Introduction
Depressive disorders are highly prevalent in childhood and adolescence, with an annual incidence of 6–7% (Costello et al. Reference Costello, Angold, Burns, Stangl, Tweed, Erkanli and Worthman1996; Merikangas & Avenevoli, Reference Merikangas, Avenevoli, Tsaung and Tohen2002). Adolescents with depression form a particular group of concern because suicide, which has a high incidence in depressed youths, is the third leading cause of death between the ages of 15 and 24 years (Murray et al. Reference Murray, Salomon and Mathers2000; CDC, 2007). Furthermore, adolescent depression is associated with a two- to fourfold risk of recurrence in adulthood along with ongoing psychosocial problems such as dysfunctional interpersonal relationships, substance abuse and poor occupational functioning (Clark et al. Reference Clark, Rodgers, Caldwell, Power and Stansfeld2007; Rao & Chen, Reference Rao and Chen2009). Of note, depression typically co-occurs with anxiety during adolescence, and a double diagnosis accounts for more impairment and more severe internalizing symptoms than either diagnosis alone (Zahn-Waxler et al. Reference Zahn-Waxler, Klimes-Dougan and Slattery2000; Guberman & Manassis, Reference Guberman and Manassis2011). Remarkably, despite these concerns, the neurobiology of adolescent depression is still poorly understood. The substantial neuronal maturation during adolescence (Casey et al. Reference Casey, Jones and Hare2008) underscores the need to examine the neurobiology of adolescent depression separately from adults. Yet, neurobiological research in adolescent depression significantly lags behind similar advances in adult depression (Hulvershorn et al. Reference Hulvershorn, Cullen and Anand2011). Bearing in mind the increased neuronal plasticity prior to adulthood (Casey et al. Reference Casey, Jones and Hare2008), studying the neurocircuitry of adolescent depression is crucial in gaining insight into the neurodevelopmental trajectories of depression and advancing the development of potential interventions that target these trajectories (Hulvershorn et al. Reference Hulvershorn, Cullen and Anand2011). This is of great importance because effective treatment strategies for adolescent depression are relatively scarce.
Neuroimaging studies implicate corticolimbic circuit dysfunction in the pathophysiology of both adult and adolescent depression (Thomas et al. Reference Thomas, Drevets, Dahl, Ryan, Birmaher, Eccard, Axelson, Whalen and Casey2001; Mayberg, Reference Mayberg2003; Rosso et al. Reference Rosso, Cintron, Steingard, Renshaw, Young and Yurgelun-Todd2005; Caetano et al. Reference Caetano, Fonseca, Hatch, Olvera, Nicoletti, Hunter, Lafer, Pliszka and Soares2007; Price & Drevets, Reference Price and Drevets2010; Hulvershorn et al. Reference Hulvershorn, Cullen and Anand2011). Key components of this circuitry include the amygdala, hippocampus, anterior cingulate cortex (ACC) and prefrontal cortex (PFC), which regulate the experience, expression and evaluation of emotions (Benes, Reference Benes2010). A review of neuroimaging findings in pediatric depression (Hulvershorn et al. Reference Hulvershorn, Cullen and Anand2011) suggests three possible trajectories for how typical neurodevelopment of this corticolimbic circuitry might be compromised in depression. First, genotype may contribute to early abnormal neurodevelopment that is further worsened by adverse gene–environment interaction; second, typical neurodevelopment early in life may gradually become abnormal with accumulating environmental adversity; and third, typical neurodevelopment through childhood may abruptly become abnormal in adolescence or adulthood, possibly due to a genetic susceptibility. Abnormal development of brain white matter (WM) is thought to constitute one element of the aforementioned corticolimbic circuit dysfunction (Fields, Reference Fields2008; Herrmann et al. Reference Herrmann, Le Masurier and Ebmeier2008). Aberrant structural integrity of corticolimbic WM pathways may impede adaptive emotional functioning, thereby rendering an individual vulnerable to psychopathology.
Diffusion tensor imaging (DTI) has proven a useful magnetic resonance imaging (MRI) technique for examining the orientation and integrity of WM tracts by indexing the diffusion of water molecules in neural tissue (Basser et al. Reference Basser, Mattiello and LeBihan1994). Fractional anisotropy (FA) is the most commonly used DTI parameter, reflecting the tendency of water molecules to diffuse in one direction as opposed to all other directions. As diffusion within WM is strongly restricted by its microstructure, FA values reflect microstructural properties such as myelin thickness and membrane integrity (Kochunov et al. Reference Kochunov, Thompson, Lancaster, Bartzokis, Smith, Coyle, Royall, Laird and Fox2007). Decreased diffusion along the principal direction of the fiber (axial diffusivity, AD) suggests axonal loss (Budde et al. Reference Budde, Xie, Cross and Song2009), whereas increased diffusion perpendicular to the principal direction of the fiber (radial diffusivity, RD) suggests demyelination (Song et al. Reference Song, Sun, Ramsbottom, Chang, Russell and Cross2002). In addition, an increase in the average of water diffusion in all directions (mean diffusivity, MD) suggests diminished myelination (Horsfield & Jones, Reference Horsfield and Jones2002). In general, decreased FA is coupled with decreased AD and/or increased RD and MD, whereas the opposite accounts for any increase in FA.
