INTRODUCTION
Brazil is well known for its abundant natural resources, biodiversity, and a very productive coastal environment that allowed the proliferation of shellmounds over the last millennia (Gaspar et al. Reference Gaspar, Klokler, Scheel-Ybert and Bianchini2013). Built by fisher-gatherers, possibily gardeners (Scheel-Ybert and Boyadjian Reference Scheel-Ybert and Boyadjian2020), these archaeological sites are mainly constituted by marine mollusk shells, but also contain a variety of materials of different origins that can be constrained to specific occupational layers within the settlement. For this reason, shellmounds can be used for environmental reconstruction. The remains recovered from these sites comprise human bones, lithic tools, charcoal fragments, and both marine and terrestrial animal remains, reflecting the surrounding environment. These sites have been used, for example, to quantify the local radiocarbon (14C) marine reservoir effect (Macario et al. Reference Macario, Souza, Aguilera, Carvalho, Oliveira, Alves, Chanca, Silva, Douka, Decco, Trindade, Marques, Anjos and Pamplona2015, Reference Macario, Alves, Carvalho, Oliveira, Bronk, David, Souza, Simone and Cavallari2016), and consequently, to study ocean dynamics (Macario et al. Reference Macario, Alves, Carvalho, Oliveira, Bronk, David, Souza, Simone and Cavallari2016, Reference Macario, Tenório, Alves, Oliveira, Chanca, Netto, Carvalho, Souza, Aguilera and Guimarães2017). The presence and frequency of certain mollusk species have been used as a record for biodiversity (e.g., De Souza et al. Reference Souza, Lima and Silva2010), while fishery techniques could be inferred from the distribution of fish otoliths within the sites (Lopes et al. Reference Lopes, Bertucci, Rapagnã, Tubino, Neto, Tomas, Tenório, Lima, Souza, Jorge Domingo Briceño, Haimovici, Macario, Carvalho and Socorro2016). Even the soil in the surroundings can provide information regarding vegetation conditions during the site occupation (e.g., Coe et al. Reference Coe, Souza, Duarte, Ricardo, Machado, Macario and Silva2017). From a more archaeological perspective, the analysis of charcoal fragments and carbonized shells and bones is important for the study of funerary rituals happening at the sites, reflecting the importance of fire for these societies (cf. Bianchini and Scheel-Ybert Reference Bianchini, Scheel-Ybert, Badal, Carrión, Macías and Ntinou2012; Scheel-Ybert Reference Scheel-Ybert and Smith2018b).
In order to assess the paleovegetation, techniques such as palynology (e.g., Salgado-Labouriau Reference Salgado-Labouriau1961; Jansonius and McGregor Reference Jansonius, McGregor, Jansonius and McGregor1996), stable isotopes (e.g., Moraes et al. Reference Moraes, Fontes, Cohen, França, Pessenda, Rossetti, Francisquini, Bendassolli and Macario2017; Lorente et al. Reference Lorente, Pessenda, Oboh-Ikuenobe, Junior, Rossetti, Giannini, Cohen, Oliveira, Mayle, Franscisquini, França, Bendassolli and Macario2018), anthracology (e.g., Scheel-Ybert Reference Scheel-Ybert and Smith2018a) and phytolith analysis (e.g., Calegari et al. Reference Calegari, Madella, Brustolin, Ruiz Pessenda, Buso, Francisquini, Bendassolli and Vidal-Torrado2017; Coe et al. Reference Coe, Macario, Jenifer, Karina, Oliveira, Gomes, Carvalho, Linares, Alves and Guaciara2014) are usually employed. Whenever possible, these should be accompanied by a proper chronological evaluation of the samples so variations in different records can be accurately correlated. For this reason, it is imperative that dating methods are applied to the same entities analyzed by the techniques mentioned above. In this context, the association of anthracology and the 14C dating of charcoal from archaeological sites allows the identification of plants used in ritual practices, the understanding of the formation process of the site and the chronology of vegetation changes in a given region. Charcoal found in archaeological sites may be the result of either natural or anthropogenic processes.
