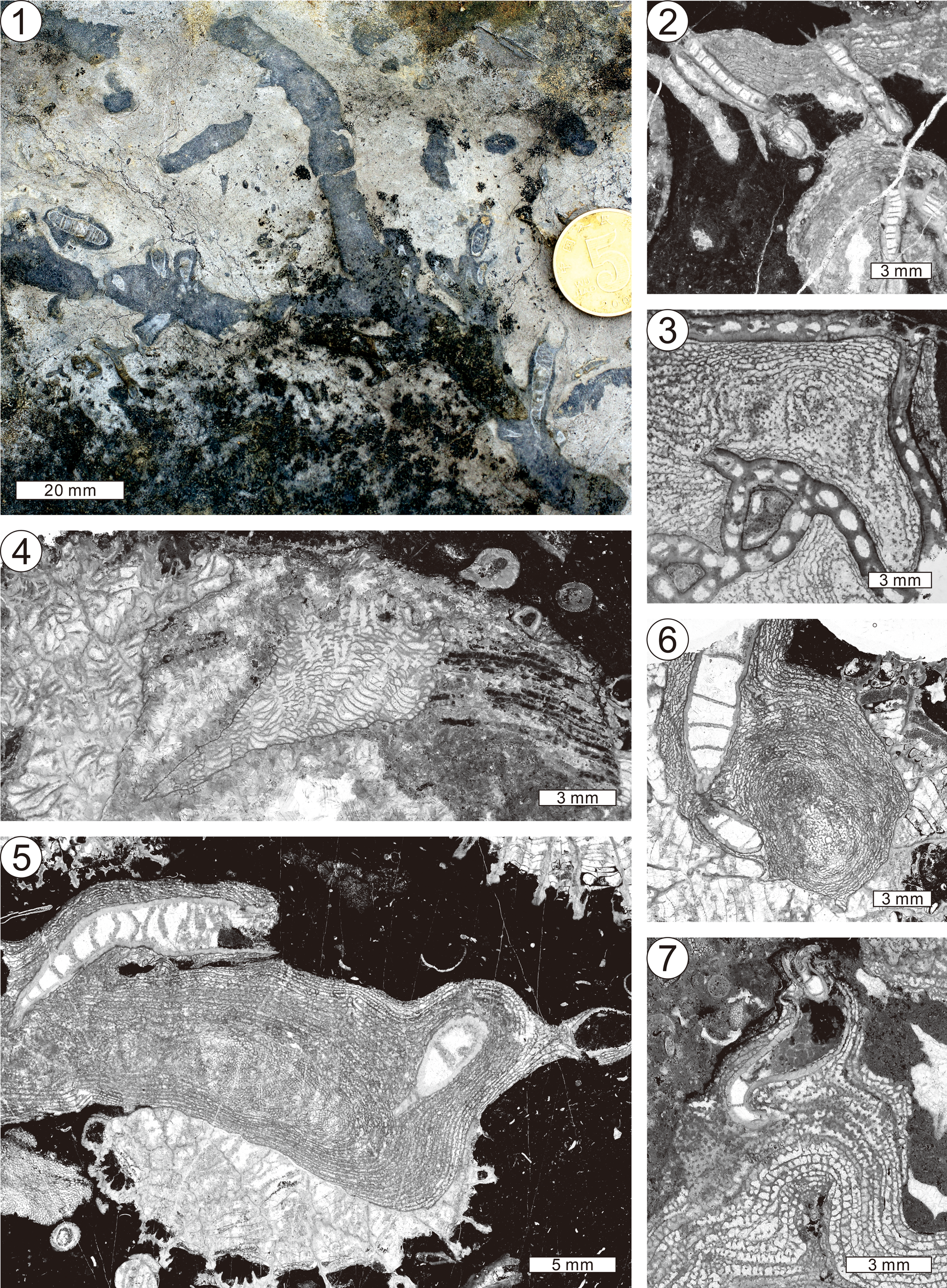
Figure 13. Diverse paleoecological interactions among stromatoporoids and other organisms. (1) Field photograph of dendroid growth form of Clathrodictyon idense Webby and Banks, Reference Webby and Banks1976 and intergrown solitary rugose coral Tryplasma. (2) Longitudinal section of Clathrodictyon cf. Cl. microundulatum and intergrown tabulate coral Catenipora, NIGP 177149-1. (3) Tangential section of Clathrodictyon cf. Cl. microundulatum and intergrown tabulate coral Catenipora, NIGP 177190. (4) Encrustation and intergrowth association among Clathrodictyon cf. Cl. mammillatum and tabulate corals Agetolites and Heliolites, NIGP 177313. Note irregular physical contacts between tabulates and stromatoporoid. Upper right is a single tube with a flat base, encrusted and partly embedded in the stromatoporoid surface. (5) Clathrodictyon idense and intergrown Tryplasma and encrusted Agetolites, NIGP 177112-10. (6) Multi-intergrowth association among Agetolites, Clathrodictyon idense, and Tryplasma, NIGP 177101. (7) Intergrowth association between Clathrodictyon megalamellatum Jeon n. sp. and Tryplasma.
