Introduction
Fluorapophyllite-(NH4), ideally NH4Ca4(Si8O20)F⋅8H2O, is a new member of apophyllite group. It was found at the Vechec andesite quarry located 2.8 km SW of the Vechec village, Vranov nad Topľou Co., Prešov Region, Slovak Republic.
Fluorapophyllite-(NH4) is named according to the current nomenclature scheme of apophyllite-group minerals (Hatert et al., Reference Hatert, Mills, Pasero and Williams2013), and is an NH4 dominant analogue of fluorapophyllite-(K), KCa4Si8O20F⋅8H2O, fluorapophyllite-(Na), NaCa4Si8O20F⋅8H2O and fluorapophyllite-(Cs), CsCa4Si8O20F⋅8H2O (Dunn and Wilson, Reference Dunn and Wilson1978; Dunn et al., Reference Dunn, Rouse and Norberg1978; Hatert et al., Reference Hatert, Mills, Pasero and Williams2013; Agakhanov et al., Reference Agakhanov, Pautov, Kasatkin, Karpenko, Sokolova, Day, Hawthorne, Muftakhov, Pekov, Cámara and Britvin2019). The new mineral and the name has been approved by the Commission on New Minerals, Nomenclature and Classification of the International Mineralogical Association (IMA2019-083, Števko et al., Reference Števko, Sejkora, Plášil, Dolníček and Škoda2020). The holotype specimen of fluorapophyllite-(NH4) is deposited in the collections of the Department of Mineralogy and Petrology, National Museum in Prague, Cirkusová 1740, 19300 Praha 9, Czech Republic under the catalogue number P1P 44/2019.
Occurrence
The first (holotype) samples with fluorapophyllite-(NH4) were collected on September 30, 2018, by one of the authors (MŠ not MS) from the fresh blast pile on the lowest level of the Vechec andesite quarry (48°51'9.58"N, 21°36'12.10"E). More specimens with fluorapophyllite-(NH4) were later collected at the same level of the Vechec quarry in April and June of 2019.
The Vechec quarry is situated in the central neck of the parasitic andesite volcano of Miocene (Sarmatian) age, which is a part of the Makovica stratovolcano in the Slanské vrchy Mts. volcanic range (Kaličiak, Reference Kaličiak1991). This quarry is well-known for the occurrence of tridymite, cristobalite, calcite, aragonite and various zeolites (mainly phillipsite-Ca, phillipsite-K, chabazite-Ca and stilbite-Ca), which fill cavities and fractures in pyroxene andesite (Ďuďa et al., Reference Ďuďa, Černý, Kaličiak, Kaličiaková, Tözsér, Ulrych and Veselovský1981, Reference Ďuďa, Kotuľák and Levendovský2001; Števko et al., Reference Števko, Timko, Myšľan and Bacher2019). The Vechec quarry andesites also contain numerous xenoliths of Neogene and Paleogene sedimentary as well as metamorphic basement rocks with very variable composition. An interesting association of minerals was described from these xenoliths including quartz, corundum, cordierite–sekaninaite, sanidine, spinel, magnesioferrite, arsenopyrite, pyrite, pyrrhotite, chlorites, diopside, richterite, ferrisepiolite, plagioclase, phlogopite, ilmenite, calcite, ankerite, dolomite, siderite, wollastonite, gyrolite, tobermorite, jennite, fluorapophyllite-(K), stilbite-Na, titanite, chlorapatite and graphite (e.g. Ďuďa et al., Reference Ďuďa, Černý, Kaličiak, Kaličiaková, Tözsér, Ulrych and Veselovský1981, Reference Ďuďa, Scharmová and Černý1993, Reference Ďuďa, Kotuľák and Levendovský2001; Košuth, Reference Košuth1999; Košuth and Marcinčáková, Reference Košuth and Marcinčáková2010; Marcinčáková and Košuth, Reference Marcinčáková and Košuth2011; Myšľan et al., Reference Myšľan, Ozdín and Timko2018).
Fluorapophyllite-(NH4) occurs in cavities of a quartz–illite–saponite–tobelite xenolith embedded in pyroxene andesite. The xenolith containing the holotype specimens was 35 cm × 20 cm × 12 cm with an irregular shape. Minerals associated with fluorapophyllite-(NH4) are calcite, tridymite, pyrite, chabazite-Ca and heulandite-Ca. Fluorapophyllite-(NH4) is a hydrothermal mineral, which was formed at the late stage of an (auto)-hydrothermal alteration of the xenoliths together with calcite and zeolites. The protolith of the xenolith was a basement sedimentary rock (most probably from the underlying Neogene evaporite sequences), which contained minor amounts of NH4.
Physical and optical properties
Fluorapophyllite-(NH4) occurs as clusters or crystalline crusts consisting of individual, well developed crystals up to 4 mm in size (Figs 1, 2) on the surface of the cavities, which occurs mostly in the central part of the xenolith. Crystals of fluorapophyllite-(NH4) are mostly prismatic with pyramidal or flat terminations, with the crystals forms: {110}, {101} and {001}. Individual crystals are frequently grouped in aggregates or hypo-parallel groups. Fluorapophyllite-(NH4) is colourless to light pink, translucent, with a white streak, a vitreous to pearly lustre and it is non-fluorescent in shortwave and longwave ultraviolet light. The Mohs hardness is estimated at ~4½ to 5 based on scratch tests and by analogy to other apophyllite-group minerals. Fluorapophyllite-(NH4) crystals show perfect cleavage on {001} and are brittle; fracture is irregular. A density of 2.325 g cm–3 was calculated using the empirical formula and unit-cell volume refined from single-crystal X-ray diffraction (SCXRD) data. Fluorapophyllite-(NH4) is uniaxial (+) with refractive indices (λ = 589 nm) ω = 1.5414(5) and ɛ = 1.5393(8) without apparent pleochroism. The Gladston–Dale compatibility (Mandarino, Reference Mandarino2007), 1-(K P/K C) is 0.000 (superior) using the ideal formula, and –0.005 (superior) using the empirical formula.

