1. Introduction
The globally distributed Marinoan cap dolostone overlying the Marinoan glaciogenic deposits represents a suite of unique sedimentary successions that continues to attract worldwide attention (Kennedy et al. Reference Kennedy, Christie-Blick and Sohl2001; Hoffman & Schrag, Reference Hoffman and Schrag2002; Jiang et al. Reference Jiang, Kennedy and Christie-Blick2003, Reference Jiang, Kennedy, Christie-Blick, Wu and Zhang2006; Shields et al. Reference Shields, Deynoux, Strauss, Paquet and Nahon2007; Bao et al. Reference Bao, Lyons and Zhou2008; Wang et al. Reference Wang, Jiang, Xiao, Li and Wei2008; Zhou et al. Reference Zhou, Bao, Peng and Yuan2010, Reference Zhou, Luo, Zhou, Xing and Gan2017; Bristow et al. Reference Bristow, Bonifacie, Derkowski, Eiler and Grotzinger2011). Yet, the perplexing sedimentary structures and anomalously negative carbon isotope signals registered in the Marinoan cap dolostone make it difficult to explain the detailed sedimentological and petrographic context, although numerous geochemical and isotopic data have been intermittently reported. Specifically, the origin of calcite with extremely negative δ13C values, and its petrographic and genetic relationships with paragenetic minerals and Marinoan deglaciation, remains disputed. Oxidization of methane was proposed to have offered excess CO3 2− inducing carbonate precipitation and explained the observed extremely negative carbon isotope anomaly (Jiang et al. Reference Jiang, Kennedy and Christie-Blick2003; Kennedy et al. Reference Kennedy, Mrofka and von der Borch2008; Wang et al. Reference Wang, Jiang, Xiao, Li and Wei2008), but whether such a process occurred during deposition/early diagenetic or later hydrothermal activity remains disputed (Zhou et al. Reference Zhou, Bao, Peng and Yuan2010, Reference Zhou, Luo, Zhou, Xing and Gan2017; Bristow et al. Reference Bristow, Bonifacie, Derkowski, Eiler and Grotzinger2011). It follows that ascertaining the temporal sequence of calcite and paragenetic minerals and their formation mechanisms would contribute to our understanding of their causal relationships with the Snowball Earth hypothesis, as well as providing a sound interpretation of geochemical data.
Calcite has frequently been utilized in probing the timing and causal relationships of biological and environmental events recorded in carbonates by means of measuring radiogenic U–Pb and stable C–O isotopic systems and trace-element compositions (Wang et al. Reference Wang, Jiang, Xiao, Li and Wei2008; Bristow et al. Reference Bristow, Bonifacie, Derkowski, Eiler and Grotzinger2011; Franchi et al. Reference Franchi, Hofmann, Cavalazzi, Wilson and Barbieri2015; Kalliomäki et al. Reference Kalliomäki, Wagner, Fusswinkel and Schultze2019; MacDonald et al. Reference MacDonald, Faithfull, Roberts, Davies, Holdsworth, Newton, Williamson, Boyce and John2019; Roberts et al. Reference Roberts, Drost, Horstwood, Condon, Chew, Drake, Milodowski, McLean, Smye, Walker and Haslam2020). This is because calcite commonly incorporates rare earth elements (REEs) and uranium during its crystallization, making it a suitable host for REE analyses and a potential chronometer for U–Pb geochronology. Calcite REE compositions are used as key evidence to distinguish its origin, as variations in REE distribution patterns are suggestive of variations of circulating fluid chemistry. Specifically, calcite with low REE concentrations and a positive Eu anomaly commonly precipitates from hydrothermal fluids, whereas calcite with positive La and Gd anomalies, a super-chondritic Y/Ho ratio and light REE (LREE) depletion commonly forms in normal seawater (Van Kranendonk et al. Reference Van Kranendonk, Webb and Kamber2003; Franchi et al. Reference Franchi, Hofmann, Cavalazzi, Wilson and Barbieri2015; Kalliomäki et al. Reference Kalliomäki, Wagner, Fusswinkel and Schultze2019).
Owing to differential geochemical behaviour and the abundance of U and Pb in seawater (Ku et al. Reference Ku, Knauss and Mathieu1977; Shen & Boyle, Reference Shen and Boyle1987), marine carbonates are particularly suitable for U–Pb dating because of their commonly high U/Pb ratios (Rasbury et al. Reference Rasbury, Ward, Hemming, Li, Dickson, Hanson and Major2004). As such, calcite geochronology could potentially produce direct age constraints in various geoscience applications (Rasbury & Cole, Reference Rasbury and Cole2009; Roberts et al. Reference Roberts, Drost, Horstwood, Condon, Chew, Drake, Milodowski, McLean, Smye, Walker and Haslam2020). Laser ablation-inductively coupled plasma-mass spectrometry (LA-ICP-MS) has been demonstrated to be an effective tool for in situ calcite U–Pb dating and REE composition measurement because of rapid in situ spot analysis with adequate levels of precision (Li et al. Reference Li, Parrish, Horstwood and McArthur2014; Roberts & Walker, Reference Roberts and Walker2016; Coogan et al. Reference Coogan, Parrish and Roberts2016; Ring & Gerdes, Reference Ring and Gerdes2016; Nuriel et al. Reference Nuriel, Weinberger, Kylander-Clark, Hacker and Craddock2017; Kalliomäki et al. Reference Kalliomäki, Wagner, Fusswinkel and Schultze2019; MacDonald et al. Reference MacDonald, Faithfull, Roberts, Davies, Holdsworth, Newton, Williamson, Boyce and John2019; Roberts et al. Reference Roberts, Drost, Horstwood, Condon, Chew, Drake, Milodowski, McLean, Smye, Walker and Haslam2020; Wu et al. Reference Wu, Yang, Roberts, Yang, Wang, Lan, Xie, Li, Xu, Huang, Xie, Yang and Wu2022). This paired approach proves robust in constraining the formation timing of calcite and fingerprinting the source of fluids and thus tracing fluid evolution processes. To this end, we carry out detailed REE and U–Pb isotope analyses combined with scanning electron microscope (SEM) and cathodoluminescence (CL) imaging techniques on calcite from the Marinoan cap dolostone at the Jiulongwan type section to decipher its genesis and timing of formation. The findings provide further constraints on our understanding of its relationship with the Snowball Earth hypothesis.