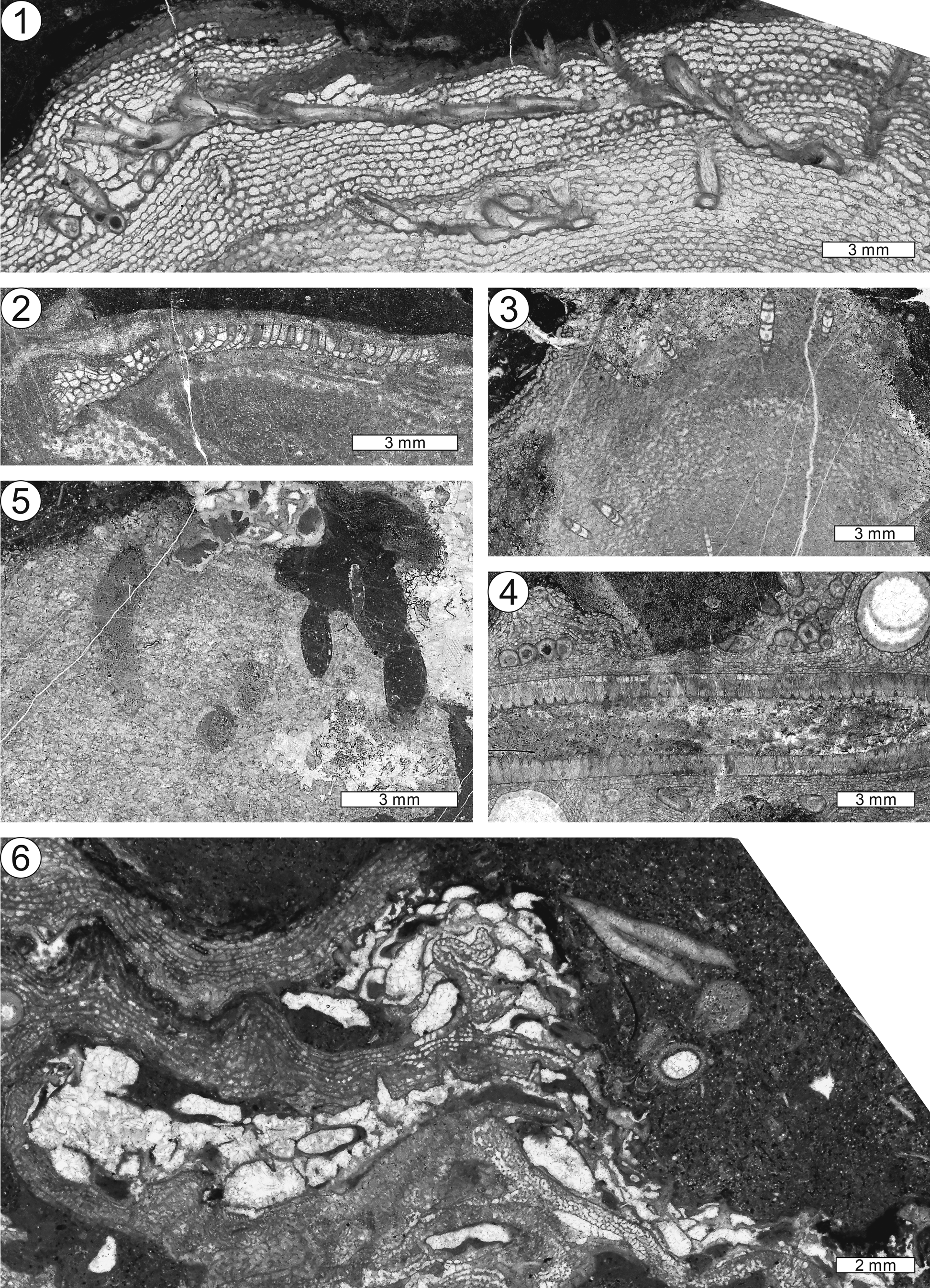
Figure 14. Diverse paleoecological interactions among stromatoporoids and other organisms. (1) Longitudinal section of Clathrodictyon cf. Cl. microundulatum showing Bajgolia were clustered along the growth surface of the stromatoporoid and finally encrusted by Clathrodictyon cf. Cl. microundulatum, NIGP 177194-6. (2) Poorly preserved clathrodictyid stromatoporoid and the interlayering bryozoan Rhombotrypa, NIGP 177314. It is difficult to distinguish whether the bryozoan grew on a partially dead or living growth surface of the stromatoporoid, but the growth of the bryozoan was terminated by the overgrown host stromatoporoid. Note the difference in preservation between bryozoan and stromatoporoid, likely indicating different original mineralogy. (3) Unidentified tubular “Eofletcheria-like organism” in the skeleton of Ecclimadictyon undatum, NIGP 177303. Note that this endobiont is found only in Ecclimadictyon undatum. (4) Crinoid stem and encrusting Clathrodictyon plicatum with intergrown Bajgolia and Tryplasma, NIGP 177250-4. (5) Oblique section of micrite-filled, enigmatic borings in the skeleton of Ecclimadictyon nestori, NIGP 177263. (6) Paleoecological interaction between Clathrodictyon cf. Cl. mammillatum and Labechia sp., interpreted as spatial competition judging from their skeletal distortion (Jeon et al., Reference Jeon, Liang, Lee and Kershaw2020b), NIGP 169634-14.