Anthracology, which is the charcoal analysis and identification based on wood anatomy criteria, deals with both archaeological and pedological/geological samples. In archaeological contexts, it provides both palaeoenvironmental and landscape reconstitutions as well as palaeoethnobotanical information on the use of plants. This discipline allows reliable reconstructions of local woody vegetation, since the confrontation with phytosociological data (vegetation structure) is rather direct, and carbonized macroremains are usually abundant in all archaeological sediments (Scheel-Ybert Reference Scheel-Ybert2000, Reference Scheel-Ybert and Smith2018a). Archaeological charcoal samples have to be classified according to the context where they are found. Concentrated charcoal fragments are usually related to hearths used for various reasons (e.g., ritual, protection, cooking) and, thus, are not particularly useful for paleoenvironmental studies (Théry-Parisot et al. Reference Théry-Parisot, Chabal and Chrzavzez2010), unless it can be asserted that these features had a long duration (Scheel-Ybert Reference Scheel-Ybert and Smith2018a). This is because these events tend to have a short duration and therefore the plant assemblage they represent is statistically poor, meaning that the vegetation record preserved is incomplete and not representative of the environment where the site is located (Chabal Reference Chabal1997). Dispersed charcoal fragments, on the other hand, are considered to be a synthetic record of the surrounding vegetation, as they comprise the remains of several activities that, in the long term, result in a charcoal assemblage that reliably represents the local vegetation (Chabal Reference Chabal1997; Scheel-Ybert Reference Scheel-Ybert and Smith2018a).
The palaeoecological reliability of anthracological studies in archaeological sites has already been largely demonstrated both in temperate (Vernet Reference Vernet1977; Chabal Reference Chabal1997) and in tropical sites (Scheel-Ybert Reference Scheel-Ybert2000, Reference Scheel-Ybert and Smith2018a), and is not hampered by human agency in the transporting of wood. Although complementary to other disciplines, such as palynology, anthracological analyses also present some advantages regarding palaeoenvironmental reconstruction (Scheel-Ybert Reference Scheel-Ybert and Smith2018a). Palynology offers information on different plant series—trees, herbs, epiphytes, etc.—but overrepresents anemophilous taxa, while anthracology only provides data on woody plants, but all species tend to have an equal chance of appearing in the charcoal record (except in the case of wood selection, which can be perceived by archaeobotanical and ecological criteria). Palynology reconstructions pertain to a regional scale, because pollen grains may be dispersed for several thousands of kilometers, while anthracology reconstructions depict the local level. Taxonomic determination is consistently more precise in charcoal (often at the species or genus level) than in pollen studies (generally at the family level). In consequence, confrontation with phytosociological data is more straightforward in anthracological studies (Scheel-Ybert Reference Scheel-Ybert and Smith2018a).
The large availability of archaeological shellmounds on the south-southeastern coasts of Brazil, with abundant charcoal presence, turns these sites into important tools for palaeovegetation reconstructions. In the Rio de Janeiro State, dozens of mounds are present amongst the open ocean shores’ and the bays’ sites. Here, we focus on the Guanabara Bay, where shellmounds have an average of 3.5 m in width of archaeological pack and 7000 m2 in area (Gaspar et al. Reference Gaspar, Klokler, Scheel-Ybert and Bianchini2013). In the present work, we studied the Amourins shellmound, aiming to (1) use 14C data to study the archaeology/chronology of mound reconstruction and (2) use anthracological data (taxonomic abundances) to reconstruct the palaeovegetation and palaeoclimate during the period of mound construction and use.
Study Area and Current Knowledge
The Amourins shellmound (22°38'53.5"S, 42°56'29"W) is located in the Guapimirim municipality (Figure 1), southeastern Rio de Janeiro State. The site is situated at the plain on the northeastern of the Guanabara Bay and stands next to a meander of the Guapimirim river (Heredia et al. Reference Heredia, Beltrão, Oliveira and Gatti1981; Gaspar et al. Reference Gaspar, Klokler, Scheel-Ybert and Bianchini2013).

Figure 1 Location of the Amourins shellmound in the study area (Google Earth images).
