Introduction
Trematodes are ubiquitous in coastal waters being among the most common and abundant parasites (Lauckner, Reference Lauckner and Kinne1983; Schmidt and Roberts, Reference Schmidt and Roberts2000). The life cycle of a typical trematode usually involves three hosts and three transmission stages. Adult trematodes live and reproduce within the definitive host releasing eggs in its intestinal tract. Then, the definitive host emits feces containing these eggs from which the free-living stage miracidium hatches and infects, usually a mollusc, as the first intermediate host. After maturation into rediae and/or sporocysts, the free-living cercariae stage is formed and emerges to actively seek a suitable second intermediate host where it settles as a metacercaria. Alternatively, metacercariae can settle immediately upon contact with appropriate aquatic substrata, i.e. encysting on rocks or vegetation (Morley, Reference Morley2015). For the life cycle to be completed, the parasitized second intermediate host (or the metacercaria itself) must be consumed by a suitable definitive host. Nevertheless, there are some examples of abbreviated life cycles, facultative or not, which are reduced to two hosts or even to one host possibly driven by evolutionary processes (Galaktionov and Dobrovolskij, Reference Galaktionov and Dobrovolskij2003).
Trematodes are intimately linked to molluscs as first intermediate hosts. Gastropods are the most commonly infected group, with only very few trematode lineages using exclusively bivalves as first intermediate hosts (i.e. during the life cycle they do not infect gastropods). Nevertheless, bivalves can act as the first, but more often as the second intermediate host or both; however, evidence reveals that bivalves as trematode hosts came later in the patterns of evolution (Cribb et al., Reference Cribb, Bray, Olson and Littlewood2003). The impact of trematodes on its molluscan host survival is species specific, depending on parasite abundance but is essentially related to the parasitic stage. The sporocyst (or redia) stage of a trematode life cycle was adopted in an evolutionary perspective, to successfully invade the host tissues, through asexual multiplication, resulting in a short-term rapid population growth and then expansion due to cercariae dispersal. As a result of this strong interaction between the parasite and vital organs of the host, sporocyst represents the most deleterious stage of a trematode life cycle with known effects at the metabolism, reproduction, growth and behaviour levels (Galaktionov and Dobrovolskij, Reference Galaktionov and Dobrovolskij2003; Longshaw and Malham, Reference Longshaw and Malham2013). Trematode effects at the individual level can be reflected on population performance (e.g. Friesen et al., Reference Friesen, Poulin and Lagrue2017) and community structure (Poulin, Reference Poulin1999). On the other hand, sporocyst/host relationship dynamics are much harder to fully understand due to intrinsic low prevalence (e.g. Thieltges et al., Reference Thieltges, de Montaudouin, Fredensborg, Jensen, Koprivnikar and Poulin2008; Magalhães et al., Reference Magalhães, de Montaudouin, Freitas, Daffe, Figueira and Gonzalez2017) or sudden prevalence outbreaks followed by mass mortalities (Jonsson and André, Reference Jonsson and André1992; Thieltges, Reference Thieltges2006).
In the present review, besides literature compilation, the authors take a unique opportunity to identify patterns or trends in a trematode/first intermediate host relationship using a long-term database. Cockles (Cerastoderma spp.) are the main focus of this review, known for being a key-species due to their role in ecosystem engineering (Donadi et al., Reference Donadi, van der Zee, van der Heide, Weerman, Piersma, de Koppel, Olff, Bartelds, van Gerwen and Eriksson2014), carbon sequestration (Morgan et al., Reference Morgan, O'Riordan and Culloty2013) and an important connection between trophic levels (Rakotomalala et al., Reference Rakotomalala, Grangere, Ubertini, Foret and Orvain2015). Cockle reproduction and development includes (Malham et al., Reference Malham, Hutchinson and Longshaw2012) a gametogenesis process occurring in Spring (February–March) followed by a rapid gonad development (April–May). Its sexual maturity is usually reached when cockle shell length reaches 12–14 mm, although it may be strongly driven by seasonality and other external environmental variables. Spawning can occur from May to July/August when mean temperatures are around 13°C (Boyden, Reference Boyden1970). Following fertilization, eggs are pelagic and the planktonic larval stage lasts from 2 to 5 weeks (approximately 6 mm shell length). After settlement, a cohort lifespan can reach up to 40 months, 5 cm in length and span a geographically variable range (Magalhães et al., Reference Magalhães, Freitas and de Montaudouin2016). Cockles are the habitat for 16 different trematode species (de Montaudouin et al., Reference de Montaudouin, Thieltges, Gam, Krakau, Pina, Bazairi, Dabouineau, Russell-Pinto and Jensen2009) and therefore an obvious choice to study these species dynamics. In this regard, the main objective of the present work is to review the literature to date on Cerastoderma spp. infection by Monorchis parvus and Gymnophallus choledochus, two of the four trematode species that use cockles as the first intermediate host (de Montaudouin et al., Reference de Montaudouin, Thieltges, Gam, Krakau, Pina, Bazairi, Dabouineau, Russell-Pinto and Jensen2009). This work also represents the first long-term (i.e. 21 years) description of these host/parasite systems phenology with the following specific aims: to understand infection relationship to host shell length, to examine seasonal and year effects, to depict correlations to temperature and host density, to assess co-infection with metacercariae and other sporocysts and finally to provide a molecular identification for both parasites as a quality control of the stereomicroscope morphological identification.
Material and methods
Literature review
All information gathered in this review was collected from an extensive search of the literature published before September 2019. A complete search on the Web of Science was performed using several combinations of keywords such as ‘Cerastoderma (or Cardium) edule’, ‘Monorchis parvus’, ‘Gymnophallus choledochus’, among others. The reference list of relevant papers and some grey literature was also consulted. The inclusion in the present manuscript was constrained to those studies that clearly identified the occurrence and description of both species under review with a total of 97 gathered publications (39 for M. parvus and 57 for G. choledochus).
Long-term monitoring
Sampling site description
The sampling station was located in Banc d'Arguin (44.60°N, 1.25°W), Arcachon Bay, France. Arcachon bay is a 180 km2 macrotidal lagoon situated on the Atlantic southwest coast of France. This lagoon opens to the Atlantic Ocean through a wide channel. The junction with the Atlantic is characterized by the presence of several sand banks, including Banc d'Arguin. Cockles (Cerastoderma edule) are distributed along the semi-sheltered, intertidal part of this bank. The sediment is largely dominated by medium sands [350 μm of median grain size (de Montaudouin et al., Reference de Montaudouin, Kisielewski, Bachelet and Desclaux2000)] with organic matter content not exceeding 2% (Baudrimont et al., Reference Baudrimont, de Montaudouin and Palvadeau2003). Salinity is 32–35 year round, while mean surface water temperature fluctuates between 9.5°C in winter and 21.1°C in summer (de Montaudouin et al., Reference de Montaudouin, Kisielewski, Bachelet and Desclaux2000). Since 1972, Arguin (43.6 km2) is a national reserve which is largely protected from anthropogenic activity. Sampling was performed under strict authorization.