Figure 15. (1–6) Thin-section examples of downward and upward deflections of the clathrodictyid stromatoporoid laminae, caused by the intergrown corals. Black arrows indicate down-bending laminae, and white arrows indicate up-bending laminae: (1) NIGP 177179-14; (2) NIGP 177194-4; (3) NIGP 177194-11; (4) NIGP 159437; (5) NIGP 176515; (6) NIGP 177289. Down-bending laminae of Clathrodictyon cf. Cl. microundulatum occur near Bajgolia in (1–4), while the up-bending laminae are in the vicinity of intergrown Tryplasma (1, 3); (5) down-bending laminae of Clathrodictyon plicatum occur near the sharp irregularly shaped outer wall of Bajgolia (see also figures and corresponding text in Jeon et al., Reference Jeon, Vinn, Liang, Zapalski, Toom and Kershawin press); (6) up-bending laminae of Ecclimadictyon nestori occur around intergrown Bajgolia. (7–17) Schematic drawings to illustrate the process of forming bending stromatoporoid laminae in the vicinity of endobiont: (7, 8) initial stage of settlement of endobionts on the growth surface of clathrodictyid species; (9–11) with the faster growth rate of clathrodictyid species than the endobionts, down-bending laminae developed near the endobionts; the endobionts were commonly killed by the overgrowth of clathrodictyid; (12–14) examples of up-bending laminae caused by the faster growth rate of endobionts than host clathrodictyid species, commonly terminated by sediment interruption; (15–17) examples of both up- and down-bending laminae around endobionts: (15) up-bending laminae formed by encrustation of pre-existing encrusting endobiont; (16, 17) faster growth of host clathrodictyid species reached up to same growth level as the endobiont and caused down-bending laminae.

Figure 16. (1, 2) Longitudinal sections of astrorhizae (black arrows) of Clathrodictyon cf. Cl. microundulatum with encrusted intergrown Bajgolia. Note that none of the distorted skeletal structures occurs near the contacts with endobionts: (1) NIGP 177196-4 from the S18 interval; (2) NIGP 177196-12 from the S18 interval. (3) Field photograph of feather-like growth form of clathrodictyid (probably Ecclimadictyon) and intergrown Bajgolia cluster from the S17 interval. (4–7) Thin-section photographs of feather-like growth form of clathrodictyids and intergrown Bajgolia clusters: (4) dendroid Ecclimadictyon nestori with intergrown Bajgolia, NIGP 159443 from the S18 interval; (5) dendroid Clathrodictyon cf. Cl. mammillatum with intergrown Bajgolia, NIGP 175173 from the S18 interval; (6) dendroid Clathrodictyon plicatum with intergrown Bajgolia NIGP 159448 from the S18 interval; (7) branching dendroid Clathrodictyon plicatum with intergrown Bajgolia, NIGP 159447 from the S18 interval. Specimens NIGP 159443, 159447, and 159448 in (4, 6, 7) were also illustrated by Lee et al. (Reference Lee, Elias, Choh and Lee2016b). (8) Abnormally widely spaced laminae of Clathrodictyon cf. Cl. microundulatum, NIGP 159446 from the S18 interval.

Figure 17. (1–5) Encrustation of diverse sessile organisms on the growth surface of clathrodictyid species: (1) encrusted and vertically grown Tryplasma on the growth surface of Clathrodictyon plicatum, NIGP 177245 from the S17 interval; (2) encrusted Heliolites on the growth surface of Clathrodictyon cf. Cl. microundulatum, showing possible spatial competition judging from their irregular physical contact, NIGP 179198 from the S15 interval; (3) syn-vivo interaction between microbe and Clathrodictyon idense, showing their twisted growth direction, NIGP 177107-1 from the S3 interval; (4) structure interpreted as a spiculate sponge encrusted on Clathrodictyon plicatum from the S17 interval, NIGP 177243; (5) multiple encrustations by tabulate coral, calcimicrobes, and bryozoans on the growth surface of Clathrodictyon cf. Cl. mammillatum, NIGP 177135 from the S18 interval. (6) Schematic reconstruction to show a variety of paleoecological interactions between clathrodictyid stromatoporoids and intergrown sessile organisms, including labechiid stromatoporoid, rugose, and tabulate corals.
Table 1. Stratigraphic distribution of host clathrodictyid stromatoporoids and endobionts in the Xiazhen Formation. The gray-colored intervals are occupied by labechiids (Stylostroma and Thamnobeatricea, respectively) without any clathrodictyid species. 1, Clathrodictyon cf. Cl. mammillatum; 2, Clathrodictyon cf. Cl. microundulatum; 3, Clathrodictyon idense; 4, Clathrodictyon megalamellatum Jeon n. sp.; 5, Clathrodictyon plicatum; 6, Ecclimadictyon nestori; 7, Ecclimadictyon undatum. Question mark indicates unidentified poorly preserved clathrodictyid stromatoporoid.

