1. Introduction
Sedimentary rocks can record the complex coupling histories between basins and orogenic belts, which is an important way to study continental structure and dynamics (Li et al. Reference Li, Wang and Zeng2000; Liu, Reference Liu2001; Wang & Li, Reference Wang and Li2003). The Central Asian Orogenic Belt (CAOB), distributed between the Siberian plate and the North China Craton (NCC), is one of the largest and most complex Phanerozoic accretion orogenic belts on Earth (Windley et al. Reference Windley, Alexeiev, Xiao, Kröner and Badarch2007; Xiao et al. Reference Xiao, Windley, Huang, Han, Yuan, Chen, Sun, Sun and Li2009). It was mainly formed by the continuous accretion of the Palaeo-Asian ocean-arc complex (Mossakovsky et al. Reference Mossakovsky, Ruzhentsev, Samygin and Kheraskova1993; Dobretsov et al. Reference Dobretsov, Berzin and Buslov1995; Sengor & Natal’in Reference Sengor, Natal’in, Yin and Harrison1996; Xiao et al. Reference Xiao, Windley, Hao and Zhai2003; Windley et al. Reference Windley, Alexeiev, Xiao, Kröner and Badarch2007; Kozakov et al. Reference Kozakov, Sal’nikova, Kovach, Yarmolyuk, Anisimova, Kozlovskii, Plotkina, Myskova, Fedoseenko, Yakovleva and Sugorakova2008; Kröner et al. Reference Kröner, Lehmann, Schulmann, Demoux, Lexa, Tomurhuu, Stipska, Liu and Wingate2010, Reference Kröner, Kovach, Belousova, Hegner, Armstrong, Dolgopolova, Seltmann, Alexeiev, Hoffmann, Wong, Sun, Cai, Wang, Tong, Wilde, Degtyarev and Rytsk2014, Reference Kröner, Kovach, Kozakov, Kirnozova, Azimov, Wong and Geng2015; Xiao & Santosh, Reference Xiao and Santosh2014; Li et al. Reference Li, Qiu, Chang and Yang2015). After the late Palaeozoic, the CAOB was tectonically controlled by the Palaeo-Asian Ocean system and records an extended period of collision and splicing between the Siberian plate and the NCC involving a series of microcontinental blocks. This continued until the final Palaeo-Asian Ocean closure (Cao et al. Reference Cao, Yang and Tian1986; XX Zhao et al. Reference Zhao, Coe, Zhou, Wu and Wang1990; Y Zhao et al. Reference Zhao, Yang and Ma1994; Nozaka & Liu, Reference Nozaka and Liu2002; Xiao et al. Reference Xiao, Windley, Hao and Zhai2003; Li Reference Li2006; Kusky et al. Reference Kusky, Windley and Zhai2007; Chen et al. Reference Chen, Chen, Xu, Lin and Shang2011). It is important to place accurate temporal constraints on Palaeo-Asian Ocean closure in order to clarify the tectonic evolution and metallogenic events of the CAOB and adjacent sedimentary basins (Li et al. Reference Li, Gao, Guan and Li2009).
Previous researchers have argued for different Palaeo-Asian Ocean closure times, including the pre-Late Devonian (Cao et al. Reference Cao, Yang and Tian1986; Xu & Chen, Reference Xu and Chen1997; Zhao et al. Reference Zhao, Chen, Xu, Faure, Shi and Choulet2013), Carboniferous (Li, Reference Li1986; Shao,Reference Shao1991), middle Permian (Li Reference Li2021), late Permian (Xiao et al. Reference Xiao, Windley, Hao and Zhai2003; JY Li, Reference Li2006; PW Li Reference Li, Gao, Guan and Li2006, Reference Li, Gao, Guan and Li2009), late Palaeozoic to early Mesozoic (Xiao et al. Reference Xiao, Windley, Hao and Zhai2003; Windley et al. Reference Windley, Alexeiev, Xiao, Kröner and Badarch2007; Tong et al. Reference Tong, Hong, Wang, Shi, Zhang and Zeng2010; Wilhem et al. Reference Wilhem, Windley and Stampfli2012), and Mesozoic (XX Zhao et al. Reference Zhao, Coe, Zhou, Wu and Wang1990; Y Zhao et al. Reference Zhao, Yang and Ma1994; Nozaka & Liu, Reference Nozaka and Liu2002). This reflects the complexity of Palaeo-Asian Ocean closure.
The Ordos Basin is located in the western NCC, to the south of the CAOB (Fig. 1). The Late Palaeozoic deposition and sedimentation in the north part of this basin are thought to have been affected by closure of the Palaeo-Asian Ocean (Windley et al. Reference Windley, Alexeiev, Xiao, Kröner and Badarch2007; Xiao et al. Reference Xiao, Windley, Sun, Li, Huang, Han, Yuan, Sun and Chen2015). To elucidate the provenance and tectonic setting of the northern margin of the basin during the late Palaeozoic, previous studies mainly focused on petrology (Liu et al. Reference Liu, Huang, Wei, Sun and Lin2003; Wang et al. Reference Wang, Tian, Chen, Li and Xiao2009; Liang et al. Reference Liang, Chen, Zhao, Mei, Liu and Yang2016) and geochemical characteristics (Chen et al. Reference Chen, Li, Hu, Li, Pang and Guo2012). Results indicated that the Upper Palaeozoic sandstone was mainly derived from Yinshan–Alxa ancient land to the north, and that the east and west sides of the basin are of different provenance. Meanwhile, some scholars clarified the clastic components, debris types and formation age of parent rocks in local areas of the basin via detrital zircon U–Pb dating of drill core samples from the northern part of the basin (Luo et al. Reference Luo, Wu and Zhao2010; Liu et al. Reference Liu, Wang, Shi, Zhang, Qin, Qi and Xu2020). However, previous researchers did not use the chronological information contained in basin sedimentary rocks to constrain the tectonic processes in the CAOB in order to refine the timing of Palaeo-Asian Ocean closure in the north of the Ordos Basin.

Fig. 1. (a) Geological map of the northern Ordos Basin with location of the sections marked as stars (modified from Li et al. Reference Li, Qiu, Chang and Yang2015). (b) Location of North China Craton and Ordos Basin (after Zuza and Yin, Reference Zuza and Yin2017). (c) Geological cross-section in the Ordos Basin (modified from Dong, Reference Dong2009).
U–Pb dating of detrital zircons informs provenance analysis and regional tectonic evolution (Gehrels et al. Reference Gehrels, Dickinson, Ross, Stewart and Howell1995; Zhao et al. Reference Zhao, Liu, Wang, Gao, Li, Zhuo, Qiao, Zhang and Jiang2015; Guo et al. Reference Guo, Liu, Wang and Deng2018). Therefore, the focus of this work is to compare the provenance on the east and west sides of the Middle Permian Lower Shihezi Formation in the northern Ordos Basin through systematic petrological and quantitative mineralogical analysis. In addition, the closure process of the Palaeo-Asian Ocean north of the Ordos Basin is investigated by in situ U–Pb dating of detrital zircon and trace element analysis.