During the transition from the Pleistocene to the Holocene, climate and vegetation in Rio de Janeiro presented several variations (Coelho et al. Reference Coelho, Barth and Chaves1999; Santos Reference Santos2000). Since the mid-Holocene, this productive environment was occupied by fisher-gatherers, possibly gardeners (Scheel-Ybert and Boyadjian Reference Scheel-Ybert and Boyadjian2020) who exploited and impacted the region (Gonzalez Reference Gonzalez2005; Lopes et al. Reference Lopes, Bertucci, Rapagnã, Tubino, Neto, Tomas, Tenório, Lima, Souza, Jorge Domingo Briceño, Haimovici, Macario, Carvalho and Socorro2016). Palynological analyses performed in sediment cores collected around the Guanabara Bay show that there have been several climatic variations and, consequently, vegetation changes in the last 6000 years, marking the transition between climates and the predominance of semideciduous seasonal forest, typical of dry environments (Santos Reference Santos2000). Between 6000 and 4000 cal BP, the dense vegetation, characteristic of humid environments, was replaced by savannah and dense campestral cover, typical of drier climates (Barros et al. Reference Barros2003). Around 5300–5030 cal BP, the Guanabara Bay was surrounded by dense vegetation, similar to the current Atlantic Forest, which has been progressively replaced by fields (Bartholomeu Reference Bartholomeu2001; Bartholomeu et al. Reference Bartholomeu, Barth and Barros2001). Studies performed on the coast of the state of Rio de Janeiro indicate the intensification of a dry and cold climate, leading to vegetation loss and the appearance of open fields during the Late Holocene (Oliveira et al. Reference Oliveira, Behling, Ledru, Barberi, Bush, Garcia, Medeanic, Barth, Scheel-Ybert and Gouveia2005).
Amador and Ponzi (Reference Amador and Ponzi1974) recorded the occurrence of a paleomangrove on the coast of the city of Magé at 4130 ± 150 BP (5030–4150 cal BP 2 σ), close to Guapimirim (Figure 1). It is believed that until the arrival of the colonizers, the entire length of the coast was occupied by mangroves (Amador Reference Amador1997). Pollen analyses on sediment cores recovered from the Guanabara Bay indicate the presence of Restinga Forest, open field formations, inundated forest, rainforest (Atlantic Forest), and mangroves (e.g., Aguiar et al. Reference Aguiar2005). At the beginning of the 16th century, different indigenous cultures inhabited the region when the Europeans arrived. Mountains, forests and swamps have been destroyed for the construction of waterways and the establishment of agricultural land (Costa Reference Costa2015; Lessa Reference Lessa2000). The Europeans would dispute over the land and even the sea, to establish residence and economic activities (Coelho Reference Coelho2007). In the 19th and 20th centuries, embankments were constructed over lagoons and swamps, giving origin to several areas that today are traditional places in the city of Rio de Janeiro (Coelho Reference Coelho2007).
After the colonial period, anthropogenic activities intensified and drastically modified the environment. Industrial activity and increasing population in the coastal areas have been gradually transforming the landscape, once dominated by diverse vegetation.
Studies performed on recent fluvial sediments (310 ± 50 BP; 410–150 cal BP, 2 σ) have shown an abrupt change in the corresponding palynological record, characterizing the anthropogenic action in the region when the coffee culture begun in the middle valley of the Paraíba do Sul river (Barros et al. Reference Barros, Barth, Mello, Moura and Peixoto2000; Souza Reference Souza2005). The present regional vegetation would be a reflection of the constant deforestation that occurred during that period. The current presence of industries and habitational buildings around the Guanabara Bay led to the loss of the natural beauty of this region and, associated with this, environmental degradation compromises the biodiversity of the bay (e.g., Soares-Gomes et al. Reference Soares-Gomes, Da Gama, Neto, Freire, Cordeiro, Machado, Bernardes, Coutinho, Thompson and Pereira2016).
Although vegetation changes in this region are widely known, they still lack a temporal framework and proper association with climate and anthropogenic phenomena.
MATERIALS AND METHODS
The Amourins shellmound (Figure 2) was constructed over muddy sediments from the Guanabara Bay and lost most of its original area due to river floods. During the 1980s, the mound was 120 m long, 60 m wide, and 3.0 m high, with approximately 22 m3 volume (Heredia and Beltrão Reference Heredia and Beltrão1980). According to Gaspar et al. (Reference Gaspar, Klokler, Scheel-Ybert and Bianchini2013), the present volume of the mound is not over 1.7 m3 (60 m long, 10 m wide, 2.8 m high). Previous zooarchaeological studies in this site had identified specimens of fish (e.g., Ariidae, Micropogonias furnieri and Pogonias cromis) and mollusks (e.g., Phacoides pectinata, Anomalocardia brasiliana and Ostrea) that are commonly found in mangroves, bays and estuaries, suggesting the occurrence of this kind of environment near the site (Cardoso Reference Cardoso2013). Similar to other shellmounds in southeastern and southern Brazil, the funerary ritual seems to have played an important role in the formation of Amourins, as indicated by the association between human burials, artifacts and combustion structures (Gaspar et al. Reference Gaspar, Klokler, Scheel-Ybert and Bianchini2013), as well as by the large quantity of fish bone and ochre in the funerary layer (Cardoso Reference Cardoso2013; Gaspar Reference Gaspar2016). In these sites, fish bones are not domestic waste, but they have been associated with ceremonial feasts and funerary offerings, while shells are presently interpreted as construction material (Gaspar et al. Reference Gaspar, DeBlasis, Fish and Fish2008; Gaspar et al. Reference Gaspar, Klokler, Scheel-Ybert and Bianchini2013; Klokler et al. Reference Klokler, Gaspar and Scheel-Ybert2018).

