1. Introduction
It is well documented that stromatolites flourished during the Proterozoic Eon, when they occupied a variety of subtidal, intertidal, supratidal and lacustrine environments and developed a range of shapes including branched, conical, columnar and domal (Awramik & Sprinkle, Reference Awramik and Sprinkle1999). Proterozoic stromatolites are a major feature of shallow-marine carbonate platforms (Walter et al. Reference Walter, Grotzinger, Schopf, Schopf and Klein1992; Grotzinger & James, Reference Grotzinger and James2000; Semikhatov & Raaben, Reference Semikhatov, Raaben, Riding and Awramik2000). An assessment of the evolution of Proterozoic carbonate platforms from a much broader perspective and in a more generalized way is a challenge, but it has been attempted on the basis of the morphology of in situ stromatolites (Grotzinger & Knoll, Reference Grotzinger and Knoll1999; Kah et al. Reference Kah, Bartley, Frank and Lyons2006, Reference Kah, Bartley, Stagner, Swart, Eberli and McKenzie2009; Bartley et al. Reference Bartley, Kah, Frank and Lyons2015). Importantly, petrographic, geochemical and sedimentological analyses of Proterozoic stromatolites, exhibiting a wide variety of shapes and microstructures, yield valuable information about palaeobathymetry, sediment source and the nature of their lithification (e.g. Kamber et al. Reference Kamber, Bolhar and Webb2004, Reference Kamber, Webb and Gallagher2014; Corkeron et al. Reference Corkeron, Webb, Moulds and Grey2012; Tosti & Riding, Reference Tosti and Riding2017 a, b; Viehmann et al. Reference Viehmann, Hohl, Kraemer, Bau, Walde, Galer, Jiang and Meister2019). However, growth patterns of Precambrian stromatolites, their mechanisms of development and bearing on the environment through space and time are still much debated.
Particularly intriguing are inclined or sinuous forms of columnar stromatolite. Slightly curved columns are not rare (Cloud & Semikhatov, Reference Cloud and Semikhatov1969); their morphology has been attributed to wave and current action (e.g. Hofmann, Reference Hofmann1973; Eagan & Liddell, Reference Eagan, Liddell, Brett and Baird1997; Tosti & Riding, Reference Tosti and Riding2017 a) and they have been used as an indicator of current direction (e.g. Logan et al. Reference Logan, Hoffman and Gebelein1974; Cecile & Campbell, Reference Cecile and Campbell1978; Southgate, Reference Southgate1989). However, the origin of stromatolites that are sinusoidal in vertical section is more problematic. This also includes their primary chemistry (aragonitic or calcitic), since the majority of Proterozoic sinuous stromatolites are essentially dolomitic (Fenton & Fenton, Reference Fenton and Fenton1937; Horodyski, Reference Horodyski1977; Tosti & Riding, Reference Tosti and Riding2017 a) and their original carbonate composition has rarely been addressed. Importantly, decoding stromatolite carbonate mineralogy and evaluating textures preserved and diagenetic changes (silicification and dolomitization in particular) using various petrographic techniques, are essential steps in understanding seawater chemistry during stromatolite development. If primary textures are not lost, they may preserve information about biogenic or abiogenic processes involved in stromatolite development and the timing of carbonate precipitation (e.g. Bartley et al. Reference Bartley, Knoll, Grotzinger and Sergeev2000; Riding, Reference Riding2008).
Growth of the Chandi stromatolites began during the early phase of platform development, and they characteristically show a sine-wave growth pattern in the lower part of the succession. The directional growth pattern, which is typical of other Precambrian stromatolites, can be a response to a variety of factors, for example, heliotropism in sinuous stromatolites with large angles of curvature typically > 130° (Nordeng, Reference Nordeng and Munyan1963; Vanyo & Awramik, Reference Vanyo and Awramik1982, Reference Vanyo and Awramik1985; Qu et al. Reference Qu, Xie and Gong2004) or chemotropism (Greinert et al. Reference Greinert, Bohrmann and Elvert2002). However, our studies of the morphology of these stromatolites provide an understanding of the mechanisms of growth of the stromatolites and their palaeobathymetry, thus contributing to the knowledge of Proterozoic shallow-marine carbonate sediments.
2. Geological setting
Peninsular India preserves an extensive record of Proterozoic successions within several intracratonic basins (Fig. 1a). The Chattisgarh Basin, a depocentre mostly during the Mesoproterozoic Era with slight extension into the Neoproterozoic Era, covers an area of c. 36 000 km2 within the Bastar craton (Naqvi & Rogers, Reference Naqvi and Rogers1987; Chaudhuri et al. Reference Chaudhuri, Saha, Deb, Patranabis Deb, Kanti Mukherjee and Ghosh2002). It hosts a c. 2300-m-thick succession of unmetamorphosed and mildly deformed sedimentary rocks, which rest on the Archaean–Palaeoproterozoic basement with a major angular unconformity. The succession has been designated the Chattisgarh Supergroup (Murti, Reference Murti and Radhakrishna1987; Das et al. Reference Das, Kundu, Das, Dutta, Kumaran, Ramamurthy, Thangavelu and Rajaiya1992).

Fig. 1. (a) Distribution of the Proterozoic cratonic basins in peninsular India; Bh – Bhima; C – Cuddapah; Ch – Chattisgarh; K – Kaladgi; Kh – Khariar; I – Indravati; V – Vindhyan. (b) Map of the western part of the Chattisgarh Basin (modified after Das et al. Reference Das, Kundu, Das, Dutta, Kumaran, Ramamurthy, Thangavelu and Rajaiya1992) showing disposition of the various units of the Raipur and Chandarpur groups of the Hirri sub-basin, and the location of Deodongar (21° 13ʼ 2.3ʼʼ N, 81° 04ʼ 29.6ʼʼ E), where the sinuous stromatolites of the Chandi Formation occur in a quarry section. Radiometric dates (red stars) after Patranabis-Deb et al. (Reference Patranabis-Deb, Bickford, Hill, Chaudhuri and Basu2007), Das et al. (Reference Das, Yokoyama, Chakraborty and Sarkar2009) and Bickford et al. (Reference Bickford, Basu, Mukherjee, Hietpas, Schieber, Patranabis-Deb, Kumar Ray, Guhey, Bhattacharya and Dhang2011a, b).
