Introduction
The slags from the famous Lavrion ancient silver and lead mines in Attiki, Greece, were dumped after smelting into the sea where their components have been reacting with the sea water for more than two thousand years, forming various mineral phases including many rare and endemic species (Gelaude et al., Reference Gelaude, van Kalmthout and Rewitzer1996; Kolitsch et al., Reference Kolitsch, Rieck, Brandstätter, Schreiber, Fabritz, Blaß and Gröbner2014). All the phases formed in the slags are not currently considered as minerals by the International Mineralogical Association because of their anthropogenic (or ‘semi-anthropogenic’) origin (Nickel and Grice, Reference Nickel and Grice1998).
Hydrocerussite Pb3(OH)2(CO3)2 is a rather common mineral found in more than a hundred different locations all over the world (https://www.mindat.org/). Structural information for a powder synthetic material was obtained by Martinetto et al. (Reference Martinetto, Anne, Dooryhée, Walter and Tsoucaris2002) and crystal structure of hydrocerussite from Merehead quarry, Great Britain was reported very recently by Siidra et al., (Reference Siidra, Nekrasova, Depmeier, Chukanov, Zaitsev and Turner2018a). Hydrocerussite from the Lavrion slags has been well known for a long time. Unit-cell parameters (a = 5.24–5.25 Å and c = 29.38–29.50 Å) were determined for the lamellar crystals which are visually similar to hydrocerussite (Kokkoros and Vassiliadis, Reference Kokkoros and Vassiliadis1953; Kolitsch et al., Reference Kolitsch, Rieck, Brandstätter, Schreiber, Fabritz, Blaß and Gröbner2014). Both of the previous works identified this phase as ‘hydrocerussite- or plumbonacrite-related’ but different from ‘classic’ hydrocerussite. According to Kokkoros and Vassiliadis (Reference Kokkoros and Vassiliadis1953), this non-classic phase is much more common than hydrocerussite in the Pb-rich slags of Lavrion. However its crystal structure has also not been solved. A slag phase with the unit-cell parameters identical to those of abellaite NaPb2(OH)(CO3)2 (Ibáñez-Insa et al., Reference Ibáñez-Insa, Elvira, Llovet, Pérez-Cano, Oriols, Busquets-Masó and Hernández2017) is also rather common in Lavrion slags (Kolitsch et al. Reference Kolitsch, Rieck, Brandstätter, Schreiber, Fabritz, Blaß and Gröbner2014).
Herein, we report data on NaPb5(CO3)4(OH)3, a hydrocerussite-related lead hydroxycarbonate from Lavrion ancient slags, with a surprisingly complex crystal structure based upon structurally and chemically different electroneutral blocks.
Occurrence
The NaPb5(CO3)4(OH)3 phase described in this paper forms aqua-transparent, colourless lamellar crystals up to 0.01 mm × 0.3 mm × 0.3 mm (similar to that shown in Fig. 1) in cavities within a pebble of ancient marine slag collected in the Pacha Limani area of the Lavrion mining district (37°40'36''N, 24°3'6''E). It is associated with aragonite and lead. The hydrocerussite-related phase was formed during the 2500 y period that the slag was exposed to seawater, causing reactions with the Pb-rich material in the slag.

Fig. 1. Typical lamellar transparent colourless crystals of the Na,Ca-bearing hydrocerussite-related phase from Pacha Limani, Lavrion, Greece. Field of view width is 0.6 mm. Photo and collection: S. Wolfsried.
Chemical composition
The chemical composition of the NaPb5(CO3)4(OH)3 phase was studied using a Jeol JSM-6480LV scanning electron microscope equipped with an INCA-Wave 500 wavelength-dispersive spectrometer (Laboratory of Analytical Techniques of High Spatial Resolution of Faculty of Geology, Lomonosov Moscow State University), with an acceleration voltage of 20 kV, a beam current of 10 nA, and a 5 µm beam diameter. The following standards were used: NaCl (Na), clinopyroxene (Ca) and PbTe (Pb). Contents of other elements with atomic numbers higher than oxygen are below detection limits. The chemical composition of the phase studied is: Na 0.89, Ca 1.44, Pb 78.05, Ccalc 3.61, Ocalc 18.04, Hcalc 0.19, total 102.22 wt.%. Contents of light elements (C, O and H) were calculated by the stoichiometry for the empirical formula calculated on the basis of Pb + Na + Ca = 6 atoms per formula unit, in accordance with the structural data (see below): Na0.51Ca0.48Pb5.01(CO3)4(OH2.51O0.49)Σ3.