DTI studies using a region-of-interest (ROI) approach indicate loss of WM integrity in adult and adolescent depression, as reflected by decreased FA in the frontal and temporal lobes, and the cingulum bundle (Nobuhara et al. Reference Nobuhara, Okugawa, Sugimoto, Minami, Tamagaki, Takase, Saito, Sawada and Kinoshita2006; Li et al. Reference Li, Ma, Li, Tan, Liu, Gong, Shu, He, Jiang and Xu2007; Yang et al. Reference Yang, Huang, Hong and Yu2007; Shimony et al. Reference Shimony, Sheline, D'Angelo, Epstein, Benzinger, Mintun, McKinstry and Snyder2009; Cullen et al. Reference Cullen, Klimes-Dougan, Muetzel, Mueller, Camchong, Houri, Kurma and Lim2010). However, by using an ROI approach, important alterations in WM microstructure outside the ROIs may have gone unobserved in these studies. Studies examining whole-brain WM integrity in adult and adolescent depression largely converge on findings of decreased FA in regions such as the corpus callosum (CC), superior longitudinal fasciculus, uncinate fasciculus (UF), sagittal stratum and internal capsule (Ma et al. Reference Ma, Li, Shu, Liu, Gong, He, Li, Tan, Stone, Zhang, Xu and Jiang2007; Cullen et al. Reference Cullen, Klimes-Dougan, Muetzel, Mueller, Camchong, Houri, Kurma and Lim2010; Kieseppa et al. Reference Kieseppa, Eerola, Mantyla, Neuvonen, Poutanen, Luoma, Tuulio-Henriksson, Jylha, Mantere, Melartin, Rytsala, Vuorilehto and Isometsa2010; Korgaonkar et al. Reference Korgaonkar, Grieve, Koslow, Gabrieli, Gordon and Williams2011; Zhu et al. Reference Zhu, Wang, Xiao, Zhong, Liao and Yao2011). Of note, some of these WM alterations are also found in healthy adolescents at familial risk for depression (Huang et al. Reference Huang, Fan, Williamson and Rao2011), suggesting that specific WM alterations early in life may serve as a vulnerability marker for depression. Finally, FA alterations in depression are also related to greater symptom severity in both adults and adolescents (Zou et al. Reference Zou, Huang, Li, Gong, Li, Ou-yang, Deng, Chen, Li, Ding and Sun2008; Zhu et al. Reference Zhu, Wang, Xiao, Zhong, Liao and Yao2011), and to poor treatment outcomes in adults (Alexopoulos et al. Reference Alexopoulos, Murphy, Gunning-Dixon, Latoussakis, Kanellopoulos, Klimstra, Lim and Hoptman2008).
Despite growing evidence of WM alterations in depression, only a few studies have examined WM microstructural integrity in adolescent depression (Li et al. Reference Li, Ma, Li, Tan, Liu, Gong, Shu, He, Jiang and Xu2007; Ma et al. Reference Ma, Li, Shu, Liu, Gong, He, Li, Tan, Stone, Zhang, Xu and Jiang2007; Cullen et al. Reference Cullen, Klimes-Dougan, Muetzel, Mueller, Camchong, Houri, Kurma and Lim2010; Zhu et al. Reference Zhu, Wang, Xiao, Zhong, Liao and Yao2011). Moreover, methodological issues such as small sample size, non-matched control subjects and medication use may to some extent limit the findings reported by these studies. Importantly, given the singular focus of these studies on FA, microstructural properties underlying the alterations in FA remain unknown. Hence, we still lack a firm understanding of WM architecture in adolescent depression. To mitigate the paucity of data and address the limitations of previous studies, we examined WM integrity in a moderate-sized sample of treatment-naive adolescents with clinical depression relative to matched controls. Additionally, to further characterize WM alterations, we examined AD, RD and MD in regions showing significant group differences in FA. The intention was to shed some new light on the neurocircuitry underlying adolescent depression.
Tract-based spatial statistics (TBSS; Smith et al. Reference Smith, Jenkinson, Johansen-Berg, Rueckert, Nichols, Mackay, Watkins, Ciccarelli, Cader, Matthews and Behrens2006), a robust and automated technique, was used for the analysis of diffusion-weighted data. We used both whole-brain and ROI TBSS to examine WM microstructure. This is the first study to use ROI-based TBSS to examine WM microstructural integrity in adolescent depression. Unlike traditional ROI analyses, ROI-based TBSS allows voxel-wise analysis of FA within an entire WM tract common to all participants (i.e. skeletonized tract), thus minimizing registration errors and partial voluming. Given the hypothesized corticolimbic circuit dysfunction in depression (Mayberg, Reference Mayberg2003; Price & Drevets, Reference Price and Drevets2010), the UF was defined as our ROI. The UF is a bidirectional WM tract that connects limbic structures such as the amygdala with the orbitomedial PFC (OMPFC) and subgenual ACC (sACC) (Petrides & Pandya, Reference Petrides and Pandya2002; Kier et al. Reference Kier, Staib, Davis and Bronen2004), thereby subserving emotional processing and memory (Von Der Heide et al. Reference Von Der Heide, Skipper, Klobusicky and Olson2013). Previous DTI studies largely converge on reduced FA in the UF in depressive disorders (Sexton et al. Reference Sexton, Mackay and Ebmeier2009; Cullen et al. Reference Cullen, Klimes-Dougan, Muetzel, Mueller, Camchong, Houri, Kurma and Lim2010), while lower as well as higher FA in the UF predicts negative emotionality in healthy individuals (Kim & Whalen, Reference Kim and Whalen2009; Westlye et al. Reference Westlye, Bjornebekk, Grydeland, Fjell and Walhovd2011; Montag et al. Reference Montag, Reuter, Weber, Markett and Schoene-Bake2012). Of interest, functional integrity of a corticolimbic circuitry was recently shown to reflect structural integrity of the UF in depressed patients (Steffens et al. Reference Steffens, Taylor, Denny, Bergman and Wang2011). We therefore hypothesized the UF a suitable candidate for further clarifying corticolimbic abnormalities in depression.