Cockle sampling and parasite identification
During 21 years (between October 1997 and September 2018), cockles were collected monthly by sampling 6 quadrats (0.25 m2 each) and sieving them through a 1 mm mesh sieve. Cockle shell length was measured to the nearest mm with a caliper. Ten cockles per cohort (identified by size-frequency histograms) were dissected and squeezed between two glass slides for trematode observation under a stereomicroscope. All digenean trematodes were identified to the species level following several authors’ descriptions (Bowers, Reference Bowers1969; Bowers et al., Reference Bowers, Bartoli, Russell-Pinto and James1996; Bartoli et al., Reference Bartoli, Jousson and Russell-Pinto2000; Desclaux et al., Reference Desclaux, Russell-Pinto, de Montaudouin and Bachelet2006; de Montaudouin et al., Reference de Montaudouin, Thieltges, Gam, Krakau, Pina, Bazairi, Dabouineau, Russell-Pinto and Jensen2009). Trematodes found in the metacercariae stage (using cockles as the second intermediate host) were counted to assess parasite abundance, i.e. the number of metacercariae per cockle. For trematodes using cockles as the first intermediate host, it was not possible to count sporocysts and/or cercariae due to the dense mass created. Therefore, only prevalence was calculated, i.e. the percentage of infected cockles (Bush et al., Reference Bush, Lafferty, Lotz and Shostak1997). During the whole study period, a temperature probe (HOBO® Water Temp Pro v2-U22-001) was settled in the sediment of the sampling area with temperature recorded every hour.
Molecular identification
DNA isolation, amplification and sequencing
Samples for DNA analysis were removed from sporocysts found in infected cockles. For both species, three replicates were collected. Sporocysts were placed in microtubes and frozen at −20°C. Extraction of DNA was done with QIAamp DNA Micro Kit (QIAGEN) following the protocol supplied by the manufacturer. About 530 bp of 18S (small subunit ribosomal RNA gene), 600 bp of ITS1 (Internal Transcribed Spacer 1) and 300 bp of COI (cytochrome c oxidase subunit I) genes were amplified using primers Bb18S and Bb18AS for 18S (de Montaudouin et al., Reference de Montaudouin, Bazairi, Mlik and Gonzalez2014), BbITS and BbITAS for ITS1 (de Montaudouin et al., Reference de Montaudouin, Bazairi, Mlik and Gonzalez2014), TremCOIS2 and TremCOIAS2 for COI were designed. All polymerase chain reaction (PCR) and sequencing primers are described in Table 1. The PCR was performed with Gotaq G2 Flexi DNA Polymerase (PROMEGA), with 50 μL mixtures containing: 10 μL of 5X Colorless GoTaq® Reaction Buffer (final concentration of 1X), 1.5 μL of MgCl2 solution (final concentration of 1.5 mm), 1 μL of PCR nucleotide mix (final concentration of 0.2 mm each dNTP), 0.5 μL of each primer (final concentration of 1 μ m), 0.2 μL of GoTaq® G2 Flexi DNA Polymerase (5 U μL−1), 1 μL template DNA and 33.8 μL of nuclease-free water. The temperature profile was as follows for 18S and ITS1: 94°C/10 min – (94°C/60 s–59°C/30 s–72°C/90 s)×40 cycles – 72°C/10 min – 4°C, for COI: 95°C/10 min – (95°C/60 s–43°C/30 s–72°C/60 s)×40 cycles – 72°C/10 min – 4°C. Amplified PCR products were analysed by electrophoresis in a 1% p/v agarose gel stained with ethidium bromide and were sent to Eurofins Company for complete double strain sequencing, using the same set of primers as used for the PCR. Overlapping sequence (forward and reverse) fragments were merged into consensus sequences and aligned using Clustal Omega. For COI, the sequences were translated into amino acid alignment and checked for stop codons to avoid pseudogenes. All sequences obtained in this study have been deposited in GenBank. Accession numbers for M. parvus are MN544855 for 18S, MN592817 for ITS and MN547970 for COI. For G. choledochus, accession numbers are: MN544854 for 18S, MN592818 for ITS and MN547969 for COI.
Table 1. Nucleotide sequences of specific primer pairs used in this study

Data analysis
Correlation between cockle shell length classes and each associated trematode species prevalence was tested using non-parametric Spearman analysis. To test the effect of seasonality on the prevalence of M. parvus and G. choledochus, a Kruskal–Wallis ANOVA on ranks and a one-way ANOVA (selected based on the result of the Cochran test for homogeneity of variance) were, respectively, performed to compare the different months, using the 21 years as replicates. Then, to test the effect of years on trematode prevalence (M. parvus and G. choledochus), two one-way ANOVAs were performed to compare the different years using the 12 months as replicates. One year was defined as the 12 months between October N and September N + 1. These analyses were narrowed to the cockle size range susceptible to be parasitized by each trematode species (6–38 and 31–36 mm for M. parvus and G. choledochus, respectively) to avoid the dilution of prevalence values related to seasonal juvenile dominance. Mean prevalence of M. parvus and G. choledochus per year was correlated with yearly cockle density, mean surface sediment temperature of each month, each year and each previous year using non-parametric Spearman analysis. Two paired Wilcoxon tests were performed to compare the abundance of trematode species that use cockles as the second intermediate host and trematode species richness in infected vs non-infected cockles with the sporocysts of M. parvus and G. choledochus (separately analysed). For each infected cockle, a pair was constituted by randomly selecting a non-infected organism of the same shell length and same sampling date. The 21-year data matrix was checked for the occurrence of cockle coinfection by Bucephalus minimus, G. choledochus and M. parvus, three out of the four trematode species known to use this bivalve as the first intermediate host (de Montaudouin et al., Reference de Montaudouin, Thieltges, Gam, Krakau, Pina, Bazairi, Dabouineau, Russell-Pinto and Jensen2009), through proportion comparisons of the expected double infection vs the real double infection (Difference test).
Results
Monorchis parvus Looss, 1902
Literature review
Description and life cycle (Fig. 1): Monorchis parvus was originally described in 1902 (Looss, 1902) infecting teleost fishes (Diplodus spp.) of the Adriatic Sea. It is a parasite from the Platyhelminthes phylum, Trematoda class, Digenea subclass and Monorchiidae family. The typical life cycle of monorchiid trematodes includes a bivalve as the first intermediate host, the same or other bivalves as the second intermediate host and teleost fish as the definitive host (Lauckner, Reference Lauckner and Kinne1983).