The Chattisgarh Supergroup comprises sandstone, shale, limestone with minor conglomerate, and tuff, and was classified initially into two groups: in stratigraphic order, the Chandarpur Group and the Raipur Group (Fig. 1) (Dutt, Reference Dutt1964; Murti, Reference Murti and Radhakrishna1987). Das et al. (Reference Das, Kundu, Das, Dutta, Kumaran, Ramamurthy, Thangavelu and Rajaiya1992) considered the easternmost part of the Chattisgarh Basin as a sub-basin and designated it as the Baradwar sub-basin. They defined three groups in this sub-basin: the middle and upper groups correspond to the Chandarpur Group and the Raipur Group, respectively, and the basal group is the Singhora Group. Das et al. (Reference Das, Kundu, Das, Dutta, Kumaran, Ramamurthy, Thangavelu and Rajaiya1992) proposed an unconformity between the Singhora Group and the overlying Chandarpur Group, but they considered that the Raipur Group gradationally overlies the Chandarpur Group. Later, while studying the eastern part of the basin, Patranabis-Deb & Chaudhuri (Reference Patranabis-Deb and Chaudhuri2008) proposed a fourfold classification, where the youngest Kharsiya Group unconformably overlies the Raipur Group. The Neoproterozoic Kharsiya Group is correlated with the Hirri sub-basin deposits (Bickford et al. Reference Bickford, Basu, Mukherjee, Hietpas, Schieber, Patranabis-Deb, Kumar Ray, Guhey, Bhattacharya and Dhang2011 a). The Raipur Group, having a maximum thickness of c. 1500 m, consists of two successive cycles of shale-to-limestone units of formation status, with a few interbedded sandstone members (Fig. 1). The Charmuria Formation, the lowermost unit of this group, represents a non-stromatolitic, micritic carbonate platform, which passes up into the thick shale/calcareous–shale unit of the Gunderdehi Formation. Stromatolites appear as small bioherms in the upper part of the section, gradationally passing up to the Chandi Formation. This formation comprises brown and greenish-grey cement-grade limestone with prolific growth of stromatolites, minor shale and a few sandstone beds (Figs 1, 2). This formation is particularly well exposed near Deodongar (where the samples were collected; 21° 13ʼ 2.3ʼʼ N, 81° 04ʼ 29.6ʼʼ E) and Dhaneli, Hasda, Achholi and Kalkasa village quarry sections. The present study focuses on the Deodongar section, where stromatolites are mostly columnar with curved axes described here as sinusoidal stromatolites (sensu Tosti & Riding, Reference Tosti and Riding2017 a).

Fig. 2. (a) Idealized stratigraphy of the Chandi Formation. Thick blue line at the bottom right schematically represents a portion of the studied profile. (b) Detailed stratigraphic log of the Chandi limestone section near the Deodongar village quarry. Sinuous and inclined stromatolites comprise a major part of the studied profile. Note the transition from inclined, branching and non-branching stromatolites in the lower part to a branching type in the upper part with increased marly layers (2–5 cm thick) forming a cyclic pattern. These marly layers are observed in the entire profile, but they are more distinct in the upper part. Scale: hammer, 28 cm; coin, 2 cm.
In general, the Chattisgarh Basin consists of gently dipping strata (2–10°), except in the vicinity of large faults where the dips vary over the range 20–25°. These large-scale faults are assumed to be the rejuvenated basin-forming faults (Chaudhuri et al. Reference Chaudhuri, Saha, Deb, Patranabis Deb, Kanti Mukherjee and Ghosh2002). Mafic dykes and sills intruded the succession, commonly showing a preferred orientation parallel to the faults. The Chattisgarh Supergroup unconformably overlies the Archaean granite–gneiss complex of the Bastar craton with slivers of greenstone belt, namely the Dongargarh-Kotri and Sonakhan belts.
2.a. Age of the Chattisgarh Basin
It is a daunting task to obtain precise ages from pre-fossiliferous Precambrian rocks, devoid of or with few synsedimentary volcanic units. Our data depend mostly on the relative age of the sedimentary package. On the basis of stromatolite assemblages of the Chandi Formation, Moitra (Reference Moitra2003) proposed a late Neoproterozoic age for the Raipur Group. From the discovery of various families of acritarchs and benthic cyanobacteria from the upper Raipur Group, Singh & Babu (Reference Singh and Babu2013) interpreted a Neoproterozoic age of the basin. Based on the presence of the microfossil Leiosphaeridia, Singh & Sharma (Reference Singh and Sharma2016) proposed the Chandarpur Group to be Meso-Neoproterozoic. However, recent geochronological data from the Singhora welded tuffs associated with the lowermost part of the Chandarpur Group suggest that the Chattisgarh Basin formed at c. 1405 ± 9 Ma (Bickford et al. Reference Bickford, Basu, Patranabis-Deb, Dhang and Schieber2011 b). Das et al. (Reference Das, Das, Chakraborty and Balakrishnan2011) also reported an age of 1421 ± 23 Ma for the basin-opening phase. U–Pb SHRIMP zircon ages of the Sukhda and Dhamda tuffs from the top of the Raipur Group yielded 207Pb – 206Pb ages in the range of 990–1020 Ma and 993 ± 8 Ma, respectively (Patranabis-Deb et al. Reference Patranabis-Deb, Bickford, Hill, Chaudhuri and Basu2007; Bickford et al. Reference Bickford, Basu, Mukherjee, Hietpas, Schieber, Patranabis-Deb, Kumar Ray, Guhey, Bhattacharya and Dhang2011 a). Detrital zircons analysed from the Sarnadih Sandstone, which forms part of the Kharsiya Group (Fig. 1), yielded a range of U–Pb ages varying from 1006 Ma to 2495 Ma, indicating a minimum age of c. 1006 Ma for the formation (Bickford et al. Reference Bickford, Basu, Mukherjee, Hietpas, Schieber, Patranabis-Deb, Kumar Ray, Guhey, Bhattacharya and Dhang2011 a). These geochronological data established that a major part of the Chattisgarh succession was deposited during the Mesoproterozoic Era and the latest part of the Neoproterozoic Era. On the basis of carbon isotope analyses, George et al. (Reference George, Ray and Kumar2019) recently proposed a Mesoproterozoic age for the Charmuria and Chandi carbonate formations of the Raipur Group, since the carbon isotope chemostratigraphy is consistent with a Mesoproterozoic age.