2. Geological setting and stratigraphy
The Ordos Basin is a large multi-cycle superimposed cratonic basin, bordered by the Alxa block and Yinshan orogenic belt to the northwest and north, the Qilian–Qinling tectonic belt to the south, the Helan–Liupan Mountains to the west and the Luliang Mountains to the east (Fig. 1). The western segment of the Yinshan orogenic belt mainly exposes Neoarchaean lower amphibolite green-schist facies granite–greenstone, Neoarchaean tonalite–trondhjemite–granodiorite (TTG) high-grade metamorphic granulite, and Late Palaeozoic granite bodies (Zhang, Reference Zhang2004; Zheng et al. Reference Zheng, Wu, Zhang, Xu, Meng and Zhang2014; Wang et al. Reference Wang, Han, Feng and Liu2015; M Liu et al. Reference Liu, Zhang, Xiong, Zhao, Di, Wang and Zhou2016a). The heavy mineral assemblage shows high contents of zircon and garnet (Wang, 2011). The Alxa block is mainly composed of middle and high-grade metamorphic rocks of the Neoarchaean Alxa Group and the Qianlishan Group, and Early Palaeozoic and Late Palaeozoic granite bodies (Li et al. Reference Li, Shen, Li, Zhou, Guo and Li2004; Feng et al. Reference Feng, Xiao, Windley, Han, Wan, Zhang, Ao, Zhang and Lin2013; Dan et al. Reference Dan, Li, Wang, Wang and Liu2014; Xue et al. Reference Xue, Ling, Liu, Zhang and Sun2017; Zhang & Gong, Reference Zhang and Gong2018) (Fig. 2). The heavy minerals assemblages are mainly characterized by high contents of tourmaline and magnetite (Wang, 2011). The Late Palaeozoic tectonic and sedimentary evolution of the Ordos Basin was mainly controlled by the rifting and subduction of the Palaeo-Asian Ocean (northern margin), Qinling Ocean (southern and southwest margins) and the associated Helan trough (Chen et al. Reference Chen, Chen, Xu, Lin and Shang2011).

Fig. 2. Distributions of crystalline rocks in surrounding tectonic units, showing primary potential sources for detrital zircons in the northern Ordos Basin, modified after 1:2 500 000 geological map of China (Chen QH, Li WH, Hu XL, Li KY, Pang JG, Guo YQ 2012 Tectonic setting andprovenance analysis of late Paleozoic sedimentary rocks in the Ordos Basin. Acta Geologica Sinica 86, 1150–1162. China Geological Survey, 2004) and Precambrian metamorphic basement (Lei et al. Reference Lei, Liu, Zhang, Wu, Wang, Cun and Sun2017; Zhang & Gong, Reference Zhang and Gong2018). The age ranges of the magmatic rocks in the Alxa block were extracted from Li et al. (Reference Li, Shen, Li, Zhou, Guo and Li2004, Reference Li, Zhai, Yang, Wang, Li, Cui, Zhou, Liu, Liu and Li2010), Feng et al. (Reference Feng, Xiao, Windley, Han, Wan, Zhang, Ao, Zhang and Lin2013), Dan et al. (Reference Dan, Li, Wang, Wang and Liu2014), Zheng et al. (Reference Zheng, Wu, Zhang, Xu, Meng and Zhang2014) and Xue et al. (Reference Xue, Ling, Liu, Zhang and Sun2017). Those for the Yinshan Orogenic Belt are from Zhang (Reference Zhang2004), Jian et al. (Reference Jian, Zhang, Liu, Jin, Jia and Qian2005), Zhong et al. (Reference Zhong, Deng, Wan, Mao and Li2007), Ma et al. (Reference Ma, Wan, Xu, Liu, Xie, Dong and Liu2012), Wang et al. (Reference Wang, Han, Feng and Liu2015), M Liu et al. (Reference Liu, Zhang, Xiong, Zhao, Di, Wang and Zhou2016a) and H Zhou et al. (Reference Zhou, Liu, Zhou and Zou2019).
Benxi, Taiyuan, Shanxi, Lower Shihezi, Upper Shihezi and Shiqianfeng formations were successively deposited in the Ordos Basin during the Late Palaeozoic. The Lower Shihezi Formation is a set of light-grey gravelly-coarse sandstone, grey-white medium-coarse sandstone and grey-green lithic quartz sandstone. The sandstone has developed large cross-bedding and low shale content. The thickness is generally 120–160 m (Fig. 3). The Late Palaeozoic sedimentary evolution of the basin included two major stages: epicontinental sea and intracontinental depression. From the late Carboniferous to to the early Permian, the basin was in the epicontinental sea stage, dominated by marine sediments. From the late Early Permian to the Late Permian, the regional structure changed from extensional to contractional tectonic settings, the seawater gradually withdrew to the SW and SE, and deposition was dominated by continental sediments. This stage can be subdivided into the transitional offshore lake basin of the Shanxi Formation and the intracontinental depression basin of the Lower Shihezi Formation – Shiqianfeng Formation (Chen et al. Reference Chen, Hou, Tian, Liu and Zhang2001). During the deposition of the Middle Permian Lower Shihezi Formation, the seawater had completely withdrawn from the Ordos Basin, and the palaeoclimate had changed from humid to dry. A set of grey-white and yellow-green terrigenous clastic sediments were deposited in the basin at this time (Xiao et al. Reference Xiao, Liu, Zhang, Lin and Zhang2019). The boundary line between Lower Shihezi Formation and Shanxi Formation is defined by the fusulinid fossils Misellina and Cancellina, and the characteristic fossil plant assemblage is Giantonoclea largelii – Alethopteris norinii (Shanxi Coalfield Geological Exploration Corporation Group 114 and Nanjing Institute of Geology and Palaeontology, 1987).

Fig. 3. Stratigraphic column of Late Palaeozoic in Ordos Basin.
3. Sampling and analytical methods
3.1 Sampling
Forty-eight sandstone samples were collected from the Middle Permian Lower Shihezi Formation on the east and west sides of the northern Ordos Basin. Ten samples from the Longwanggou section, fourteen samples from the Liulin section, six samples from the Shabatai section, and eighteen samples from the Qianlishan section of the Lower Shihezi Formation were selected for microscopic observation. The Lower Shihezi Formation in these sections consists of light-grey, gravelly coarse sandstone, grey-white medium-coarse sandstone and small amounts of grey-green fine sandstone and mudstone. Detrital zircon laser ablation – inductively coupled plasma – mass spectrometry (LA-ICP-MS) U–Pb dating was undertaken on six samples (sample locations identified with a yellow dot in Fig. 4). The Qianlishan and Shabatai sections are located in the western part of the study area, while the Longwanggou, Fugu and Liulin sections are located in the eastern part of the study area. The Middle Permian Lower Shihezi Formation in these sections is well developed and well exposed.

Fig. 4. Schematic stratigraphy. (a, b) Photographs of Qianlishan outcrop sections; (c) photograph of Shabatai outcrop sections; (d) outcrop photos – rose diagram inset shows palaeocurrent directions for each section as determined during fieldwork in this study; (e) photograph of Longwanggou outcrop sections; (f) photograph of Fugu outcrop sections; (g) photograph of Liulin outcrop sections; (h) schematic stratigraphy of the Lower Shihezi Formation in Qianlishan outcrop sections; (i) schematic stratigraphy of the Lower Shihezi Formation in Shabatai outcrop sections; (j) schematic stratigraphy of the Lower Shihezi Formation in Longwanggou outcrop sections; (k) schematic stratigraphy of the Lower Shihezi Formation in Fugu outcrop sections; (l) schematic stratigraphy of the Lower Shihezi Formation in Liulin outcrop sections. Sampled horizons are marked by yellow dot in all sections.