Judging from the presence of both free-living and intergrown growth modes (Lee et al., Reference Lee, Elias, Choh and Lee2016b; Jeon et al., Reference Jeon, Liang, Lee and Kershaw2020b), we interpret the intergrowth associations between clathrodictyid stromatoporoids and other sessile organisms to have occurred by chance rather than an obligate association. The laminae of clathrodictyids are concavely or convexly curved near the contact with the intergrown corals, possibly showing the different growth rates of host clathrodictyids and intergrown organisms (Fig. 15). It can be inferred that most of the endobionts had little to no impact on most of the host clathrodictyids, and some endobionts even encrusted or grew near the astrorhizae of the host stromatoporoid (Fig. 16.1, 16.2). However, in some cases, the host clathrodictyids show evidence of having been affected by the intergrown organisms, judging from a narrow range of columnar to dendritic growth forms (Fig. 16.3–16.7), abnormally spaced laminae (Fig. 16.8), and skeletal distortions (Fig. 14.6; see Jeon et al., Reference Jeon, Liang, Lee and Kershaw2020b, figs. 2.4, 3, 4). Host stromatoporoids that contain intergrown Bajgolia are commonly restricted to the branching dendroid forms (Fig. 16.3–16.7; Lee et al., Reference Lee, Elias, Choh and Lee2016b), which indicates that the growth direction and orientation of the intergrown organism could affect the growth of the host stromatoporoid in some cases. Skeletal deformation with zigzag, crumpled, distorted laminae of the host clathrodictyid is also observed near the physical contacts with intergrown labechiids, indicating spatial competition between these two stromatoporoids (compare Figs. 13–17 with Jeon et al., Reference Jeon, Liang, Lee and Kershaw2020b, figs. 2.4, 3, 4).
Paleobiogeographic pattern of Ordovician clathrodictyid stromatoporoids
Ordovician clathrodictyid stromatoporoids rapidly reached a global distribution concurrently with their earliest appearance in the middle Katian of the Late Ordovician (Stock et al., Reference Stock, Nestor, Webby and Selden2015). A total of 27 clathrodictyid species were reported in the Late Ordovician interval (middle Katian to Hirnantian). The wide range of skeletal variation in clathrodictyids may confuse discrimination of lowest-level taxa and lead to over splitting, but resolving this issue requires examination of published materials, which is outside the scope of this study. Nevertheless, the clathrodictyid species examined here belong to four genera (Clathrodictyon, Ecclimadictyon, Camptodictyon, and Labyrinthodictyon) and have been recorded from nine terranes (Fig. 18). Many species were endemic and occurred within a single terrane. Peri-Gondwanan terranes, including North China, Australia, South China, and other terranes (e.g., Tarim) share more clathrodictyid species in common than the other terranes. In general, the network analysis diagram shows three independent clathrodictyid faunal provinces (peri-Gondwana–Tarim–Altai, Laurentia, and Baltica) (Fig. 18).

Figure 18. (1) Network analysis diagram of clathrodictyid stromatoporoid during the Late Ordovician. These listed species are clathrodictyids shared between two or more paleocontinents. (2) Three major faunal provinces of clathrodictyid stromatoporoid distribution during the Late Ordovician. Red question marks on Mongolia and Kazakh terranes indicate uncertainty over whether these regions should be included in the peri-Gondwana–Tarim–Altai faunal province due to insufficient investigation of clathrodictyid stromatoporoid faunas. Paleogeographic reconstruction modified from Cocks and Torsvik (Reference Cocks and Torsvik2020). Note that this faunal province has a high similarity with the “Cathay-Tasman Province” proposed by Cocks and Torsvik (Reference Cocks and Torsvik2020).
Peri-Gondwana–Tarim–Altai Province
Peri-Gondwanan terranes, particularly Australia and South China, show diverse clathrodictyids and share many common species of Clathrodictyon, Ecclimadictyon, Camptodictyon, and Labyrinthodictyon. It is noteworthy that New South Wales and Tasmania do not share any common clathrodictyid species although they were paleogeographically close to each other (Webby, Reference Webby1969; Webby and Banks, Reference Webby and Banks1976; Webby and Morris, Reference Webby and Morris1976; Webby et al., Reference Webby2000).
Camptodictyon amzassensis (Khalfina, Reference Khalfina1960) is an important species for defining this faunal province in that it shows the widest paleobiogeographic distribution (Fig. 18), occurring in New South Wales (Webby, Reference Webby1969), South China (present study), Tarim (Dong and Wang, Reference Dong and Wang1984), and Altai–Sayan Fold Belt (Khalfina, Reference Khalfina1960). This species has not yet been found in North China, and only a single clathrodictyid species (Clathrodictyon cf. Cl. microundulatum Nestor, Reference Nestor1964) occurs in both North China and the other peri-Gondwanan terranes (i.e., South China and New South Wales). In addition to this species, two other species, Clathrodictyon? sp., possessing distinctive skeletal features, and Ecclimadictyon sp. B (Lin and Webby, Reference Lin and Webby1988), have been reported only from North China.