Based on the putative link between abnormal WM FA and depression (Sexton et al. Reference Sexton, Mackay and Ebmeier2009), we hypothesized that, in depressed adolescents, whole-brain analysis of FA would reveal abnormalities within tracts previously implicated in depression. Moreover, we hypothesized that, in depressed adolescents, ROI analysis of FA would reveal abnormalities in the UF. In line with previous TBSS studies (Versace et al. Reference Versace, Almeida, Hassel, Walsh, Novelli, Klein, Kupfer and Phillips2008; Cole et al. Reference Cole, Chaddock, Farmer, Aitchison, Simmons, McGuffin and Fu2012), FA was the main dependent variable, with AD, RD and MD providing complementary information to aid interpretation of FA alterations. We therefore hypothesized that reduced FA would be coupled with reduced AD and/or elevated RD and MD, whereas the opposite was expected for any increase in FA. Finally, in line with previous findings (Zou et al. Reference Zou, Huang, Li, Gong, Li, Ou-yang, Deng, Chen, Li, Ding and Sun2008; Zhu et al. Reference Zhu, Wang, Xiao, Zhong, Liao and Yao2011), we hypothesized that abnormal FA values would relate to greater depression symptom severity.
Method
Participants
Forty-six adolescents (25 patients, 21 controls) were selected, as part of the Emotional Pathways Imaging Study in Clinical Adolescents (EPISCA). EPISCA is a longitudinal MRI study in which adolescents with clinical depression and healthy controls were followed over a 6-month period (from January 2010 to August 2012). The clinically depressed group underwent an MRI scanning protocol prior to the start of their regular cognitive behavioral therapy (CBT), and 3 and 6 months after the start of CBT. Healthy control adolescents were recruited through local advertisements. The current study reports on cross-sectional data from both groups.
Inclusion criteria for the patient group were: having clinical depression as assessed by categorical and/or dimensional measures of DSM-IV depressive disorders (for more detail see the section on Clinical Assessment), being referred for CBT at an out-patient care unit, and no current or prior use of antidepressants. Inclusion criteria for the control group were: no current or past DSM-IV diagnoses of Axis I and/or Axis II disorders, no clinical scores on validated mood and behavioral questionnaires, no history of traumatic experiences, and no current psychotherapeutic and/or psychopharmacological intervention of any kind. Exclusion criteria for all participants were: (1) a primary DSM-IV diagnosis of attention deficit hyperactivity disorder, oppositional defiant disorder, conduct disorder, pervasive developmental disorders, post-traumatic stress disorder, Tourette's syndrome, obsessive–compulsive disorder, bipolar disorder, and psychotic disorders, (2) current use of psychotropic medication, (3) current substance abuse, (4) a history of neurological disorders or severe head injury, (5) age < 12 or > 21 years, (6) pregnancy, (7) left-handedness, (8) IQ score < 80, as measured by either the Wechsler Intelligence Scale for Children (WISC; Wechsler, Reference Wechsler1991) or the Wechsler Adult Intelligence Scale (WAIS; Wechsler, Reference Wechsler1997), and (9) general MRI contraindications (e.g. metal implants, claustrophobia).
From the original group of 59 adolescents (29 patients, 30 controls), four (one patient and three controls) were excluded prior to the start of the current DTI study because of image artifacts in T1-weighted anatomical scans. For the current study, an additional nine adolescents were excluded because of: incorrect DTI data collection (one patient and one control), image artifacts in the DTI dataset (one patient and one control), and group-wise matching for age, sex and IQ (one patient and four controls). Eventually, a moderate-sized sample of 46 participants (25 patients, 21 controls) aged 13 to 19 years (mean = 15.07, s.d. = 1.55) were included in the DTI analysis. Participants were scanned within 2 weeks of initial screening, and all were new to MRI scanning procedures. The medical ethics committee of Leiden University Medical Center approved the study and written informed consent was obtained from all adolescents and their parents.