Samples QL-1 (39° 50′ 35.67″ N, 106° 59′ 53.3″ E) and QL-2 (39° 50′ 35.5″ N, 106° 59′ 53.1″ E) were collected from the lower and middle successions of the Lower Shihezi Formation in the Qianlishan section, respectively. Samples SB (39° 17′ 22.64″ N, 106° 34′ 29.65″ E) was taken from the Lower Shihezi Formation in the Shabatai section. Samples LW (39° 25′ 53.10″ N, 111° 16′ 06.00″ E) was taken from the Longwanggou section. Samples FG (39° 01′ 57.02″ N, 111° 05′ 19.66″ E) and LL (37° 25′ 55.61″ N, 110° 52′ 56.12″ E) were taken from the Fugu section and the Liulin section, respectively (Fig. 4).
3.2 Quantitative mineralogical analysis
Automated quantitative mineralogy studies of the studied rocks were carried out using the TESCAN integrated mineral analyzer (TIMA) platform at the John de Laeter Centre (JdLC) in Curtin University, Western Australia. Samples were analysed using the Dot Mapping acquisition mode at a 3.0 μm pixel resolution. The raw data were processed using TIMA Software v1.5.47. The TIMA integrates a high-resolution field emission scanning electron microscope with four silicon drift energy-dispersive spectroscopy detectors, allowing ultrafast measurements of mineral associations, concentrations and grain sizes, as well as element distributions on multiple samples of grain mounts, thin-sections or polished sections (Ward et al. Reference Ward, Merigot and McInnes2018). Its capability for microscale mineralogical analysis of rock chip samples is thus valuable for lithological characterization of sedimentary basins (Z Li et al. Reference Li, McInnes, Tessalina and Ware2018).
3.3 Zircon U–Pb dating trace element analysis and Lu–Hf isotopes
Zircons were separated from 3 kg hand specimens using conventional heavy liquid and magnetic techniques at the Langfang Regional Geological Survey, Hebei Province, China. Handpicked zircons were mounted in epoxy resin and polished to expose zircon grain interiors. All the zircons were photographed in transmitted and reflected light to characterize external morphology. Back-scattered electron (BSE) and cathodoluminescence (CL) images were obtained on a JEOL JXA-8100 electron microprobe. The LA-ICP-MS U–Pb isotope and trace element analyses were performed using a RESOlution 193 excimer laser ablation system coupled to an Agilent 7700 quadrupole ICP-MS in the GeoHistory Facility, JdLC, Curtin University, Western Australia. The detailed analytical procedures for detrital zircon LA-ICP-MS analysis in this facility have been described elsewhere (e.g. Barham et al. Reference Barham, Reynolds, Kirkland, O’Leary, Evans, Allen, Haines, Hocking and McDonald2018; Jabaloy-Sánchez et al. Reference Jabaloy-Sánchez, Talavera, Gómez-Pugnaire, López-Sánchez-Vizcaíno, Vázquez-Vílchez, Rodríguez-Peces and Evans2018) and are briefly summarized here. Following two cleaning pulses and 40 s of baseline acquisition, ablations were carried out at a fixed spot size of 33 μm, frequency of 4 Hz and on-sample energy of 2 J cm−2. Standard blocks (NIST 610 and NIST 612 silicate glasses and GJ-1, 91500, OGC and Plesoviçe reference zircons) were analysed every 20 unknowns yielding 17 replicates of each trace element and age standard. For trace element determination, matrix-matched, well-characterized zircon GJ-1 was used as the primary reference material for correction of instrument drift and calculation of most elemental abundances (assuming stoichiometric 43.14 % 91Zr in unknowns). Where recommended elemental abundance was not certified in GJ-1 (e.g. P and Ta), NIST 610 was used as the primary reference material to calculate elemental concentrations using 29Si as the internal standard element and assuming 14.76 % 29Si in zircon unknowns. Stoichiometric values are taken from webmineral.com. With NIST 610 as the primary reference material, secondary trace element standard NIST 612 yielded recommended elemental abundances within 3 % (Jochum et al. Reference Jochum, Weis, Stoll, Kuzmin, Yang, Raczek, Jacob, Stracke, Birbaum, Frick, Gunther and Enzweiler2011).
For geochronology, a block of primary and secondary zircon reference materials was included. The 206Pb/238U ages for younger zircons (<1500 Ma) were calculated using 91500 as the primary reference material, whereas OGC (Stern et al. Reference Stern, Bodorkos, Kamo, Hickman and Corfu2009) was the primary reference for older zircons (>1500 Ma) (Spencer et al. Reference Spencer, Kirkland and Taylor2016). Errors cited for individual analysis are at the 2σ level. Zircon reference materials GJ-1 and Plesoviçe were treated as unknowns and yielded a mean 206Pb/238U age of 601.6 ± 1.1 Ma (MSWD = 1.07) for GJ-1 and 338.9 ± 1.6 Ma (MSWD = 0.24) for Plesoviçe, in good agreement with the recommended ages of 599.8 ±1.7 Ma and 337.13 ± 0.37 Ma, respectively (Jackson et al. Reference Jackson, Pearson, Griffin and Belousova2004; Sláma et al. Reference Sláma, Košler, Condon, Crowley, Gerdes, Hanchar, Horstwood, Morris, Nasdala, Norberg, Schaltegger, Schoene, Tubrett and Whitehouse2008). With 91500 as the primary, the mean 207Pb/206Pb age obtained on OGC was 3468.1 ± 3.3 Ma (2σ, n = 50, MSWD = 3.1), in good agreement with the recommended age (3465.4 ± 0.6 Ma (2σ); Stern et al. Reference Stern, Bodorkos, Kamo, Hickman and Corfu2009). Detrital zircon data with concordance values ((206Pb/238U) age/(207Pb/235U) age × 100 %) of 70–130 % are used in the discussion below. Raw.csv data were reduced using the Trace Elements and UPbGeochron4 data reduction schemes in Iolite 3.7. Zircon U–Pb age concordia and probability density plots were made in ISOPLOT (ver. 3.0) (Ludwig, Reference Ludwig2003). To avoid altered zircons or accidental analysis of inclusions, zircon trace element analyses with Ti > 50 ppm and REE + Y > 1 wt % were discarded (Hoskin & Schaltegger, Reference Hoskin and Schaltegger2003).
In situ zircon Lu–Hf isotopic analysis was carried out on a Neptune Plus MC-ICP-MS (Thermo Fisher Scientific, Germany) in combination with a Geolas 2005 excimer ArF laser ablation system (Lambda Physik, Göttingen, Germany) hosted at the State Key Laboratory of Geological Processes and Mineral Resources, China University of Geosciences (Wuhan). Instrumental conditions and data acquisition are described by Liu et al. (Reference Liu, Gao, Hu, Gao, Zong and Wang2010). Analyses were conducted with a beam diameter of 44 μm and a hit rate of 6 Hz. In order to correct for the isobaric interferences of 176Lu and 176Yb on 176Hf, 176Lu/175Lu = 0.02655 and 176Yb/173Yb = 0.7962 ratios were determined (Chu et al. Reference Chu, Taylor, Chavagnac, Nesbitt, Boella, Milton, German, Bayon and Burton2002). For instrumental mass bias correction Yb isotope ratios were normalized to 172Yb/173Yb of 1.35274 (Chu et al. Reference Chu, Taylor, Chavagnac, Nesbitt, Boella, Milton, German, Bayon and Burton2002) and Hf isotope ratios to 179Hf/177Hf of 0.7325 (Patchett & Tatsumoto, Reference Patchett and Tatsumoto1980) using an exponential law.