Powder X-ray diffraction data
Powder X-ray diffraction (XRD) data were obtained using a Rigaku R-AXIS Rapid II single-crystal diffractometer equipped with cylindrical image plate detector using Debye-Scherrer geometry (with d = 127.4 mm), after crushing the crystal fragments used for the single-crystal XRD analysis. Data (in Å for CoKα) are given in Table 1. The unit-cell parameters calculated for a hexagonal unit cell, space group P63/mmc, are: a = 5.2514(7), c = 29.428(6) Å, V = 702.8(2) Å3 and Z = 2.
Table 1. Powder X-ray diffraction data for NaPb5(CO3)4(OH)3 from Lavrion.
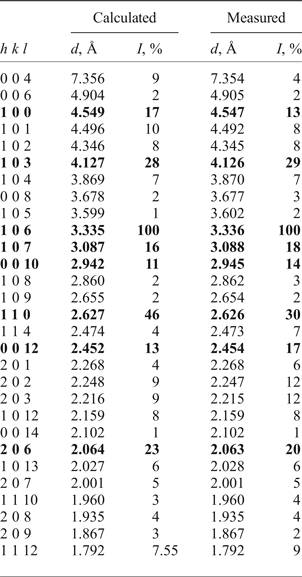
The eight strongest lines are given in bold.
Infrared spectroscopy
In order to obtain infrared (IR) absorption spectra, powdered samples of the phase NaPb5(CO3)4(OH)3 from Lavrion and hydrocerussite from Merehead quarry, Somerset, England (Fig. 2), were mixed with anhydrous KBr, pelletized, and analysed using an ALPHA FTIR spectrometer (Bruker Optics) at a resolution of 4 cm–1 and 16 scans were collected for each spectrum. The IR spectrum of an analogous pellet of pure KBr was used as a reference.

Fig. 2. IR spectra of NaPb5(CO3)4(OH)3 from Lavrion (a) and hydrocerussite from the Merehead quarry, Somerset, England (b). IR spectrum of synthetic abellaite analogue (c) drawn based on data of Belokoneva et al. (Reference Belokoneva, Al’-Ama, Dimitrova, Kurazhkovskaya and Stefanovich2002).
The IR spectrum of NaPb5(CO3)4(OH)3 contains two bands of O–H-stretching vibrations of OH groups located at 3529 and 3515 cm–1. These values are intermediate between those of hydrocerussite and abellaite, which corresponds to intermediate strengths of H bonds (Libowitzky, Reference Libowitzky1999). The weak peak at 1735 cm–1 is a combination band of the stretching and bending vibrations of ${\rm CO}_{3}^{2-} $ groups. The strong band at 1431 cm–1 with the shoulder at 1365 cm–1 corresponds to the asymmetric stretching vibrations of carbonate groups. The presence of the weak band of symmetric stretching vibrations of carbonate groups at 1044 cm–1 (non-degenerate mode) reflects weak distortion of the CO3 triangle. The bands at 848 and 834 cm–1 are assigned to the in-plane bending vibrations of
${\rm CO}_{3}^{{2-}} $ groups. The band at 683 cm–1 corresponds to the out-of-plane bending vibrations of
${\rm CO}_{3}^{{2-}} $. The absorptions below 500 cm–1 are the lattice modes involving Pb–O and Na–O stretching and CO3 librational vibrations.
As can be seen from Fig. 2, the IR spectrum of NaPb5(CO3)4(OH)3 is close to that of abellaite in the region of asymmetric stretching vibrations of carbonate groups and similar to the IR spectrum of hydrocerussite in the region of O–C–O bending vibrations.