Fig. 1. Clusters of pinkish crystals of fluorapophyllite-(NH4) from the Vechec quarry. Field of view is 14 mm. Photo: Pavel Škácha, specimen in private collection.

Fig. 2. Pyramidal crystal of fluorapophyllite-(NH4) associated with calcite. Field of view is 6.2 mm. Photo: Pavel Škácha, specimen in private collection.
Raman and infrared spectroscopy
The Raman spectrum of fluorapophyllite-(NH4) (Fig. 3) was collected in the range 4000–40 cm–1 using a DXR dispersive Raman Spectrometer (Thermo Scientific) mounted on a confocal Olympus microscope. The Raman signal was excited by an unpolarised red 633 nm He–Ne gas laser and detected by a CCD detector. The experimental parameters were: 100× objective, 60 s exposure time, 100 exposures, 50 μm pinhole spectrograph aperture and 8 mW laser power level. The spectra were repeatedly acquired from different grains in order to obtain a representative spectrum with the best signal-to-noise ratio. The eventual thermal damage of the measured point was excluded by visual inspection of the excited surface after measurement, by observation of possible decay of spectral features in the start of excitation and checking for thermal downshift of Raman lines. The instrument was set up by a software-controlled calibration procedure using multiple neon emission lines (wavelength calibration), multiple polystyrene Raman bands (laser-frequency calibration) and standardised white-light sources (intensity calibration). Spectral manipulations were performed using the Omnic 9 software (Thermo Scientific).