2. Geological setting and sampling information
The late Mesoproterozoic to earliest Neoproterozoic Jiangnan Orogeny resulted in the formation of the South China Block (SCB) by means of amalgamating the Yangtze Block to the northwest with the Cathaysia Block to the southeast, along the Jiangnan Orogen (Li et al. Reference Li, Li, Li, Lo, Wang, Ye and Yang2009). The SCB experienced a subsequent intracontinental rifting, and accommodated abundant well-preserved Neoproterozoic sedimentary successions in the Nanhua Basin: the largest Neoproterozoic basin in the SCB (Wang & Li, Reference Wang and Li2003). Such a suite of sedimentary successions is well exposed in the Hubei–Hunan–Guizhou–Guangxi regions of the Nanhua Basin. The Ediacaran Yangtze platform in South China developed on a Neoproterozoic rifted continental margin that is interpreted to have started along the southeastern side of the Yangtze Block at c. 800 Ma (Wang & Li, Reference Wang and Li2003; Jiang et al. Reference Jiang, Kaufman, Christie-Blick, Zhang and Wu2007). The Ediacaran carbonate and siliciclastic rocks were assumed to have been accommodated in a passive margin setting (Jiang et al. Reference Jiang, Kennedy and Christie-Blick2003, Reference Jiang, Kaufman, Christie-Blick, Zhang and Wu2007). Ediacaran sedimentary rocks in South China are composed of the fossiliferous Doushantuo and Dengying formations, which show a wide spatial occurrence around the Yangtze Block, preserving key biostratigraphic and chemostratigraphic records regarding the coevolution of life and environmental conditions (Fig. 1a, b; Lan et al. Reference Lan, Sano, Yahagi, Tanaka, Shirai, Papineau, Sawaki, Ohno, Abe, Yang, Liu, Jiang and Wang2019 and references therein).

Fig. 1. (a) Geological map of China with the Yangtze platform highlighted in purple colour. (b) Reconstructed Ediacaran depositional environments on the Yangtze platform (Jiang et al. Reference Jiang, Shi, Zhang, Wang and Xiao2011). Red star marks the study location. (c) Stratigraphic column showing the sampling horizon in the cap dolostone of Doushantuo Formation.
Previous sedimentological research suggests deposition of the Doushantuo Formation in two stages. The first stage witnessed deposition of the couplet of cap carbonate and overlying black shale of the lower Doushantuo Formation in an open shelf/ramp depositional environment, whereas the second stage is typical of a rimmed carbonate shelf with a shelf-margin barrier separating the intra-shelf lagoon from open ocean settings (Jiang et al. Reference Jiang, Shi, Zhang, Wang and Xiao2011). The Yangtze Gorges area accommodated the most complete and continuous outcrops of the Doushantuo Formation exposed at the Jiulongwan, Huajipo and other sections (Jiang et al. Reference Jiang, Shi, Zhang, Wang and Xiao2011). The Doushantuo Formation is best exposed at the intra-shelf basin Jiulongwan section (Jiang et al. Reference Jiang, Shi, Zhang, Wang and Xiao2011). Therein, the Doushantuo Formation has a conformable contact with the underlying Nantuo Formation (Fig. 2a, b), and is composed of c. 160 m thick mixed shale and carbonate. The Nantuo Formation is dominated by glaciogenic greenish diamictite with minor sandstone beds correlative with the late Cryogenian ‘Marinoan glaciation’ (Lang et al. Reference Lang, Chen, Cui, Man, Huang, Fu, Zhou and Shen2018). The Doushantuo Formation is dividable into, in ascending order, Member 1 composed of cap dolostone, Member 2 dominated by black shale, Member 3 dominated by dolostone and Member 4 composed exclusively of black shale (with carbonate concretions). Regionally traceable cap carbonates in the basal Doushantuo Formation show anomalously negative 13Ccarb excursions and intriguing sedimentary structures such as stromatactis-like cavities, botryoidal cemented breccias, layer-parallel sheet cracks and tepee-like positive reliefs (Jiang et al. Reference Jiang, Kennedy and Christie-Blick2003; Wang et al. Reference Wang, Jiang, Xiao, Li and Wei2008), which has led to them receiving the most intensive investigation among the worldwide Neoproterozoic successions.

Fig. 2. Field and petrographic photos showing the cap dolostone of the Doushantuo Formation at the Jiulongwan section, Three Gorges area, South China. (a) The boundary between the Nantuo and Doushantuo formations. (b) Cap dolostone of the Doushantuo Formation. (c, d) Cap dolostone with botryoidal structures. Hammers in (c) and (d) are c. 35 cm long. (e) Merged reflected light images showing the petrography of grape-structured dolostone that is dominated by dolomite and calcite that are quartz cemented. D – dolomite; C – calcite; Q – quartz. The white dotted circles indicate laser analysis spots in calcite.
A time interval between c. 635 Ma and 550 Ma was constrained for the depositional age of the Doushantuo Formation exposed at the Jiulongwan and Jijiawan sections by means of thermal ionization mass spectrometry (TIMS) U–Pb dating of zircon grains from two interbedded tuff beds around the basal Doushantuo Formation and near the Doushantuo–Dengying Formation boundary (Condon et al. Reference Condon, Zhu, Bowring, Wang, Yang and Jin2005; Yang et al. Reference Yang, Rooney, Condon, Li, Grazhdankin, Bowyer, Hu, Macdonald and Zhu2021; Fig. 1c). The cap dolostone at the Jiulongwan section is mainly composed of three components; they are a disrupted poorly stratified limestone/dolostone layer, laminated limestone/dolostone displaying teepee-like structures, and laminated silty limestone/dolostone (Jiang et al. Reference Jiang, Kennedy and Christie-Blick2003; Fig. 1c). Multiple generations of botryoidal cements filled in irregular cavities possibly formed by vertical extension of micritic crusts (Jiang et al. Reference Jiang, Kennedy and Christie-Blick2003; Fig. 2c, d). The teepee-like structures display positive relief and are made up of laminated micrite and dolomicrite, which bear layer-parallel sheet cracks surrounding the host carbonate (Jiang et al. Reference Jiang, Kennedy and Christie-Blick2003). These characteristics suggest they were probably formed by means of layer-parallel compression and vertical extension (c.f. Hoffman & Macdonald, Reference Hoffman and Macdonald2010). Sample 1-2 is a fresh grape-structured dolostone collected from the disrupted dolostone layer that is c. 130 cm below the c. 635 Ma tuff bed; it is mainly composed of dolomite and calcite that are quartz cemented (Fig. 2e).