Clinical assessment
For all participants, several clinical measures were used for categorical and dimensional assessment of DSM-IV depressive disorders. For all patients, after the clinical assessment by child and adolescent psychiatrists, categorical DSM-IV diagnoses were further assessed based on the child and parent versions of the Anxiety Disorders Interview Schedule (ADIS; Silverman & Albano, Reference Silverman and Albano1996), a diagnostic tool for obtaining DSM-IV-based classifications of anxiety and depressive disorders. Additional scales were then used to assess the severity of depressive and internalizing symptoms, including the Children's Depression Inventory (CDI; Kovacs, Reference Kovacs1992), the Revised Child Anxiety and Depression Scale (RCADS; Chorpita et al. Reference Chorpita, Yim, Moffitt, Umemoto and Francis2000), the Youth Self-Report (YSR; Achenbach, Reference Achenbach1991b ) and its parent version the Child Behavior Checklist (CBCL; Achenbach, Reference Achenbach1991a ). A detailed description of these questionnaires is provided in the online Supplementary Material. The same measures were applied for the control group, and control participants were excluded when they fulfilled the criteria for a DSM-IV diagnosis or had (sub)clinical scores on clinical questionnaires.
DTI data acquisition and preprocessing
DTI data were collected using a Philips 3.0-T Achieva MRI scanner (Philips Medical Systems, The Netherlands) with an eight-channel sensitivity-encoding (SENSE) head coil. A single-shot echo–planar imaging (EPI) sequence was used with the following scan parameters: repetition time = 11 000 ms, echo time = 56 ms, flip angle = 90°, b factor = 1000 s/mm2, voxel dimensions = 2.3 mm isotropic, number of slices = 73, and no slice gap. DTI data were acquired along 32 directions, together with a baseline image having no diffusion weighting (b = 0). The total scanning time was approximately 7 min. Collected DTI data were preprocessed and analyzed using the Oxford Centre for Functional MRI of the Brain (FMRIB) Software Library (FSL; http://fsl.fmrib.ox.ac.uk/fsl/fslwiki/) version 4.1.9. First, DTI data were corrected for distortion and motion artifacts induced by eddy currents or by simple head motions, using affine registration of each diffusion-weighted image to the b = 0 reference image. Next, non-brain tissue was removed using the Brain Extraction Tool. Finally, to generate individual FA, AD, RD and MD maps for each participant, the diffusion tensor model was fitted to each voxel using FMRIB's Diffusion Toolbox. AD was defined as the largest eigenvalue (L1), RD was calculated as the average of the two small eigenvalues (L2 and L3), and MD was calculated as the average of the three eigenvalues (L1, L2 and L3).
Whole-brain TBSS
TBSS version 1.2 was used for voxel-wise analysis of the preprocessed FA data. First, individual FA images were aligned to the FMRIB58_FA standard-space image using FMRIB's non-linear registration tool (FNIRT). Next, a mean FA image was generated and thinned to create a mean FA skeleton representing the centers of all tracts common to the entire group. The mean FA skeleton was then thresholded at an FA value of ⩾0.35 to exclude peripheral tracts and minimize partial voluming. Finally, each participant's aligned FA images were projected onto the mean FA skeleton. In a similar manner, AD, RD and MD data were projected onto the skeleton using the FA registration and skeleton projection parameters. Subsequently, the skeletonized FA, AD, RD and MD data were fed into voxel-wise permutation-based analysis.
ROI TBSS
To test for regional specific FA alterations with ROI-based TBSS, we followed the procedure used by Westlye et al. (Reference Westlye, Walhovd, Bjornerud, Due-Tonnessen and Fjell2009, Reference Westlye, Bjornebekk, Grydeland, Fjell and Walhovd2011). A binary mask of the bilateral UF was created as the ROI using the probabilistic Johns Hopkins University (JHU) WM atlas (Mori et al. Reference Mori, Wakana, Nagae-Poetscher and van Zijl2005) provided by FSL. The UF was masked by the thresholded mean FA skeleton to include only voxels comprised in both the UF and the skeleton. This confines the statistical analysis to voxels from the center of the tract, thereby minimizing anatomic intersubject variability, registration errors and partial voluming (Westlye et al. Reference Westlye, Walhovd, Bjornerud, Due-Tonnessen and Fjell2009). The resulting UF mask was used for voxel-wise permutation-based ROI analysis.
Statistical analysis
Given the non-parametric distribution of diffusion-weighted data, non-parametric independent two-sample t tests were performed using FSL's permutation-based Randomize tool with threshold-free cluster enhancement (TFCE; Smith & Nichols, Reference Smith and Nichols2009). FA was examined throughout the WM skeleton whereas AD, RD and MD were only examined in regions showing significant group differences in FA. Five thousand random permutations were generated to build up the null distribution of the cluster size statistic, while testing the following contrasts: (1) controls > patients, (2) patients > controls, (3) group × age interaction. Age, sex and IQ (demeaned across groups) were included in the analysis as covariates, as these variables could impact the major outcomes under investigation. Given that we tested for higher as well as lower FA in patients relative to controls, the resulting statistical maps were corrected for multiple comparisons (i.e. family-wise error, FWE) at p < 0.025 rather than p < 0.05. However, to minimize Type II errors, effects that passed the ‘gold standard’ criterion of p < 0.05 FWE corrected are also reported and discussed, albeit with caution. As for AD, RD and MD, a statistical threshold of p < 0.05 FWE corrected was applied, given our explicit hypotheses regarding the direction of effects. The JHU WM and Juelich histological atlases were used to label clusters with significant WM alterations.