Figure 2 Profile of the Amourins Shellmound (source: Gaspar et al. Reference Gaspar, Klokler, Scheel-Ybert and Bianchini2013; modified).
The samples were collected during archaeological interventions performed by a team from the National Museum of the Federal University of Rio de Janeiro (MN/UFRJ), the National School of Public Health (FIOCRUZ), and collaborators from other institutions (Gaspar et al. Reference Gaspar, Klokler, Scheel-Ybert and Bianchini2013). Archaeological excavations were performed according to cultural levels but also following a 10 cm stratigraphic control. All artifacts, ecofacts and sediment samples were labelled with a provenance number (PN).
Five archaeological layers composed by mollusk shells and sediments and containing different proportions of fish bones, lithic and bone artifacts, were identified (Figure 3). Layer 1 is the base of the site, closest to the mangrove muddy sediments, composed essentially of oysters (80%); it is interpreted as a landfill. Layer 2 is composed of dark brown sandy sediments containing mollusk shells and fish bones, besides large hearths and burials; it was recognized as a funerary layer. Layer 3 is a thick brown layer composed of reddish mollusk shells, fragments of fish bones and small charcoal fragments. Layer 4 is a brown-grayish layer predominantly composed of mollusk shells, while layer 5 is a more compact layer formed by fragile fragments of shells, some charcoal fragments and charred material. Ash lenses are frequent between layers 4 and 5 (Cardoso Reference Cardoso2013). Previous dating for the Amourins shellmound gave results of 3800 ± 40 BP and 3530 ± 60 BP for a different sector (Gaspar et al. Reference Gaspar, Klokler, Scheel-Ybert and Bianchini2013).

Figure 3 Representation of profile 30–35 m of the Amourins site, in which the zooanthracological column was established. The archaeological layers, hearths, lenses, as well as one of the burials are highlighted (modified from Gaspar et al. Reference Gaspar, Klokler, Scheel-Ybert and Bianchini2013).
Sediment samples for anthracological and zooarchaeological analyses were collected in standard volume from a column with dimensions 2.00 m × 0.50 m excavated at the 30–35-m trench according to standard sampling methodology (Scheel-Ybert et al. Reference Scheel-Ybert, Klokler, Gaspar and Figuti2006b, Reference Scheel-Ybert, Gaspar and Mendonça de Souza2013). Charcoal samples were separated from the sediments through flotation. Short-lived samples were selected for 14C dating and were then subjected to physical and chemical pretreatment at the Radiocarbon Laboratory of the Fluminense Federal University (LAC-UFF). Conventional acid-base-acid treatment was followed by combustion and graphitization following standard protocols applied at the LAC-UFF (Macario et al. Reference Macario, Gomes, Anjos, Carvalho, Linares, Alves, Oliveira, Castro, Chanca, Silveira and Pessenda2013; Oliveira et al. Reference Oliveira, Macario, Carvalho, Moreira, Alves, Chanca, Diaz, Jou, Hammerschlag, Netto, Oliveira and Assumpção2021). 14C ages (Table 1) were calibrated with the Southern Hemisphere curve SHCal20 (Hogg et al. Reference Hogg, Heaton, Hua, Palmer, Turney, Southon, Bayliss, Blackwell, Boswijk, Bronk Ramsey, Pearson, Petchey, Reimer, Reimer and Wacker2020). Archaeostratigraphy and 14C data were used for the construction of a sequential model using the OxCal software version 4.4.2 (Bronk Ramsey Reference Bronk Ramsey1995, Reference Bronk Ramsey, Scott and van der Plicht2013). The model code is presented in Figure 4.
Table 1 Position, description, conventional, calibrated, and modeled ages of the analyzed samples.