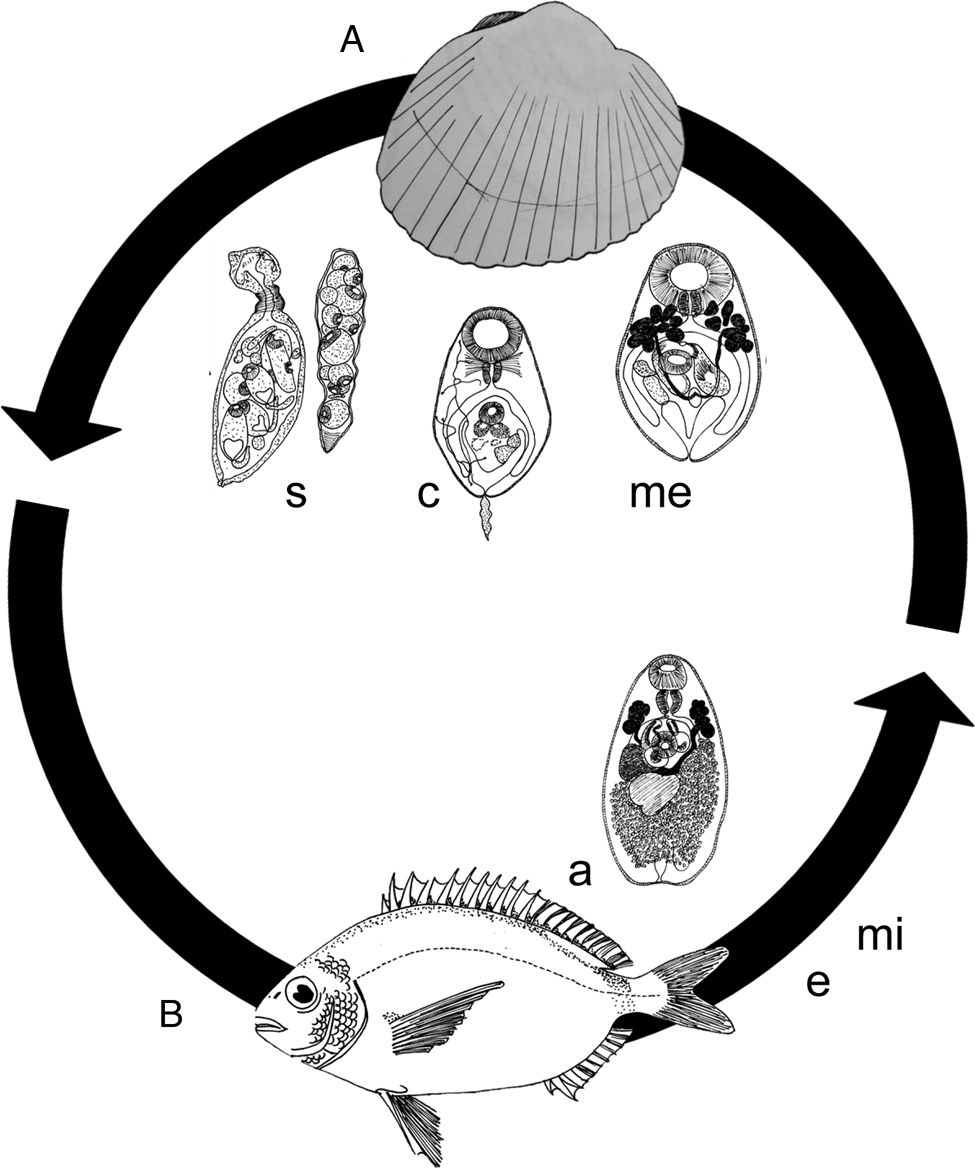
Fig. 1. Schematic representation of the Monorchis parvus life-cycle with parasite life stages and respective intermediate hosts. (A) Cerastoderma spp., the host of sporocysts (s), cercaria (c) and metacercaria (me); eggs (e) and miracidia (mi) are not represented and (B) Diplodus spp., hosts of the adult (a) form.
Over time, M. parvus was found infecting cockles (Cerastoderma spp.) but for a long time was misidentified as Distomum sp., Lepodora rachiaea, Lepidapedon rachion and received the synonym Cercaria cerastodermae I. Firstly, this species was found infecting C. edule (the edible cockle) and wrongly assigned to Distomum sp. (Lebour, 1905, 1907b) and then to L. rachiaea (Lebour, 1907a, 1912). Later on, this trematode was found infecting C. edule and C. glaucum (the lagoon cockle) but was incorrectly identified as L. rachion (the former L. rachiaea) (Bowers, Reference Bowers1965; Boyden, Reference Boyden1970) from the Lepidapedidae family, which usually also infects teleost fish as a definitive host. The mother sporocyst, the daughter sporocyst, the fully formed cercariae and the encysted metacercariae of this species were first described in 1978, infecting cockles, and renamed as C. cerastodermae I (Sannia et al., Reference Sannia, James and Bowers1978). This species was finally assigned to the correct family (Monorchiidae) maintaining the name C. cerastodermae I for 15 years (Sannia and James, Reference Sannia and James1978; Sannia et al., Reference Sannia, James and Bowers1978; Lauckner, Reference Lauckner and Kinne1983; Jonsson and André, Reference Jonsson and André1992; Russell-Pinto, Reference Russell-Pinto1993). It was only at the end of the 20th century, with morphological and molecular data (Bartoli et al., Reference Bartoli, Jousson and Russell-Pinto2000), that C. cerastodermae I (until then reported to infect cockles as the first and second intermediate host) was identified as being the same species described almost 100 years before by Looss in its adult stage (Looss, 1902), i.e. M. parvus.
Bartoli et al. (Reference Bartoli, Jousson and Russell-Pinto2000) provided thus, the first full description of M. parvus life cycle (all references gathered in Table 2): (1) the sporocysts invariably start to occur in the gonad, dispersing then to the gills, foot and visceral mass of C. edule or C. glaucum presenting a variable size range [from 445 × 143 to 919 × 162 μm (Bartoli et al., Reference Bartoli, Jousson and Russell-Pinto2000)] that depends on the sporocyst content (cercariae or metacercariae, respectively); (2) the cercariae [body length ranging from 91 to 120 μm (Bartoli et al., Reference Bartoli, Jousson and Russell-Pinto2000; de Montaudouin et al., Reference de Montaudouin, Kisielewski, Bachelet and Desclaux2000)] do not leave the first intermediate host, remaining inside the daughter sporocyst; (3) the metacercariae [variable diameter between 65 and 298 μm (Bartoli et al., Reference Bartoli, Jousson and Russell-Pinto2000; de Montaudouin et al., Reference de Montaudouin, Thieltges, Gam, Krakau, Pina, Bazairi, Dabouineau, Russell-Pinto and Jensen2009)] encystment occurs inside the first intermediate host, which is therefore also the second intermediate host; (3) the adult stages were found in wild Diplodus annularis, in wild and experimentally infected D. sargus and in wild D. vulgaris with maximum length varying between 480 and 629 μm (Bartoli et al., Reference Bartoli, Jousson and Russell-Pinto2000).