Crystal structure
Experiment
The single-crystal study of lead hydroxycarbonate compounds is challenging because of the lamellar habit of crystals, high X-ray absorption and strong pseudosymmetry. Thus, the choice of crystal for XRD study is important. In this work, we have used several thin platy crystals of NaPb5(CO3)4(OH)3. Only the crystals giving the best results are reported below. Crystals of the title compound were mounted on thin glass fibres and placed on a Bruker DUO APEX II CCD four-circle diffractometer with a Mo-IμS micro-focus tube at 50 kV and 40 mA. More than a hemisphere of XRD data was collected with frame widths of 0.5° in ω, and with 90 s counting time for each frame. The data were integrated and corrected for absorption using an empirical ellipsoidal model using the APEX and XPREP Bruker programs. The observed systematic absences for NaPb5(CO3)4(OH)3 were consistent with the space group P $\bar 3$1c. In this space group, light atoms (C and O) could not be refined anisotropically. The obtained structure model was transformed to the space group P63/mmc using the ADDSYM algorithm incorporated in the PLATON program package (le Page, Reference le Page1987; Spek, Reference Spek2003). The structure was refined successfully with the use of the SHELX software package (Sheldrick, Reference Sheldrick2015). Structure refinement in this group resulted in the crystallographic agreement index R 1 = 0.047 (Table 2). The final coordinates and anisotropic displacement parameters of atoms are given in Table 3 and selected interatomic distances in Table 4. Hydrogen atom positions were not localized. The crystallographic information files have been deposited with the Principal Editor of Mineralogical Magazine and are available as Supplementary material (see below).
Table 2. Crystallographic data and structure-refinement details for NaPb5(CO3)4(OH)3 from Lavrion.

Table 3. Atomic coordinates and displacement parameters (Å2) for NaPb5(CO3)4(OH)3 from Lavrion.

*Site occupancy factor = ⅙; ** Na0.51Ca0.49; ***(OH)0.51O0.49.
Table 4. Selected interatomic distances in the crystal structure of NaPb5(CO3)4(OH)3 from Lavrion.

Cation and anion coordination
The structure contains three symmetrically independent Pb sites, one Na site and two C sites (Fig. 3, Table 3). The Pb1 and Pb3 atoms are coordinated in one coordination hemisphere by seven O atoms, taking into account strong Pb2+–O bonds shorter than 3.1 Å. In addition, both of the atoms form three long Pb2+–O bonds >3.1 Å each in the opposite coordination hemisphere. This type of coordination geometry of the Pb2+ cation can be described as ‘hemidirected’ (Shimoni-Livny et al., 1998). The asymmetric coordination of the Pb1 and Pb3 atoms in NaPb5(CO3)4(OH)3 indicates a strong degree of the stereochemical activity of the 6s 2 lone pairs of electrons. The coordination sphere of the split Pb2 site (site occupancy factor = ⅙) demonstrates rather uniform distribution of shorter and stronger bonds and can be described as ‘holodirected’. ‘Holodirected’ coordination for Pb2+ cations is much less preferable than ‘hemidirected’ (with shorter and stronger Pb–O bonds concentrated in one coordination hemisphere) in oxysalt compounds.

Fig. 3. Cation site coordination environments in the structure of NaPb5(CO3)4(OH)3. The split Pb2 site is represented as fully ordered for clarity and bond distances are not shown.
The splitting of the Pb2 site makes bond-valence analysis of the structure of NaPb5(CO3)4(OH)3 non-applicable. Identical positional disorder for the Pb2 site was observed in the structure of the synthetic analogue of hydrocerussite: Pb3(OH)2(CO3)2 (Martinetto et al., Reference Martinetto, Anne, Dooryhée, Walter and Tsoucaris2002) determined by powder XRD utilizing synchrotron radiation. It is also identical to the Pb2 site in the hydrocerussite from Merehead quarry, England (Siidra et al., Reference Siidra, Nekrasova, Depmeier, Chukanov, Zaitsev and Turner2018a).
One symmetrically independent Na site is coordinated by six O atoms thus forming a trigonal prism. Ca2+ detected by the electron microprobe analysis (see above) substitutes Na+ in the Na site. Occupancy of Na site (Table 3) was fixed in agreement with microprobe data on the last stages of refinement. In order to maintain charge balance, substitution of Na+ for Ca2+ requires some compensation, which we hypothesize to be OH– → O2– on the OH2 site. A similar charge balance mechanism was described recently in the crystal structure of grootfonteinite Pb3O(CO3)2 (Siidra et al., Reference Siidra, Jonsson, Chukanov, Nekrasova, Pekov, Depmeier, Polekhovsky and Yapaskurt2018b). The NaO6 coordination polyhedron contains six equal Na–O bonds of 2.450(14) Å each. The coordination of (Na,Ca) in NaPb5(CO3)4(OH)3 is typical. In general, coordination polyhedron of the Na site is similar to Pb2 (Fig. 3), ignoring the split of the latter. However, the Pb2–OH1 bonds (Table 4) in the equatorial plane of the coordination sphere must be taken into account for bond-valence calculation in NaPb5(CO3)4(OH)3, whereas the Na–OH2 distances (3.033(6) Å × 3 with 0.03 vu each) can be ignored, as they do not contribute significantly to the charge saturation of the Na+ cations.