3.4 Sandstone modal analysis
In addition to the measurement of the mineral associations of four samples by TIMA, the petrographic analysis of 39 sandstone samples in the study area was carried out according to the method of Gazzi Dickinson (Dickinson et al. Reference Dickinson, Beard, Brakenridge, Erjavec, Ferguson, Inman, Knepp, Lindberg and Ryberg1983; Ingersoll et al. Reference Ingersoll, Bullard, Ford, Grimm, Pickle and Sares1984). On average, 300 points were counted on every standard thin-section using a petrographic microscope, and the numbers of point-count grains were normalized to percentages.
3.5 Palaeocurrent direction
Sedimentary interpretations for sections in the study area are constructed on the basis of field investigations on lithological assemblages and sedimentary structures. Four sections are established to exhibit lithology and sedimentary variation (Fig. 4). Palaeocurrent data were obtained by field measurements on cross-stratifications of sandstone. Finally, the original palaeocurrent was restored by stereographic projection and rose map.
4. Results
4.1. Lithology and quantitative mineralogy
The microscopic observation results are plotted in the triangular QFL compositional diagram (classification after Garzanti, Reference Garzanti2019) (Fig. 5). Most data from the Qianlishan and Shabatai sections plot in the FQ and Q field, while the data from the Longwanggou and Liulin sections plot in the lFQ, fLQ and LQ field.

Fig. 5. Triangular QFL plot with data plotted from microscopic observation and the TIMA analysis for the Lower Shihezi Formation sandstones in the northern Ordos Basin (QFL plot from Garzanti, Reference Garzanti2016, Reference Garzanti2019). Q = quartzose; F = feldspathic; L = lithic; FQ = feldspatho-quartzose (fFQ = feldspar-rich; qFQ = quartz-rich); QF = quartzo-feldspathic; LF = litho-feldspathic; FL = feldspatho-lithic; QL = quartzo-lithic; LQ = litho-quartzose; lFQ = litho-feldspatho-quartzose; lQF = litho-quartzo-feldspathic; qLF = quartzo-litho-feldspathic; qFL = quartzo-feldspatho-lithic; fQL = feldspatho-quartzo-lithic; fLQ = feldspatho-litho-quartzose.
Sandstones of the Lower Shihezi Formation of the northern Ordos Basin are mostly medium- to coarse-grained, with sub-angular to sub-rounded grains. The Qianlishan section is composed of medium-grained lithic quartz sandstone and feldspathic quartz sandstone. The Shabatai section is mainly composed of medium-grained lithic quartz sandstone. There is rare volcanic material but more metamorphic rock debris in these two sections (Fig. 6a, b, c). Some volcanic ash in the Shabatai section sandstone has been altered (Fig. 6c). The Longwanggou section consists of tuffaceous sandstone, tuff and quartz sandstone with tuffaceous debris. The content of volcanic quartz is high (30–40 %). Feldspar grains show evidence of dissolution or abrasion. The rock debris content is small, and is primarily siliceous. There is a lot of volcanic ash found in the matrix (Fig. 6e). The Liulin section is dominated by medium-grained lithic sandstone (Fig. 6f). Sandstone contains more metamorphic, volcanic and sedimentary rock debris.

Fig. 6. (a) Metamorphic rock debris from the Lower Shihezi Formation in the Qianlishan section; (b) lithic quartz sandstone with gravel included from the Lower Shihezi Formation in the Qianlishan section; (c) volcanic ash matrix in lithic quartz sandstone from the Lower Shihezi Formation in the Shabatai section; (d) extrusive rock debris included in sandstone from the Lower Shihezi Formation in the Longwanggou section; (e) volcanic quartz in sandstone from the Lower Shihezi Formation in the Longwanggou section; (f) rock-fragment sandstone from the Lower Shihezi Formation in the Liulin section.
The plagioclase An (anorthite) content in sandstone samples from the Qianlishan and Shabatai sections in the west is low, whereas those in eastern samples generally show higher An content. This is consistent with the modal mineral abundance revealed by TIMA. TIMA analysis shows that the content of albite is high and the content of anorthite is low in sandstone samples from the Qianlishan and Shabatai sections in the west, while the content of anorthite in eastern samples increases significantly (Fig. 7). In terms of heavy minerals, the samples from the eastern sections of the northern Ordos Basin contain abundant zircons, and the heavy mineral assemblage is zircon–rutile–tourmaline–garnet, while the samples from the western sections in the North Ordos Basin contain high contents of hematite/magnetite. The heavy mineral assemblage is mainly hematite/magnetite – tourmaline – rutile. These TIMA results are consistent with the results of microscopic observation.

Fig. 7. The TIMA mineral maps for four samples and the energy-dispersive spectroscopy of minerals.
4.2. Zircon morphologies and genesis
A total of 640 zircon grains from the six sandstone samples were characterized. Representative CL images of analysed grains are presented in Figure 5. According to the CL images, zircons from the study area can be divided into two types. Most zircons display clear oscillatory zoning or fan-shaped zoning structures (Hu et al. Reference Hu, Zhai, Tang, Wang and Wang2016) (Fig. 8), characteristic of magmatic zircon. The internal structure of metamorphic zircon can be non-zonal or weakly zonal, or characterized and diversified zonal (Wu & Zheng, Reference Wu and Zheng2004). Most grains are sub-rounded and sub-angular, which reflects transformation by weathering, transport and abrasion. Other acicular zircons indicate short transport distance or derivation from ash. All zircon grains are 60–150 μm in length, with length/width ratios ranging from 1:1 to 3:1 (Fig. 8). Th/U ratios are good discriminators of whether a zircon is likely metamorphic or igneous (Hoskin & Black, Reference Hoskin and Black2000; Hartmann & Santos, Reference Hartmann and Santos2004; Kirkland et al. Reference Kirkland, Smithies, Taylor, Evans and McDonald2015). The Th/U ratio of zircon grains ranges from 0.04 to 2.87. Most plot above the Th/U = 0.1 line (Fig. 9), which usually reflects a magmatic signature (Hoskin & Black, Reference Hoskin and Black2000; Wang et al. Reference Wang, Williams, Chen, Huang and Li2011).

Fig. 8. CL images of representative detrital zircons from the Lower Shihezi Formation sandstones in the northern Ordos Basin. In each case, the yellow circle represents the location of a 33 µm ablation spot.

Fig. 9. The distribution of zircon U–Pb ages vs Th/U ratio of detrital zircons in the Permian Lower Shihezi Formation sandstone in the northern Ordos Basin.
4.3. U–Pb ages and Lu–Hf isotopes
U–Pb concordia plots and relative U–Pb age probability plots for all samples are shown in Figure 10. Following Gehrels (Reference Gehrels, Busby and Azor2012) we used a 30 % discordance cut-off for zircons to preserve the relative proportion of zircons with different ages.

Fig. 10. Concordia diagrams and relative probability plots showing detrital zircon U–Pb ages.
The QL-1 sample: 107 grains were used to produce the probability plot. The measured ages generally range from 2584 Ma to 286 Ma, and show major age groups at 339–286 Ma and 505–412 Ma, with subordinate groups at 2540–2317 Ma and 2150–1680 Ma, which define distinct age peaks at 301 Ma, 325 Ma, 443 Ma and 2482 Ma, respectively.
The QL-2 sample: 106 grains were used to produce the probability plot. The four major age peaks are at 298 Ma, 326 Ma, 435 Ma and 2485 Ma, respectively. There is one minor peak at 1917 Ma.