Fig. 3. Raman spectrum of fluorapophyllite-(NH4) from the Vechec quarry, full range (split at 2000 cm-1).
The main bands observed are (in wavenumbers): 3565, 3556, 3540, 3204, 3119, 3034, 1689, 1462, 1114, 1059, 1008, 854, 792, 763, 662, 586, 551, 486, 431, 373, 340, 321, 300, 271, 229, 208, 165, 141, 108, 91 and 54 cm–1. The presence of the ammonium cation is shown by a weak band at 1462 cm–1 (bending vibrations of NH4). The N–H stretching vibrations together with the O–H stretching vibrations of H2O molecules were observed in the range 3600–2800 cm–1. A weak band at 1689 cm–1 is connected with ν2 (δ) H2O-bending vibrations of hydrogen-bonded water molecules. The complex bands below 1200 cm–1 are attributed to ν (Si–O) vibrations, libration and translation modes of water molecules, Ca2+ translation modes and framework vibrations and their assignment is not unambiguous (Adams et al., Reference Adams, Armstrong and Best1981).
The infrared (IR) vibrational spectrum of fluorapophyllite-(NH4) was recorded by the attenuated total reflection (ATR) method with a diamond cell on a Nicolet iS5 spectrometer. Spectra over the 4000–400 cm–1 range were obtained by the co-addition of 64 scans with a resolution 4 cm–1 and a mirror velocity of 0.4747 cm/s. Spectra were co-added to improve the signal-to-noise ratio.
The main bands in the infrared spectrum of fluorapophyllite-(NH4) from the Vechec quarry are indicated in Fig. 4. The presence of an (NH4) group in fluorapophyllite-(NH4) is confirmed by a distinct band at 1464 cm–1 with a shoulder at 1440 cm–1 connected with bending vibrations of the ammonium cation. The possible representation of N–H stretching vibrations in the range 3300–3100 cm–1 is overlapped by O–H stretching vibrations of H2O molecules observed in the range 3600–2800 cm–1. A band at 1679 cm–1 is connected with ν2 (δ) H2O bending vibrations of hydrogen-bonded water molecules. The bands below 1200 cm–1 are attributed to ν (Si–O) vibrations, libration and translation modes of water molecules, Ca2+ translation modes and framework vibrations and their assignment is not unambiguous (Adams et al., Reference Adams, Armstrong and Best1981).

Fig. 4. Infrared spectrum of fluorapophyllite-(NH4) from the Vechec quarry, full range (split at 2000 cm-1).
Chemical composition
Quantitative chemical analyses (11 points) of fluorapophyllite-(NH4) were performed at the Department of Mineralogy and Petrology, National Museum, Prague, Czech Republic on the Cameca SX100 electron microprobe (EMPA) equipped with five wavelength-dispersive spectrometers (WDS). Analytical conditions were the following: 15 kV accelerating voltage, 5 nA beam current, 10 μm beam diameter and WDS mode. Concentrations of other measured elements (Al, Ba, Cl, Cs, Fe, Mn, P, Rb, S and Sr) were below detection limits (ca. 0.03 to 0.05 wt.%). Raw X-ray intensities were corrected for matrix effects with a φ(ρz) algorithm (Pouchou and Pichoir, Reference Pouchou, Pichoir, Heinrich and Newbury1991). Due to the limited amount of material available, the H2O content was not measured directly and is instead calculated by stoichiometry with respect to the crystal structure; the presence of water was also confirmed by spectroscopic (Raman and infrared) study. Analytical data for fluorapophyllite-(NH4) as well as standards used are given in Table 1.
Table 1. Chemical composition (in wt.%) of fluorapophyllite-(NH4).

H2O* – calculated on the basis 8 H2O and F + OH = 1 apfu
S.D. = standard deviation
The empirical formula of fluorapophyllite-(NH4) based on 29 O + F atoms per formula unit (apfu) is [(NH4)0.55K0.32Na0.07Ca0.06]Σ1.00(Ca4.01Mg0.02)Σ4.03Si7.97O20[F0.84(OH)0.16]Σ1.00⋅8H2O. The ideal formula is NH4Ca4(Si8O20)F⋅8H2O, which requires (NH4)2O 2.94, CaO 25.31, SiO2 54.24, F 2.14, H2O 16.27, total 100 wt.%. Some of the studied crystal fragments of fluorapophyllite-(NH4) from the Vechec quarry show a wide range of NH4–K (Fig. 5) and F–OH (Fig. 6) substitutions. Importantly, in the fragment used for the SCXRD study, the NH4 content (0.50–0.59 apfu) clearly predominates over the K content (0.28–0.42 apfu) with an associated significant F content (0.80–0.95 apfu), which is consistent with the resulting crystal chemical formula.

Fig. 5. The NH4 (pfu) vs. K (apfu) plot for studied fluorapophyllite-(NH4) from the Vechec quarry.