Table 2. Review of the literature regarding Monorchis parvus and Gymnophallus choledochus infection
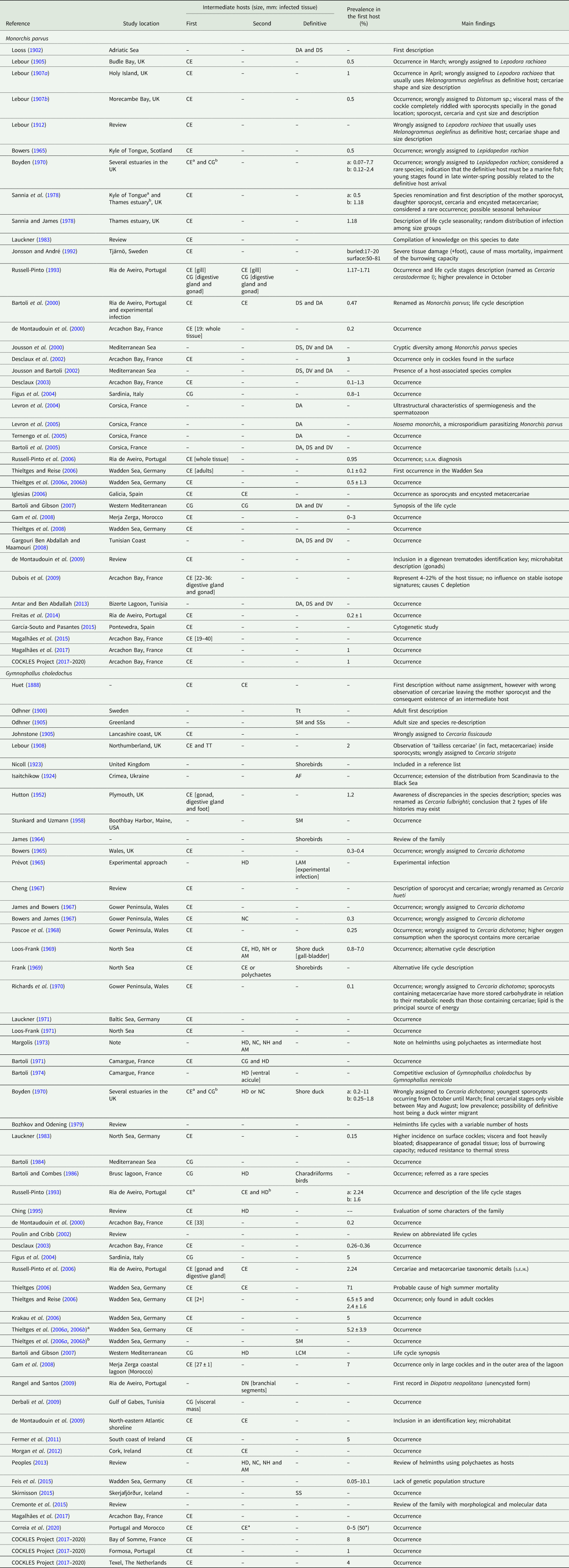
DA, Diplodus annularis; DS, D. sargus; CE, Cerastoderma edule; CG, C. glaucum; DV, D. vulgaris; TT, Tellina tenuis; HD, Hediste diversicolor; NC, Nephtys caeca; NH, Nephtys hombergi; AM, Arenicola marina; DN, Diopatra neapolitana; Tt, Tadorna tadorna; SM, Somateria mollissima; SS, S. spectabilis; AF, Aythya ferina; LAM, Larus argentatus michahellis; LCM, L. cachinnans michaelis.
Data are chronologically exhibited according to published year and separated by trematode species. Reference; study location; intermediate host species [with respective mention to host size (mean and/or range of the shell length or age) and infected tissues when available]; prevalence of infection in the first intermediate host [mean, range or both] and main findings are specified.
Distribution and prevalence: As mentioned before, M. parvus was observed for the first time in the Adriatic Sea infecting the definitive host Diplodus spp. (Looss, 1902). Since then, it was described infecting cockles from Tjärnö, in Sweden (Jonsson and André, Reference Jonsson and André1992) to Merja Zerga, in Morocco (Gam et al., Reference Gam, Bazairi, Jensen and De Montaudouin2008), including several estuaries and coastal lagoons of the European Atlantic coast [Germany (e.g. Thieltges and Reise, Reference Thieltges and Reise2006), UK (e.g. Lebour, 1905), France (e.g. de Montaudouin et al., Reference de Montaudouin, Kisielewski, Bachelet and Desclaux2000), Spain (e.g. Iglesias, Reference Iglesias2006), Portugal (e.g. Russell-Pinto, Reference Russell-Pinto1993)] and Mediterranean Sea (Bartoli and Gibson, Reference Bartoli and Gibson2007).
Monorchis parvus was often referred to as a rare trematode (Boyden, Reference Boyden1970; Lauckner, Reference Lauckner and Kinne1983), whose prevalence in cockles ranged most commonly of low values (0.07% registered in the Crouch estuary, UK by Boyden (Reference Boyden1970)) to rare episodes of high values (81% registered in Täjrnö, Sweden by Jonsson and André (Reference Jonsson and André1992)). The shell length of these infected cockles was rarely specified in the literature. Nevertheless, there is some evidence of a M. parvus random distribution within age and size groups of the first intermediate host (Sannia and James, Reference Sannia and James1978). This indicates that all age groups are equally susceptible to infection by this parasite which combined with the usual low prevalence may protect the host population as a whole. Some authors agree that M. parvus presents a seasonal behaviour with young stages most commonly found in late winter and spring (Boyden, Reference Boyden1970) and large number of developing cercariae during the summer (Sannia et al., Reference Sannia, James and Bowers1978), possibly related to definitive host seasonal migratory movement that arrives to cockles beds in late winter and leaves after spring (Boyden, Reference Boyden1970; Sannia and James, Reference Sannia and James1978).
First host effects: Monorchis parvus can occupy 4–22% of the total cockle tissue (Dubois et al., Reference Dubois, Savoye, Sauriau, Billy, Martinez and de Montaudouin2009); therefore, old mature infections (i.e. well-developed sporocysts containing mainly fully developed cercariae and metacercariae) exert an excessive destruction of the host tissues and visceral mass, consuming the host energy, inhibiting shell growth, impairing the cockles burrowing capacity, increasing the susceptibility to infection by other digenean species and consequently resulting in cockles mass mortality (Sannia and James, Reference Sannia and James1978; Jonsson and André, Reference Jonsson and André1992; Dubois et al., Reference Dubois, Savoye, Sauriau, Billy, Martinez and de Montaudouin2009).
Long-term monitoring
Shell length of cockles infected by M. parvus ranged from 6 to 38 mm. The maximal prevalence was 5.00% for the 38 mm shell length class (Fig. 2). Cockle shell length and M. parvus prevalence showed a positive correlation (R = 0.37; P = 0.02).

Fig. 2. Prevalence of Monorchis parvus (grey line) and Gymnophallus choledochus (black line) by shell length class and a respective number of dissected cockles (bars).
From the total number of sampled cockles, 5377 (i.e. 92%) were positioned in the 6–38 mm shell length range and therefore susceptible to be infected by M. parvus. Out of these cockles, 41 were infected by M. parvus with an overall prevalence of 0.76%.
The prevalence of M. parvus ranged between 0.08% in September and 1.32% in October (Fig. 3A, N = 21 years) without significant differences (Kruskal–Wallis ANOVA on ranks, P = 0.71). The prevalence of M. parvus was different among years (one-way ANOVA, P < 0.001). The Tukey post hoc test discriminated 4 years as different from the others: 1999–2000, 2006–2007, 2014–2015 and 2015–2016 with 2.48, 2.55, 3.33 and 1.67%, of prevalence, respectively (Fig. 3B).