The SB sample: 106 zircon grains yielded concordant ages of 2660–291.6 Ma. The age population displays four major peaks (297 Ma, 324 Ma, 444 Ma and 2521 Ma) and one minor peak (1860 Ma).
The LW sample: The age range of these 109 zircon grains is 2581–287.7 Ma, with one main age peak at 298 Ma, and two subordinate peaks at 1938 Ma and 2510 Ma.
The FG sample: The age range of these 102 zircon grains is 2625.61–286.7 Ma, with three main age peaks at 316 Ma, and two subordinate peaks at 1838 Ma and 2423 Ma.
The LL sample: 111 zircon grains yielded concordant ages. The measured ages ranged from 2517 Ma to 298 Ma. The probability plots show various zircon populations with peaks at 302 Ma, 1850 Ma and 2460 Ma.
Notably, the zircons in the eastern sections do not present an Ordovician age peak at c. 440 Ma, which is the dominant age peak in the Shabatai and Qianlishan samples.
A total of 69 Phanerozoic zircons (with concordances of > 70 %) were analysed for Hf isotope compositions. All of the analysed zircons yield negative ϵHf(t) values ranging from −2.0 to −27.8.
4.4. Detrital zircon trace elements
U, Th, Hf and rare earth elements (REEs) are refractory in zircon, and can be used to elucidate the nature of the parent rock (Grimes et al. Reference Grimes, John, Kelemen, Mandab, Wooden, Cheadle, Hanghøj and Schwartz2007). In the chondrite-normalized REE diagram, most of the zircon grains show similar REE distribution patterns. The ∑HREE /∑LREE ratios of the samples are 0.93–413.21, with an average of 84.24, thus demonstrating enrichment of heavy REEs (HREEs). Most of the zircon grains show positive Ce anomalies (Ce/Ce* = 0.51–861.87, with an average of 38.92) and negative Eu anomalies (Eu/Eu* = 0.02–1.16, with an average of 0.34) (Fig. 11), typical of magmatic zircons (Hoskin & Ireland, Reference Hoskin and Ireland2000; Belousova et al. Reference Belousova, Griffin, O’Reilly and Fisher2002).
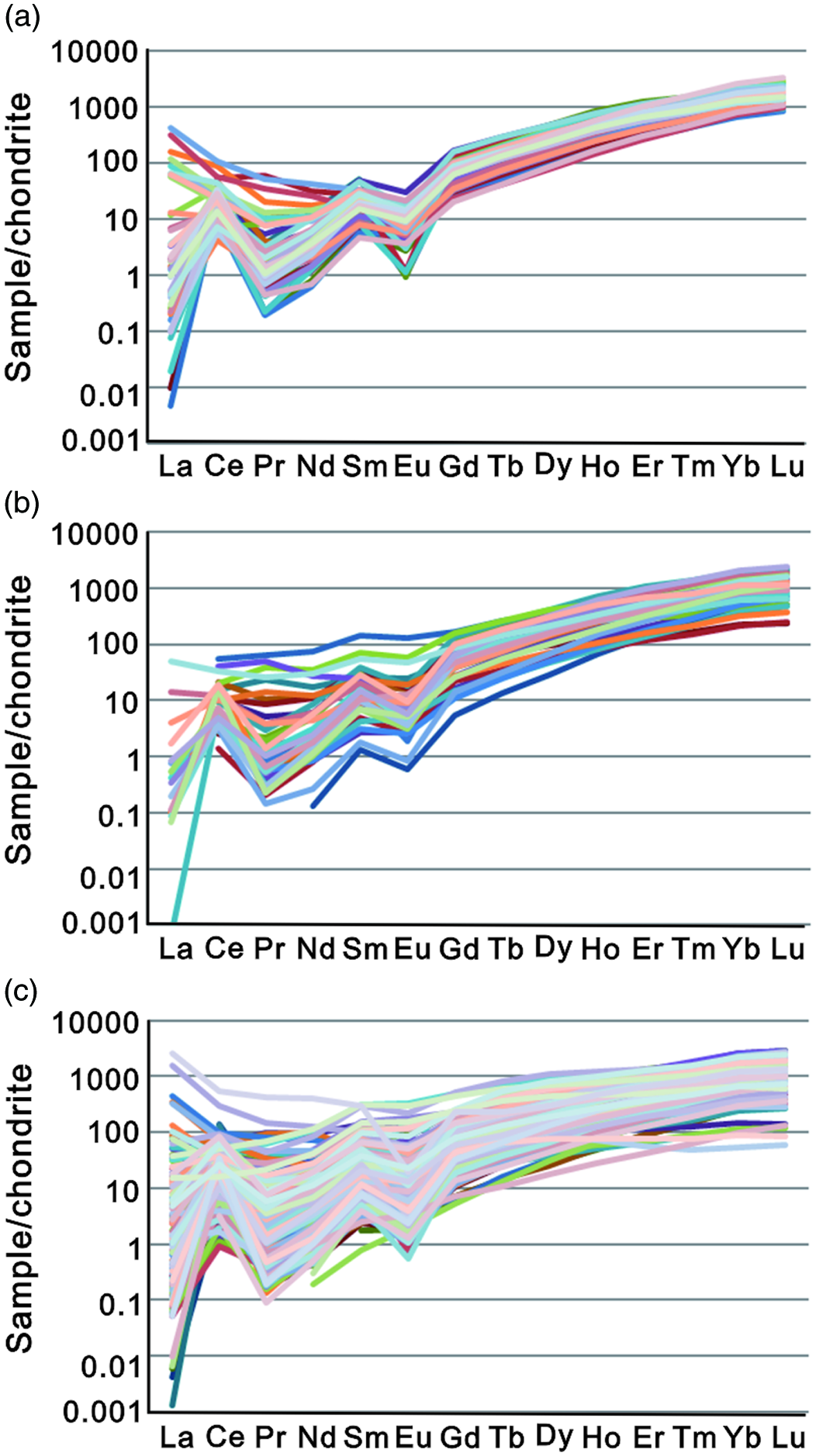
Fig. 11. Chondrite-normalized REE patterns of all analysed zircons from the northern Ordos Basin. (a) Late Palaeozoic REE patterns; (b) Early Palaeozoic REE patterns; (c) Neoarchaean–Palaeoproterozoic REE patterns. Chondrite values from McDonough & Sun (Reference McDonough and Sun1995).
5. Discussion
5.1 Provenance analysis
The sandstone petrology observed in both light microscope and TIMA characterization emphasizes the stark contrast between eastern and western samples from this study. The proportion of metamorphic and extrusive rock debris in the western sections is significantly higher than that in the eastern sections, but the content of volcanic quartz in the eastern section is much higher than that in the western sections. TIMA results indicate that the heavy mineral assemblage in the western sections is mainly hematite/magnetite – rutile – tourmaline, which corresponds to the rock characteristics of the Alxa Group and Qianlishan Group in the west. The heavy mineral assemblage in the eastern sections is zircon–rutile–tourmaline–garnet, which is similar to the heavy mineral characteristics of Wulashan Group, Jining Group, Zhaertaishan Group and Baiyun Obo Group in the east (Dou et al. Reference Dou, Hou and Dong2009). Rose diagrams, made after sedimentary strata correction of sedimentary structure obtained from field measurements, indicate that palaeocurrents at Qianlishan and Shabatai were primarily southeastward, while the palaeocurrents at Longwanggou were primarily south-southwestward (Fig. 4; Table 1).