3. Analytical methods
In this study, both imaging analysis and in situ element and isotope analyses were conducted in order to reveal the formation timing and origin of calcite and its relationship with a syngenetic mineral assemblage in cap dolostone, as detailed below.
3.a. Imaging analysis
Polished thin-sections were prepared for petrographic observations and selection of spots for in situ mineral chemistry and calcite geochronology. A Nikon Eclipse E800 microscope equipped with a Nikon DS-Fi 1 camera and Zeiss 1555 VP-FESEM at the State Key Laboratory of Lithospheric Evolution, Institute of Geology and Geophysics, Chinese Academy of Sciences (SKLLE, IGGCAS) were utilized to detect textural relationships for syngenetic minerals. The SEM was specifically manipulated under backscattered electron mode to get an optimal resolution at 50–650× magnification. It was adjusted to an optimal working distance of 6–8 mm and a voltage of 10 kV. In order to obtain CL images of carbonates, a cold CL microscope at the Key Lab of Petroleum Resources at IGGCAS was utilized with a beam voltage of 16 kV, a current of 325 μA and a beam diameter of 4 mm.
3.b. Calcite REE composition analysis
Trace-element contents of calcite were determined by LA-ICP-MS employing an Element XR HR-ICP-MS instrument (Thermo Fisher Scientific, USA) coupled to a 193 nm ArF excimer laser system (Geolas HD, Lambda Physik, Göttingen, Germany) at SKLLE, IGGCAS. The approach is similar to those outlined in Wu et al. (Reference Wu, Karius, Schmidt, Simon and Woerner2018) with isotopes measured using a peak-hopping mode with a laser beam diameter of c. 44 μm, a repetition rate of 5 Hz and a laser energy density of ∼3.0 J cm−2. Helium was employed as the ablation gas to improve the transporting efficiency of ablated aerosols. ARM-3 (Wu et al. Reference Wu, Worner, Jochum, Stoll, Simon and Kronz2019) reference glass was used as a calibration reference material, and BIR was analysed for data quality control. Calcium (40Ca) was used as an internal standard. The resulting data were reduced using the GLITTER program (Griffin et al. Reference Griffin, Powell, Pearson, O Reilly and Sylvester2008). For most trace elements (>0.05 μg/g), the accuracy is better than ±10 % with analytical precision (1 RSD) of ±10 %.
3.c. Calcite U–Pb dating
Detailed procedures of the instrumentation and analytical protocol for calcite U–Pb dating are given in Wu et al. (Reference Wu, Yang, Roberts, Yang, Wang, Lan, Xie, Li, Xu, Huang, Xie, Yang and Wu2022) and are only briefly summarized herein. A Photo Machine Analyst G2 laser ablation system (Teledyne CETAC, Omaha, USA) coupled to an Element XR (Thermo Fisher Scientific, Bremen, Germany) was adopted to carry out in situ calcite U–Pb dating on polished thin-sections using LA-ICP-MS at SKLLE, IGGCAS. A high-capacity vacuum pump was used to make the high-performance Jet sample cone work efficiently. The guard electrode was used to improve sensitivity (Wu et al. Reference Wu, Yang, Wang, Huang, Xie and Yang2020 a,b), while a T junction was adopted to add N2 into the Ar sample gas flow behind the ablation cell.
A blind spot analysis was conducted using a laser beam of 80 μm to screen for high uranium regions for subsequent detailed in situ U–Pb spot dating analysis. An automated mode in sequences of 60 to 80 spot analyses was used to acquire raw data. Each spot analysis is composed of 8 s of background acquisition followed by 25 s of sample ablation and 30 s washout. A peak jumping mode with a total integration time of 0.30 s to produce 130 mass scans was adopted to detect the signals of 202Hg, 204(Hg + Pb), 206Pb, 207Pb, 208Pb, 232Th and 238U during the data acquisition. In this study, a total of four reference materials (RMs) were used including two calcite U–Pb reference materials for calibration of U/Pb ratios and validation of the results (WC-1 and Duff Brown Tank; Hill et al. Reference Hill, Polyak, Asmerom and Provencio2016; Roberts et al. Reference Roberts, Rasbury, Parrish, Smith, Horstwood and Condon2017) and two glass reference materials for calibration of Pb/Pb ratios and drift correction (ARM-3 and NIST SRM 614; Woodhead & Hergt, Reference Woodhead and Hergt2001; Wu et al. Reference Wu, Yang, Jochum, Romer, Glodny, Savov, Agostini, De Hoog, Peters, Kronz, Zhang, Bao, Wang, Li, Tang, Feng, Yu, Li, Zhang, Lin, Zeng, Xu, Wang, Cui, Deng, Xiao, Liu, Xue, Zhang, Jia, Wang, Xu, Huang, Xie, Pack, Woerner, He, Li, Yuan, Huang, Li, Yang, Li and Wu2021). Two analyses of glass NIST SRM 614 and ARM-3 in combination with three analyses of WC-1 were analysed bracketing every ten analyses of unknown samples. The Duff Brown Tank (64.04 ± 0.67 Ma; Hill et al. Reference Hill, Polyak, Asmerom and Provencio2016) was treated as an unknown sample to monitor data accuracy.