No detailed taxonomic work on clathrodictyids from the Ordovician of Qaidam has been undertaken, unfortunately, and thus our analysis is based on the few records in the literature. Ecclimadictyon cf. E. koigense Nestor, Reference Nestor1964 is the only clathrodictyid species known from Qaidam (Lin and Webby, Reference Lin and Webby1988). Occurrence of the tabulate coral Agetolites species and labechiid stromatoporoids in Qaidam indicates a close paleobiogeographic relationship with South China (Li and Lin, Reference Li and Lin1982), presumably indicating the two blocks belong to the same clathrodictyid paleobiogeographic unit. Accordingly, we include Qaidam in the peri-Gondwana–Tarim–Altai Province in this study.
Laurentia Province
Clathrodictyon cf. Cl. kudnavzevi Riabinin, Reference Riabinin1951, Clathrodictyon sp. 3 (Bolton, Reference Bolton1988), Labyrinthodictyon angulosum Nestor, Copper, and Stock, Reference Nestor, Copper and Stock2010, Ecclimadictyon anticostiense Nestor, Copper, and Stock, Reference Nestor, Copper and Stock2010, and Ecclimadictyon sp. (Bolton, Reference Bolton1988) are known to occur in this province. Of these, Ecclimadictyon sp. and Clathrodictyon sp. 3 in Bolton (Reference Bolton1988) are from the Portage Chute and Red River formations of Manitoba, respectively, while the other three species are from the Ellis Bay Formation of Anticosti Island (Nestor et al., Reference Nestor, Copper and Stock2010).
Baltic Province
This province contains Clathrodictyon microundulatum Nestor, Reference Nestor1964, Clathrodictyon vormsiense Riabinin, Reference Riabinin1951, Clathrodictyon gregale Nestor, Reference Nestor1964, Clathrodictyon zonatum Nestor, Reference Nestor1964, Ecclimadictyon geniculatum Bogoyavlenskaya, Reference Bogoyavlenskaya1973, Ecclimadictyon porkuni (Riabinin, Reference Riabinin1951), and Ecclimadictyon koigiense Nestor, Reference Nestor1964. Among them, Clathrodictyon microundulatum, Clathrodictyon vormsiense, and Ecclimadictyon geniculatum are from the Katian Stage (including Vormsi and Pirgu regional stages) while the other four species are from the Hirnantian Stage (Porkuni Regional Stage) (Nestor, Reference Nestor1964).
Discussion
Occurrence of clathrodictyid stromatoporoids and their paleobiogeographic implication
The paleobiogeographic affinity of Ordovician stromatoporoids in South China was postulated to have been close to the other peri-Gondwana terranes (e.g., Australia), judging from the co-occurrence of some Clathrodictyon species and characteristic agetolitid corals from the Sanqushan Formation (Webby, Reference Webby1980; Lin and Webby, Reference Lin and Webby1988, Reference Lin and Webby1989). A recent study of clathrodictyids from the Sanqushan Formation (Jeon et al., Reference Jeon, Li, Na, Liang and Zhang2022a) and the present analysis correspondingly show that South China has close faunal affinity to New South Wales and Tasmania. It is noteworthy that New South Wales and Tasmania do not share any common clathrodictyid species despite their close paleogeographic distance (Webby, Reference Webby1969; Webby and Banks, Reference Webby and Banks1976; Webby and Morris, Reference Webby and Morris1976; Webby et al., Reference Webby2000). Percival et al. (Reference Percival, Popov, Zhan, Ghobadi Pour, Gutiérrez-Marco, Rábano and García-Bellido2011) noted that the brachiopods of New South Wales and Tasmania share surprisingly few faunal taxa, similarly to the clathrodictyid stromatoporoids. This pattern was interpreted to be due to a deep ocean basin swept by strong currents, which separated the benthic organisms of the two regions (Webby et al., Reference Webby2000). If true, this strong oceanic current might have also affected the migration of those Australian clathrodictyid species to South China, judging from the high faunal similarity of clathrodictyids between South China and those two Australian regions.
The analysis presented here indicates that North China had little faunal similarity with the other peri-Gondwanan terranes (i.e., South China, Australia) in terms of clathrodictyids, although this contrasts the paleobiogeographic pattern of labechiid stromatoporoids, which shows North and South China have faunal similarities (Jeon et al., Reference Jeon, Liang, Park, Kershaw and Zhang2022b); the implication is that these different taxa groups of stromatoporoids were under different controls with respect to their dispersal. Only one species (Clathrodictyon cf. Cl. microundulatum) occurs in North China, South China, and New South Wales of peri-Gondwanan terranes. Note that none of the Camptodictyon species have been found in North China, despite the wide distribution of Camptodictyon amzassensis during the Late Ordovician (Fig. 18), ranging from the Altai–Sayan Fold Belt (Khalfina, Reference Khalfina1960), Tarim (Dong and Wang, Reference Dong and Wang1984), and South China (present study) to New South Wales (Webby, Reference Webby1969). However, Late Ordovician stromatoporoids from North China and other adjacent terranes (i.e., Qaidam, Mongolia, and Central Asia) have not been well studied. Further studies are required for a comprehensive evaluation of stromatoporoids and paleobiogeographic patterns of these terranes.