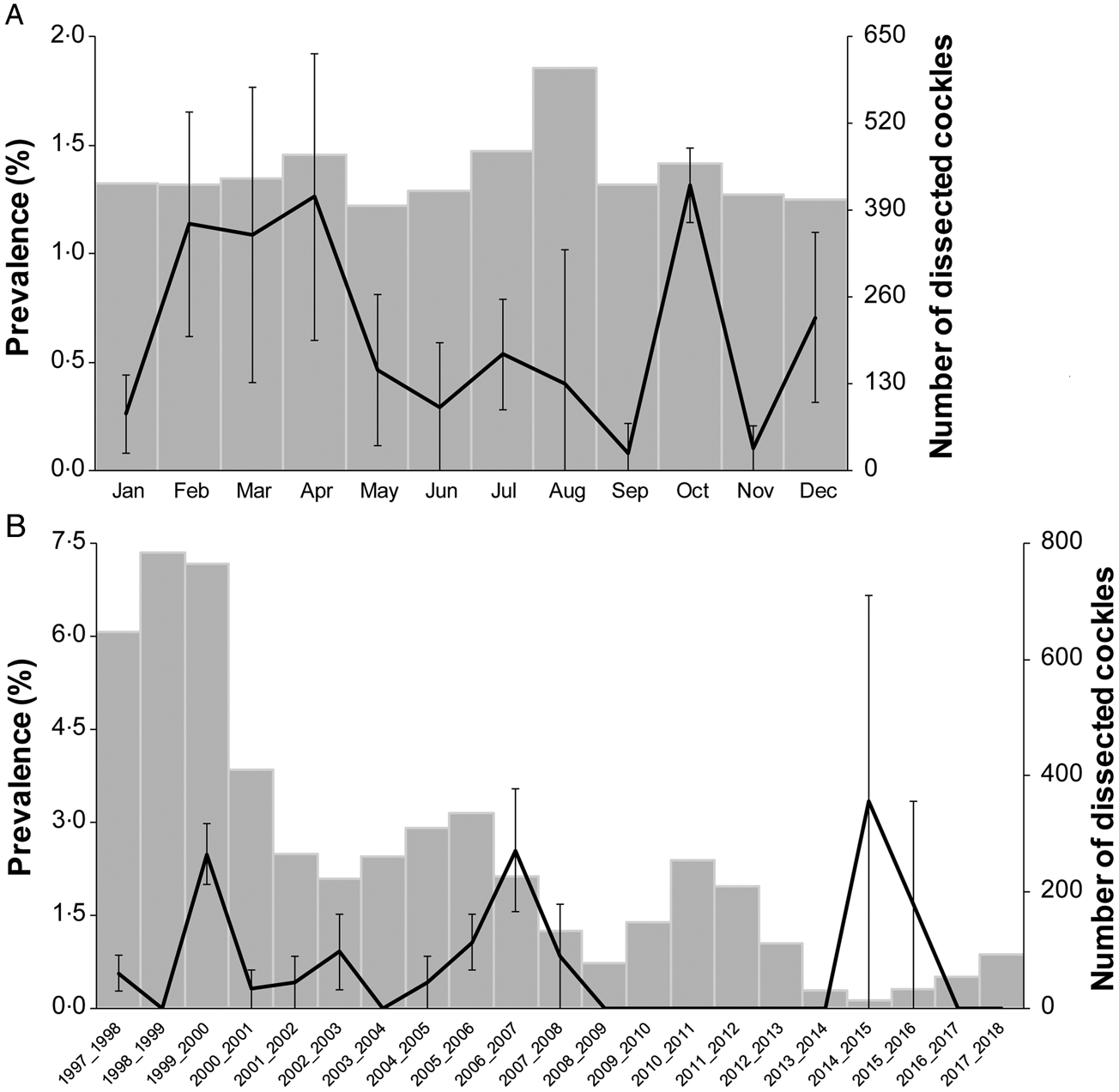
Fig. 3. Prevalence of Monorchis parvus (±standard error) and a respective number of dissected cockles placed in the parasite infection size range (6–38 mm), (A) per month gathering all sampled years and (B) per year.
There were no significant correlations between the mean prevalence of M. parvus per year and the mean temperature of each month, each year, each previous year and yearly cockle density.
The 41 pairs of cockles infected and non-infected by M. parvus were analysed considering the abundance and species richness of the other trematode species using C. edule as the second intermediate host. Species richness was the only variable that showed to be slightly but significantly lower (paired Wilcoxon test, P = 0.04) when cockles were found positive for M. parvus infection (Table 3).
Table 3. Results of Wilcoxon pairwise test for comparison between infected (+) or not infected (−) cockles in terms of trematode species abundance and species richness

Molecular identification
Sequences of 18S, ITS1 and COI were obtained for specimens previously identified with morphological criteria. The amplified product of 18S, ITS1 and COI for M. parvus presented 525, 889 and 329 bp, respectively. The ITS1 sequences were identical to those stored in Genbank (KM268112) but no identical sequences were found for 18S and COI.
Gymnophallus choledochus Odhner, Reference Odhner1900
Literature review
Description and life cycle (Fig. 4): Gymnophallus choledochus was originally described by Odhner (Reference Odhner1900) infecting its definitive host Tadorna tadorna (the common shelduck) in Sweden. It is a parasite from the Platyhelminthes phylum, Trematoda class, Digenea subclass and Gymnophallidae family, known by its furcocercous cercariae. Gymnophallids are a small group of digeneans found infecting a narrow group of marine birds as the definitive host whose typical life cycle includes bivalves as the first intermediate host and the same or other bivalves and polychaetes as the second intermediate host (Scholz, Reference Scholz and Gibson2002).