3. Methods
3.a. Sample collection
Two representative specimens of sinuous stromatolitic limestone belonging to the Chandi Formation, S1 and S2, were collected from an open pit limestone quarry. Heights of S1 and S2 are 43 cm and 112 cm, respectively. For petrography and chemical analysis, five samples from S1 (1A–1E) were collected at 4, 12, 21, 29 and 43 cm levels, while eight samples were collected from S2 (2A1, 2A2, 2B1, 2B2, 2C1, 2C2, 2D1 and 2D2) from an interval of 28 cm from the top and bottom (Fig. 3a).
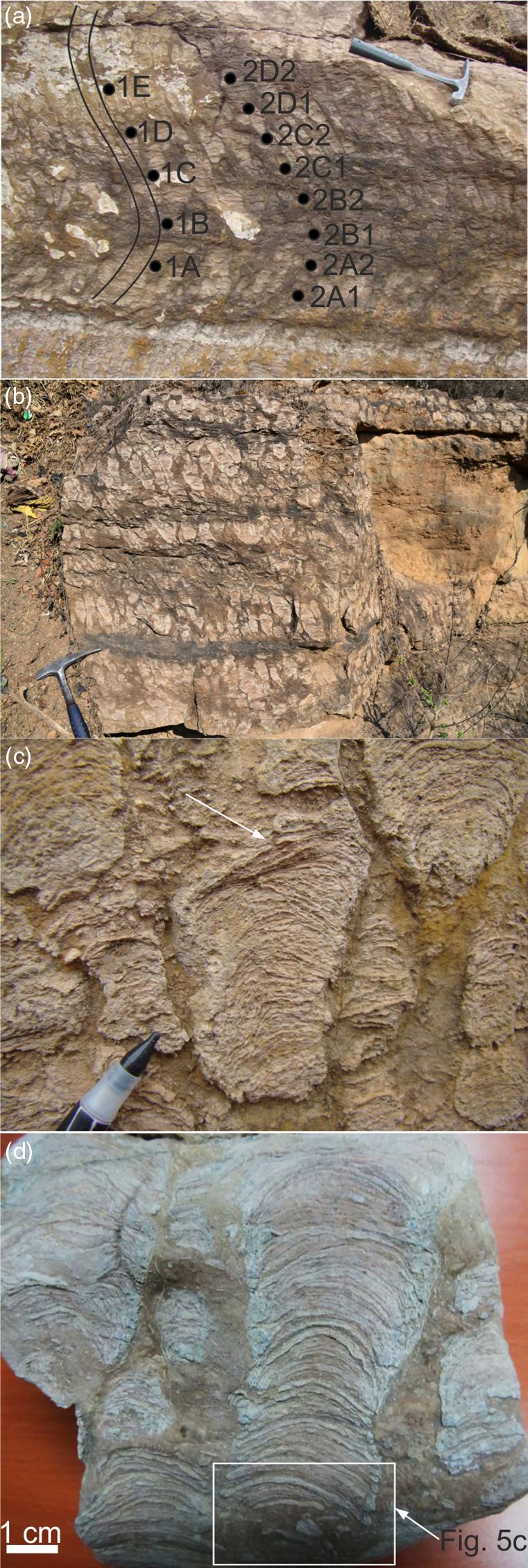
Fig. 3. Chandi Formation stromatolites in near-vertical sections, perpendicular to the bedding plane: (a) sinuous non-branching stromatolites (hammer, 28 cm), where 1A–E and 2A–D are sampling points and the curves to the left of the numbers 1A–E show the pattern of the sinuous stromatolites; (b) non-branching stromatolitic biostromes with thin (2–5 cm) marl layers (hammer, 28 cm); (c) inclined non-branching stromatolites showing convex-up laminae; arrow points to the truncation between lamina sets within a stromatolite column (pen tip, 2 cm); (d) columnar stromatolite with regular convex-up laminae disrupted in the lower part (box).
3.b. Petrography and scanning electron microscopy with energy-dispersive spectroscopy
Thin-sections of sinuous stromatolite samples were examined under the petrographic microscope and scanning electron microscope (SEM) FE-SIGMA VP (Carl Zeiss Microscopy GmbH) with an energy-dispersive spectrometer (EDS) (Quantax XFlash 3|10, Bruker Nano GmbH). Thin-sections were placed on the mount with carbon conductive tape. Samples were coated with a 20 nm layer of carbon by a vacuum coater (Quorum 150T ES). Carbon tape bridges were made for each sample to avoid excessive accumulation of charge. Analyses were performed with 120 μm aperture and 15 keV acceleration voltage. Beam intensity was 2.5 nA and working distance was 7.5 mm. In order to detect any microbial remnants, before SEM imaging samples were etched with 5% HCl, cleaned with alcohol and coated with chromium.
3.c. Cathodoluminescence
Cathodoluminescence (CL) petrography of selected sinuous stromatolite samples was performed using a Technosyn Mark II instrument at Durham University. The voltage was held at 12–15 kV, current at 480–570 μA and chamber vacuum at 50–60 mTorr.
3.d. Major and trace elements
Thirteen selected stromatolitic limestone whole-rock samples from S1 and S2 were powdered for bulk major, trace and rare earth element (REE) analyses at the Activation Laboratories Ltd, Canada. For major oxide detection, X-ray fluorescence (XRF) was used on whole-rock powdered samples fused into glass discs using lithium metaborate/lithium tetraborate flux; for XRF trace-element analysis, pressed powder pellets were made. Multi-acid digestion, sodium peroxide fusion and lithium metaborate/lithium tetraborate fusion were used for the whole-rock REE analysis. REE analysis was performed using an inductively coupled plasma mass spectrometer (ICP-MS; PerkinElmer SCIEX ELAN DRC-e). Concentrations of REE + Y (n = 13) were normalized to the Post-Archaean Australian Shale (PAAS), representing an estimate for the composition of average terrigenous input to the oceanic environment. Specifically, REE fractionation was calculated as PrSN/YbSN (where ‘SN’ indicates shale normalized) to avoid problems in case of anomalous La and Ce concentrations. To avoid any anomalous behaviour of La, Ce, Eu and Gd, the anomalies were calculated using the geometric equations of Lawrence & Kamber (Reference Lawrence and Kamber2006) and are given as Ce/Ce*, Eu/Eu*, Gd/Gd* and La/La*. Chemical composition of the analysed samples is provided in online Supplementary Tables S1 and S2 (available at http://journals.cambridge.org/geo).