A total of 40 spot analyses were carried out on sample 1-2. The data reduction method follows those described recently by Wu et al. (Reference Wu, Yang, Roberts, Yang, Wang, Lan, Xie, Li, Xu, Huang, Xie, Yang and Wu2022), and uses the premise of calibrating against a material with known age but heterogeneous composition as per Roberts et al. (Reference Roberts, Rasbury, Parrish, Smith, Horstwood and Condon2017) and Chew et al. (Reference Chew, Petrus and Kamber2014). The Iolite 3.7 data reduction software and the VizualAge data reduction scheme were used to reduce the raw data (time-resolved intensities) from the ICP-MS (Paton et al. Reference Paton, Hellstrom, Paul, Woodhead and Hergt2011; Petrus & Kamber, Reference Petrus and Kamber2012). Correction of instrument drift and calculation of isotope ratios of 207Pb/206Pb is accomplished by linear fit of ARM-3. The recommended value of 207Pb/206Pb is taken from Wu et al. (Reference Wu, Yang, Jochum, Romer, Glodny, Savov, Agostini, De Hoog, Peters, Kronz, Zhang, Bao, Wang, Li, Tang, Feng, Yu, Li, Zhang, Lin, Zeng, Xu, Wang, Cui, Deng, Xiao, Liu, Xue, Zhang, Jia, Wang, Xu, Huang, Xie, Pack, Woerner, He, Li, Yuan, Huang, Li, Yang, Li and Wu2021). NIST SRM 614 is used for the accuracy monitoring of 207Pb/206Pb. Ten analyses of NIST SRM 614 yielded a mean of 0.8684 ± 0.0093 (2s), which is in agreement with the recommended value of 0.8710 ± 0.0004 (2s) (Baker et al. Reference Baker, Peate, Waight and Meyzen2004). The downhole fractionation profile from ARM-3 glasses was used to calibrate the laser induced elemental fractionations of WC-1 and other samples (Wu et al. Reference Wu, Yang, Roberts, Yang, Wang, Lan, Xie, Li, Xu, Huang, Xie, Yang and Wu2022). After the initial correction, WC-1 was used for further calibration of matrix-induced mass bias in 238U/206Pb ratios between RMs and unknown samples, which was accomplished in Microsoft Excel. The uncertainty propagation of LA-ICP-MS calcite geochronology is a modified approach based upon Horstwood et al. (Reference Horstwood, Kosler, Gehrels, Jackson, McLean, Paton, Pearson, Sircombe, Sylvester, Vermmesch, Bowring, Condon and Schoene2016), wherein the final age is quoted without and with systematic uncertainties, as ± α/β, respectively.
4. Results
4.a. Petrography of the studied samples
Field outcrops show that botryoidal structures and cavities widely occur in the basal cap dolostone, which is poorly stratified (Fig. 2a, c, d). Initial deposition of cap dolostone was followed by subsequent uplifting because of isostatic rebound, during which the cap dolostone experienced karstic dissolution in shallow marine to marginal depositional settings in South China, forming abundant cavities (Zhou et al. Reference Zhou, Bao, Peng and Yuan2010). The botryoidal structures probably formed during a subsequent transgression, which induced a series of mineral precipitation within these cavities. The botryoidal structures not only encrust the bed surface but also the surface of cavities, with a thickness of 1–5 cm (Fig. 2c, d). Microscopic observations show the dolostone sample is mainly composed of dolomite, calcite, pyrite with minor barite and iron oxide (Figs 3, 4, 5). In most cases, calcite occurs as void and crack fillings among isopachous (about 200–500 μm thick) dolomite (Fig. 3a, b, f). Most of the crack fillings display tapering ends. Finer cracks locally occur abundantly as meshworks (Fig. 3b, c). In some places, calcite veins, 30–500 μm thick, co-occur with pyrite (up to 250 μm) as vein fillings extending in various directions (Fig. 3c, d). Some calcite occurs as flextured isopachous veins, about 250 μm thick in quartz cements (recrystallized chalcedony and microquartz) (Fig. 3e). Aside from these phenomena, fibrous/amorphous calcite cements are seen to co-occur with fine barite and iron oxides/pyrite of 30–100 μm within dolomites (Fig. 4a, e). Amorphous quartz cement widely occurs filling the pore spaces of calcite cement (Fig. 4b) or entombing euhedral calcite or iron oxide grains (Fig. 4c, d). The euhedral calcite grains have a size range of 50–350 μm, some of which bear porous margins infilled by quartz cements (e.g. Fig. 4d). The iron oxides, 50–150 μm in size, are intimately associated with calcite (Fig. 4f). Under CL, calcite cements display a bright orange colour, whereas dolomite crystals show a blue colour (Fig. 5).
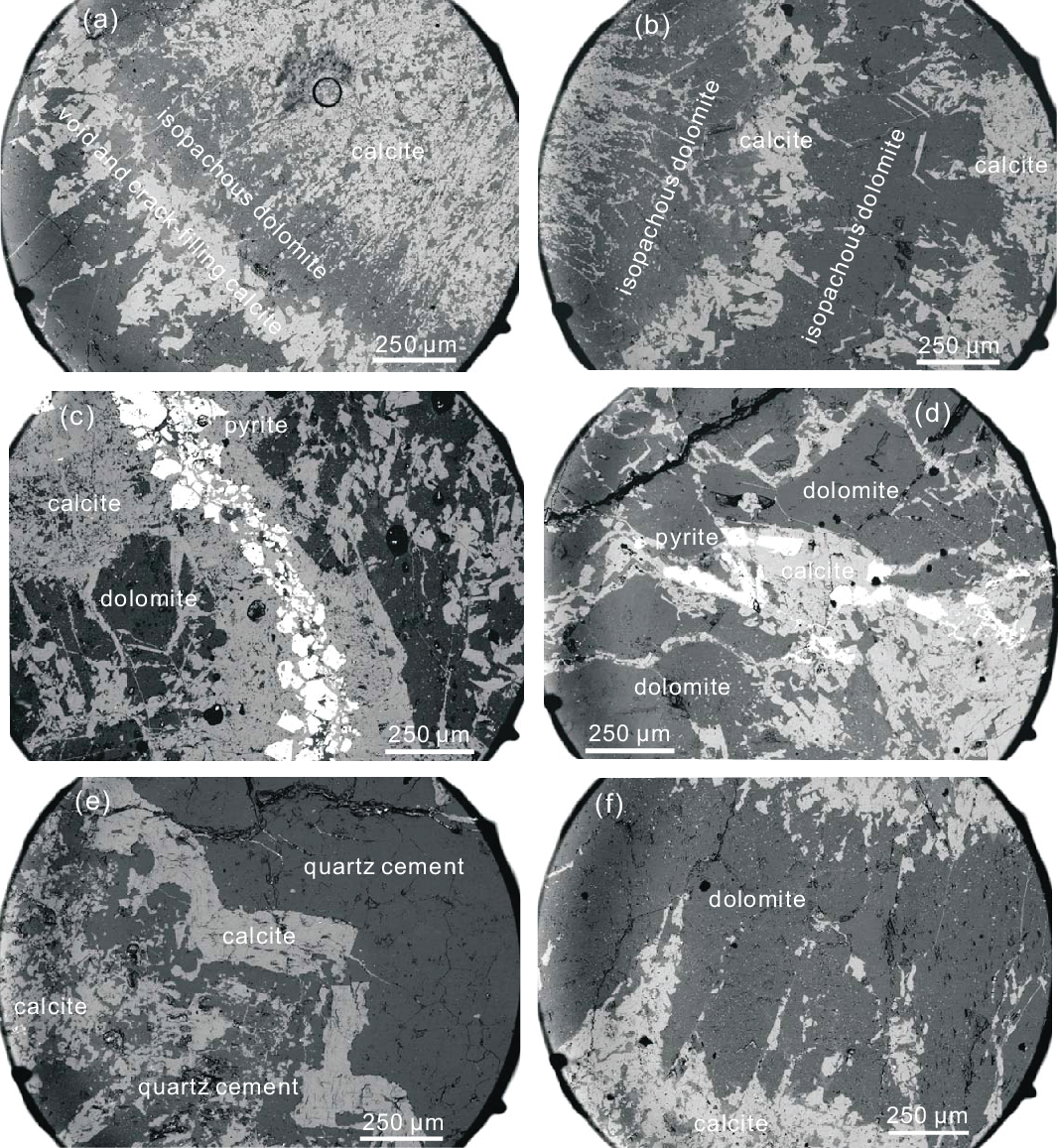
Fig. 3. Backscattered electron (BSE) images of cap dolostone showing paragenetic mineral assemblage. Initial dolostone deposition was followed by exposure and karstification due to isostatic rebound, forming voids and cracks that were subsequently filled by multidirectional straight or flextured pyrite and calcite cements/veins (a–e), which were then quartz cemented (e). The dolostone is mainly composed of isopachous dolomite. Black circle in (a) represents laser analysis spot.