To examine the association between FA alterations and symptom severity in patients, CDI depression scores and mean FA values within regions of significant group differences were fed into linear regression analyses in SPSS (SPSS Inc., USA). Age and IQ (demeaned across groups) were included in the analyses as covariates to correct for their confounding effects. As FA values throughout the brain tend to correlate, we aimed to minimize multicollinearity by performing separate regression analyses for every brain region showing significant group differences in FA. As depressive symptoms are known to vary with age during adolescence (Ryan et al. Reference Ryan, Puig-Antich, Ambrosini, Rabinovich, Robinson, Nelson, Iyengar and Twomey1987), an age × CDI interaction effect on FA was also tested in the same model. Finally, statistical thresholds were adjusted to control for multiple testing (i.e. p < 0.05 divided by the number of tests).
Results
Sample characteristics
As shown in Table 1, the matched groups did not differ with respect to age (t 44 = −1.98, p > 0.05), sex (84–86% females; χ 2 1 = 0.26, p > 0.05) and IQ (t 44 = 0.83, p > 0.05). The CDI revealed higher depression scores in patients (t 29 = −6.56, p < 0.001) and the RCADS revealed both higher depression (t 32 = −4.97, p < 0.001) and anxiety scores (t 36 = −4.42, p < 0.001) in the patient group. Moreover, the YSR (t 39 = −7.1, p < 0.001) and CBCL (t 29 = −9.54, p < 0.001) both revealed more internalizing symptoms in patients. The patient group comprised 25 treatment-naive adolescents with clinical depression, as assessed by categorical and/or dimensional measures of depression. Most patients (n = 18) fulfilled criteria for one or more co-morbid anxiety disorders on the ADIS, including generalized anxiety disorder, social phobia and panic disorder with agoraphobia (Table 1). Six of these patients also fulfilled criteria for co-morbid externalizing disorders, including attention deficit hyperactivity disorder, oppositional defiant disorder and conduct disorder.
Table 1. Demographic and clinical characteristics of adolescents with clinical depression (patients) and healthy control participants (controls)

IQ, Intelligence quotient; CDI, Children's Depression Inventory; RCADS, Revised Child Anxiety and Depression Scale; YSR, Youth Self-Report; CBCL, Child Behavior Checklist.
a One patient did not complete the questionnaire.
b Three patients and their parents/primary caregivers did not complete the questionnaire.
Because less than 20% of the items in the CDI, RCADS, YSR and CBCL were missing, expectation maximization as the regression method was used to calculate the scale scores.
Values are given as mean ± standard deviation or number.
* Significant at p < 0.001.
TBSS analysis
As shown in Fig. 1, whole-brain TBSS analysis revealed that patients had lower FA values in the body of the CC (p < 0.025, TFCE and FWE corrected), coupled with elevated RD and MD, and preserved AD (p < 0.05, TFCE and FWE corrected). Compared to controls, patients did not show higher FA values in any WM tracts. By contrast, ROI-based TBSS analysis in the UF revealed that patients had higher FA values in the anterior segment of the left UF (p < 0.05, TFCE and FWE corrected), coupled with elevated AD, reduced RD and preserved MD (p < 0.05, TFCE and FWE corrected). WM tracts with lower FA values for patients than for controls were not identified in the ROI-based analysis.
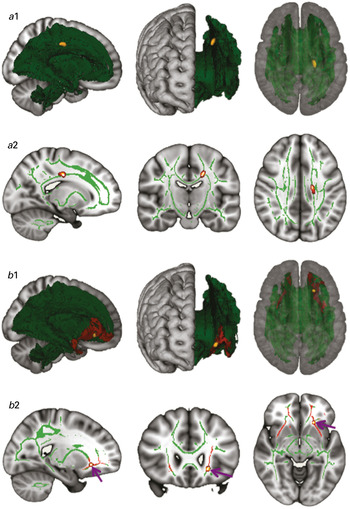
Fig. 1. (a) Whole-brain tract-based spatial statistics (TBSS) results. Three-dimensional (3D) renderings (a1) and sagittal, coronal and axial sections (a2) of the white-matter (WM) skeleton (green), with a subregion of the corpus callosum (CC) showing significantly reduced fractional anisotropy (FA) in patients relative to healthy controls [p < 0.025, threshold-free cluster enhancement (TFCE) and family-wise error (FWE) corrected] (yellow/orange). Reduced FA in this subregion was coupled with elevated RD and MD, and preserved AD (p < 0.05, TFCE and FWE corrected). (b) Region-of-interest (ROI) TBSS. 3D renderings (b1) and sagittal, coronal and axial sections (b2) of the WM skeleton (green) and the bilateral uncinate fasciculus (UF) (red), with a subregion of the UF showing significantly elevated FA in patients relative to healthy controls (p < 0.05, TFCE and FEW corrected) (yellow/orange). Elevated FA in this subregion was coupled with elevated axial diffusivity (AD), reduced radial diffusivity (RD) and preserved mean diffusivity (MD) (p < 0.05, TFCE and FEW corrected). For better visibility, the results are thickened using the ‘tbss-fill’ command, and purple arrows mark the effect site. All images are in radiological convention (right is left and vice versa).