The peri-Gondwana–Tarim–Altai Province of clathrodictyid stromatoporoids corresponds well with the recently proposed brachiopod benthic faunal province, named the “Cathay–Tasman Province” by Cocks and Torsvik (Reference Cocks and Torsvik2020). However, the Altai–Sayan Fold Belt is not included in Cocks and Torsvik's faunal provinces, which is the only difference from the present clathrodictyid faunal provinces. The accurate paleogeographic location of Tarim continues to be controversial, but consistent in the paleobiogeographic analyses among different fossil groups (compare Fig. 18 with Cocks and Torsvik, Reference Cocks and Torsvik2020, fig. 6). The occurrence of the brachiopod Altaethyrella–Schachriomonia assemblage in Tarim provided useful evidence, indicating a close paleobiogeographic connection with South China (Sproat and Zhan, Reference Sproat and Zhan2019), which corresponds with the occurrence of Camptodictyon amzassensis in Tarim and South China.
Laurentia, Baltica, and Siberia do not share any common Ordovician clathrodictyid species. The species-level diversity of clathrodictyids in these terranes is much lower than that of labechiids (Jeon et al., Reference Jeon, Liang, Park, Kershaw and Zhang2022b). The limited dispersal of clathrodictyid species in these faunal provinces is possibly due to the later appearance of clathrodictyid stromatoporoids than labechiids, so there was insufficient time for dispersal of clathrodictyids between terranes before the Late Ordovician glaciation.
Role of clathrodictyid stromatoporoids in intergrowth associations and implications in relation to the GOBE
During the Ordovician, in conjunction with spectacular diversity increases of marine invertebrate organisms, the rise of the Paleozoic evolutionary fauna is well known to possess a much-expanded occupation of ecospace and greater ecological complexity than in the earlier Cambrian period (Servais et al., Reference Servais, Owen, Harper, Kröger and Munnecke2010; Zhang et al., Reference Zhang, Zhan, Fan, Cheng and Liu2010). These complexities include better-developed food webs and increased competition between taxa (Servais et al., Reference Servais, Owen, Harper, Kröger and Munnecke2010; Zhang et al., Reference Zhang, Zhan, Fan, Cheng and Liu2010), which have been interpreted to be major controlling factors of this magnificent evolutionary event (Harper et al., Reference Harper, Cocks, Popov, Sheehan, Bassett, Copper, Holmer, Jin, Rong, Webby, Paris, Droser and Percival2004). Accordingly, the fossil record of paleoecological interactions increased dramatically during the Ordovician and multiplied in subsequent periods, together with the increase of predation pressure (Huntley and Kowalewski, Reference Huntley and Kowalewski2007; Zhang et al., Reference Zhang, Zhan, Fan, Cheng and Liu2010). It is considered that there were various modes of paleoecological associations (e.g., mutualism, commensalism, parasitism, competition) during the Ordovician (e.g., Young and Xu, Reference Young and Xu2002; Tapanila and Holmer, Reference Tapanila and Holmer2006; Lee et al., Reference Lee, Elias, Choh and Lee2016b; Jeon et al., Reference Jeon, Liang, Lee and Kershaw2020b), but it may have been underestimated in the development of Ordovician marine ecosystems (Zhang et al., Reference Zhang, Zhan, Fan, Cheng and Liu2010); such associations may have played critical roles in community organization and evolution through deep time (Young and Xu, Reference Young and Xu2002).
Almost coinciding with the appearance and development of massive calcareous skeletal organisms (e.g., bryozoans, corals, stromatoporoids) during the GOBE, a new symbiotic growth mode occurred during the Ordovician, which is well known as an intergrowth association (also commonly referred to as an endosymbiotic association). Before the Ordovician, a facultative simple attachment of one skeleton to another is dominant in paleoecological associations (e.g., Topper et al., Reference Topper, Holmer and Caron2015, Zhang et al., Reference Zhang, Strotz, Topper, Chen, Chen, Liang, Zhang, Skovsted and Brock2020), an association referred to as “ectosymbiotic.” Vinn (Reference Vinn2017) interpreted Cambrian epibionts to have simply benefited from a suspension-feeding strategy. One representative example of this ectosymbiotic interaction is Wiwaxia sp. and Nisusia sp. in the Cambrian Burgess Shale of Canada (Topper et al., Reference Topper, Holmer and Caron2015). The earliest known intergrowth association involved the early bryozoan Orbiramus and diverse sclerobionts reported from the Early Ordovician of South China (Ma et al., Reference Ma, Taylor and Buttler2021). Bryozoans commonly hosted various endobionts, such as cornulitid tubeworms and rugose corals, in the subsequent stages of the Ordovician (e.g., Vinn and Mõtus, Reference Vinn and Mõtus2012; Vinn et al., Reference Vinn, Wilson, Mõtus and Toom2014, Reference Vinn, Ernst and Toom2016, Reference Vinn, Ernst and Toom2017, Reference Vinn, Ernst and Toom2018a, Reference Vinn, Toom and Ernstb, Reference Vinn, Ernst, Wilson and Toom2019) and later (e.g., Plusquellec and Bigey, Reference Plusquellec and Bigey2019; Sendino et al., Reference Sendino, Suárez Andrés and Wilson2019). By contrast, early stromatoporoids (i.e., labechiids) seemingly did not involve intergrowth associations during the late Early to early Late Ordovician interval. The growth surface of those labechiid stromatoporoids with superposed vertical skeletal elements (i.e., pillars, papillae) has been interpreted as an unfavorable substrate for the settlement of endobionts’ larvae (Mori, Reference Mori1970), which may explain the lack of endobionts during the entire evolutionary history of labechiids.