Fig. 6. The NH4/(NH4+K) vs. F (apfu) plot for studied fluorapophyllite-(NH4) from the Vechec quarry.
X-ray crystallography and structure refinement
Powder X-ray diffraction data of fluorapophyllite-(NH4) were recorded using a Bruker D8 Advance diffractometer equipped with a solid-state LynxEye detector and secondary monochromator producing CuKα radiation housed at the Department of Mineralogy and Petrology, National Museum, Prague, Czech Republic. The instrument was operating at 40 kV and 40 mA. In order to minimise background, powder samples were placed on the surface of a flat silicon wafer. Powder patterns were collected in Bragg–Brentano geometry in the range 3–75°2θ CuKα, with 0.01° step and counting time of 30 s per step (total duration of experiment was ca. 3 days). The positions and intensities of diffractions were found and refined using the Pearson VII profile-shape function of the ZDS program package (Ondruš, Reference Ondruš1993) and the unit-cell parameters were refined by the least-squares program of Burnham (Reference Burnham1962). The powder X-ray diffraction data of fluorapophyllite-(NH4) presented in Table 2 show good agreement with the pattern calculated from the structure determination. Unit-cell parameters of fluorapophyllite-(NH4) refined for the tetragonal space group P4/mnc are: a = 8.992(2) Å, c = 15.800(3) Å, V = 1277.5(6) Å3 and Z = 2.
Table 2. Powder X-ray diffraction data of fluorapophyllite-(NH4) from the Vechec quarry; the seven strongest diffractions are reported in bold.

Single-crystal X-ray studies of fluorapophyllite-(NH4) were carried out using a Rigaku SuperNova single-crystal diffractometer with an Atlas S2 CCD detector and monochromatised MoKα radiation from the microfocus X-ray tube. The fragment investigated by single-crystal X-ray studies was separated from a polished section of an aggregate pre-analysed by means of electron microprobe. The CrysAlis Pro software package was used for processing the structure data. The empirical absorption correction (multi-scan) was applied to the data in CrysAlis during the data reduction process. The Jana2006 program (Petříček et al., Reference Petříček, Dušek and Palatinus2014) was used for the refinement of the structure retrieved from the diffraction data using the SHELXT program (Sheldrick, Reference Sheldrick2015). All non-hydrogen atoms were refined with anisotropic displacement parameters while the water molecule H atoms (as located in difference-Fourier maps) were refined using a riding model with soft restraints on the O–H distances and with U iso values set at 1.2× the U eq of the bonded O atom (O4). Data collection and refinement details are given in Table 3, atom coordinates and displacement parameters in Table 4, respectively, and selected bond distances in Table 5. Results of bond-valence analysis are given in Table 6. The crystallographic information files have been deposited with the Principal Editor of Mineralogical Magazine and are available as Supplementary material (see below).
Table 3. Data collection and structure refinement details for fluorapophyllite-(NH4).

Table 4. Atom coordinates, displacement parameters (Å2) and occupation factors for the structure of fluorapophyllite-(NH4).

# constrained to 0.02 Å2.
Table 5. Selected interatomic distances (in Å) in the structure of fluorapophyllite-(NH4).
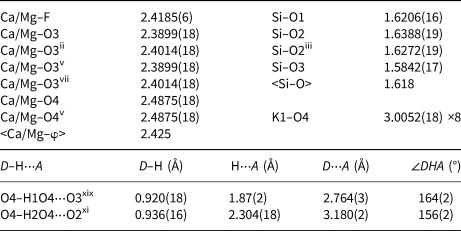
Symmetry codes: (i) –x, –y, z; (ii) –y, x, z; (iii) y, –x, z; (iv) –x, –y, –z; (v) x, y, –z; (vi) y, –x, –z; (vii) –y, x, –z; (xi) –y+½, –x+½, –z+½; (xiii) –x+½, y–½, z+½; (xix) y, –x + 1, z.
D – donor; A – acceptor
Table 6. Bond-valence analysis for fluorapophyllite-(NH4)*.