FA alterations and symptom severity
Two separate regression analyses were performed (one for CC and one for UF) to address the association between observed FA alterations and symptom severity in patients (adjusted for age and IQ). Hence, statistical thresholds were set at p < 0.025 (i.e. p < 0.05 divided by the number of tests). As illustrated in Fig. 2, mean FA values in regions of the CC showing significant group differences demonstrated a trend for a negative association with CDI depression scores (β = −0.43, p = 0.055). That is, lower FA values were associated with more depressive symptoms. By contrast, mean FA values in regions of the UF showing significant group differences did not correlate with CDI depression scores (β = −0.30, p = 0.15). Finally, no age × symptom severity interaction was found for FA in the CC (β = −0.05, p = 0.85) or the UF (β = −0.55, p = 0.05).

Fig. 2. Scatter plot visualizing a trending negative association between patients' fractional anisotropy (FA) in the effect site of the corpus callosum (CC) and Children's Depression Inventory (CDI) scores (β = −0.43, p = 0.055).
Discussion
The current study used whole-brain and ROI-based TBSS to examine WM microstructural integrity in a sample of treatment-naive adolescents with clinical depression relative to matched controls. We hypothesized that, in depressed adolescents, whole-brain analysis of FA would reveal abnormalities within tracts previously implicated in depression, whereas ROI analysis of FA would reveal abnormalities in the UF. Moreover, we hypothesized that reduced FA would be coupled with reduced AD and/or elevated RD and MD, whereas the opposite was expected for any increase in FA. Whole-brain analysis revealed that patients had lower FA values in the body of the CC, coupled with elevated RD and MD, and preserved AD. Conversely, ROI analysis revealed that patients had higher FA values in the UF, coupled with elevated AD, reduced RD and preserved MD. Moreover, a trend for an association was found between reduced FA in the CC and more severe depressive symptoms in patients. These results largely confirm this study's hypotheses and add to converging lines of evidence implicating WM abnormalities in the pathophysiology of depression.
Several DTI studies implicate reduction in CC FA in both adult and adolescent depression (Sexton et al. Reference Sexton, Mackay and Ebmeier2009; Cullen et al. Reference Cullen, Klimes-Dougan, Muetzel, Mueller, Camchong, Houri, Kurma and Lim2010; Kieseppa et al. Reference Kieseppa, Eerola, Mantyla, Neuvonen, Poutanen, Luoma, Tuulio-Henriksson, Jylha, Mantere, Melartin, Rytsala, Vuorilehto and Isometsa2010; Korgaonkar et al. Reference Korgaonkar, Grieve, Koslow, Gabrieli, Gordon and Williams2011). In line with these reports, our whole-brain analysis revealed reduced FA in the body of the CC in adolescents with clinical depression. Of note, this reduction in FA was coupled with elevated RD and MD, and preserved AD, suggesting that demyelination (i.e. elevated RD and MD) without axonal loss (i.e. preserved AD) could underlie lower FA in the CC. Further analysis also revealed a trending association between reduced FA in the body of the CC and more severe depressive symptoms in patients, which in line with previous reports (Lamar et al. Reference Lamar, Charlton, Morris and Markus2010; Cole et al. Reference Cole, Chaddock, Farmer, Aitchison, Simmons, McGuffin and Fu2012). The CC is a thick interhemispheric fiber tract that connects most of the neocortex and is composed of neural circuits implicated in cognitive and emotional processing (Gazzaniga, Reference Gazzaniga2000; Paul et al. Reference Paul, Lautzenhiser, Brown, Hart, Neumann, Spezio and Adolphs2006; Tamietto et al. Reference Tamietto, Adenzato, Geminiani and de Gelder2007). The CC integrates interhemispheric information but also facilitates inhibition of cortical areas by homologous cortical areas (Bloom & Hynd, Reference Bloom and Hynd2005). Of note, the body of the CC contains fibers that connect to the cingulate, insular and temporal cortices (Seltzer & Pandya, Reference Seltzer and Pandya1986), areas frequently implicated in depression (Thomas et al. Reference Thomas, Drevets, Dahl, Ryan, Birmaher, Eccard, Axelson, Whalen and Casey2001; Mayberg, Reference Mayberg2003; Rosso et al. Reference Rosso, Cintron, Steingard, Renshaw, Young and Yurgelun-Todd2005; Caetano et al. Reference Caetano, Fonseca, Hatch, Olvera, Nicoletti, Hunter, Lafer, Pliszka and Soares2007; Price & Drevets, Reference Price and Drevets2010). Decreased WM integrity of the CC, as indicated by lower FA values, may thus hinder interhemispheric interactions and promote widespread network dysfunction in adolescents with depression. Consistent with this notion, abnormal interhemispheric processing of emotions was previously shown in depression (Davidson & Irwin, Reference Davidson and Irwin1999), and various studies implicate widespread network dysfunction in the pathophysiology of depression (Drevets et al. Reference Drevets, Price and Furey2008; Price & Drevets, Reference Price and Drevets2010). Our finding, together with previous reports of altered CC microstructure in depression, raises the possibility that alterations in CC microstructure might reflect a vulnerability marker for affective disorders. This notion is further supported by a recent study demonstrating altered CC microstructure in healthy adolescents at familial risk for depression (Huang et al. Reference Huang, Fan, Williamson and Rao2011).