Along with the appearance of clathrodictyid stromatoporoids during the middle Late Ordovician, diverse endobionts have been found within the skeletons of clathrodictyids (Table 2). Endobionts were first described from the middle Katian strata (Upper Ordovician) of New South Wales (Webby, Reference Webby1969; Webby and Morris, Reference Webby and Morris1976), including three clathrodictyid species and three different types of the endobiont coral Bajgolia (Webby, Reference Webby1969), tubeworms and unidentified tabular organisms (Webby, Reference Webby1969; Webby and Morris, Reference Webby and Morris1976). Other records are from the Upper Ordovician successions of Tasmania (Webby and Banks, Reference Webby and Banks1976) and South China (Lin and Webby, Reference Lin and Webby1988; Young and Xu, Reference Young and Xu2002; Lee et al., Reference Lee, Elias, Choh and Lee2016b; Jeon et al., Reference Jeon, Liang, Lee and Kershaw2020b). In particular, diverse endobionts are found in diverse clathrodictyid species from the Xiazhen Formation of South China (Table 1), which may correspond with the high biodiversity in South China during the GOBE (Rong et al., Reference Rong, Fang, Zhou, Zhan, Wang and Yuan2006). Most of the Ordovician examples of the intergrowth association between stromatoporoids and other organisms are previously known from peri-Gondwanan regions of the Late Ordovician (e.g., Lin and Webby, Reference Lin and Webby1988; Lee et al., Reference Lee, Elias, Choh and Lee2016b; Jeon et al., Reference Jeon, Liang, Lee and Kershaw2020b; Table 2), while many more examples have been reported worldwide in Silurian and Devonian limestones (e.g., Mori, Reference Mori1970; Kershaw, Reference Kershaw1987; Young and Noble, Reference Young and Noble1989; Zhen and West, Reference Zhen and West1997; Nestor et al., Reference Nestor, Copper and Stock2010; Vinn and Wilson, Reference Vinn and Wilson2010; Da Silva et al., Reference Da Silva, Kershaw and Boulvain2011; Vinn and Mõtus, Reference Vinn and Mõtus2014; Stearn, Reference Stearn and Selden2015b; Vinn, Reference Vinn2016; Kershaw et al., Reference Kershaw, Munnecke and Jarochowska2018).
Table 2. All reported occurrences of intergrowth associations between stromatoporoids and other organisms in the Ordovician are compiled by age and locality. Note that the Katian occurrences are all from peri-Gondwanan terranes, together with the earliest known appearances of clathrodictyids; (T), tabulate coral; (U), uncertain; (SR), solitary rugose coral; (St), stromatoporoid; (B), bryozoan; (TW), tubeworm.

Reef-forming organisms (i.e., bryozoans, corals, and sponges) diversified during the late Middle to the Late Ordovician, with their biodiversity peaks in the Katian (Carrera and Rigby, Reference Carrera, Rigby, Webby, Paris, Droser and Percival2004; Webby, Reference Webby, Webby, Paris, Droser and Percival2004; Ernst, Reference Ernst2018 Servais and Harper, Reference Servais and Harper2018), and it is apparent that the GOBE likely underpins the establishment of complex reef communities (Servais and Harper, Reference Servais and Harper2018). For benthic sessile organisms, their occupation of higher substrate by encrusting other skeletal organisms enhanced suspension feeding efficiency beyond that achieved by direct settlement on the seafloor (Vinn and Wilson, Reference Vinn and Wilson2010; Vinn et al., Reference Vinn, Wilson, Toom and Mõtus2015; Lee et al., Reference Lee, Elias, Choh and Lee2016b; Vinn, Reference Vinn2016; Jeon et al., Reference Jeon, Liang, Lee and Kershaw2020b). The intergrowth associations may have protected the endobionts from water turbulence and benefited suspension feeding as well (e.g., Kershaw, Reference Kershaw1987; Vinn and Wilson, Reference Vinn and Wilson2010; Vinn et al., Reference Vinn, Ernst and Toom2018a). Some cases of specificity between endobionts and particular host stromatoporoids may reflect complex biological interactions (see Fig. 10.6; Kershaw et al., Reference Kershaw, Munnecke and Jarochowska2018).