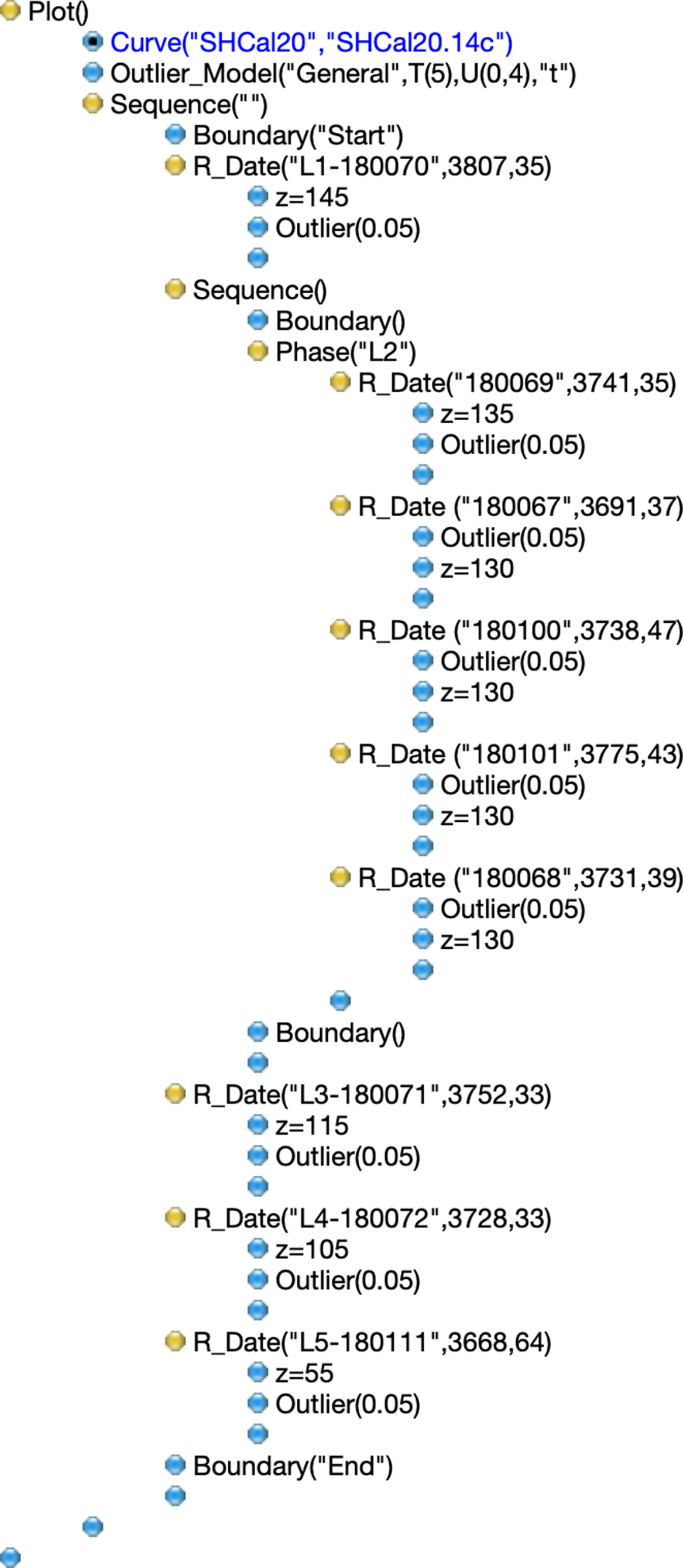
Figure 4 OxCal code for the Sequence model used after the removal of sample LACUFF 180112. Samples were ordered from layers 1–5, and a Phase model was used to represent layer 2. A general outlier model was used since the dated samples were short-lived palm nuts.
For anthracological investigations, we have analyzed 2150 charred pieces randomly picked from different sediment samples of each archaeological layer, in addition to samples associated with two burials. We established a minimum of 100 charcoal fragments to be studied from each archaeological layer, but in layer 1 only half of this was available; all the archaeological layers and features were rather poor in charcoal, hampering the analysis of a larger sample. In addition to that, we counted as many palm nuts as they appeared in the sample until the 100th charcoal fragment was reached (Table 2). In the laboratory, the charcoal fragments were manually broken in three fundamental sections (transversal, longitudinal tangential, and longitudinal radial) and observed using a brightfield/darkfield reflected light microscope. Taxonomic identification was based in analysis of the wood anatomy and aided by comparison with databases, reference collections and descriptions and images available in the literature (Scheel-Ybert et al. Reference Scheel-Ybert, Carvalho, Moura, Gonçalvez, Scheel and Ybert2006a; Scheel-Ybert Reference Scheel-Ybert and Smith2018a). (Table 3). The results were organized in an anthracological diagram in which the identified taxa were classified according to vegetation types using the C2 software (Juggins Reference Juggins2005).