4. Results
4.a. Morphology of the stromatolites at outcrop
Stromatolites of the Raipur Group first appear as small isolated lenticular bodies in the upper part of the Gunderdehi Shale that gradationally passes up to the stromatolitic carbonate platform, the Chandi Formation (Fig. 1; Patranabis-Deb et al. Reference Patranabis-Deb, Słowakiewicz, Tucker, Pancost and Bhattacharya2016). In the lower part of the studied section near Deodongar village, the stromatolites appear as tabular beds (20–50 cm in thickness) forming biostromes. These biostromes form laterally persistent units, which can be traced for a few metres (Fig. 3). Higher up in the section the microbial deposits change their pattern and form bioherms, which range in size from 3–5 m (Figs 2, 4) to more than 20 m in length. Small bioherms consisting of a single build-up or mound normally range over 60–80 cm in height. Larger bioherms, on the other hand, may be more than 4–5 m high. Larger bioherms are composites of smaller mounds, that is, each is separated from the adjoining bioherm by micrite or mixed siliciclastic–carbonate (Fig. 2). Small individual mounds or larger composite bodies consist of columnar stromatolites, which in places are all elongate in shape and in cross-section have a very persistent direction of elongation (Fig. 4a).

Fig. 4. Examples of Chandi Formation biohermal stromatolites: (a) an individual columnar stromatolitic mound viewed from above; (b) a close-up of non-branching, columnar, inclined stromatolites; (c) columnar stromatolites; (d) example of columnar stromatolites showing spacing between the columns filled with argillaceous micrite/lime mud. Scale: hammer, 28 cm; pen, 15 cm.
Stromatolites within bioherms and biostromes are composed of branched or non-branched stromatolite columns with a straight (Fig. 4c) or sinusoidal geometry (Fig. 3a–d). Elongate stromatolites are commonly closely associated with columnar stromatolites (Fig. 4c). Non-branching columnar stromatolites occur as parallel to sub-parallel, circular to slightly elongate, columns with a constant diameter from the base to the top of the structures (Fig. 4b). In plan-view, the structures are circular to slightly elongate with concentric laminae, with diameters ranging from 0.5 to 2 cm in general although they may reach 30 cm in the elongate type (Fig. 4a). The size of the columns, both diameter as well as height, varies considerably between adjacent colonies, although the column size remains constant within a single colony. Spacing of the columns also varies considerably between different colonies, and the inter-columnar areas are filled with argillaceous lime mud (Fig. 4a–d). Slightly inverted columns, cone-like with a narrow base and wide top, are also seen, with heights from 1–3 cm to about a metre (Fig. 3c, d). They are unwalled and show very low synoptic relief. Column margins are smooth, although not enveloped. The laminae within each stromatolite column are convex-upwards with variable curvature and synoptic relief. In branching forms, the convex-upwards laminae start to develop two subtle diverging humps from which the bifurcation begins. These humps provided a short-wavelength roughness that acted as substrates for individual stromatolite branches. The angle between two branches is always acute and varies over 5–45°. However, in some cases a few of the branches lean over and occur nearly parallel to the bedding plane. In steeply sloping or nearly bedding-parallel branches, the laminae become subvertical; as a result, one side of the lamina slopes more steeply than the other. Branched morphologies vary in the geometry of individual branched laminae, relief and the degree of branch divergence from the underlying stromatolite. The type of branching can be described either as expanding, radiate or multi-branching (Cloud & Semikhatov, Reference Cloud and Semikhatov1969). The growth of the branching and non-branching stromatolites is locally truncated by an erosive surface, as identified by laminae being truncated and a change in stromatolite morphology (Fig. 3c). As mentioned earlier, the stromatolite columns vary in shape; some have a narrow base and wider top, some have a broad base and narrow top, whereas others have a constant column width.
The bioherms develop a bedded appearance where column height is constant and successive stromatolite beds are separated by mudstone (Fig. 4d). Elongate stromatolites are unlinked to partially linked, with elongation aspect ratios > 6:1 (Fig. 4c). Column heights may range from 40 to 60 cm, and they commonly develop in the upright position making parallel ridge-like structures (Fig. 4c). In sections perpendicular to the long axis, the structures look like narrow columns with width ranging over 4–6 cm. The constituent laminae show slight upwards curvature in transverse sections, whereas they appear as nearly flat in sections parallel to the long axis. The inter-column areas are filled up with brown micrite/lime mud. The columns exhibit a very persistent direction of elongation. In several mounds, elongate stromatolites occur in close association with non-branching columnar types.
Columnar stromatolites with curved axes are defined as sinusoidal stromatolites (Tosti & Riding, Reference Tosti and Riding2017 a) and generally have a fixed column width of 4–5 cm (Fig. 3a). The symmetric convex-up laminae follow the column curvature and are approximately normal to the column axis. As the column progressively curves to the left or right, lamina orientation rotates and follows the curvature of the column. Locally, the stromatolite columns are broken and/or displaced within the sinuous stromatolite-bearing horizons; in particular, such deformed fragments are common where the column curvature is greatest. Spaces between stromatolite columns are filled with reddish-brown calcareous shale and rarely with platy lime clasts. In places, the columns are broken and randomly oriented. The columns can have smooth and/or broken margins with or without a wall. The nature of successive lamina growth and shape of stromatolites (synoptic relief) are moderate to high (width W c. height H to < H; Hofmann, Reference Hofmann1969; Grey & Awramik, Reference Grey and Awramik2020), and this gradually passes into a lower relief (W > H; Hofmann, Reference Hofmann1969; Grey & Awramik, Reference Grey and Awramik2020) stromatolite, which ultimately passes into a thick tidal sand body, lacking any trace of stromatolite. In the shallowing-upwards cycle, synoptic relief decreases upwards; increases in the synoptic relief indicate deepening. It is noteworthy that the associated sediments commonly exhibit hummocky cross-stratification and soft-sediment deformation structures such as contortions, slumps and flames.