Understanding the intergrowth association between stromatoporoids and associated endobionts provides valuable information about not only paleoecological interactions but also paleobiological features on growth characteristics of associated organisms (Kershaw et al., Reference Kershaw, Munnecke and Jarochowska2018). Bending of laminae (including both upward and downward bending) in host stromatoporoids has been proposed as an indicator for judging the paleoecological relationships between host stromatoporoids and endobionts (e.g., Zapalski and Hubert, Reference Zapalski and Hubert2011. Downward bending of stromatoporoid laminae is common in the vicinity of the endobionts (black arrows in Fig. 15.1–15.5), and upward-bending laminae are commonly developed as well, even inside the same stromatoporoid skeleton (white arrows in Fig. 15.1, 15.3, 15.6). The deflection of host stromatoporoid laminae near endobiont tubes is variably developed during the Siluro–Devonian interval among host stromatoporoid species and intergrown organisms (e.g., Da Silva et al., Reference Da Silva, Kershaw and Boulvain2011; Kershaw, Reference Kershaw2013; Vinn, Reference Vinn2016; Kershaw et al., Reference Kershaw, Munnecke and Jarochowska2018). This is interpreted to indicate variation of growth rates between each host stromatoporoid and intergrown organisms during their syn–vivo interactions (Fig. 15.7–15.17). It is difficult to determine whether the interaction strategy is commensalism or mutualism due to lack of critical evidence (Zapalski, Reference Zapalski2011), but it seems unlikely that such interactions result from hostile actions, judging from the absence of skeletal distortion.
Overall, the increasingly diverse examples of the intergrowth association during the GOBE may be regarded as evidence for early development of complex reef ecosystems. The new growth strategy of syn–vivo association reflects increased competition for food and space in response to the dynamic increase of marine biodiversity. For stromatoporoids, the intergrowth association seems to have been facultative, depending on the emergence of the clathrodictyids as a new group of stromatoporoids during the Late Ordovician. Those host clathrodictyids represent high skeletal density compared with the earlier labechiids (Mistiaen, Reference Mistiaen1994; Vinn, Reference Vinn2016). The laminate structures of clathrodictyid stromatoporoids seemingly contributed to development of a complex reef ecosystem during the GOBE by providing stable substrate, enabling an increased substrate availability by suitable host clathrodictyid taxa (Fig. 17). As a result, the examples of intergrowth association between host clathrodictyid stromatoporoids and other intergrown organisms in the Xiazhen Formation reflect the highly complex paleoecological interactions of organisms in benthic communities during the GOBE.
Conclusions
Clathrodictyid stromatoporoids are abundant fossils in the Upper Ordovician Xiazhen Formation of South China. A total of nine species belonging to four genera of clathrodictyids are identified, including Clathrodictyon idense Webby and Banks, Reference Webby and Banks1976, Clathrodictyon megalamellatum Jeon n. sp., Clathrodictyon cf. Cl. mammillatum (Schmidt, Reference Schmidt1858), Clathrodictyon cf. Cl. microundulatum Nestor, Reference Nestor1964, Ecclimadictyon nestori Webby, Reference Webby1969, E. undatum Webby and Banks, Reference Webby and Banks1976, Camptodictyon amzassensis (Khalfina, Reference Khalfina1960), and Labyrinthodictyon cascum (Webby and Morris, Reference Webby and Morris1976). These clathrodictyid taxa are characterized by a combination of taxa recorded in New South Wales and Tasmania, although these two Australian regions themselves do not show any faunal affinity in terms of clathrodictyid stromatoporoids. The occurrence of the Xiazhen clathrodictyid assemblage may have resulted from independent faunal migration between South China and these two Australian regions. The northward shift of South China in the northeastern peri-Gondwanan region during the Middle to Late Ordovician may have facilitated the development of a favorable environment for the migration of clathrodictyid stromatoporoids among peri-Gondwanan terranes. Frequent intergrowth associations of these diverse clathrodictyid species with other sessile organisms are interpreted as evidence of increasing paleoecological interactions, which is critical for the development of complex benthic communities. The emergence of clathrodictyids in late Ordovician time increased the paleoecological complexity and benthic community stability of reef environments during the GOBE.
Acknowledgments
This study was funded by the Chinese Academy of Sciences (XDB26000000) and the National Natural Science Foundation of China (grant nos. 42030510, 41402013, and J1210006) to Y.D. Zhang and K. Liang. This study was also supported by the National Research Foundation of Korea (2019R1I1A1A01061336) to J. Park and by the Korea Polar Research Institute (KOPRI) project PE22060 to M. Lee. Chinese Academy of Sciences (CAS) “One Belt and One Road” Master Fellowship, ANSO Scholarships for Young Talents, and the 2019 and 2020 Nanjing Municipal Government International Students Scholarship to J. Jeon are also acknowledged.
The authors thank editors S. Zamora and J. Botting and reviewers L. Muir (National Museum Wales) and I. Percival (Geological Survey of New South Wales) for their valuable reviews. This manuscript was particularly improved by thoughtful comments from I. Percival. We sincerely appreciate U. Toom (Tallinn University of Technology), O. Obut (Trofimuk Institute of Petroleum Geology and Geophysics), and P. Smith (Australian Museum) for providing references and opportunities to check the stromatoporoid type specimens from Estonia, Altai, and New South Wales of Australia. We are also grateful to D.-J. Lee (Jilin University), X.-D. Wang (Nanjing University), and H. Park for their assistance in fieldwork and thin-section preparation during the past decade. The warm hospitality and assistance of the residents of Zhuzhai village during fieldwork are also greatly appreciated. This paper is a contribution to IGCP 735 “Rocks and the Rise of Ordovician Life: Filling knowledge gaps in the Early Palaeozoic Biodiversification.”
Data availability statement
Data available from the Dryad Digital Repository: https://doi.org/10.5061/dryad.83bk3j9t5.