Background
Children with CHD suffer from deranged cardiopulmonary physiology. The underlying cardiac illness may cause airway compression, pulmonary oedema, atelectasis, pulmonary hypertension, and infections, making them susceptible to recurrent respiratory compromise. Reference Healy, Hanna and Zinman1 The need for complex surgical procedures and operative complications such as chylothorax, airway oedema, and diaphragmatic paralysis further complicates their respiratory management. Reference Healy, Hanna and Zinman1
Invasive mechanical ventilation is the conventional approach to provide respiratory support to these children. Although effective, it is associated with ventilator-induced lung injury and ventilator-associated pneumonia. In cardiac patients, prolonged ventilation is associated with increased mortality, prolonged ICU and hospital stay, and higher healthcare cost. The risk factors associated with prolonged mechanical ventilation after cardiac surgery include young age, low weight, heart failure, nosocomial respiratory infections, pulmonary hypertension, and higher risk adjustment for congenital heart surgery-1 score. Reference Tabib, Abrishami, Mahdavi, Mortezaeian and Totonchi2,Reference Shi, Zhao and Liu3
Non-invasive ventilation minimises the risks inherent to invasive mechanical ventilation while potentially improving lung recruitment and gas exchange. The use of non-invasive ventilation has steadily increased in neonatal and paediatric critical care, including cardiac critical care. Reference Morley4 Administration of non-invasive ventilation as either continuous positive airway pressure or bilevel positive airway pressure can be achieved using various interfaces. These include nasal prongs, nasal cannula, nasal and face masks. RAM cannula is one such interface composed of soft curved prongs intended to fill 80% of the nares. It is endorsed for use with low and high flow humidified oxygen by the manufacturer. Interface-specific data for RAM cannula are not well described.
Therefore, the purpose of this study is to describe the use, safety, and outcomes of RAM cannula to provide non-invasive respiratory support in paediatric cardiac patients less than one year of age.
Materials and methods
Patients
All patients under one year of age and cared for in the cardiac ICU at the Children’s Healthcare of Atlanta between June 2015 to March 2018 and treated with non-invasive ventilation using RAMTM cannula (Neotec, Valencia, CA) were included in the study. Patients supported with RAM cannula were identified using the data from electronic medical records. The use of mode of support was driven by physician choice. As an institutional practice, RAM cannula was used in bilevel positive airway pressure mode with intial settings of inspiratory positive airway pressure between 20 and 30 cmH2O, expiratory positive airway pressure of 6–10 cmH2O and a rate of 20–30 breaths per minute. Patients older than one year of age were excluded. The study population was stratified into two cohorts based on the indication of respiratory support. When non-invasive ventilation was used as an initial respiratory support modality for worsening respiratory status, events were categorised into the escalation cohort. The escalation group included all primary non-invasive ventilation events for new-onset respiratory worsening, irrespective of prior episodes of mechanical or non-invasive ventilation.
On the other hand, the de-escalation cohort included events where non-invasive ventilation was used as a step-down modality to facilitate weaning from mechanical ventilation. Only those events where invasive respiratory support was directly weaned to non-invasive ventilation were included in this cohort. Non-invasive ventilation’s success was defined as non-escalation of respiratory support in the form of need for intubation within 48 hours of instituting non-invasive ventilation. On the other hand, intubation for invasive ventilation within 48 hours of non-invasive ventilation was categorised as treatment failure. The decision and timing of intubation (failure of non-invasive ventilation) were driven by the physician caring for the patient.
Data collection
Patient demographics, clinical and surgical diagnosis, ventilation data, ICU parameters such as length of stay, duration of ventilation, complications, morbidity, and mortality were collected from data in the medical records. Mortality was defined as ICU mortality for that admission. Vital signs, arterial blood gas, and oxygenation parameters were documented at the initiation, at 12 hours, and 24 hours of instituting non-invasive ventilation. Chest radiographs performed before initiating non-invasive ventilation were assessed for the presence of pulmonary oedema, atelectasis, and pleural effusion. Once on non-invasive ventilation, repeat chest radiographs done within 24 to 48 hours were reviewed for improvement or worsening. Risk adjustment for congenital heart surgery-1 categorisation was used to stratify surgical complexity. Reference Jenkins, Gauvreau, Newburger, Spray, Moller and Iezzoni5 The institutional review board approved the study. The data used were deidentified before analysis.