Fig. 4. Backscattered electron (BSE) images of cap dolostone showing paragenetic mineral assemblage. Various kinds of calcite cements/grains, pyrite or iron oxide, and barite filled in the cracks and cavities in dolostone that was formed via karstification, and was then quartz cemented. Black circles in (e) represent laser analysis spots.

Fig. 5. Cathodoluminescence (CL) photomicrographs for sample 1-2 used as reference for choosing laser spots. (a) and (c) are reflected light images, whereas (b) and (d) are their corresponding CL images. Calcite cements show a distinct bright orange colour, which is in sharp contrast to the isopachous dolomite crystals which show a blue colour.
4.b. Calcite REE composition
LA-ICP-MS calcite REE compositions are tabulated in online Supplementary Material Table S1. Overall, the calcite shows a bell-shaped distribution pattern. They are characterized by positive La and Y anomalies, and high Y/Ho ratios (39–61 with an average of 50) (Fig. 6). Except for three points that have Y/Ho ratios of 39–41, the other 20 points all have Y/Ho ratios of >44. A prominent positive Gd anomaly is also present with δGd in the range of 1.1–1.96. Eu is dominated by positive anomalies (δEu = 1.02–1.38) with minor slightly negative anomalies (δEu = 0.79–0.96). PrSN/YbSN ratios vary from 0.3 to 3.0 with an average of 0.9.
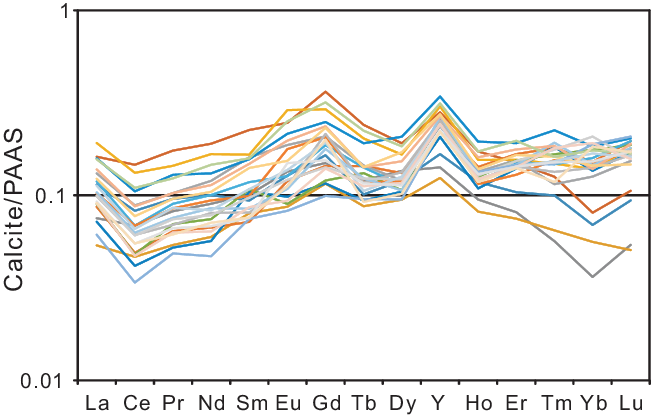
Fig. 6. Calcite rare earth element (REE) post-Archaean Australian shale (PAAS) normalized plot.
4.c. Calcite U–Pb geochronology
LA-ICP-MS calcite U–Pb dating results are tabulated in online Supplementary Material Table S2. A total of 185 spot analyses (over one month) were conducted for Duff Brown Tank, which yielded a pooled age of 62.44 ± 0.91 Ma (MSWD = 7.2) (Fig. 7a), whereas 10 spot analyses during the analytical session for sample 1-2 yielded an intercept age of 62.63 ± 2.80 Ma (MSWD = 1.7). Both of these two ages are within uncertainly of the published isotope dilution age (Hill et al. Reference Hill, Polyak, Asmerom and Provencio2016). These results suggest the long-term reproducibility across different analytical sessions is no larger than the limiting 2.5 % age uncertainty of the WC-1 reference material. During analysis, only calcite cements with smooth surfaces were chosen for U–Pb geochronology. A total of 40 spot analyses were conducted on the calcite cements of sample 1-2. Four spots fall to the right of a distinct regression in Tera-Wasserburg space, and thus were removed; they may reflect a slight amount of open-system behaviour, i.e. U mobility, in the sampled region. The remaining 36 spots yield a lower intercept age of 636.5 ± 7.4 Ma (2σ, MSWD = 1.6, n = 36/40) (Fig. 7b). After propagation of decay constant and reference material (WC-1) uncertainties, the final age uncertainty that is required for age comparison is 17.8 Ma. As such, 636.5 ± 7.4/17.8 Ma is interpreted as the depositional age of the sampling horizon.

Fig. 7. Tera-Wasserburg Concordia plots (using IsoplotR; Vermeesch, Reference Vermeesch2018) of (a) reference material Duff Brown Tank and (b) sample 1-2. The upper intercepts define common Pb compositions, whereas the lower intercepts define dates that are quoted as ± α/β; see text for explanation.