In addition to our whole-brain analysis, our ROI-based analysis revealed elevated FA values in the left UF in adolescents with clinical depression. This increase in FA was coupled with elevated AD and reduced RD, suggesting that abnormally increased fiber bundle coherence (i.e. elevated AD) and myelination (i.e. reduced RD) could underlie higher FA in the UF. Various studies implicate abnormal UF microstructure in the pathophysiology of adult and adolescent depression (Sexton et al. Reference Sexton, Mackay and Ebmeier2009; Cullen et al. Reference Cullen, Klimes-Dougan, Muetzel, Mueller, Camchong, Houri, Kurma and Lim2010). The UF serves an integral role in corticolimbic interactions by linking limbic structures such as the amygdala with the OMPFC and sACC (Petrides & Pandya, Reference Petrides and Pandya2002; Kier et al. Reference Kier, Staib, Davis and Bronen2004). The amygdala, OMPFC and sACC are part of a ventral corticolimbic circuit that supposedly evaluates the emotional significance of a stimulus and produces an affective response to that stimulus (Phillips et al. Reference Phillips, Drevets, Rauch and Lane2003a ). We speculate that exaggerated directional coherence within the UF, as reflected by higher FA values, might mediate exaggerated function and functional connectivity in this ventral corticolimbic circuit and impede mood regulation. Indeed, elevated function of the amygdala, OMPFC and sACC on the one hand and augmented bottom-up (amygdala–sACC) and top-down (OMPFC–amygdala) functional interactions on the other are thought to underlie some of the affective symptoms in depression (Phillips et al. Reference Phillips, Drevets, Rauch and Lane2003b ; Stein et al. Reference Stein, Wiedholz, Bassett, Weinberger, Zink, Mattay and Meyer-Lindenberg2007; Price & Drevets, Reference Price and Drevets2010; Masten et al. Reference Masten, Eisenberger, Borofsky, McNealy, Pfeifer and Dapretto2011). Following this perspective, Steffens et al. (Reference Steffens, Taylor, Denny, Bergman and Wang2011) show that, in depressed patients, higher FA in the left UF relates to increased functional connectivity between the amygdala and the ventrolateral PFC (VLPFC), possibly reflecting aberrant top-down emotion regulation. As the VLPFC communicates with the amygdala through the OMPFC (Phillips et al. Reference Phillips, Drevets, Rauch and Lane2003a , Reference Phillips, Ladouceur and Drevets2008), this finding suggests a tight relationship between abnormal UF microstructure and suboptimal corticolimbic interactions. Our finding therefore not only complements previous findings that implicate corticolimbic circuit dysfunction in the pathophysiology of depression, but further clarifies changes in the microstructural properties of a corticolimbic WM pathway strongly implicated in depressive disorders.
There is currently a misconception in interpreting elevated FA in pathological conditions (e.g. affective disorders), as higher FA is thought to reflect healthier WM (Thomason & Thompson, Reference Thomason and Thompson2011). However, some data do suggest that, in specific disease processes or developmental stages, an increase in FA might be a pathologic process caused by neuronal changes (Li et al. Reference Li, Huang, Yang, Li, Wu, Zhang, Lui, Kemp and Gong2011; Thomason & Thompson, Reference Thomason and Thompson2011). As such, higher FA values have been reported in affective disorders (Versace et al. Reference Versace, Almeida, Hassel, Walsh, Novelli, Klein, Kupfer and Phillips2008; Ayling et al. Reference Ayling, Aghajani, Fouche and van der Wee2012) and in other psychiatric conditions (Hubl et al. Reference Hubl, Koenig, Strik, Federspiel, Kreis, Boesch, Maier, Schroth, Lovblad and Dierks2004; Nakamae et al. Reference Nakamae, Narumoto, Shibata, Matsumoto, Kitabayashi, Yoshida, Yamada, Nishimura and Fukui2008; Li et al. Reference Li, Huang, Yang, Li, Wu, Zhang, Lui, Kemp and Gong2011). Our analysis revealed that, in depressed adolescents, reduced FA in the CC was driven by demyelination without axonal loss whereas elevated FA in the UF was mainly driven by exaggerated fiber bundle coherence and myelination. Our findings therefore suggest that adolescent depression may involve a pathologic process in which exaggerated as well as hampered neuroplasticity may give rise to the experience of depression.
Our finding of elevated FA in the UF, however, contrasts with previous reports of reduced FA in the UF in mainly adults with depression (Sexton et al. Reference Sexton, Mackay and Ebmeier2009). We suggest three possible explanations for these discrepancies. First, the typical neuromaturation of the UF follows an inverted U-shaped path, with increasing FA values from early childhood to the early thirties followed by decreasing FA values after the age of 36 (Lebel et al. Reference Lebel, Gee, Camicioli, Wieler, Martin and Beaulieu2012). We speculate that depression could involve accelerated abnormal maturation of the UF, as reflected by an earlier increase in FA during adolescence and an earlier decrease in FA during adulthood. This could partly explain the elevated FA values in our sample of clinically depressed adolescents but may also account for the reduced FA frequently reported in adults with depression. Second, chronic depression in adults and medication use in both adults and adolescents are thought to influence neural microstructure (Sexton et al. Reference Sexton, Mackay and Ebmeier2009; Hulvershorn et al. Reference Hulvershorn, Cullen and Anand2011). These confounding effects make it difficult to replicate findings, and could explain some of the discrepancies between our and previous findings of altered UF microstructure in depression. Finally, in contrast to previous studies, we used ROI-based TBSS to examine UF microstructure. Whereas traditional ROI analyses search for alterations in overall FA within an entire tract, ROI-based TBSS allows voxel-wise analysis of local FA alterations within an entire tract common to all participants (e.g. skeletonized UF tract). Although this difference in analytical approach may yield discrepant findings, TBSS has proved more robust and sensitive in revealing true alterations in FA by minimizing registration errors and partial voluming (Smith et al. Reference Smith, Jenkinson, Johansen-Berg, Rueckert, Nichols, Mackay, Watkins, Ciccarelli, Cader, Matthews and Behrens2006).