The carbonate triangles are very similar and show typical bond lengths, with a <C–O> of 1.277(13) and 1.274(13) Å for C1- and C2-centred triangles, respectively.
Coordination of OH1 and OH2 sites attributed to hydroxyl groups is different. The OH1 site forms one short OH1–Pb1 bond (0.62 vu) and three weaker OH1–Pb2 bonds. In contrast, two short and strong OH2–Pb3 bonds (0.66 vu each) are formed for the OH2 site (Fig. 3). Coordination of the OH1 site is identical to ones in hydrocerussite from Merehead quarry and the powder synthetic analogue of this mineral reported in Martinetto et al. (Reference Martinetto, Anne, Dooryhée, Walter and Tsoucaris2002), whereas coordination environments of OH2 are similar in abellaite NaPb2(CO3)2(OH) (Krivovichev and Burns, Reference Krivovichev and Burns2000; Belokoneva et al., Reference Belokoneva, Al’-Ama, Dimitrova, Kurazhkovskaya and Stefanovich2002; Ibáñez-Insa et al., Reference Ibáñez-Insa, Elvira, Llovet, Pérez-Cano, Oriols, Busquets-Masó and Hernández2017; Siidra et al., Reference Siidra, Jonsson, Chukanov, Nekrasova, Pekov, Depmeier, Polekhovsky and Yapaskurt2018b). Note, the OH1 site demonstrates additional static disorder in the synthetic analogue of hydrocerussite Pb3(OH)2(CO3)2 (Martinetto et al., Reference Martinetto, Anne, Dooryhée, Walter and Tsoucaris2002), whereas our data do not reveal this phenomenon in NaPb5(CO3)4(OH)3.
Structure description
PbOn (with Pb–O bonds < 3.1 Å) and NaO6 polyhedra share common oxygen atoms with CO3 triangles thus forming two types of the two-dimensional (2D) blocks shown in Fig. 4. The first block is formed by Pb1, Pb2, C1, O1 and OH1 atoms, whereas Pb3, Na, C2, O2 and OH2 atoms build the second block (Table 3). Notionally, these blocks can be decomposed into three separate sheets (Fig. 5). The two outside sheets in every block contain the Pb atoms and CO3 groups in a 1:1 ratio and are fully ordered thus giving electroneutral [PbCO3]0. Each [PbCO3]0 sheet consists of Pb2+ cations, coordinated by three CO3 triangles each, to form an infinite two-dimensional ‘trigonal’ mesh pattern perpendicular to the c axis. For the sake of discussion these sheets will be given the generic designation C, whereby the symbol refers to cerussite, PbCO3, in the crystal structure of which such sheets occur. Two symmetrically independent C1 and C2 sheets are formed in the structure of NaPb2(CO3)2(OH). The stereochemically active lone electron pairs on Pb2+ cations of Pb1 and Pb3 sites point to each other (Fig. 4). The lone electron pairs on the cations behave as soft ligands that associate together in the interblock space. Sheets of composition [Pb(OH)2]0 formed by the Pb2 and OH1 sites are sandwiched between the first pair of C-type sheets. We denote these sheets as LHO (LHO = lead hydroxide, according to the Pb(OH)2 composition). Adjacent C1 sheets of the ···C1–LHO–C1*··· block are related by inversion, and this relationship is indicated by a star symbol: C1 → C1* (Figs 4, 5). The resulting [Pb3(OH)2(CO3)2]0 block with the positional disorder of Pb atoms in the LHO sheet is identical to the one in the structure of hydrocerussite Pb3(OH)2(CO3)2.