5. Discussion
5.a. Constraints on the cap dolostone
The complex sedimentary structures and anomalously negative carbon isotope signals registered in the cap dolostone are deemed to be associated with methane release induced by destabilization of low-latitude permafrost clathrates, which have aided in triggering the Marinoan deglaciation and subsequent flooding of continental shelves (Kennedy et al. Reference Kennedy, Christie-Blick and Sohl2001, Reference Kennedy, Mrofka and von der Borch2008; Jiang et al. Reference Jiang, Kennedy and Christie-Blick2003). Authigenic calcite cements with extremely negative δ13Ccarb values (<−45 ‰) are the critical evidence in favour of the hypothesis that oxidization of methane hydrate offered excess CO3 2− and induced the precipitation of cap dolostone (Jiang et al. Reference Jiang, Kennedy and Christie-Blick2003; Kennedy et al. Reference Kennedy, Mrofka and von der Borch2008; Wang et al. Reference Wang, Jiang, Xiao, Li and Wei2008). It remains disputed, however, whether such a process occurred during deposition or early diagenesis, or during later hydrothermal activity (Zhou et al. Reference Zhou, Bao, Peng and Yuan2010, Reference Zhou, Luo, Zhou, Xing and Gan2017; Bristow et al. Reference Bristow, Bonifacie, Derkowski, Eiler and Grotzinger2011). Our petrographic, geochemical and geochronological data demonstrate that these calcite cements were either originally precipitated in normal seawater and subsequently overprinted by early Ediacaran hydrothermal activities or were purely formed in early Ediacaran hydrothermal fluids, as detailed below.
It is commonly accepted that the shale-normalized REE + Y pattern of modern seawater is characterized by strong LREE depletion (PrSN/YbSN = 0.2–0.4), positive La and Gd (Gd/Gd* = 1.05–1.30) and negative Ce anomalies, and super-chondritic Y/Ho ratios ranging from 44 to 74 (Franchi et al. Reference Franchi, Hofmann, Cavalazzi, Wilson and Barbieri2015 and references therein). The post-Archaean Australian shale (PAAS)-normalized REE distribution patterns for the calcite cements in the cap dolostone display an overall slightly LREE depletion (PrSN/YbSN = 0.3 to 3.0), positive La and Gd anomalies (Gd/Gd* = 1.10–1.96) and negative Ce anomalies, and high Y/Ho ratios (most of which are >44 with an average of 50), which are typical of precipitation from normal marine seawater. Y and Ho have the same charge and similar ionic radius, with the latter removed from seawater much faster than the former because of differential complexation behaviour (Nozaki et al. Reference Nozaki, Zhang and Amakawa1997). This explains why the calcite has high Y/Ho ratios. Ce is present as Ce(IV) in oxygenated environments that can be readily incorporated into Fe-oxyhydroxides (Derry & Jacobsen, Reference Derry and Jacobsen1990), resulting in a negative Ce anomaly in marine precipitate. As such, Ce anomalies can be used to infer the seawater redox state; a negative Ce anomaly indicates an oxygenated oceanic condition, whereas a positive Ce anomaly reflects an anoxic depositional environment (Hu et al. Reference Hu, Feng, Peckmann, Roberts and Chen2014; Franchi et al. Reference Franchi, Hofmann, Cavalazzi, Wilson and Barbieri2015). The uniform negative Ce anomalies in our calcites are consistent with an oxygenated oceanic condition.
Insofar as barite is cogenetic with dolomite, calcite, pyrite/Fe-oxide and quartz in the basal Doushantuo Formation at the Jiulongwan section, a seawater origin also receives support from Ba-isotopes of barite (BaSO4) in the Jiulongwan and other basal cap dolostone sections in South China (Crockford et al. Reference Crockford, Wing, Paytan, Hodgskiss, Mayfield, Hayles, Middleton, Ahm, Johnston, Caxito, Uhlein, Halverson, Eickmann, Torres and Horner2019), which have nearly invariable isotope compositions of ≤0.1 ‰ that are within the range of modern pelagic barite and barites in worldwide Marinoan cap dolostones. This is in sharp contrast to modern cold-seep barites, which have variable Ba-isotope compositions of ≤0.9 ‰ (Crockford et al. Reference Crockford, Wing, Paytan, Hodgskiss, Mayfield, Hayles, Middleton, Ahm, Johnston, Caxito, Uhlein, Halverson, Eickmann, Torres and Horner2019). Whereas the Ba-isotopes suggest a well-mixed residual snowball brine for the source of Ba, Δ17O anomalies of atmospheric origin recorded in the same barites suggest a runoff origin for the sulfate. Redox reaction may have driven barite precipitation in the context of mixing of anoxic brine with meteoric ice meltwater (Bao et al. Reference Bao, Lyons and Zhou2008; Zhou et al. Reference Zhou, Bao, Peng and Yuan2010).
On the other hand, REE patterns with positive Eu and negative Ce anomalies are suggestive of mixing of hydrothermal solutions with seawater at unknown dilution ratios (Franchi et al. Reference Franchi, Hofmann, Cavalazzi, Wilson and Barbieri2015). Hydrothermal solutions are commonly enriched in LREEs and Eu because of breakdown of plagioclase; thus hydrothermal influence tends to induce LREE enrichment and positive Eu anomalies (e.g. Michard, Reference Michard1989; Van Kranendonk et al. Reference Van Kranendonk, Webb and Kamber2003). Almost half of our REE analyses show positive Eu and negative Ce anomalies, which, coupled with less distinctive LREE depletion patterns, can be interpreted to have resulted from a hydrothermal overprint. A hydrothermal overprint on the seawater composition is also demonstrated by the non-linear correlation between Y/Ho and La in our calcite cements, which are characterized by La excess (c.f. Kamber & Webb, Reference Kamber and Webb2001; Franchi et al. Reference Franchi, Hofmann, Cavalazzi, Wilson and Barbieri2015), and commonly high Mn contents (7500–15 000 ppm) that are frequently recorded in typical hydrothermal calcite (Kalliomäki et al. Reference Kalliomäki, Wagner, Fusswinkel and Schultze2019).
A hydrothermal influence also receives support from clumped isotope (Δ47) analyses of calcite, fluid inclusion analyses of quartz cements and alteration of trioctahedral clays into chlorite (Bristow et al. Reference Bristow, Bonifacie, Derkowski, Eiler and Grotzinger2011; Derkowski et al. Reference Derkowski, Bristow, Wampler, Sŕodoń, Marynowski, Elliott and Chamberlain2013; Zhou et al. Reference Zhou, Luo, Zhou, Xing and Gan2017). On the basis of clumped isotope (Δ47) analyses, Bristow et al. (Reference Bristow, Bonifacie, Derkowski, Eiler and Grotzinger2011) found these calcite cements formed under a high-temperature regime (T >400 °C) and thus interpreted them as thermogenic methane oxidation products by means of hydrothermal activity during early Ediacaran or even Cambrian time (Bristow et al. Reference Bristow, Bonifacie, Derkowski, Eiler and Grotzinger2011). Meanwhile, fluid inclusion analyses in quartz cements produced homogenization temperatures of c. 160–220 °C with both high salinity (18.0–20.8 wt % NaCl equiv.) and low-salinity (6.3–8.3 wt % NaCl equiv.) end-members, which are suggestive of mixing of high- and low-salinity hydrothermal fluids (Zhou et al. Reference Zhou, Luo, Zhou, Xing and Gan2017). Alteration of saponite into chlorite via corrensite increased from lower in Member 2 to the cap dolostone, which is commensurate with a decrease of δ18O and increase of δD in trioctahedral clays and is interpreted to have resulted from hydrothermal fluid activity in the underlying Nantuo Formation and cap dolostone (Derkowski et al. Reference Derkowski, Bristow, Wampler, Sŕodoń, Marynowski, Elliott and Chamberlain2013).