Our analysis revealed that WM alterations within the CC and UF were located exclusively in the left hemisphere. Depression studies report abnormal CC and UF microstructure in the left as well as the right hemisphere (Sexton et al. Reference Sexton, Mackay and Ebmeier2009), so a consensus regarding lateralization is still lacking. Nevertheless, interhemispheric imbalance is often observed in depressive disorders. For instance, abnormal interhemispheric processing of emotions accompanies depressed mood (Davidson & Irwin, Reference Davidson and Irwin1999) whereas left hemisphere lesions often induce depressed mood (Braun et al. Reference Braun, Larocque, Daigneault and Montour-Proulx1999; Vataja et al. Reference Vataja, Pohjasvaara, Leppavuori, Mantyla, Aronen, Salonen, Kaste and Erkinjuntti2001). Our findings thus add to the notion that interhemispheric imbalance may be involved in the pathophysiology of depression.
Study limitations and strengths
The cross-sectional nature of this study does not allow for conclusions regarding causality. Hence, we cannot ascertain whether microstructural alterations reported in this study preceded or followed the onset of depressive symptoms. Moreover, because mainly young adolescents were included in our sample, we were not able to examine age-related changes in FA and its potential contribution to the pathophysiology of adolescent depression. Additionally, the small number of adolescent males in our sample did not allow for an adequate test of an age × gender interaction effect on depressive symptoms. Nevertheless, in larger samples girls demonstrate increasing depressive symptoms during adolescence, whereas in boys depressive symptoms tend to decline slightly during adolescence (Wichstrom, Reference Wichstrom1999; Angold et al. Reference Angold, Erkanli, Silberg, Eaves and Costello2002). Longitudinal research in larger samples with a wide age range and a more balanced male-to-female ratio, and DTI studies of individuals at risk for affective disorders, could tackle these limitations. In addition, as socio-economic status and illness duration were not assessed, we cannot rule out the possibility that WM differences may be attributable to these variables. Regarding illness duration, however, it should be noted that the included group did not receive any treatment prior to this study, which might suggest a relatively short illness duration. Finally, in line with other studies examining adolescent depression, depression co-occurred with anxiety in most of our patients, and this might affect the specificity of our results. This co-morbidity, however, is deemed a typical element of clinical depression in adolescents, and exclusion of these patients therefore would have resulted in a highly atypical sample (Zahn-Waxler et al. Reference Zahn-Waxler, Klimes-Dougan and Slattery2000; Costello et al. Reference Costello, Mustillo, Erkanli, Keeler and Angold2003; Cullen et al. Reference Cullen, Gee, Klimes-Dougan, Gabbay, Hulvershorn, Mueller, Camchong, Bell, Houri, Kumra, Lim, Castellanos and Milham2009). Despite these limitations, our study has several strengths that increase the reliability of our results. First, to circumvent potential confounding effects of medication or psychotherapy on WM microstructure, only treatment-naive participants were included in our sample. Second, to allow more accurate group comparison of WM integrity, patients and healthy controls were matched for age, sex and IQ, while including these variables in the analyses as covariates to account for their possible confounding effects. Finally, TBSS was used for both whole-brain and ROI analysis to minimize registration errors and partial voluming, thus allowing more robust and sensitive investigation of WM microstructure.
Conclusions
In summary, adolescents with clinical depression showed WM microstructural alterations within pathways facilitating cognitive and emotional functioning. These findings add to converging lines of evidence implicating WM abnormalities in the pathophysiology of depression, and suggest that these abnormalities are already present early in the course of the disorder. For a deeper understanding of WM architecture in depressive disorders, it would be both important and interesting to examine whether WM alterations predict susceptibility, treatment response and risk of relapse in depressive disorders. Our group will address these questions in subsequent studies using longitudinal data from the EPISCA cohort.
Supplementary material
For supplementary material accompanying this paper visit http://dx.doi.org/10.1017/S0033291713003000.
Acknowledgments
We thank all participants involved in the study and M. Versluis and L. ter Beek for their contributions. We also thank the following participating centers for financial support: the Department of Child and Adolescent Psychiatry of GGZ Rivierduinen, the LUMC Departments of Psychiatry and Radiology, and the Leiden Institute for Brain and Cognition. Part of this research was supported by the Netherlands Organization for Scientific Research – National Initiative Brain and Cognition (NWO-NIHC, project no. 056-23-011).
Declaration of Interest
None.