Fig. 4. General projections of the crystal structures of (a) NaPb5(CO3)4(OH)3 from Lavrion (weak Pb–O bonds are shown by dashed lines), (b) hydrocerussite from Merehead (Siidra et al., Reference Siidra, Nekrasova, Depmeier, Chukanov, Zaitsev and Turner2018a) and (c) abellaite (Ibáñez-Insa et al., Reference Ibáñez-Insa, Elvira, Llovet, Pérez-Cano, Oriols, Busquets-Masó and Hernández2017) (modified and drawn for the structure in P63/mmc). The c parameter values are highlighted for each structure. The crystal structure of NaPb5(CO3)4(OH)3 is based on 2D blocks very similar to ones in hydrocerussite, [Pb3(OH)2(CO3)2]0 (blue) and abellaite, [NaPb2(OH)(CO3)2]0 (green). Lone electron pairs on Pb2+ cations are symbolized by e in between the blocks in the structure of NaPb5(CO3)4(OH)3, hydrocerussite and abellaite. See the text for details.

Fig. 5. [PbCO3] (denoted C1), [Pb(OH)2] (denoted LHO) and [PbCO3] (denoted C1*) sheets in a hydrocerussite block (above) and [PbCO3] (denoted C2), [NaOH] (denoted SHO) and [PbCO3] (denoted C2’) sheets in an abellaite block (below) in the structure of NaPb5(CO3)4(OH)3. See the text for details.
Similarly the [Na(OH)]0 sheet (SHO = sodium hydroxide, according to the approximate Na(OH) composition) is sandwiched between the other two [PbCO3]0 sheets (C2) thus forming the [NaPb2(OH)(CO3)2]0 block described previously in the structure of abellaite NaPb2(OH)(CO3)2 (Ibáñez-Insa et al., Reference Ibáñez-Insa, Elvira, Llovet, Pérez-Cano, Oriols, Busquets-Masó and Hernández2017). The full structural formula of this 2D block can be written as {[Pb(CO3)][(Na0.51Ca0.49)((OH)0.51O0.49)][Pb(CO3)]}0. As mentioned before, the presence of minor O in the OH2 site plays the role of a charge-compensating agent for the partial substitution of Na+ by Ca2+. The stacking of the sheets in abellaite-type block can be also symbolized as ···–C2–SHO–C2’–···, whereby the primed symbol indicates that the C sheets within a given ···C2–SHO–C2’··· block are related by reflection across a plane perpendicular to [001].
Analysis of the crystal structures of abellaite, hydrocerussite and grootfonteinite Pb3O(CO3)2 (Siidra et al., Reference Siidra, Jonsson, Chukanov, Nekrasova, Pekov, Depmeier, Polekhovsky and Yapaskurt2018b) (Table 5) allows us to suggest that positional disorder of the cations between [PbCO3]0 sheets occurs when Pb2+ is intruded (i.e. in hydrocerussite and grootfonteinite), and is absent in the case of the smaller cation Na+ (in abellaite).
Table 5. Comparative data of NaPb5(CO3)4(OH)3, hydrocerussite and abellaite.

*Hydrocerussite from the Merehead quarry, Somerset, England; **Palache et al. (Reference Palache, Berman and Frondel1951) and Anthony et al. (Reference Anthony, Bideaux, Bladh and Nichols2003) do not provide structural data; *** powder XRD data were indexed in Ibáñez-Insa et al. (Reference Ibáñez-Insa, Elvira, Llovet, Pérez-Cano, Oriols, Busquets-Masó and Hernández2017) after the structural data provided in Krivovichev and Burns (Reference Krivovichev and Burns2000); the correct space group is most likely P63/mmc (Siidra et al., Reference Siidra, Jonsson, Chukanov, Nekrasova, Pekov, Depmeier, Polekhovsky and Yapaskurt2018b).
Thus the structure of the slag phase from the Lavrion is built from alternating [NaPb2(OH)(CO3)2]0 and [Pb3(OH)2(CO3)2]0 blocks. There are two blocks (Fig. 4) of each type per unit cell, which corresponds to the following formula: [Pb3(OH)2(CO3)2][NaPb2(OH)(CO3)2] or NaPb5(CO3)4(OH)3 in simplified form.