*All values in valence units; bond-valence parameters used: Ca2+–O, Mg2+–O, K+–O, Si4+–O and H+–O from Gagné and Hawthorne (Reference Gagné and Hawthorne2015), NH4+–O from Garcia-Rodriguez et al. (Reference García-Rodríguez, Rute-Pérez, Piñero and González-Silgo2000), Mg2+–F from Brown and Altermatt (Reference Brown and Altermatt1985)
The SCXRD study of fluorapophyllite-(NH4) shows it to be isostructural with fluorapophyllite-(K) (Stahl, Reference Ståhl1993), but with the mineral defining 8-coordinate cation site now occupied predominantly by NH4+. Given the significantly mixed nature of this site as indicated by the EMPA, refinement of the site scattering based on N and K (as a proxy for the non-ammonium content) was considered appropriate and confirmed the predominance of NH4 over (significant) K, while still allowing for the minor amounts of Na and Ca, and was in good agreement with the EMPA. The structure of fluorapophyllite-(NH4) is displayed in Fig. 7. The site occupied by K+ in fluorapophyllite-K was found to be occupied by nitrogen and therefore refined as a mixed site. The site-scattering refinement confirmed the predominance of N over K at the site. Unfortunately, it was not possible to localise H atoms associated with the NH4 moiety from the difference-Fourier maps, probably due to some degree of freedom (rotation) of NH4 in the structure, bonded by the relatively weak H bonds (corresponding Donor⋅⋅⋅Acceptor distance, N⋅⋅⋅O, ~3 Å).

Fig. 7. Crystal structure of fluorapophyllite-(NH4) (a) viewed down b, and (b) down c showing the coordination of interlayer cations and part of hydrogen bonding network. Si tetrahedra are green, Ca atoms plum, N1/K1 site dark blue, F atoms are light green (located at the origin), O atoms are red, H atoms are grey and corresponding H-bonds (emanating from molecular H2O) are displayed as blue dashed lines. Unit-cell edges are outlined by black lines; interatomic distance is given in Å.
Relationship to the known species
Fluorapophyllite-(NH4), NH4Ca4(Si8O20)F⋅8H2O, is the NH4 dominant analogue of fluorapophyllite-(K), KCa4Si8O20F⋅8H2O, fluorapophyllite-(Na), NaCa4Si8O20F⋅8H2O and fluorapophyllite-(Cs), CsCa4Si8O20F⋅8H2O, and belongs to the apophyllite group (Dunn and Wilson, Reference Dunn and Wilson1978; Dunn et al., Reference Dunn, Rouse and Norberg1978; Hatert et al., Reference Hatert, Mills, Pasero and Williams2013; Agakhanov et al., Reference Agakhanov, Pautov, Kasatkin, Karpenko, Sokolova, Day, Hawthorne, Muftakhov, Pekov, Cámara and Britvin2019). Marriner et al. (Reference Marriner, Tarney and Langford1990) discussed the existence of an NH4-enriched variety (‘ammonian apophyllite’) of fluorapophyllite-(K) in samples from Mexico, with up to 25% of stoichiometric K+ replaced by NH4+. A comparison of the physical properties of valid mineral species of the apophyllite group is shown in Table 7.
Table 7. Comparison of the physical properties for a valid members of the apophyllite group.

References: [1] this work; [2] Chao (1971); [3] Ståhl et al. (1987); [4] Marriner et al. (1990); [5] Anthony et al. (1995); [6] Matsueda et al. (1981); [7] Agakhanov et al. (2019); [8]Dunn et al. (1978).
In the Nickel–Strunz classification system Fluorapophyllite-(NH4) fits in subdivision 9.EA.15 (Phyllosilicates with single nets of tetrahedra with 4-, 5-, (6-), and 8-membered rings.
Supplementary material
To view supplementary material for this article, please visit https://doi.org/10.1180/mgm.2020.44
Acknowledgements
The authors appreciate the helpful comments of Stuart Mills, an anonymous reviewer and Peter Leverett. Pavel Škácha is acknowledged for photography. The study was supported financially by the Ministry of Culture of the Czech Republic (long-term project DKRVO 2019-2023/1.II.b; National Museum, 00023272). JP acknowledges the support through the project No. LO1603 under the Ministry of Education, Youth and Sports National sustainability program of the Czech Republic.