Table 2 Number of sediment samples analyzed in each archaeological layer and their stratigraphic position; there seems to be overlapping in the stratigraphic levels, but this happens because the limits between archaeological layers are not linear, therefore the sample position depends on the part of the profile they were collected from. The number of charcoal pieces and number of palm nuts of the analyzed samples are also given.

Table 3 Taxa identified in the Sambaqui de Amourins organized according to the vegetation types in which they occur. “Restinga” taxa comprise genera and species that occur mostly in open restinga but may also occur in the restinga forest. “Restinga forest/Atlantic forest” taxa are those that present species in both formations or that may occur in any of these formations.

RESULTS AND DISCUSSION
Chronological Framework
Considering the 14C analyses, sample selection by anthracology guarantees the use of short-lived plant parts such as barks, twigs and nuts. In this work, 7 nutshell samples were selected to represent the 5 archaeological layers sampled within the studied sector. Three other charcoal samples were analyzed for comparison. Table 1 shows the description of each sample, the conventional, calibrated and modeled ages, the latter assuming a simple sequence, which takes into account the temporal ordering of the samples (Figure 5). A larger number of samples from layer 2 was dated because this layer was richer in charcoal and in archaeological features than the others.

Figure 5 Simple sequence model based on charcoal dates from the Amourins shellmound after removal of the outlier (sample LACUFF 180112). Light gray distributions represent the regular calibration (as individual samples) while dark gray distributions represent the modeled probabilities. Individual samples’ agreement within the model (A) and overall agreement (Amodel) are satisfactory. All individual results gave a posterior outlier probability of 3%. Median values are marked as crosses and 2 sigma ranges are presented for the modeled distributions.
The 14C ages obtained for the nut shells cover a range from 3807 ± 35 to 3503 ± 70 BP, in agreement with the available dates for the other sector (Gaspar et al. Reference Gaspar, Klokler, Scheel-Ybert and Bianchini2013). The outlier model was useful to evaluate whether the samples belong to the layers where they had been found. The results for the charcoal samples are in agreement with nutshell results for the same layers. For most of the samples, there was no significant temporal variation between the ages of each layer, since the results are statistically overlapping.
The well-defined stratigraphy of the site can be observed in the zooanthracological column representation shown in Figure 3. Based on this ordering, a sequential age model was constructed and is presented in Figure 4. A general outlier model was used to test the behavior of each date within the chronological model. For all layers a short-lived sample is present, but for the burial, a few charcoal samples were measured to evaluate their synchronicity to the model. Considering that all the results have a prior 5% probability of being outliers and the obtained overall agreement for the model, sample LAC-180112 was discarded with 22% posterior outlier probability. The Amodel index, which measures the agreement between models, is 148% after removing this outlier. According to Bronk Ramsey (Reference Bronk Ramsey2009), Amodel values above 60% statistically ensure good agreement between model and measurements. The presence of this sample can be interpreted as an intrusion, a charcoal fragment mixed with an inferior layer by disturbance of the archaeological package, for example by the opening of a stack hole.
Based on the sequential chronological model built, the period between the Start and End boundaries was considered the most likely related to the occupation of the studied sector, and therefore, to the vegetation types identified in this work. Such probability distribution is displayed in Figure 6, representing the mean of the two boundary distributions (Start and End boundaries in the sequence). The most likely period representing the dataset is then 4130–3960 cal BP (1 σ) or 4310–3800 cal BP, (2 σ).

Figure 6 Probability distribution of the interval between Start and End boundaries in the sequence. The most likely period representing the dataset is 4130–3960 cal BP (1 σ) or 4310–3800 cal BP (2 σ).
Vegetation Reconstruction
The anthracological results demonstrate that several vegetation types existed around the Amourins shellmound during the time it was occupied, including the Atlantic Forest, the restinga forest, open restinga formations and the mangrove (Figure 7). The Atlantic Forest presently covers the hills and valleys inland, while mangroves occur by the edges of lagoons and rivers. The restinga vegetation thrives on the Quaternary coastal sandy deposits; it is a mosaic of plant associations with diverse physiognomies that vary from sparse open plant communities, such as herbaceous and shrub formations (“open restinga”) to a denser evergreen forest (“restinga forest”) typical of the Brazilian coast (Araujo and Henriques Reference Araujo and Henriques1984). It is likely that during the shellmound occupation all these vegetation types already occurred along these same geomorphological settings.