Table 1. Contrasting characteristics of samples west and the east of the Permian Lower Shihezi Formation, northern Ordos Basin

Some zircons are euhedral (Fig. 8), which indicates that their source region was relatively close to the depositional site. The Th/U ratios of most grains are >0.4, indicating a magmatic origin (Belousova et al. Reference Belousova, Griffin, O’Reilly and Fisher2002; Corfu et al. Reference Corfu, Hanchar, Hoskin and Kinny2003). CL and Th/U indicate igneous origins for the vast majority of grains and we can therefore use the U–Pb age spectra (Fig. 12) to reveal crustal formation and magmatic events in the source region.
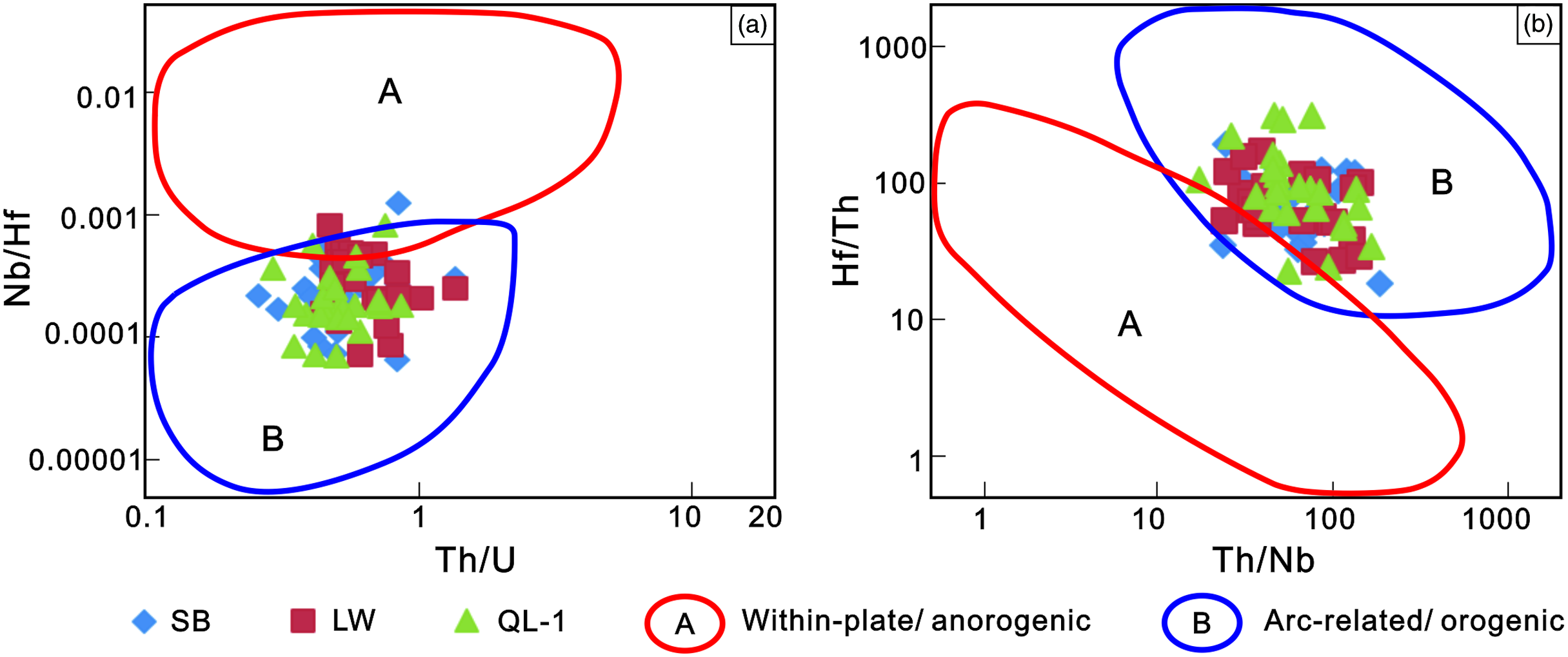
Fig. 12. Magmatic zircon trace element discrimination diagrams Th/U–Nb/Hf and Th/Nb–Hf/Th (after Yang et al. Reference Yang, Cawood, Du, Huang, Huang and Tao2012).
The zircon concordant U–Pb age spectra for 433 representative detrital zircons from the Lower Shihezi Formation in the northern Ordos Basin reveal five distinct populations (Fig. 10; Fig 14, further below): (1) c. 2600–2400 Ma; (2) c. 2000–1800 Ma; (3) c. 500–400 Ma; (4) c. 340–320 Ma; and (5) c. 310–290 Ma.

Fig. 13. Tectonic setting discrimination diagrams for zircon grains from the Late Palaeozoic in the northern Ordos Basin (after Carley et al. Reference Carley, Miller, Wooden, Padilla, Sohmitt, Economos, Bindeman and Jordan2014).
The oldest age population at 2600–2400 Ma corresponds to the tectonic thermal events of the Yinshan orogenic block and Alxa block in the NCC during the Neoarchaean–Palaeoproterozoic interval (Wan et al. Reference Wan, Liu, Dong, Xu, Wang, Wilde, Liu, Yang and Zhou2009; Jian et al. Reference Jian, Kröner, Windley, Zhang, Zhang and Zhang2012; Gong et al., Reference Gong, Zhang, Wang, Yu, Li and Li2016). The formation ages of the original rocks in the Yinshan block, including the greenstone belts, granitic intrusions and high-grade metamorphic complexes, are mainly 2560 to 2510 Ma, at the end of the Neoarchaean period (Zhang et al. Reference Zhang, Gou, Diwu, Liu, Zhao and Hu2018). All samples in the study area show this age peak.
The second age population at c. 2000–1800 Ma is found in all sections. The discovery of two ancient Himalayan-type collisional orogenic belts (c. 1950 Ma Khondalite Belt in the western part of the NCC and c. 1850 Ma Trans-North China Orogen in the central part) confirmed that the basement of the NCC resulted from the amalgamation of several microcontinental blocks at c. 1950 Ma and c. 1850 Ma, recording assembly of the Columbia supercontinent (Wilde et al. Reference Wilde, Zhao and Sun2002; Wan et al. Reference Wan, Liu, Dong, Xu, Wang, Wilde, Liu, Yang and Zhou2009; Yin et al. Reference Yin, Zhao, Guo, Sun, Xia, Zhou and Liu2011; Peng et al. Reference Peng, Guo, Windley, Liu, Chu and Zhai2012; Li et al. Reference Li, Zhao and Sun2016; Wang et al. Reference Wang, Guo, Yin and Peng2017).
The age population in the range of the Neoproterozoic (1000 to 800 Ma) is only found in samples QL-1, QL-2 and SB. This age group is absent in the NCC, but there are a large number of magmatic records in the Alxa block at that time (Fig. 14, further below; Zhao et al. Reference Zhao, Liu, Wang, Zhao, Wang and Zhang2016).
The age population at c. 500–400 Ma is found only in the Qianlishan and Shabatai sections, the two western samples. This population represents magmatic activity in the initial stage of the Caledonian Movement (400–500 Ma) and is best explained as being related to the subduction of Qilian towards the Alxa block (Wei et al. Reference Wei, Hao, Lu, Zhao, Zhao and Shi2013; Duan et al. Reference Duan, Li, Qian and Jiao2015; Zhang et al. Reference Zhang, Zhang, Zhang, Xiong, Luo, Yang, Pan, Zhou, Xu and Guo2017). It is likely that the Alxa block was the main source of the Lower Shihezi Formation sediments in the Qianlishan and Shabatai sections (Fig. 12). Samples from the eastern sections conspicuously lack the age peak at 440 Ma, indicating that the Alxa block was not a source for sediments in the eastern sections.