Statistical analysis
The analysis was stratified based on the indication of non-invasive ventilation into escalation and de-escalation groups. Continuous variables were reported as median with interquartile ranges, while categorical variables were reported as numbers and percentages. Statistical significance was tested using the Mann–Whitney U-test for continuous variables and Chi-square/Fisher’s exact test for categorical variables. The continuous variables were recoded into clinically relevant categories and tested for association with non-invasive ventilation success using the chi-square test. To create a model for predicting non-invasive ventilation’s success, those variables with a p < 0.05 were entered into a multiple regression model using backward elimination. Hosmer–Lemeshow test was used to assess the model’s goodness of fit. For the sake of robustness, regression analysis was applied to the complete database and not stratified based on the indication. The length of cardiac ICU stay was compared between success and failure groups using Kaplan–Meier analysis. A p < 0.05 was considered significant. Statistical analysis was performed using SPSS version 23.
Results
During the study period, there were 279 events where RAM cannula was utilised as an interface for administering non-invasive ventilation. Four events were excluded because of non-invasive ventilation duration’s brevity and the consequent lack of meaningful data. Of the remaining 275 events, 139 (50.5%) received non-invasive ventilation to escalate respiratory support. The remaining 136 (49.5%) received it as a step-down modality for de-escalation of care from invasive ventilation. Of the total cohort, 191 (69.5%) responded favourably to the treatment, while 84 (30.5%) required escalation of respiratory support within 48 hours of initiating non-invasive ventilation. Figure 1 depicts the study flow, and Table 1 summarises the demographic and baseline characteristics stratified according to non-invasive ventilation’s indication (escalation versus de-escalation).

Figure 1. Flow of the study.
Table 1. Baseline characteristics among escalation and de-escalation groups
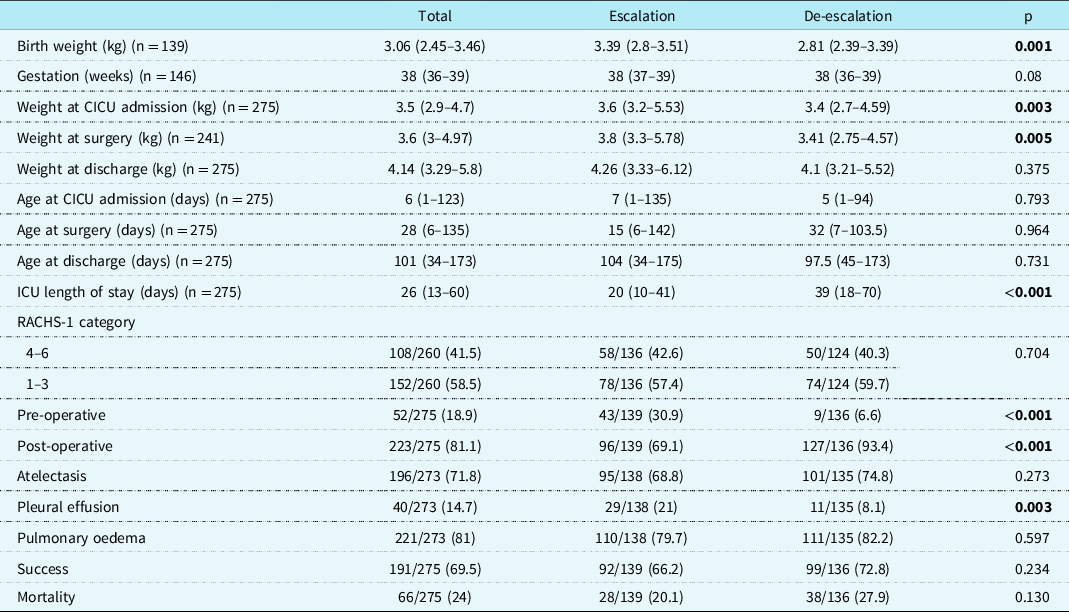
Values are median (interquartile range), or n (%).
CICU = cardiac ICU; RACHS-1 = risk adjustment for congenital heart surgery.