Figure 7 Anthracological diagram, Sambaqui de Amourins, profile 30–35 m, in relative frequency of each taxon. Dispersed charcoal from each archaeological layer and concentrated charcoal associated with burials (layer 2) are separated by a dashed line. Nut shells are not indicated, as their frequencies are not ecologically significant. However, they are present in all samples and constitute a very good material for dating. Nsp = Number of taxa identified.
Mangrove species, especially Laguncularia sp., but also an undetermined Combretaceae (probably Laguncularia), Combretum sp., and Avicennia sp. are predominant in all samples. Clusia sp., Myrtaceae, Rubiaceae, and Melastomataceae species follow as the most frequent taxa, attesting the importance of restinga formations in the region. The Atlantic Forest is represented by only three taxa (Xylopia sp., Luehea sp., Mouriri sp., see Table 3), which are limited to the two most recent archaeological layers, indicating that this type of vegetation was less used for firewood gathering (Figure 7).
Several typical restinga taxa, together with taxa that may occur both in the restinga and the Atlantic forest are present in both burials and in the profile layers, indicating that they were common species. This corroborates the results of previous studies, which demonstrated that shellmound builders were strongly associated with the restinga environment (Scheel-Ybert Reference Scheel-Ybert2000, Reference Scheel-Ybert and Smith2018b).
Palm nuts were the most abundant charred remains in all five archaeological layers, especially in the first one; they are less frequent in the burials, when compared to charcoal, but still preponderant (Table 2). Although their prevalence indicates the importance of palms in the surrounding environment, their frequencies are not ecologically significant since they are purposely collected as food.
Despite minor variations, species richness and composition are overall very similar in all archaeological layers and burial features analyzed. Layer 1 stands out for the scarcity of charcoal remains, low species richness and strong dominance of palm nuts. Layer 2 presents the highest species richness in all the assemblage; the burial features analyzed (which are situated in layer 2) present lower species richness, but this is expected for short-duration fire activities which characterize hearths. Layers 3, 4, and 5 are very similar in species richness and composition, though Atlantic forest elements are restricted to layers 4 and 5.
Together, the 14C and anthracology results show that at 4130–3960 cal BP typical restinga and mangrove vegetations were well represented in the area around the Guanabara Bay restinga. Atlantic forest elements were rarer, but this is due to the fact that this vegetation was situated farther inland.
Previous palaeoenvironmental and landscape reconstructions based on anthracological studies at the Southeastern and Southern Brazilian coasts attest that shellmounds used to be established in the restinga environment, but always in the range of access to other vegetation types, especially the mangrove and the Atlantic Forest (Scheel-Ybert Reference Scheel-Ybert2000; Scheel-Ybert et al. Reference Scheel-Ybert, Eggers, Wesolowski, Petronilho, Boyadjian, DeBlasis, Barbosa-Guimarães and Gaspar2003, Reference Scheel-Ybert, Bianchini and DeBlasis2009; Scheel-Ybert and Dias Reference Scheel-Ybert and Dias2007; Bianchini et al. Reference Bianchini2011). Mangrove elements have already been recorded in several sites (Forte, Boca da Barra, Salinas Peroano, Ponta da Cabeça, Corondó, Pontinha and Beirada, in the Lagos Region, southeastern Rio de Janeiro State; Jabuticabeira-II and Encantada-III in Santa Catarina State, Southern Brazil). However, higher frequencies of mangrove in relation to other vegetation types were only verified in the more recent levels of site Ponta da Cabeça (at ca. 2080 ± 40 BP, or 2100–1890 cal BP) and in the earlier levels of site Encantada-III (at ca. 4320 ± 40 BP, or 5030–4640 cal BP). In both cases, the prevalence of mangrove is probably related to human choices in the firewood gathering, and not to environmental changes (Scheel-Ybert Reference Scheel-Ybert2000; Scheel-Ybert et al. Reference Scheel-Ybert, Bianchini and DeBlasis2009).