Discussion
The structural study demonstrated that at least a part of the well-known presumed ‘hydrocerussite’ from the Lavrion slags is a different phase formed by both the abellaite- and hydrocerussite-type blocks. This phase is not related directly to plumbonacrite as was suggested by Kolitsch et al. (Reference Kolitsch, Rieck, Brandstätter, Schreiber, Fabritz, Blaß and Gröbner2014). Its structure type is novel for both minerals and synthetic compounds. The structure studied of NaPb5(CO3)4(OH)3 is rather two dimensional and the cohesion of the framework is insured by the weak Pb–O bonds only. The two-dimensional layered structure of NaPb5(CO3)4(OH)3 is clearly reflected in the lamellar habit of its crystals and perfect cleavage. This feature is characteristic for other lead hydroxycarbonate minerals including hydrocerussite Pb3(OH)2(CO3)2 (Martinetto et al., Reference Martinetto, Anne, Dooryhée, Walter and Tsoucaris2002; Siidra et al., Reference Siidra, Nekrasova, Depmeier, Chukanov, Zaitsev and Turner2018a), plumbonacrite Pb5O(OH)2(CO3)3 (Rumsey et al., Reference Rumsey, Siidra, Krivovichev, Spratt, Stanley and Turner2012), abellaite NaPb2(OH)(CO3)2 (Ibáñez-Insa et al., Reference Ibáñez-Insa, Elvira, Llovet, Pérez-Cano, Oriols, Busquets-Masó and Hernández2017), grootfonteinite Pb3O(CO3)2 (Siidra et al., Reference Siidra, Jonsson, Chukanov, Nekrasova, Pekov, Depmeier, Polekhovsky and Yapaskurt2018b) and leadhillite polymorphs Pb4(SO4)(CO3)2(OH)2 (Giuseppetti et al., Reference Giuseppetti, Mazzi and Tadini1990; Steele et al., Reference Steele, Pluth and Livingstone1998; Steele et al., Reference Steele, Pluth and Livingstone1999). Electroneutral 2D blocks of only one topology per structure are observed in the structures of all these minerals. The crystal structure of NaPb5(CO3)4(OH)3 is unique for lead hydroxycarbonates and, moreover, exclusive for layered minerals in general, as it demonstrates interstratification of neutral blocks of minerals significantly different in chemical composition and topology. However, the structural architecture of [Pb3(OH)2(CO3)2][NaPb2(OH)(CO3)2] = NaPb5(CO3)4(OH)3 resembles the organization of the crystal structure of [${\rm Tl}_{5}^{+} $(SiO4)(OH)]2[
${\rm Tl}_{6}^{+} $(SO4)(OH)4] =
${\rm Tl}_{{16}}^{+} $(SiO4)2(SO4)(OH)6 (Siidra et al., Reference Siidra, Britvin, Krivovichev, Klimov and Depmeier2014). Note, stereochemically active 6s 2 lone electron pairs on Pb2+ or Tl+ cations play an important role for the formation of such structural architectures and may result in the very different stackings of 2D blocks.
The formation of NaPb5(CO3)4(OH)3, as well as the previously known abellaite-type phase NaPb2(OH)(CO3)2 in Lavrion slags, is caused by the contact of lead slags with the seawater over the last several thousand years. However the recent find of abellaite (Ibáñez-Insa et al., Reference Ibáñez-Insa, Elvira, Llovet, Pérez-Cano, Oriols, Busquets-Masó and Hernández2017) in the Eureka mine, Catalonia, Spain, indicates the possibility of its formation under different conditions. Hydrocerussite itself is a stable mineral under different environmental conditions with high pH values. Thus we hypothesize that the NaPb5(CO3)4(OH)3 phase may exist as a mineral at some exotic conditions. Recently we have described grootfonteinite Pb3O(CO3)2 (Siidra et al., Reference Siidra, Jonsson, Chukanov, Nekrasova, Pekov, Depmeier, Polekhovsky and Yapaskurt2018b) from the Kombat mine, Namibia. The topology of 2D blocks in the crystal structure of grootfonteinite can be considered as intermediate between those of abellaite and hydrocerussite. Further, new lead hydroxycarbonate minerals with structural architectures organized via similar building principles (i.e. based on 2D blocks of different composition and topology) as in NaPb5(CO3)4(OH)3 are anticipated.
Acknowledgements
The authors are grateful to Peter Leverett, Gerald Giester and Daniel Atencio for valuable comments. This work was supported by St. Petersburg State University through the internal grant 3.38.238.2015 and 3.42.1495.2015 (for part of the XRD and crystal-structure studies) and by the Russian Science Foundation, grant no. 14-17-00048 (for part of the electron probe and IR spectroscopy studies). Technical support by the X-Ray Diffraction Resource Centre of Saint-Petersburg State University is gratefully acknowledged.
Supplementary material
To view supplementary material for this article, please visit https://doi.org//10.1180/minmag.2017.081.058.