Significant p values are highlighted in bold.
Baseline parameters
The median age and weight at admission in our study population were six days, IQR (1–123) and 3.5 kg, IQR (2.9–4.7), respectively. Two hundred and twenty-three (81.1%) patients received non-invasive ventilation in the post-operative period. The overall success rate of non-invasive ventilation via RAM cannula was 69.5%. The escalation group differed significantly from the de-escalation group for birth weight (3.39, IQR 2.8–3.51 versus 2.81, IQR 2.39–3.39, p = 0.001), weight at cardiac ICU admission (3.6, IQR 3.2–5.53 versus 3.4, IQR 2.7–4.59, p = 0.003), weight at surgery (3.8, IQR 3.3–5.78 versus 3.41, IQR 2.75–4.57, p = 0.005), and proportion of patients with pleural effusion prior to starting non-invasive ventilation, 21%, versus 8.1% (p = 0.003). The success rate did not differ significantly between the two groups. The ICU mortality rate was 24% in the overall cohort, 20.1% in the escalation group, and 27.9% in the de-escalation group (p = 0.130).
Upon stratifying the analysis on the outcome, the success group differed significantly from the failure group in terms of older age at cardiac ICU admission (23 days, IQR 1–134 versus 2 days, IQR 0–94, p = 0.016) and a lesser proportion of patients with atelectasis before starting non-invasive ventilation, 67.5 % versus 81.7% (p = 0.019). The mortality rate was 18.8% in the success group compared to 35.7% in the failure group (p = 0.003).
Table 2 presents a summary of the underlying cardiac diagnoses of the patient population.
Table 2. Summary of cardiac diagnoses

Length of cardiac ICU stay
The median time to discharge from the cardiac ICU was analysed using the Kaplan–Meier statistic. Patients who died were excluded from this analysis. The median time to discharge from the cardiac ICU was 19 days (95% confidence interval (CI) 16.6–21.4) in the success group compared to 28 days (95% CI, 20.8–35.2) in the failure group (log-rank p = 0.006). The difference remained significant in the escalation group with a median length of stay of 14 days (95% CI, 9.7–18.3) in the success group compared to 27 days (95% CI, 17.8–36.2) in the failure group (log-rank p = 0.004). The difference approached statistical significance even in the de-escalation group, with a median length of stay of 24 days (95% CI, 14.4–33.6) in the success group compared to 47 days (95% CI, 12. 9–81.1) in the failure group (log-rank p = 0.073) (Table 3).
Table 3. The median time to discharge from the cardiac ICU

Escalation group
A total of 139 patients received non-invasive ventilation for escalation of respiratory support. Indications for escalation were primarily defined by the attending provider and were quite variable. The two most common indications were clinical worsening of respiratory distress or work of breathing in 69/139 (49%) cases and worsening atelectasis in 32 cases (23%). Less common indications were failure of high flow nasal cannula in the opinion of provider (10%) and hypoxia (2.9%).Upon stratifying results based on outcomes, the proportion of patients with pulmonary atelectasis before initiating non-invasive ventilation was 63% in the success group compared to 80.4% in the failure group (p = 0.038). All other baseline parameters were similar in these two groups. The mortality rate was 17.2% in the success and 25.5 % in the failure group (p = 0.258). At baseline, SpO2, PaO2, and pH were significantly higher, while FiO2, PaCO2, and lactate were significantly lower in the success group. The difference retained significance for FiO2 and PaCO2 at 12 hours and for FiO2 at 24 hours of non-invasive ventilation therapy (Table 4). Baseline factors associated with success were i) age at cardiac ICU admission >30 days (p = 0.048), ii) age at surgery >30 days (p = 0.034), iii) weight at surgery >3.5 kg (p = 0.029), and iv) PaCO2 ≤50 mmHg (p = 0.021). Factors associated with success at 12 hours on non-invasive ventilation were i) SpO2 ≥85% (p = 0.033), ii) FiO2 ≤40% (p = 0.011), iii) PaCO2 ≤50 mmHg (p = 0.004), iv) pH ≥7.35 (p = 0.035), and v) absence of worsening on follow-up chest X-ray (p ≤ 0.001).