4.b. Microscopic observations
Microscopic investigations were conducted on samples from sinuous stromatolites. The main micromorphology observed in thin-section is parallel and crinkly/folded lamination (Fig. 5). Lamination consists of alternations of dark thin (50–100 µm) and light thick (< 0.5 mm) laminae, showing different degrees of neomorphism. The laminae are well preserved but some laminae are disrupted in the lower parts of columns (Figs 3d, 5c). Light laminae in the lower and middle parts of a single sinuous stromatolite contain abundant, poorly preserved, round, oval or ovoid peloids (peloidal laminae) with a diameter varying over 200–300 µm (Fig. 5a). All peloids are entirely composed of micrite and some are elongate (< 500 µm long) and occur in more clay-rich laminae. In the upper part, larger, elongate (200–600 µm), oval to angular-shaped intraclasts are observed in the light laminae.
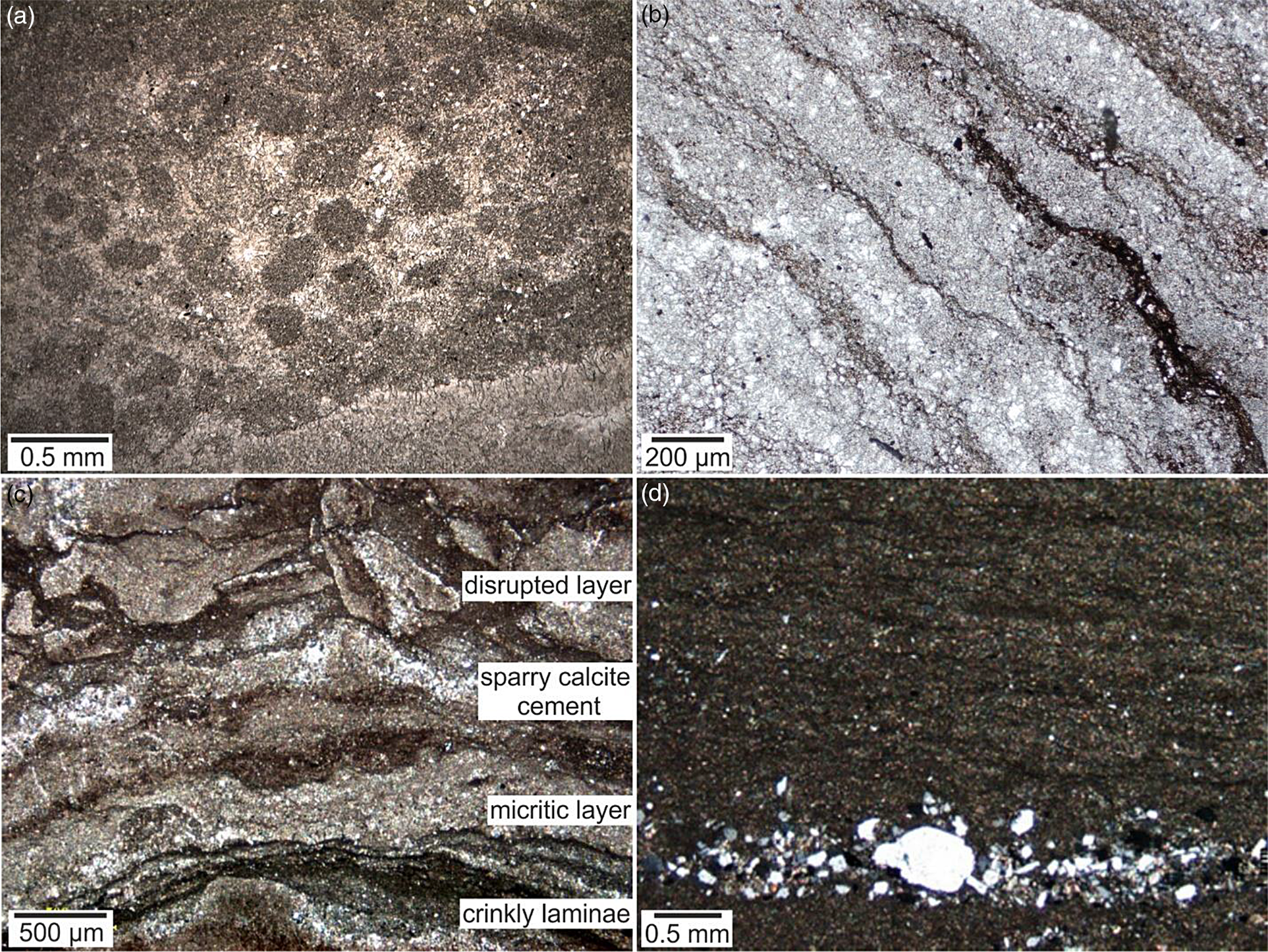
Fig. 5. Photomicrographs of Chandi Formation sinuous stromatolites: (a) round and oval peloids in the light laminae under plane-polarized light (PPL); (b) plane-polarized and (c) crossed-polarized light and dark crinkly laminae, some disrupted; patches of micrite replaced by larger rhombic and euhedral calcite crystals (microspar) are also visible in the light laminae; (d) crossed-polarized wispy organic-rich laminae and thicker micritic laminae in a stromatolite; note the lamina of silt and sand grains, mostly composed of quartz.
A feature of some laminae is the presence of small spheroidal grains with diameters varying from 50 to 200 µm (Fig. 6a–c). Some of these appear to have a nucleus of micritic crystals and are surrounded by clear coarse calcite spar. Others with a nucleus have a vague pattern of radiating crystals.
Dark thin laminae are crinkly or folded and made up of clay (< 2%) or apparently preserved organic matter, whereas light thick laminae contain fine-to-coarse euhedral to subhedral calcite grains, defining a near-parallel alignment (Fig. 5b–d). In some places, light laminae are graded upwards from coarse-grained to micritic calcite. Further, there are patches of coarse calcite crystals, with an outline of an incomplete circle.
Chemical analyses obtained with EDS show that stromatolites are dominated by a calcite mineralogy both in the light and dark laminae. The dark laminae also contain subordinate amounts of quartz, amphibole, pyrite and feldspar (Fig. 5d). The upper portions of stromatolite columns contain dolomite crystals, in places dominating over calcite (Fig. 6). After etching, no evidence of microbial relicts has been observed with the SEM.

Fig. 6. (a, b) Euhedral (mostly fine) dolomite (dol) crystals dominating over coarser calcite (cal) crystals, commonly present in the upper portions of stromatolite columns. Spherical-shaped collections of calcite crystals with apparent centres/nuclei, which could be calcite replaced aragonitic ooids (s). Grey crystals are quartz (q) and black crystals are pyrite (py). (a) plane-polarized and (B) crossed-polarized. (c) Calcitic spheroids which look like spherulites (arrows). Some of them could have nuclei and be small ooids, originally aragonite. The irregular lamina (L) is a calcitic lamina with some spheroidal grains within it. Plane-polarized.