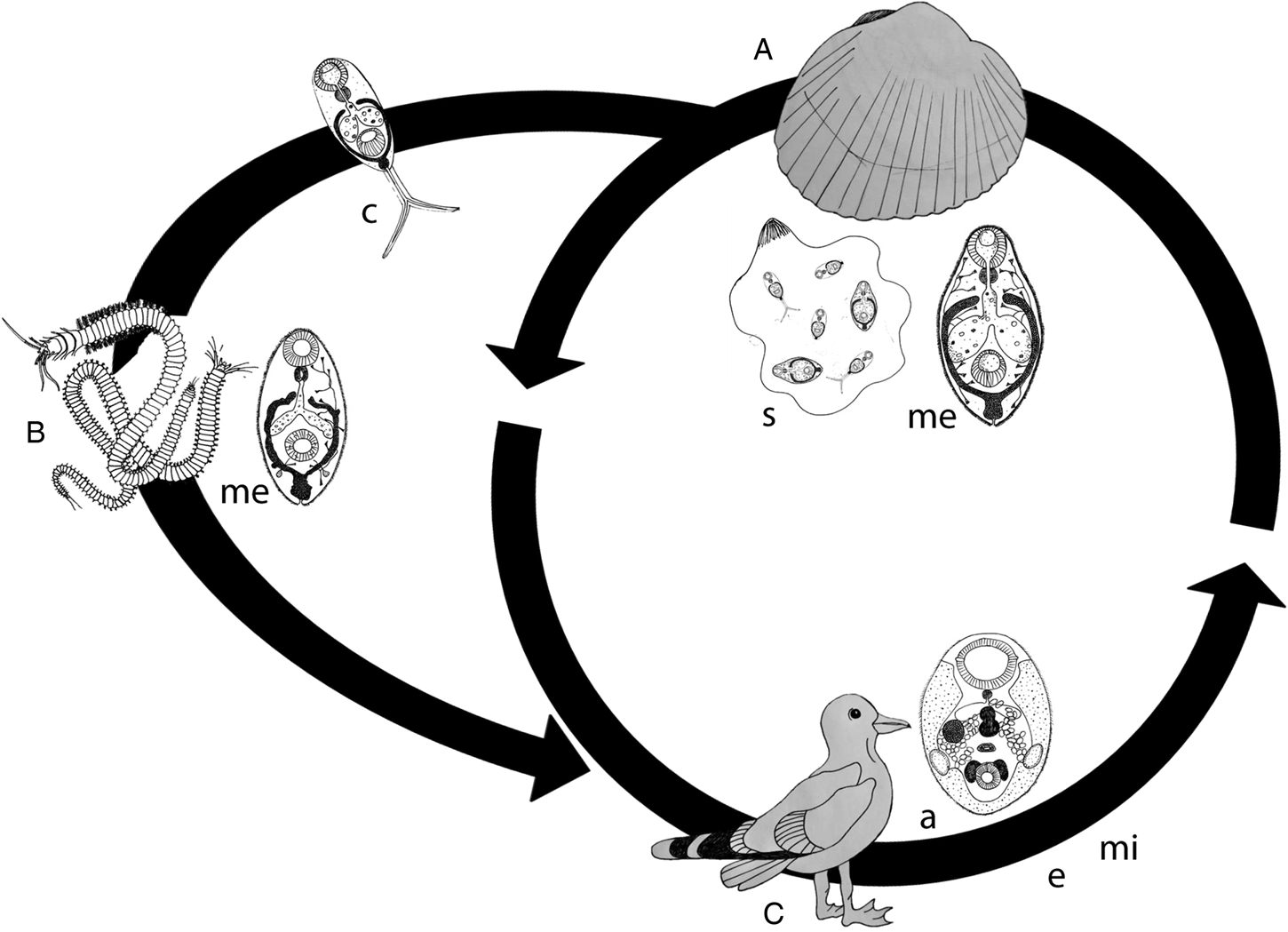
Fig. 4. Schematic representation of the Gymnophallus choledochus life-cycle with parasite life stages and respective intermediate hosts. (A) Cerastoderma spp., the host of sporocysts (s) and metacercariae (me); (B) Several polychaete species, hosts of unencysted metacercariae (me); eggs (e) and miracidia (mi) are not represented and (C) shorebirds, hosts of the adult (a) form.
In fact, the first observation of G. choledochus infecting C. edule was made by Huet (Reference Huet1888), whose work included a complete description of sporocyst and cercaria stages but without assigning a name. Since then, G. choledochus was found infecting cockles of the genus Cerastoderma but wrongly assigned to Cercaria fissicauda (Johnstone, Reference Johnstone1905), C. strigata (Lebour, 1908), C. dichotoma (Bowers, Reference Bowers1965; James and Bowers, Reference James and Bowers1967; Pascoe et al., Reference Pascoe, Richards and James1968; Boyden, Reference Boyden1970; Richards et al., Reference Richards, Pascoe and James1970) and C. hueti (Cheng, Reference Cheng and Russell1967). Gymnophallus choledochus was also previously named by its synonyms C. fulbrighti and G. fulbrighti (Hutton, Reference Hutton1952) and its life cycle was only fully described for the first time in 1969 (Loos-Frank, Reference Loos-Frank1969).
The sporocysts and cercariae of G. choledochus start to occupy the gonad of C. edule and C. glaucum (all references gathered in Table 2). Polychaetes such as Hediste diversicolor, Nephtys hombergii, N. caeca, Arenicola marina and Diopatra neapolitana are usually infected as the second intermediate host by unencysted metacercariae. This parasite can also follow an alternative pathway and settle as metacercariae in the first intermediate host (Cerastoderma spp. cockles), inside the sporocyst. This host alternation particularity of the G. choledochus life cycle was attributed either to low temperatures during the cold season (Frank, Reference Frank1969; Loos-Frank, Reference Loos-Frank1969) or due to evolutionary suppression of the polychaete second intermediate host (e.g. Russell-Pinto et al., Reference Russell-Pinto, Goncalves and Bowers2006). The adults of G. choledochus mature in the gall-bladder of gulls, ducks and wading birds.
Distribution and prevalence: Gymnophallus choledochus was found infecting cockles from the North Sea (e.g. Loos-Frank, Reference Loos-Frank1969) to the northern African coast [Morocco (Gam et al., Reference Gam, Bazairi, Jensen and De Montaudouin2008), Tunisia (Derbali et al., Reference Derbali, Jarboui and Ghorbel2009)] and other areas of the Mediterranean Sea (e.g. Bartoli, Reference Bartoli1984). However, it was mainly reported in estuaries and coastal lagoons of the European Atlantic coast: Germany (e.g. Lauckner, Reference Lauckner1971), UK (e.g. Lebour, 1908), France (e.g. Bartoli, Reference Bartoli1971) and Portugal (e.g. Russell-Pinto, Reference Russell-Pinto1993).
The prevalence of G. choledochus in the first intermediate host is often low, ranging between 0.05 and 10%, both reported for the Wadden Sea, Germany (Feis et al., Reference Feis, Thieltges, Olsen, de Montaudouin, Jensen, Bazairi, Culloty and Luttikhuizen2015), but can also reach high values [71% registered also in the Wadden Sea by Thieltges (Reference Thieltges2006)]. The few references that indicate the infected cockles size range reveal a preference of the parasite for adult hosts (Thieltges and Reise, Reference Thieltges and Reise2006; Gam et al., Reference Gam, Bazairi, Jensen and De Montaudouin2008). Similarly to what was observed with other trematode species infecting cockles as the first intermediate host (Magalhães et al., Reference Magalhães, Freitas and de Montaudouin2015), G. choledochus is considered a rare species (Boyden, Reference Boyden1970; Bartoli and Combes, Reference Bartoli and Combes1986) which consequently makes difficult to study patterns such as infection seasonality. Nevertheless, Boyden (Reference Boyden1970) noted the youngest sporocyst stages of G. choledochus occur from October until March with final cercarial stages only visible between May and August and Thieltges (Reference Thieltges2006) reported a G. choledochus outbreak in summer.
First host effects: The sporocyst (or redia) stage of a trematode life cycle multiplies asexually, ultimately invading all host tissues and therefore being the most deleterious stage (Lauckner, Reference Lauckner and Kinne1983), with the ability to impair some of the basic functions of its host. Gymnophallus choledochus is not an exception, reported as causing bloating of the viscera and foot of cockles, the disappearance of gonadal tissue, being even able to reduce or disrupt their burrowing capacity and resistance to thermal stress (Lauckner, Reference Lauckner and Kinne1983). Gymnophallus choledochus was reported as the probable cause of cockles occurring on the sediment surface (Lauckner, Reference Lauckner and Kinne1983). Mass mortalities (Thieltges, Reference Thieltges2006) can be caused directly by the tissue destruction described above or indirectly by energy depletion. Accordingly, some authors demonstrated that mature sporocysts, i.e. containing cercariae and eventually metacercariae, present higher oxygen consumption (Pascoe et al., Reference Pascoe, Richards and James1968) and higher metabolic needs (Richards et al., Reference Richards, Pascoe and James1970).