Table 4. Comparison of cardiorespiratory, oxygenation, and arterial blood gas parameters in the escalation cohort between the success and failure groups

Values are median (interquartile range).
MAP = mean arterial pressure; SpO2 = oxygen saturation; FiO2 = fraction of inspired oxygen; PaO2 = arterial partial pressure of oxygen; PaCO2 = arterial partial pressure of carbon dioxide.
Significant p values are highlighted in bold.
* Missing results: Arterial blood gas was available for only two patients in the failure group due to escalation of respiratory support before the 24-hour time point, thus precluding a meaningful analysis.
De-escalation group
A total of 136 patients received non-invasive ventilation for de-escalation of respiratory support. There was no difference in baseline or demographic parameters between the success and failure groups in this cohort. The mortality was 20.2% in the success and 48.6 % in the failure group (p = 0.001). Before de-escalation, the median duration of invasive mechanical ventilation was seven days in the success group compared to ten days in the failure group (p = 0.054). Arterial pH was significantly higher at baseline,12, and 24 hours of non-invasive ventilation in the success group. At 12 hours, SpO2 was significantly higher, while FiO2 and respiratory rate were significantly lower in the success group. At 24 hours, PaO2 was significantly higher, while PaCO2 was significantly lower in the success compared to the failure group (Table 5). Baseline factors associated with success were i) duration of preceding mechanical ventilation ≤5 days (p = 0.05), ii) pH ≥7.35 (p ≤ 0.001). Factors associated with success at 12 hours on non-invasive ventilation were i) FiO2 ≤40% (p = 0.04), ii) PaO2 ≥50 mmHg (p = 0.043), iii) pH ≥7.35 (p = 0.003), and iv) absence of worsening on follow-up chest X-ray (p = 0.002).
Table 5. Comparison of cardiorespiratory, oxygenation, and arterial blood gas parameters in the de-escalation cohort between the success and failure groups
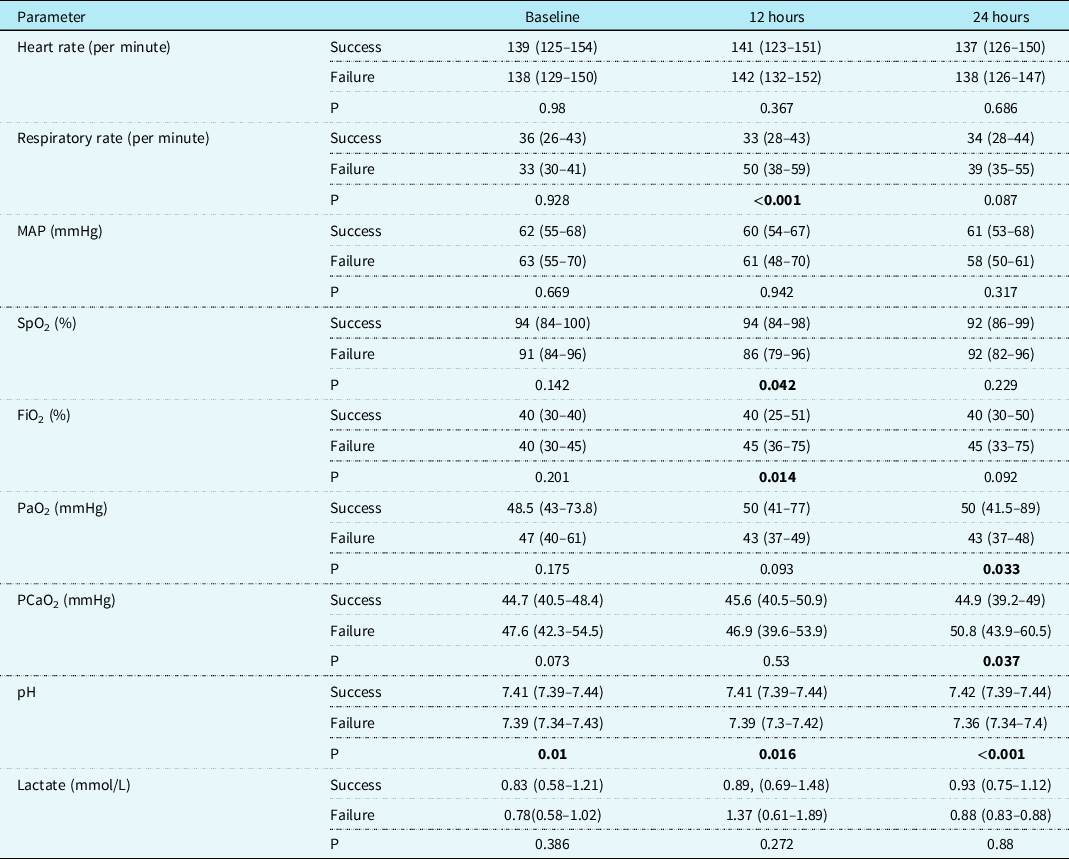
Values are median (interquartile range).