The c. 340–320 Ma age population is found only in the Qianlishan and Shabatai sections. The Carboniferous (350–320 Ma) magmatic rocks of the Longshou Mountains, Beida Mountains and Bayanwula Mountains and the Honggueryulin and Langshan areas in the Alxa block mainly include intermediate-acid adakitic intrusive rocks and a few mafic rocks. The c. 340–320 Ma detrital zircons in the Lower Shihezi Formation in the western Ordos Basin were likely mainly derived from the Alxa block.
The detrital zircon age population at 290–310 Ma is comparable to the emplacement age of many Carboniferous igneous rocks widely exposed in the Yinshan orogenic belt and in the CAOB (Fig. 2). The discovery of hornblende gabbro–diorite–granodiorite–granite plutons in the northern margin of the NCC of Late Carboniferous to Early Permian age (SH Zhang et al. Reference Zhang, Zhao, Kröner, Liu, Xie and Chen2009b) supports the Yinshan orogenic belt as a potential source for the 290–310 Ma population of detrital zircon in the northern Ordos Basin. The CAOB contains almost no Archaean or Proterozoic zircons (Fig. 12), which contrasts with the zircon age spectra of the study area. However, almost all the Late Palaeozoic zircons of the region display negative ϵHf(t) values ranging from −2.0 to −27.8, indicating that the magmatic source was derived from crustal reworking. In contrast, the Carboniferous–Permian sediments in the CAOB show mainly positive ϵHf(t) values ranging between −4.2 and +16.3 (Li et al. Reference Li, Chen, Wang, Hou and Liu2011; Su et al. Reference Su, Qin, Sakyi, Li, Yang, Sun, Tang, Liu, Xiao and Malaviarachchi2011; R Zhou et al. Reference Zhou, Liu, Zhou and Zou2019), making it unlikely that the CAOB is the source of zircons analysed in this work.
According to the results of petrology and palaeocurrent analysis, the sediments in the western sections of the Lower Shihezi Formation were probably mainly derived from the Yinshan orogenic belt and the Alxa block, and those in the eastern section were mainly derived from the Yinshan orogenic belt.
5.2 Implication for the geological environment
A large number of Carboniferous–Permian intrusive rocks, including diorite, quartz diorite, granite diorite and granite (SH Zhang et al. Reference Zhang, Zhao, Song, Hu, Liu, Yang, Chen, Liu and Liu2009a; Bai et al. Reference Bai, Liu, Wang, Yang and Li2013), developed in the northern margin of NCC. The sandstone in the study area contains volcanic materials and anorthite (Fig. 6), and anorthosite can be used as a landmark mineral in the active continental margin (Beard, Reference Beard1986; Wilson, Reference Wilson1989; Zhou et al. Reference Zhou, Xu, Dong and Li1994). Petrological results show that magmatic activities related to subduction of the active continental margin may have developed in the northern margin of NCC (SH Zhang et al. Reference Zhang, Zhao, Kröner, Liu, Xie and Chen2009b).
Zircons record the evolution of magma, and both crystallization environment and tectonic setting can be fingerprinted using the trace element compositions of zircons (Belousova et al. Reference Belousova, Griffin, O’Reilly and Fisher2002; Grimes et al. Reference Grimes, John, Kelemen, Mandab, Wooden, Cheadle, Hanghøj and Schwartz2007). On zircon trace element discrimination diagrams (Fig. 13), the Late Palaeozoic detrital zircons from the northern Ordos Basin mainly plot in the arc-related/orogenic fields. The studied detrital zircons with Late Palaeozoic ages present trace element geochemistry features comparable to zircons crystallized in an arc/orogenic environment (Yang et al. Reference Yang, Cawood, Du, Huang, Huang and Tao2012).

Fig. 14. Distribution of crystalline rocks in surrounding tectonic units, showing primary potential sources for detrital zircons in the northern Ordos Basin. The age ranges of the magmatic rocks in the middle section of Yinshan Orogenic Belt are from Zhang (Reference Zhang2004), Jian et al. (Reference Jian, Zhang, Liu, Jin, Jia and Qian2005); Wu et al. (Reference Wu, Sun, Li, Zhao and Xia2006), Zhong et al. (Reference Zhong, Deng, Wan, Mao and Li2007), CY Dong et al. (Reference Dong, Liu, Wan, Xu, Liu and Yang2009), C Zhang et al. (Reference Zhang, Han, Liu, Ji, Zhao and Zhang2009c), Luo et al. (Reference Luo, Wu and Zhao2010), XJ Dong et al. (Reference Dong, Xu, Liu and Sha2012a, b), L Liu et al. (Reference Liu, Zhang, Dai, Wang and Li2012), MZ Ma et al. (Reference Ma, Wan, Xu, Liu, Xie, Dong and Liu2012, Reference Ma, Xu, Zhang, Dong, Dong, Liu, Liu and Wan2013), SJ Liu et al. (Reference Liu, Dong, Xu, Santosh, Ma, Xie, Liu and Wan2013), Wan et al. (Reference Wan, Xu, Dong, Nutman, Ma, Xie, Liu, Liu, Wang and Cu2013), Mo et al. (Reference Mo, Guo, Tong, Wang, Liu and Li2014), PH Liu et al. (Reference Liu, Liu, Cai, Yang, Wang, Liu, Liu and Shi2016b, Reference Liu, Liu, Cai, Liu, Liu, Wang, Xiao and Shi2017b), XD Ma et al. (Reference Ma, Zhong and Santosh2018), Li et al. (Reference Li, Li, Wang and Wen2019), Wang et al. (Reference Wang, Dong, Xu, Ren, Wang and Chen2019) and H Zhou et al. (Reference Zhou, Liu, Zhou and Zou2019). Those for the Alxa block are from ZH Li (Reference Li2018 and references therein). Those for the Permo-Carboniferous sandstone in the southeastern Central Asian Orogenic Belt are from Chen et al. (Reference Chen, Zhang, Li, Yu and Wu2016), and data from Qianlishan, Shabatai and Longwanggou are from this study.
To obtain more insight into the geological environment, the Late Palaeozoic detrital zircons from the studied sandstones are plotted in the tectonic background discrimination diagrams for zircon trace elements (Fig. 14) proposed by Carley et al. (Reference Carley, Miller, Wooden, Padilla, Sohmitt, Economos, Bindeman and Jordan2014). All samples from the four studied sections plot in the continental arc field. Furthermore, according to the analysis of U–Pb ages of detrital zircons and rock composition of all potential source areas, it is judged that these Late Palaeozoic detrital zircons come from the orogenic belt in the north of the study area. This indicates that the northern Ordos Basin was adjacent to the continuous continental arc orogenic belt in the Late Palaeozoic (Xiao et al. Reference Xiao, Windley, Huang, Han, Yuan, Chen, Sun, Sun and Li2009; SH Zhang et al. Reference Zhang, Zhao, Kröner, Liu, Xie and Chen2009b; Wilhem et al. Reference Wilhem, Windley and Stampfli2012; Zhang et al. Reference Zhang, Zhao, Liu and Hu2016).