Long-term monitoring
Gymnophallus choledochus infected cockles within a narrow shell length range (31–36 mm). The maximal prevalence was 3.90% for the 36 mm shell length class (Fig. 2). Cockle shell length and G. choledochus prevalence showed a positive correlation (R = 0.36; P = 0.02).
From the total number of sampled cockles, 785 (i.e. 13%) were positioned in the susceptibility range for G. choledochus infection. Out of these cockles, six were found positive for this parasite infection with an overall prevalence of 0.76%.
The prevalence of G. choledochus ranged between 0.21% in April and 1.67% in October (Fig. 5A) without significant differences (one-way ANOVA, P = 0.78). The prevalence of G. choledochus ranged between 1% in 2002–2003 and 2.38% in 2000–2001 (Fig. 5B) without significant differences (one-way ANOVA, P = 0.52).

Fig. 5. Prevalence of Gymnophallus choledochus (±standard error) and a respective number of dissected cockles placed in the parasite infection size range (31–36 mm), (A) per month gathering all sampled years and (B) per year.
A significant positive correlation (P < 0.05) between G. choledochus mean prevalence and mean monthly temperature was only found at two occasions in April (R = 0.59) and in July (R = 0.57).
The six pairs of cockles infected and non-infected by G. choledochus were analysed considering the abundance and species richness of the other trematode species using C. edule as the second intermediate host and showed no significant differences (paired Wilcoxon text, P > 0.05) (Table 3).
Molecular identification
Sequences of 18S, ITS1 and COI were obtained for specimens previously identified with morphological criteria. The amplified products of 18S, ITS1 and COI for G. choledochus presented 554, 554 and 284 bp, respectively. The ITS1 sequences were identical to those stored in Genbank (Y18937) but no identical sequences were found for 18S and COI.
Trematode species found and sporocyst coinfection
During the whole study period (October 1997–September 2018), 5833 cockles were dissected (with shell length ranging between 1 and 59 mm) and 12 different trematode species were identified: one species using cockles exclusively as the first intermediate host – B. minimus (Bucephalidae); two species using cockles both as the first and second intermediate host – G. choledochus (Gymnophallidae) and M. parvus (Monorchiidae); and nine species using cockles exclusively as the second intermediate host – Curtuteria arguinae, Himasthla continua, H. elongata, H. interrupta and H. quissetensis from the Himasthlidae family, Parvatrema minutum, Psilostomum brevicolle, Renicola roscovitus and Diphterostomum brusinae from the families Gymnophallidae, Psilostomidae, Renicolidae and Zoogonidae, respectively.
The number of double infections found was significantly lower than the expected probability (P < 0.001). Out of the 41 cockles found infected by M. parvus, one presented a double infection with B. minimus. From the six cockles infected by G. choledochus, one was simultaneously infected by B. minimus. Monorchis parvus and G. choledochus were never found infecting the same cockle.
Discussion
Low prevalence as first intermediate host
This present first long-term data analysis of the relationship between M. parvus and G. choledochus and their first (and second) intermediate host confirms that low prevalence is the most natural scenario. Poulin and Mouritsen (Reference Poulin and Mouritsen2003) showed that among 54 species of gastropods, only 16.9% (±20.3) were infected by several trematode species (mean species richness = 3); Thieltges et al. (Reference Thieltges, de Montaudouin, Fredensborg, Jensen, Koprivnikar and Poulin2008) refer to a mean prevalence of 6.8% (±10.31) concerning 16 different trematode species using gastropods as the first intermediate host; and Magalhães et al. (Reference Magalhães, Freitas and de Montaudouin2015) reviewing the literature on a single trematode species infecting cockles as the first intermediate host showed that low prevalence is frequent. Being an outcome of long-term monthly monitoring, this study departs high prevalence outbreaks and subsequent host mass mortality as the cause for this constant low prevalence. At least in the studied area, low prevalence is more likely to be the consequence of a complex and rare interaction between abiotic and biotic factors.
It is clear in trematode ancestry studies, the close association between molluscs and parasites within which gastropods are often considered the plesiomorphic form (Galaktionov and Dobrovolskij, Reference Galaktionov and Dobrovolskij2003). In this sense, bivalve appearance in trematode life-cycle, especially as the first intermediate host, is later in the trematode/host coevolution and results from gastropod host-switching (Cribb et al., Reference Cribb, Bray, Olson and Littlewood2003). This evolutionary pattern associated with the narrow specificity of miracidium infection may be responsible for the commonly observed low prevalence. On the other hand, with evolutionary theories aside, bivalve sedentary habit (living near in the same place all their life) and the related restricted diet [focused mainly on suspended organic material (Iglesias et al., Reference Iglesias, Navarro, Jorna and Armentia1992)] can also contribute to the low prevalence of sporocysts. Convergent ideas were exposed by Bartoli (Reference Bartoli1981) when discussing a limited penetration of gymnophallid cercariae associated with limited host mobility. Simultaneously, different hosts, even belonging to the same species, may present different behaviours or phenotypes making them more or less susceptible to a given infection. As an example, trematode infection in different snails of the same species was found to be dependent not on the miracidium abundance but on each snail ecotype (Bojko et al., Reference Bojko, Grahame and Dunn2017).
Size-dependent infection
The majority (86% of the total prevalence) of M. parvus infection occurred in cockles with shell length >18 mm, indicating a preferential development of the sporocysts in mature cockles. In fact, this parasite tends to establish first and to feed in the cockles gonads (de Montaudouin et al., Reference de Montaudouin, Thieltges, Gam, Krakau, Pina, Bazairi, Dabouineau, Russell-Pinto and Jensen2009; Dubois et al., Reference Dubois, Savoye, Sauriau, Billy, Martinez and de Montaudouin2009), a more favourable microhabitat [e.g. gonads store most lipids (Fernandez-Reiriz et al., Reference Fernandez-Reiriz, Garrido and Irisarri2015)]. Therefore, the ‘adults preference’ referred by some authors concerning trematode infection (Magalhães et al., Reference Magalhães, Freitas and de Montaudouin2015 and references within) could be no more than a different time scale between miracidium penetration and sporocysts maturation among species.
Conversely to what was found with the M. parvus infection profile, G choledochus was only registered as infecting cockles in a narrow size range (>31 mm). Miracidia of the genus Gymnophallus emerge only after the eggs reach the digestive gland of the first intermediate host (passive infection) (Stunkard and Uzmann, Reference Stunkard and Uzmann1958). Thus, assuming that G. choledochus eggs have the theoretical potential to infect the cockles of any size class through filtration activity, this larger shell length ‘preference’ can be interpreted as a longer (compared to M. parvus) period between eggs entrance and sporocysts development and/or as a different level of pathogenicity among the two trematode species. However, this result could be a sign that G. choledochus has a preference for adult cockles, probably related to gonad development in the host (Thieltges and Reise, Reference Thieltges and Reise2006; Gam et al., Reference Gam, Bazairi, Jensen and De Montaudouin2008).