There are small cavities within the stromatolites and adjacent sediment (Figs 7–9), some beneath elongate intraclasts. In some cases the cavities are lined with an isopachous, near-fibrous (Figs 7, 8), pale brown, inclusion-rich, brightly luminescing calcite, which may also occur as a cement between grains (Fig. 9a, b). Cavities are filled with a clear calcite spar showing a drusy texture. There are patches of microspar and coarser spar within the micritic sediment itself, which shows a moderate luminescence (Fig. 9a–d). In addition, there are scattered dolomite rhombs and small crystal aggregates in the micrite (Fig. 9e, f), with a dark red luminescence from the presence of Fe. Fractures cutting the limestones are filled with non-luminescent calcite (Fig. 9).

Fig. 7. Fibrous calcite cements partly filling primary cavities with late-stage clear calcite spar: (a, b) plane-polarized; and (c) crossed-polarized.

Fig. 8. Cavity structure formed below intraclasts of microbial laminae filled with geopetal sediment and later calcite spar with a drusy fabric: (a) plane-polarized and (b) crossed-polarized.

Fig. 9. (a) Plane-polarized and (b) CL photomicrographs showing disrupted dark laminae composed of calcite micrite with a cavity structure (containing geopetal sediment, with bright specks) lined first by an isopachous inclusion-rich, brightly luminescent, fibrous calcite cement, likely marine, and a later coarser, non-luminescent calcite spar cement, likely burial. A late-stage fracture crosses the field of view and is also filled with non-luminescent calcite. Bright luminescence indicates elevated Mn from suboxic conditions and non-luminescence indicates quenching by Fe. (c) Plane-polarized and (d) CL images showing the presence of grains, round to angular shaped, interpreted as peloids and reworked microbial mat clasts, in a medium-grey, fine-grained sediment with local patchy microspar, and pale-grey, clear microspar in a zone upper right and slightly darker microspar bottom right. A fracture crosses the field of view. The CL image shows grain (G) and sediment relicts in a red colour, microspar (M) in a more yellowish colour and the darker red colours are small patches of dolomite (D). The late fracture is filled with dark red luminescent calcite; (e) Plane-polarized and (f) CL images showing scattered dolomite rhombs in a micritic calcitic sediment, showing a central part of moderately bright red luminescence and an outer zone of non-luminescence. Note that under CL, peloids can be discerned by surrounding rhombs.
4.c. Geochemistry
The major-element, trace-element and REE data (online Supplementary Tables S1, S2) collected from the Chandi Formation stromatolitic limestones can be used as a proxy to decipher redox conditions and reconstruction of palaeo-ocean chemistry (Frimmel, Reference Frimmel2009). It is, however, worth mentioning that the abundance and variation patterns of major-element, trace-element and REEs are strongly dependent on the detrital contamination of the carbonate rocks. Microbial mats are good at trapping detrital clays and contaminants; it would therefore be beneficial to test the geochemical data for contamination before starting a detailed discussion on the redox setting and palaeo-seawater chemistry. REE and other trace elements from continental silicate detritus usually have a much higher concentrations of these elements than the carbonate, and can be correlated with the detrital contents in the rock samples (Ling et al. Reference Ling, Chen, Li, Wang, Shields-Zhou and Zhu2013). The elevated Fe and Al concentrations and strong positive correlation between Fe versus Al and Ti (Fig. 10a, b) imply incorporation of terrigenous material, suggesting that iron is present as a silicate component rather than as oxy-hydroxides (e.g. Murray et al. Reference Murray, Buchholtz ten Brink, Gerlach, Russ and Jones1992; Webb & Kamber, Reference Webb and Kamber2000; Ling et al. Reference Ling, Chen, Li, Wang, Shields-Zhou and Zhu2013).

Fig. 10. Cross-plots of (a, b) Fe vs Al and Ti; (c, d) V, Cr, Co vs Al and Ti; and (e, f) U, Th vs Al and Ti from Chandi Formation sinuous stromatolites (oxide percent is converted into ppm = oxide × conversion factor × 10 000).
To check the detrital control on the trace-element concentration, we plotted cross-plots of the trace element versus Al and Ti (which are of detrital origin and usually remain immobile during diagenesis; Fig. 10c–f) following Tribovillard et al. (Reference Tribovillard, Algeo, Lyons and Riboulleau2006). The cross-plots of V, Cr, Co, U and Th versus Al and Ti (Fig. 10c–f) show a strong positive correlation, suggesting contamination by detrital components. The terrigenous detrital influx in the carbonate is also reflected by the tendency towards uniform, flat shale-normalized REE+Y patterns (Nothdurft et al. Reference Nothdurft, Webb and Kamber2004; Frimmel, Reference Frimmel2009). In addition, the ΣREE values of the Chandi stromatolite limestone samples are significantly high (average, 96.3 ppm; standard deviation (SD), 21.5 ppm). Given that marine carbonate sediments in general have low ΣREE, ranging over 0.04–14 ppm (Turekian & Wedepohl, Reference Turekian and Wedepohl1961), they probably indicate contamination by detrital minerals. The ΣREE of these stromatolite samples also shows a strong positive correlation with Al, Si, Fe and Ti (Fig. 11a–d) that probably suggests significant input of detrital material, notably clay minerals, Fe–Mn-oxides and sulphide sources (Frimmel, Reference Frimmel2009). In view of the likely detrital influence, the addition of REE from the contaminants would also weaken the Ce anomaly of the carbonate. The geochemical data collected from the Chandi Formation stromatolitic limestones are therefore contaminated by terrigenous material, and cannot be used any further as proxies to decipher redox conditions and to reconstruct the palaeo-ocean chemistry.

Fig. 11. Cross-plots showing variation in total REE contents relative to (a) Al content, (b) Si content, (c) Mn content and (d) Fe content. Shale contamination trend is from Frimmel (Reference Frimmel2009).