Petrographic observations allow identification of three sequences of depositional/cementation events. Dolomite was first precipitated, followed by the formation of calcite cements/minerals, pyrite/iron oxide and barite, which subsequently experienced quartz cementation (Figs 3, 4). Note that barite only occasionally occurs as finer microscopic grains in association with calcite and dolomite in our samples, whereas abundant macroscopic barite coating dolomites is present at shallow-platform facies sections in South China and NW Africa (Shields et al. Reference Shields, Deynoux, Strauss, Paquet and Nahon2007; Zhou et al. Reference Zhou, Bao, Peng and Yuan2010). Such mineral assemblages could suggest multiple stages of cavity/crack filling in response to a complicated interplay between eustasy and isostatic rebound and hydrothermal solutions (Shields et al. Reference Shields, Deynoux, Strauss, Paquet and Nahon2007; Zhou et al. Reference Zhou, Bao, Peng and Yuan2010, Reference Zhou, Luo, Zhou, Xing and Gan2017). Dolomite was initially precipitated during glacier retreat and relative sea-level changes, and was subsequently uplifted and exposed as a result of isostatic rebound. This prompted the dolomite to experience a period of karstic dissolution followed by sequential calcite/pyrite/iron oxide/barite precipitation and quartz cementation. The wide occurrence of quartz cementation may suggest precipitation from silica-rich hydrothermal fluids (Zhou et al. Reference Zhou, Luo, Zhou, Xing and Gan2017), which was compounded by intense silicate weathering of glacial loess during the Marinoan Snowball Earth deglaciation (Penman & Rooney, Reference Penman and Rooney2019).
How long calcite precipitation postdates the initial deposition of dolomite remains unclear. Gan et al. (Reference Gan, Luo, Pang, Zhou, Zhou, Wan, Li, Yi, Czaja and Xiao2021) documented a LA-ICP-MS U–Pb age of 632 ± 17 Ma from the isopachous dolomite within the cap dolostone, which we recalculate as 629.3 ± 16.7/22.9 Ma, using IsoplotR to directly compare with our own date. This published date is within uncertainty consistent with the calcite U–Pb age of 636.5 ± 17.8 Ma in this study. Despite relatively large analytical uncertainties, these U–Pb ages deny a broad time interval between the dolomite and calcite precipitation, which means the latter should have been influenced by hydrothermal fluids associated with the early Ediacaran but not Cambrian volcanism, as previously assumed (Bristow et al. Reference Bristow, Bonifacie, Derkowski, Eiler and Grotzinger2011). Derkowski et al. (Reference Derkowski, Bristow, Wampler, Sŕodoń, Marynowski, Elliott and Chamberlain2013) reported illite K–Ar ages of c. 430 Ma from Member 4 shale and c. 325 Ma from Member 2 K-bentonite at the Jiuqunao section, which is c. 25 km from the Jiulongwan section. These dates are best explained as Ar diffusion due to the effect of resetting of the K–Ar isotope system at 430–325 Ma. Instead, these earliest periods of calcite cementation possibly ascribe to early stage fluid activities in response to eustasy and isostatic rebound not exceeding 1.6 Myrs (Shields et al. Reference Shields, Deynoux, Strauss, Paquet and Nahon2007; Zhou et al. Reference Zhou, Bao, Peng and Yuan2010), which was subsequently overprinted by c. 632 Ma hydrothermal fluid activity (Bristow et al. Reference Bristow, Bonifacie, Derkowski, Eiler and Grotzinger2011).
Cui et al. (Reference Cui, Orland, Denny, Kitajima, Fournelle, Baele, De Winter, Goderis, Claeys and Valley2019) specifically measured the C-isotope data of calcite cements within the basal cap dolostone, which reveals low δ13Ccarb values (down to –53.8 ‰) in multiple generations of vug-filling calcite cement surrounding the pre-existing dolomite crystals with positive δ13Ccarb values as high as +6.3 ‰. Such isotopic signatures occur in multiple sections in South China, including the Jiulongwan section from which samples were collected in this study. The commonly low δ13Ccarb values mean these calcite cements should have a common origin, i.e. precipitation in early Ediacaran seawater and subsequently overprinted by early Ediacaran, but not late Ediacaran or Cambrian, hydrothermal activity. These calcite cements should have formed within <3 Myr considering the interbedded tuff zircon TIMS U–Pb ages of c. 635 Ma and c. 632 Ma within the basal and lower cap dolostone. Such a time interval can be negligible considering the analytical errors (c. 7 Myr) of our calcite U–Pb age.
In this regard, both anoxic oxidation of methane (Shields et al. Reference Shields, Deynoux, Strauss, Paquet and Nahon2007; Zhou et al. Reference Zhou, Bao, Peng and Yuan2010) and thermogenic methane oxidation (Bristow et al. Reference Bristow, Bonifacie, Derkowski, Eiler and Grotzinger2011; Zhou et al. Reference Zhou, Luo, Zhou, Xing and Gan2017) could have occurred to account for the calcite + pyrite + barite + iron oxide assemblage and particularly anomalously negative δ13Ccarb values recorded in the cap dolostone. In the former case, precipitation of calcite with extremely negative 13Ccarb values would be nearly synchronous with deposition of cap dolostone, which means deglaciation was related to methane release into the atmosphere by means of destabilization of clathrates (Jiang et al. Reference Jiang, Kennedy and Christie-Blick2003; Kennedy et al. Reference Kennedy, Mrofka and von der Borch2008; Wang et al. Reference Wang, Jiang, Xiao, Li and Wei2008). In the latter case, precipitation of calcite with extremely negative 13Ccarb values should postdate the initial deposition of cap dolostone to a time interval of c. 3 Myr, which means thermogenic methane oxidation would have occurred much later in the cap dolostone. In this scenario, deglaciation would be unrelated to methane release into the atmosphere by means of destabilization of clathrates (Shields et al. Reference Shields, Deynoux, Strauss, Paquet and Nahon2007; Zhou et al. Reference Zhou, Bao, Peng and Yuan2010).