5.3 Time constraints for closing of the Palaeo-Asian Ocean to the north of the Ordos Basin
Previous studies have shown that a large-scale magmatic rock belt distributed in the east–west arc of the Late Palaeozoic was developed near the CAOB, including the North Yili magmatic arc (Zhang et al. Reference Zhang, Lv, Liu, Chen, Chen, Gao and Liu2013), the Yabulaishan–Bayinnuoer Gong–Honggulyulin magmatic belt (Gong et al. Reference Gong, Zhang, Wang, Yu and Wang2018) in northern Alxa, the Bainaimiao magmatic arc ( Xiao et al. Reference Xiao, Liu, Zhang, Lin and Zhang2019) in southern Inner Mongolia, the Inner Mongolia palaeo-uplift (SH Zhang et al. Reference Zhang, Zhao, Kröner, Liu, Xie and Chen2009b) and many more. These magmatic belts show the characteristics of multi-stage eruption, and have bimodal volcanic rocks and corresponding plutonic rocks (Tang, Reference Tang1992).
The geochemical characteristics of volcanic rocks and calc-alkaline rocks widely developed in the Hercynian CAOB reveal that they are mainly related to the continuous subduction of the Late Palaeozoic Palaeo-Asian Ocean (Li et al. Reference Li, Gao, Guan and Li2009; Xiao et al. Reference Xiao, Windley, Huang, Han, Yuan, Chen, Sun, Sun and Li2009; SH Zhang et al. Reference Zhang, Zhao, Kröner, Liu, Xie and Chen2009b, Reference Zhang, Zhao, Liu and Hu2016; Jian et al. Reference Jian, Liu, Kroner, Windley, Shi, Zhang, Zhang, Miao, Zhang and Tomurhuu2010). The Carboniferous magmatic rocks of the Alxa block were produced by partial melting of the thickened lower crust and are possibly the products of remelted Archaean–Palaeoproterozoic continental crust materials (M Liu et al. Reference Liu, Zhang, Xiong, Zhao, Di, Wang and Zhou2016a; Xue et al. Reference Xue, Ling, Liu, Zhang and Sun2017). Multi-stage magmatic materials reflect the fact that the crust/mantle was in an active state during the suture process between the NCC and the Siberian plate. This also shows that strong interaction and deep material circulation occur between different strata and different blocks.
The Palaeo-Asian Ocean experienced a long-term and complex closure process during the Palaeozoic. The Permian is a critical period for the debate on the closure of the Palaeo-Asian Ocean (Xiao et al. Reference Xiao, Windley, Hao and Zhai2003; JY Li, Reference Li2006; PW Li et al. Reference Li, Gao, Guan and Li2006, Reference Li, Gao, Guan and Li2009; Windley et al. Reference Windley, Alexeiev, Xiao, Kröner and Badarch2007; Tong et al. Reference Tong, Hong, Wang, Shi, Zhang and Zeng2010; Wilhem et al. Reference Wilhem, Windley and Stampfli2012). The Ordos Basin is located to the south of the middle part of the Palaeo-Asian Ocean. The sedimentary rocks in the north of the Ordos Basin are directly affected by the closure of the Palaeo-Asian Ocean (Windley et al. Reference Windley, Alexeiev, Xiao, Kröner and Badarch2007; Xiao et al. Reference Xiao, Windley, Sun, Li, Huang, Han, Yuan, Sun and Chen2015; Zhou et al. Reference Zhou, Jiao and Zhao2017; Chen, Reference Chen2020). Therefore, study of the distribution characteristics in provenance of the northern Ordos Basin during the Permian helps to reveal the spatial variability in the closure process of the Palaeo-Asian Ocean. The Late Palaeozoic age peaks of the western sections are c. 300 Ma and c. 325 Ma, respectively (Fig. 15). However, in the eastern sections, except for sample 3 (Liu et al. Reference Liu, Wang, Shi, Zhang, Qin, Qi and Xu2020), there is only one Late Palaeozoic age peak (c. 300 Ma). It may be inferred that sample 3 was jointly affected by the eastern and western provenances. This indicates that there were two main magmatic periods in the west and one in the east, reflecting temporal differences in the main closure of the Palaeo-Asian Ocean in each section. The main collision period in the west began in the Late Carboniferous, and the main collision period in the east began in the Early Permian. Therefore, the Palaeo-Asian Ocean was not completely closed before the Early Permian. This also indicates that the collision and closure of the Palaeo-Asian Ocean occurred earlier in the west than in the east, consistent with the previous viewpoint that the closure of the Palaeo-Asian Ocean occurred from west to east (Yang et al. Reference Yang, Guo, Song, Li and He2015; Zhang et al. Reference Zhang, Zhao, Liu and Hu2016; Q Liu et al. Reference Liu, Zhao, Han, Eizenhöfer, Zhu, Hou, Zhang and Wang2017).

Fig. 15. Diagram showing age distributions of detrital zircons from the Late Palaeozoic in the northern Ordos Basin.
Moreover, along the fault zone marked by the Wendur Temple North-Xilamulun River (Fig. 1), the flora from early Carboniferous to early Permian times reflects flora from the Siberian craton and NCC (Li et al. Reference Li, Gao, Guan and Li2009), but in the late Permian the Angara flora and Cathaysian flora mixed, forming an integrated flora belt near the docking zone. This indicates that the Siberian craton and NCC were joined during the early Permian to the late Permian (Huang & Ding, Reference Huang and Ding1998; Shi & Archbold, Reference Shi and Archbold1998; Li et al. Reference Li, Gao, Guan and Li2009). The palaeontological evidence is in good agreement with the tectonic evolution indicated by zircon ages.
To sum up, the Late Palaeozoic tectonic evolution in the northern Ordos Basin was closely related to the southward subduction and closure of the Palaeo-Asian Ocean. During the Late Carboniferous, the Palaeo-Asian Ocean began to subduct under the NCC, and gradually closed, with subduction moving from west to east. Closure of the part of the Palaeo-Asian Ocean adjacent to the north of the Ordos Basin could not have occurred earlier than the Early Permian.
6. Conclusions
Large amounts of chronological data of detrital zircons from the Lower Shihezi Formation sedimentary rocks have been presented to study the provenance of the Lower Shihezi Formation in the northern Ordos Basin. The sedimentary domain of the study area can be divided into eastern and western parts. Sediments in the western region were primarily derived from the Yinshan orogenic belt and the Alxa block; those in the eastern region, however, were primarily derived from the Yinshan orogenic belt. The magmatic periods in the western and eastern regions are different in the Late Palaeozoic, reflecting the closure process of the Palaeo-Asian Ocean. The Palaeo-Asian Ocean began to subduct under the NCC in the Late Carboniferous and gradually closed from west to east thereafter.
Acknowledgements
The work was supported by the National Major Science and Technology Projects of China (Grant No. 2016ZX05050005). Part of this research was undertaken using the TESCAN integrated mineral analyzer (TIMA) (ARC LE140100150) at the John de Laeter Centre, Curtin University, Western Australia, and we thank Alexander Prent for assistance with analysis. Analysis in the JdLC GeoHistory laser ablation Facility was enabled by the Research Office, Curtin, AuScope (auscope.org.au) and the Australian Government via the National Collaborative Research Infrastructure Strategy (NCRIS). Brad McDonald is thanked for help with LA-ICP-MS set-up.
CRediT authorship contribution statement
Rong Chen: Conceptualization, Formal analysis, Investigation, Writing – original draft. Feng Wang: Conceptualization, Funding acquisition, Writing – review & editing, Supervision. Zhen Li: Writing – review & editing. Noreen J. Evans: Writing – review & editing. Hongde Chen: Resources, Project administration.