5. Discussion
5.a. Depositional environment
The Chandi Formation, with microbialites, hummocky cross-stratification and soft-sediment deformation structures, is interpreted as a shallow-water carbonate facies (Patranabis-Deb et al. Reference Patranabis-Deb, Słowakiewicz, Tucker, Pancost and Bhattacharya2016). The well defined sinuous pattern of light and dark laminae in the Chandi stromatolites suggests current-dominated conditions, with growth in the near-subtidal zone with rare episodes of sub-aerial exposure. On the other hand, inclined non-branching stromatolites with marly layers imply current conditions, which in the present case are characterized by rhythmic (periodic or episodic) transport of clayey material. Importantly, the material between stromatolite columns contains more clay than within the stromatolites themselves, which suggests a high clay/mud input to the basin. The inter-stromatolite material is higher in clay content, whereas the stromatolites have thick carbonate-rich laminae alternating with thin clayey material (Figs 2b, 4a). In this case, carbonate is being produced within the microbial communities that form the stromatolite. Moreover, the presence of micritic light layers and micrite remnants in the dark layers, and the occurrence of peloids and intraclasts, collectively suggest that carbonate mud was the dominant building material available for these stromatolites, a typical feature of late Proterozoic stromatolites (Awramik & Riding, Reference Awramik and Riding1988). Furthermore, the fine light–dark lamination is also characteristic of Proterozoic stromatolites and the evidence of likely microbial precipitation is in contrast to many Phanerozoic stromatolites accreted through trapping and binding of detrital sediment, which then commonly show poor to coarse lamination (Grotzinger, Reference Grotzinger1989).
The association of sinuous stromatolites, the elongate stromatolites and the branched stromatolites, all with very low synoptic relief, also indicates a shallow-water depth. The sedimentation and growth rate of these stromatolites were well balanced throughout the platform. Furthermore, the elongate bioherms with the well defined trend and the sinuosity itself are likely related to the direction of water flow and sediment supply (cf. Hoffman, Reference Hoffman1967; Gebelein, Reference Gebelein1969; Chaudhuri, Reference Chaudhuri1970; Semikhatov et al. Reference Semikhatov, Gebelein, Cloud, Awramik and Benmore1979). They are typical of subtidal to intertidal settings of high to moderate energy ramps, where elongation of the mounds as well as the structures within them is dependent on the relative amount of wave surge and/or tidal strength (Playford & Cockbain, Reference Playford and Cockbain1969, Reference Playford, Cockbain and Walter1976; Hofmann, Reference Hofmann and Walter1976; Grotzinger, Reference Grotzinger1989; Beukes, Reference Beukes1987). Based on all signatures, the Chandi bioherms suggest exposure to strong tidal currents along with wave action and occasional storm surges in an open headland setting (Hofmann, Reference Hofmann and Walter1976).
Peloids represent an important and common constituent of shallow-marine carbonate sediments. However, in contrast to Phanerozoic marine stromatolites, larger grains exceeding c. 0.1 mm in diameter are only locally found in Proterozoic columnar stromatolites (Gebelein, Reference Gebelein1969; Awramik & Riding, Reference Awramik and Riding1988; Riding et al. Reference Riding, Braga and Martin1991). In this regard, the large micritized peloids occurring in the Chandi sinuous stromatolites are significant and similar to those reported from Precambrian stromatolites elsewhere (e.g. Tewari & Tucker, Reference Tewari, Tucker, Tewari and Seckbach2011). These large peloids do not form aggregates or have the clotted microfabrics typically produced by small (< 150 μm) micritized bacterial cells such as coccoid cyanobacteria (Kaźmierczak et al. Reference Kaźmierczak, Coleman, Gruszczyński and Kempe1996; Riding & Tomás, Reference Riding and Tomás2006). Further, the oval and elongate peloids and intraclasts observed in the light laminae (Fig. 5a) do not show any particular orientation, which, if present, would be caused by current transport as is commonly observed in other Phanerozoic and Proterozoic stromatolites and reefal stromatolites (Flügel, Reference Flügel2010). However, these grains were disrupted during vertical accretion of the already lithified stromatolitic structure upwards into the zone of physical destruction dominated by wave action and subsequently cemented (Fig. 5a). The Chandi stromatolites therefore probably represent prolific in situ carbonate precipitation in the form of major build-ups and bioherms. In addition, an elevated seawater carbon saturation state (Grotzinger, Reference Grotzinger1989; Bartley & Kah, Reference Bartley and Kah2004) may also have contributed to the preservation of these unusual stromatolitic forms by their early lithification. Finally, the direction of growth was controlled by the current direction, as in the case of the Tieling stromatolites (Tosti & Riding, Reference Tosti and Riding2017 a), and is similar to clastitropism (the directional accretion of Shapiro et al. Reference Shapiro, Aalto, Dill and Kenny1995).
In addition, there is no evidence of the truncation of grains, which would indicate a brittle lithified fabric. Furthermore, the absence of deformed columns and contortions of laminae points to the likelihood of their early lithification prior to burial, which is also supported by the presence of broken stromatolitic microbial laminae observed in the disrupted layer (Fig. 5c). These observations also confirm that the sinuous stromatolite had a rigid but not firm consistency since early cements are present in the Chandi stromatolites (Figs 5a, 6).
Some of the clear sparitic spheroidal grains (Fig. 6) could be replaced ooids. The texture could indicate an original aragonite mineralogy. Some are reminiscent of the spherulites described from many stromatolites (e.g. Kirkham & Tucker, Reference Kirkham and Tucker2018) and ascribed to a microbial origin, at least for the central nucleus. The surrounding fibrous crystal texture could be a syntaxial abiotic overgrowth.
The numerous vugs and fractures, particularly in the light laminae, might have resulted from the trapping of gas generated by organic matter decay (as in fenestrae); other voids formed beneath intraclasts of microbial lamina fragments, or from early diagenetic dissolution of calcite micrite (Figs 5 and 6; Grotzinger et al. Reference Grotzinger, Watters and Knoll2000). The isopachous fringes of fibrous calcite lining the cavities are probably a marine precipitate, with the later coarse calcite spar being a burial precipitate (Fig. 9a). Patchy areas of coarser microspar and spar in the dark laminae are likely the result of neomorphism and recrystallization of micrite.