5.b. Reliability of calcite U–Pb geochronology for Precambrian application
A published tuff zircon TIMS U–Pb age from the lower cap carbonate of the Doushantuo Formation provides a direct test of our calcite laser U–Pb age. A tuff bed c. 230 cm above the base of the cap carbonate of the Doushantuo Formation yielded a zircon TIMS U–Pb age of 635.23 ± 0.57 Ma (MSWD = 0.55) (Condon et al. Reference Condon, Zhu, Bowring, Wang, Yang and Jin2005). Our results from the sampling horizon, which is c. 130 cm from the dated tuff bed, produce a calcite U–Pb age of 636.5 ± 17.8 Ma. These ages overlap within uncertainty, with only a 0.2 % offset, indicating that the calcite U–Pb age is a robust estimate of the timing of sedimentation within the quoted analytical uncertainty. Also, our calcite U–Pb age of 636.5 ± 17.8 Ma is within uncertainty of the dolomite U–Pb age of 632 ± 17 Ma (Gan et al. Reference Gan, Luo, Pang, Zhou, Zhou, Wan, Li, Yi, Czaja and Xiao2021), again indicating its robustness for age estimation. LA-ICP-MS U–Pb dating of carbonates has been applied to material with ages ranging from the Mesoproterozoic to Quaternary, but mostly to the Mesozoic to Cenozoic (see Roberts et al. Reference Roberts, Drost, Horstwood, Condon, Chew, Drake, Milodowski, McLean, Smye, Walker and Haslam2020). Because of high ratios of common to radiogenic lead, most older dates reported from Precambrian to Cambrian carbonates (Liivamägi et al. Reference Liivamägi, Šrodoń, Bojanowski, Gerdes, Stanek, Williams and Szczerba2018, Reference Liivamägi, Šrodoń, Bojanowski, Stanek and Roberts2021; Meinhold et al. Reference Meinhold, Roberts, Arslan, Jensen, Ebbestad, Högström, Høyberget, Agić, Palacios and Taylor2020) are rather imprecise (e.g. 5–12 % uncertainties 2σ). Along with the dolomite U–Pb age of 632 ± 17 Ma reported by Gan et al. (Reference Gan, Luo, Pang, Zhou, Zhou, Wan, Li, Yi, Czaja and Xiao2021), and the recently reported 1010 ± 36 Ma age from an older sedimentary sequence in South China (Lan et al. Reference Lan, Roberts, Zhou, Zhang, Li and Zhao2022), our result is one of the most precise Precambrian dates obtained so far using the LA-ICP-MS method for U–Pb carbonate geochronology. These studies highlight the practicality of the LA-ICP-MS method and demonstrate the advantages of using an in situ technique, which namely, are the ability to make use of the heterogeneous distribution of U and Pb within calcite, in the measurement of a large spread in U–Pb ratios from a single sample along with the avoidance of altered or mixed domains (Roberts et al. Reference Roberts, Drost, Horstwood, Condon, Chew, Drake, Milodowski, McLean, Smye, Walker and Haslam2020). These advantages are difficult to achieve with dissolution and dilution-based methods owing to the averaging effect of coarser sampling. The success of this study gives us confidence that reliable U–Pb carbonate ages for other Precambrian successions can be obtained. Given that Precambrian carbonate rocks contain key information regarding the coevolution of life and environment, laser ablation U–Pb dating of carbonate minerals has great potential for providing important age constraints on biological and environmental events such as the Great Oxygenation Event and the Bitter Springs Anomaly.
6. Summary and conclusions
Based on an integrated calcite LA-ICP-MS U–Pb dating and REE measurement combined with SEM and CL imaging analyses, the following conclusions can be drawn:
-
(1) Calcite cements widely occur as irregular cavity/crack fillings in the cap dolostone of the Doushantuo Formation at the Jiulongwan section, South China, where they co-occur with pyrite, iron oxide and barite.
-
(2) Petrographic observations show calcite (including cements and single finer grains) and pyrite postdate the initial deposition of dolomite but pre-date quartz cements. Some calcite cements show a bright orange CL colour, probably indicating hydrothermal overprint.
-
(3) Calcites show positive La and Gd anomalies (δGd = 1.1–1.96), a negative Ce anomaly, high Y/Ho ratios (39–61 with an average of 50) and slightly LREE depletion patterns. Some samples show positive Eu anomalies (δEu = 1.02–1.38), whereas others show slightly negative Eu anomalies (δEu = 0.79–0.96). Such REE distribution patterns suggest a dominant seawater origin with subsequent hydrothermal overprints.
-
(4) Calcite cements within the cap dolostone give a U–Pb age of 636.5 ± 7.4/17.8 Ma (2σ, MSWD = 1.6, n = 36/40), which is within uncertainty of a previous dolomite U–Pb age of 629.3 ± 16.7/22.9 Ma. These ages suggest Cambrian hydrothermal activity could not have been responsible for the formation of these calcite cements. Instead, they were probably formed during the early Ediacaran period via oxidization of methane clathrates or thermogenic methane oxidation.
-
(5) Petrographic, geochronological and geochemical data point to early Ediacaran oxidization of methane clathrates in response to complex interactions between eustasy and isostatic rebound and hydrothermal solutions.
Supplementary material
To view supplementary material for this article, please visit https://doi.org/10.1017/S001675682200019X
Acknowledgements
Paul Hoffman and an anonymous reviewer are appreciated for reviewing this manuscript and giving constructive comments. This project was funded by the National Science Foundation of China (grant 41673016 to ZWL), State Key Laboratory of Lithospheric Evolution, Institute of Geology and Geophysics, Chinese Academy of Sciences (grant SKL-Z202001 to ZWL), State Key Laboratory of Palaeobiology and Stratigraphy, Nanjing Institute of Geology and Palaeontology, Chinese Academy of Sciences (grant 193112 to ZWL), and State Key Laboratory of Geological Processes and Mineral Resources, China University of Geosciences (grant GPMR201902 to ZWL).