MAP = mean arterial pressure; SpO2 = oxygen saturation; FiO2 = fraction of inspired oxygen; PaO2 = arterial partial pressure of oxygen; PaCO2 = arterial partial pressure of carbon dioxide.
Significant p values are highlighted in bold.
Factors associated with success were also analyzed using multiple logistic regression incorporating the entire cohort’s data, irrespective of the indication. These results are shown in Table 6 and are consistent with the findings above for the sub-group.
Table 6. Multiple logistic regression model for the success of non-invasive ventilation

OR = odds ratio; CI = confidence interval; CICU = cardiac intensive care unit; FiO2 = fraction of inspired oxygen; PaO2 = arterial partial pressure of oxygen; PaCO2 = arterial partial pressure of carbon dioxide.
Significant p values are highlighted in bold.
Discussion
This large, single-centre study demonstrates that RAM cannula non-invasive ventilation can be used to escalate and de-escalate respiratory support with an overall success rate of 69.5%. The morbidity associated with invasive ventilation is well established in paediatric cardiac patients. Reference Rivera and Tibballs6,Reference Pinhu, Whitehead, Evans and Griffiths7 Ben-Abraham et al. reported a mortality of 15.7 % in children who required prolonged (>48 hours) mechanical ventilation after cardiac surgery compared to 4.6 % in those with <48 hours. Reference Ben-Abraham, Efrati and Mishali8 As modes of delivering adequate non-invasive ventilation continue to improve, the use of non-invasive ventilation is slowly increasing. Reference Morley4,Reference Fernandez Lafever, Toledo and Leiva9 Fernandez-Lafever et al. reported an increase from 13.2 to 29.2% in non-invasive ventilation use in children after cardiac surgery highlighting the changing paradigm in their respiratory management. Reference Fernandez Lafever, Toledo and Leiva9 Analysis of the Pediatric Cardiac Critical Care Consortium (PC4) data showed that NIV was used for 72% of neonates and infants cared for in the 15 participating cardiac ICU’s. Reference Romans, Schwartz and Costello10 Non-invasive ventilation can be provided using various interfaces. Nasal breakdown rates of 20–60% have been reported with standard non-invasive ventilation interfaces. Reference Newnam, McGrath, Estes, Jallo, Salyer and Bass11 RAM cannula is an alternative to conventional interfaces due to lesser potential for nasal discomfort and trauma due to smaller bore prongs.