5.b. Origin of Chandi sinuous stromatolites
Sinuous forms that resemble the Chandi stromatolites are reported from the c. 1400 Ma Altyn Formation, Montana (Fenton & Fenton, Reference Fenton and Fenton1937, pl. 9; Horodyski, Reference Horodyski1977, fig. 4b) and also the c. 1450 Ma Helena/Siyeh Limestone (Fenton & Fenton, Reference Fenton and Fenton1937, pl. 18; Horodyski, Reference Horodyski1977, fig. 4b; Horodyski, Reference Horodyski, Nagy, Weber, Guerrero and Schidlowski1983, figs 13e, 15a) and the c. 1400 Ma Tieling Formation (Tosti & Riding, Reference Tosti and Riding2017 a). Platella described from the 1272–1211 Ma (Gorokhov et al. Reference Gorokhov, Semikhatov, Arakelyants, Fallick, Mel’nikov, Turchenko, Ivanovskaya, Zaitseva and Kutyavin2006) Debengda Formation (Serebryakov, Reference Serebryakov and Walter1976, fig. 1) has sinuous columns, but they rather resemble the ridge–runnel system characteristic of Tieling stromatolites (Tosti & Riding, Reference Tosti and Riding2017 a). Similarly, Chandi sinuous stromatolites differ from modern Hamlin Pool stromatolites (Awramik & Vanyo, Reference Awramik and Vanyo1986), which are rather inclined. In addition, the Chandi sinuous stromatolites are characterized by an ideal ‘S’ (angles of +45° to –45°) shape of their columns, similar to S-shaped Tieling stromatolites near Zhoukoudian, China (Qu et al. Reference Qu, Xie and Gong2004). Importantly, as in the case of the Tieling sinuous stromatolites (Tosti & Riding, Reference Tosti and Riding2017 a), Chandi sinuous stromatolites accompanied by non-branching inclined forms (Fig. 4a–c) lacking sinuosity grew in different positions on the carbonate platform where the current action was dominant, as in the case of various Palaeoproterozoic stromatolitic forms controlled by wave agitation, depth and tidal action (Patranabis-Deb et al. Reference Patranabis-Deb, Majumder and Khan2018) and as observed in modern Shark Bay (Jahnert & Collins, Reference Jahnert and Collins2011, Reference Jahnert and Collins2012). The change of one stromatolite form to another is also linked to variations of sediment supply and framework growth (Planavsky & Grey, Reference Planavsky and Grey2008).
It is therefore suggested that inclined and sinuous columns do not have a heliotropic origin, but rather their column curvature is a product of current and tidal action. Indeed, Hofmann (Reference Hofmann1973) regarded inclined and curved stromatolites as derived from currents and Shapiro (Reference Shapiro and Miller2007) noted that stromatolites may accrete into the current when high-velocity currents are present. Such a phenomenon is observed in modern Lee Stocking stromatolite columns, which grow or lean towards the incoming tidal flow (Dill et al. Reference Dill, Shinn, Jones, Kelly and Steinen1986). A similar interpretation was given by Tosti & Riding (Reference Tosti and Riding2017 a, b) for inclined and sinuous columns of the Tieling stromatolites. Additionally, the ability of the stromatolites to change from a low synoptic relief to a high synoptic relief indicates that they could adapt to changing environmental conditions.
5.c. Seawater chemistry
Pure carbonate rocks can record the chemical signatures of seawater at the time and these may reflect the redox changes in the depositional environment (Webb & Kamber, Reference Webb and Kamber2000). Geochemical data of the Chandi stromatolitic limestone (and many other stromatolitic limestone successions around the world) are unfortunately invalidated by the incorporation of terrigenous material, meaning that it is not possible to give any reliable interpretations of palaeo-redox conditions and other features of the original palaeo-ocean chemistry. This result is consistent with the sedimentological and microscopic observations of this unit containing muddy carbonates, clay-rich laminae and the presence of subordinate amounts of quartz, amphibole, pyrite and feldspar (Fig. 5d). In this regard, stromatolites can trap various detrital grains whose amount is controlled by changing hydrodynamic conditions (Gerdes et al. Reference Gerdes, Klenke and Noffke2000; Frantz et al. Reference Frantz, Petryshyn and Corsetti2015; Lan et al. Reference Lan, Zhang, Tucker, Li and Zhao2020).
The present understanding of the Mesoproterozoic Ocean suggests that the surface water during that time was mostly oxic but that the deep ocean remained anoxic and ferruginous with widespread euxinic (anoxic and sulphidic) conditions prevailing along the continental margins (Poulton et al. Reference Poulton, Fralick and Canfield2010; Planavsky et al. Reference Planavsky, McGoldrick, Scott, Li, Reinhard, Kelly, Chu, Bekker, Love and Lyons2011; Poulton & Canfield, Reference Poulton and Canfield2011). It is presently argued, at least locally, from some of Mesoproterozoic basins, that the ocean water redox conditions during that time (c. 1400 Ma) were variable and the deep ocean waters were probably also oxygenated (Zhang et al. Reference Zhang, Wang, Wang, Bjerrum, Hammarlund, Costa, Connelly, Zhang, Su and Canfield2016; Wang et al. Reference Wang, Zhang, Wang, Bjerrum, Hammarlund, Haxen, Su, Wang and Canfield2017). However, due to detrital contamination, geochemical proxies from the Chandi stromatolitic limestones cannot be used to give information on the shallow-marine ocean chemistry and the redox conditions at that time.
6. Conclusions
The Chandi stromatolites consist of sinuous (S-shaped), branched and non-branching inclined columns forming biostromes and bioherms ranging from several centimetres to several metres in height. Sinuous columns are predominant types and reflect the interaction between microbial mat growth, carbonate precipitation and current-supplied detrital sediment. We attribute the S-shaped pattern to changes in current direction and trapping of fine-grained sediment in suspension, with some in situ precipitated carbonate. The inclined columns display a cyclic pattern of growth interrupted by marly/clayey material. The sinuosity of the Chandi stromatolitic columns shows similarities to mid-Mesoproterozoic examples in Laurentia (c. 1400 Ma, Altyn Formation, Helena/Siyeh Limestone and Tieling Formation) and Siberia (Debengda Formation, 1272–1211 Ma).
Supplementary material
To view supplementary material for this article, please visit https://doi.org/10.1017/S0016756821000674
Acknowledgements
Julie Bartley and an anonymous reviewer are thanked for their constructive comments and suggestions that helped to improve our manuscript. Debanuj Sinha is acknowledged for providing photomicrographs and Marcin D. Syczewski is thanked for helping with scanning electron microscopy. This work was supported by, and contributes to, the Proterozoic research programme of the Indian Statistical Institute. This study was also supported by the Russian Government Programme of Competitive Growth of the Kazan Federal University.
Declaration of interest
We declare no conflict of interest.