Finally, both trematode species were significantly more prevalent in larger cockles. Beyond what was previously stated, overall it is assumed that larger (and consequently older) cockles are more likely to be infected because they were longer exposed to parasite pressure and filter more intensively. This same positive correlation between cockles size and trematode prevalence was previously recorded (Thieltges, Reference Thieltges2008; Magalhães et al., Reference Magalhães, Freitas and de Montaudouin2015, etc.).
Infection seasonality
It was expected to find a seasonal pattern in cockle infection, at least in the case of G. choledochus due to the seasonal variation of its definitive host (marine birds), which in practice was not verified. This lack of seasonal influence on the prevalence of these two trematodes can be ascribed to constant favourable conditions for miracidium infection regardless of seasonal abiotic and biotic environmental factors. Notwithstanding, data from small samples (in this case, 10 cockles/cohort/month) must be interpreted with caution, even though they are issued over 21 years. Besides, the seasonality pattern should be preferentially assessed through evaluation over time of the larvae maturation stage rather than only assessing the presence/absence. Monorchis parvus prevalence did not follow any seasonal pattern and the same conclusion as for G. choledochus can be suggested. Monorchis parvus prevalence showed to be significantly higher in 1999–2000, 2006–2007, 2014–2015 and 2015–2016 (the latter with less expression). However, temperature does not explain these higher prevalence values, suggesting the influence of other abiotic or biotic factors is still to be identified.
Interspecific parasite co-occurrence
Neither M. parvus nor G. choledochus infection influenced the prevalence and abundance of other trematode species using cockles as the second intermediate host, contrary to what was observed in the same study area but concerning B. minimus infection (Magalhães et al., Reference Magalhães, Freitas and de Montaudouin2015). Nonetheless, those cockles infected by M. parvus presented lower trematode species richness compared to non-M. parvus-infected cockles. Interspecific competition may potentially be determining this dominance, meaning that the presence of M. parvus is able to exclude the other trematode species either by consuming the entire space (priority of occupancy) or even by direct competition. Observations of sporocysts antagonistic interaction have already been reported in a freshwater snail host (Basch et al., Reference Basch, Lie and Heyneman1969). Lower trematode species richness in cockles infected by M. parvus can also result from physiological alterations induced by this parasite invasion, such as the decrease in the host filtration capacity making it less likely to be infected by other species (de Montaudouin et al., Reference de Montaudouin, Wegeberg, Jensen and Sauriau1998). However, the literature available on cockles infection by M. parvus showed an opposite trend (§ 3.1.1), caution is needed when interpreting these results because the lower species richness found in M. parvus-infected cockles may just be the result of the difficulty to determine other species presence masked by the dense mass of M. parvus sporocysts that can represent up to 22% of the total cockle tissue (Dubois et al., Reference Dubois, Savoye, Sauriau, Billy, Martinez and de Montaudouin2009).
Similar to other mollusc hosts (Kuris and Lafferty, Reference Kuris and Lafferty1994), cockles presented no or rare double infection by parasites using this bivalve as the first intermediate host. Again, it can be the result of general low prevalence, making it less likely to detect a coinfection in the sample. However, some authors loss of host chemical attractiveness (Baiocchi et al., Reference Baiocchi, Lee, Choe and Dillman2017), physiological or behavioural alterations (Binning et al., Reference Binning, Shaw and Roche2017) or changes in the immunity (Loker, Reference Loker1994) that prevent the development of later infections.
Molecular identity of G. choledochus and M. parvus
Finally, molecular identification was performed for both species using 18S, ITS1 and COI sequences. Concerning M. parvus, ITS1 sequence matched with other sequences stored in GenBank. Surprisingly, our sequence also matched completely with a sequence of M. monorchis (Accession number: Y18937) (Bartoli et al., Reference Bartoli, Jousson and Russell-Pinto2000). However, confusion occurs because the sequence is associated with M. monorchis but details refer to M. parvus. The ITS1 sequence used for G. choledochus in the present study matched with two other sequences stored in GenBank. Our study provides for the first time 18S and COI sequences for G. choledochus. Our COI sequence showed no match with a GenBank stored sequence (Feis et al., Reference Feis, Thieltges, Olsen, de Montaudouin, Jensen, Bazairi, Culloty and Luttikhuizen2015) due to correspondence to different parts of the same gene. These data and species identity confirmation provides on the one hand a quality control of the stereomicroscope morphological identification and on the other hand will allow further confirmation (or not) of the identity of sporocysts found infecting cockles in other parts of their distribution area. Parasites found to be morphologically similar to M. parvus or G. choledochus can therefore be more accurately identified in the future.
Conclusion
Overall, this study on the occurrence of two trematode species in their first intermediate host improves our understanding of the relationship between trematode parasites and their bivalve hosts and of the processes shaping the prevalence and distribution of parasitism. By confirming that low prevalence is often the rule, this study highlighted that beyond constant trematode assemblage monitoring, there is a need to identify the main predictors of sporocysts infection, such as the definitive host presence and abundance and eggs/miracidium infection processes, for improved future management of severe host disease and mortality episodes. Diplodus spp. (Bauchot, Reference Bauchot, Fischer, Bauchot and Schneider1987) are dispersed along the whole climate gradient, although differing in abundance and diversity according to the depicted territory. In its turn, wading birds are known as long distance travellers, but fish species chose certain geographic locations that present better survival conditions (UNEP/CMS, 2014). Hence, changes in the distribution of these definitive trematode hosts during the last decades due to climate-related migration (Howard et al., Reference Howard, Stephens, Tobias, Sheard, Butchart and Willis2018; Morley et al., Reference Morley, Selden, Latour, Frolicher, Seagraves and Pinsky2018) may lead to new records of these parasites in other regions or to changes in the current prevalence patterns. Future studies are encouraged, as there are certainly numerous parasite species remaining to be described and many infection modelling processes to be identified.
Acknowledgements
The authors are grateful to the captain and the crew members of the R/V Planula IV (CNRS-INSU-FOF). Many thanks to the staff of the National Reserve of Banc d'Arguin (SEPANSO). The authors are grateful to the students who contributed to this long-term survey, among them I. Kisielewski, C. Desclaux, H. Blanchet, N. Lavesque A. Palvadeau, M. C. Sajus, H. Raigné, G. Lassalle, I. Paul-Pont, C. Dang, M. Gam, S. Dubois, L. Lanceleur, T. Do, C. Binias, H. Guyard and A. Dairain. The authors are indebted to anonymous reviewers for pertinent suggestions and improvement of the manuscript and also to Kate Mahony for English correction.
Financial support
Luísa Magalhães benefited from a post-doctoral research fellowship funded by INTERREG-ATLANTIC programme through the research project COCKLES (EAPA_458/2016 COCKLES Co-Operation for Restoring CocKle SheLlfisheries & its Ecosystem-Services in the Atlantic Area). Thanks are due to FCT/MCTES for the financial support to CESAM (UIDP/50017/2020+UIDB/50017/2020), through national funds.
Conflict of interest
None.
Ethical standards
Not applicable.