The utility of RAM cannula has been reported in various clinical settings. In the neonatal ICU, Nzegwu et al. reported a 63% success rate using RAM cannula. Reference Nzegwu, Mack and DellaVentura12 Drescher et al. have reported a significant reduction in total days on respiratory support, total non-invasive respiratory support days, supplemental O2 duration, nasal injuries with a trend towards a lower incidence of bronchopulmonary dysplasia using RAM cannula in preterm neonates. Reference Drescher and Hughes13 Non-interface-specific non-invasive ventilation use has been studied in paediatric cardiac patients; however, no study focused on using RAM cannula is available. Kovacikova et al. evaluated 107 episodes of non-invasive ventilation use in 82 cardiac patients and reported a success rate of 59.8%. Reference Kovacikova, Skrak, Dobos and Zahorec14 Gupta et al. had a success rate of 77.8% in 221 events of acute respiratory failure supported with non-invasive ventilation. Reference Gupta, Kuperstock and Hashmi15 Both of these studies included patients up to 18 years of age. In comparison, the success rate of non-invasive ventilation in our study was 69.5%. A younger and smaller patient population could explain the observed difference. Although not significant, the observed success rate was higher in the de-escalation group, 72.8% versus 66.2% in the escalation group. The de-escalation group had a higher proportion of post-operative patients. Surgical correction of the underlying cardiac defect is likely to positively impact cardiorespiratory physiology, translating into a higher success rate. Gupta et al. reported a similar success rate of 74% in patients treated with non-invasive ventilation to facilitate extubation but had a much higher success rate of 79% for patients receiving non-invasive ventilation as a primary respiratory support modality. Reference Gupta, Kuperstock and Hashmi15
While analysing vital signs, arterial blood gas, and oxygenation parameters between success and failure outcomes, significant differences were identified at baseline and 12 hours on non-invasive ventilation. Better oxygenation, respiratory, and haemodynamic status before and during non-invasive ventilation lead to favourable results. It could also mean that patients in the failure group were sicker, to begin with, hence not appropriate non-invasive ventilation candidates, thus leading to failure. These baseline characteristics associated with success may also help in creating guidelines for appropriate candidacy for RAM use. Higher observed mortality in the failure group could also stem from the same reasoning. In the logistic regression model applied to the entire cohort’s data, baseline factors associated with the successful use of non-invasive ventilation were i) age at cardiac ICU admission >30 days, ii) FiO2 ≤40%, iii) PaCO2 ≤50 mmHg. Factors associated with success at 12 hours of non-invasive ventilation were i) respiratory rate ≤60/min, ii) PaO2 ≥50 mmHg, iii) PaCO2 ≤50 mmHg, iv) no worsening in chest X-ray. Patients who failed were younger at admission and had more pre-existing atelectasis. Factors associated with successful non-invasive ventilation use in paediatric cardiac patients previously described in the literature are a lower risk adjustment for congenital heart surgery-1 classification, presence of atelectasis, steroid therapy received within 24 hours of non-invasive ventilation, and normalisation of heart rate and oxygen saturation within 24 hours of non-invasive ventilation. Reference Gupta, Kuperstock and Hashmi15 A high-complexity surgical score, concomitant infection, residual cardiac defect, and pH <7.36 in the first hour of non-invasive ventilation have been reported to predict non-invasive ventilation failure. Reference Kovacikova, Skrak, Dobos and Zahorec14 The association of surgical complexity with the outcome was not replicated in our study. The longer length of ICU stay in the de-escalation cohort likely resulted from a more prolonged need for preceding invasive ventilation. These findings entail the importance of prospectively studying patient selection criteria and monitoring protocol to optimise outcomes further. As shown in the results, non-invasive ventilation’s success can translate into shorter ICU stays and reduced healthcare costs.
Limitations
The limitations of this study are due to its single centre, retrospective nature. Decisions regarding timing and threshold for escalation or de-escalation were all based on the attending clinician’s discretion. Therefore, these parameters could not be defined and standardised, and their potential impact on the outcomes could not be studied. Another limitation is that some patients either required escalation of care or improved quickly even before 12 or 24 hours’ time point, resulting in missing data. Likewise, it is also possible that some patients clinically worsened but continued on non-invasive ventilation beyond 48 hours with subsequent need for escalation of care. These did not necessarily constitute failure of non-invasive ventilation based on the definition used. We did not have data regarding specific complications related to the nasal cannula, such as nasal bridge injury or regarding causes of mortality in the cohort. Based on these limitation, there is a need to conduct a prospective study with well-defined criteria for use of non-invasive ventilation as well as guidelines around escalation and de-escalation of care to further understand appropriate use.
Conclusion
This is the single most extensive study assessing RAM cannula use in cardiac intensive care and demonstrates its applicability and safety in providing non-invasive ventilation in infants for escalation and de-escalation of respiratory support, irrespective of the underlying cardiac diagnosis and surgical status. Factors associated with successful outcomes can be used to decide candidacy and appropriate timing for instituting non-invasive ventilation. Protocolised administration and management of patients on non-invasive ventilation might further improve the results.
Acknowledgements
None.
Conflicts of interest
None.