Environmental changes were recorded by oscillations in the mangrove vegetation in sites from southeastern Rio de Janeiro State (southeastern Brazil). This study recorded at least two more humid episodes (from ca. 6000 to 5500/5000 cal BP and from ca. 2300 to 2000 cal BP), intercalated with two episodes of increased dryness with enhanced lagoon salinity (from ca. 5500/5000–2300 cal BP and from ca. 2000 to 1200 cal BP) (Scheel-Ybert Reference Scheel-Ybert2000). These episodes were correlated with modifications of lagoon sedimentation, which also suggest regional climatic variations during the Holocene (Tasayco-Ortega Reference Tasayco-Ortega1996). During the same period, however, the coastal restinga vegetation has not suffered any considerable variations, which was attributed to the edaphic character of coastal restinga formations, adapted to sandy soils, and therefore much more resilient to climatic variations and climate changes. Even though the coastal geomorphology was in permanent change during the Holocene, especially due to significant sea level variations, the coastal plains remained during all this time covered by the restinga vegetation, of which the structure and floristic composition remained relatively stable (Scheel-Ybert Reference Scheel-Ybert2000).
The results obtained from the Amourins site, in turn, stand out for the strong dominance of mangrove taxa in all samples. The site location explains these results. Indeed, Amourins is situated next to a meander of the Guapimirim river, which was certainly covered by a mangrove swamp during the site’s construction and occupation, and plants from the mangrove provided most of the firewood used in this site. The restinga, however, was also present during the site occupation, as attested both by the anthracological results presented here (in which restinga taxa are the second most important vegetation in frequency of charcoal pieces) and by the geomorphological nature of the area (Suguio and Tessler Reference Suguio and Tessler1984).
Sedimentological analysis in this same area also recorded paleomangroves at 4130 ± 150 BP (5030–4150 cal BP) (Amador and Ponzi Reference Amador and Ponzi1974). Only remnants of these mangroves exist in the present day, but they were still abundant at least until the 18th century, when European colonization increased the persistent destruction of the native vegetation (Amador Reference Amador1997).
Palynological studies in different sites around the Guanabara Bay recorded the occurrence of the Atlantic Forest, restingas, savannas, mangroves, and marshes since at least 4520 ± 80 BP, 5440–4860 cal BP (Barreto et al. Reference Barreto, Barth, Luz, Baptista Neto and Vilela2005; Barros and Barth Reference Barros and Barth2005; Bartholomeu et al. Reference Bartholomeu, Barros, Lopes, Vilela and Barth2014). These studies detected environmental degradation due to European colonization through the increase in field vegetation and the presence of exotic pollen grains in historical times (after 530 ± 25 BP, 540–500 cal BP). Barros and Barth (Reference Barros and Barth2005) highlight that regional vegetation is better represented in their analyses than local vegetation, which may explain the high importance of Atlantic Forest elements in most palynological records.
All these studies agree in presenting a scenario where different plant formations occurred around the Guanabara Bay during the Late Holocene. The humid Atlantic Forest thrived on the hills and valleys inland; the various restinga formations (including restinga forests and different open restinga facies) occupied the coastal plains; marshes and herbaceous vegetation thrived in the edges of the bay and coastal lagoons and in depressed areas; mangrove swamps occurred in river margins and also in some places by the edges of the bay and of coastal lagoons.
CONCLUSIONS
This work shows the results of the association of the techniques of anthracological analysis and 14C dating in archaeological charcoal samples from the Amourins shellmound, located in the northeastern portion of Guanabara Bay. The application of both techniques to the same sample enhanced the accuracy of the results used in the construction of a sequential model. This allowed for a representation of the environmental picture of Guanabara Bay during the Holocene. Such information benefits not only the study and cataloguing of the species that have occupied the area, but also serves as a base for the implementation of actions and projects aimed at the recovery of the region, remodeling, and revitalizing the coastal ecosystem, highly degraded today.
The presence of several families and genera characteristic of the Atlantic Forest, restinga forest, open restinga and mainly mangrove in the site’s successive layers confirms that from ca. 4040 cal BP these vegetation types resisted the climatic changes and were perpetuated in the place, remaining until the present day, despite having been greatly affected by anthropogenic interference. The dates show a period of about 220 years when the major dominance of the vegetation cover was characteristic of estuarine environments.
ACKNOWLEDGMENTS
The authors would like to thank Brazilian financial agencies CNPq (Conselho Nacional de Desenvolvimento Científico e Tecnológico, 307771/2017-2 and INCT-FNA, 464898/2014-5) and FAPERJ (Fundação Carlos Chagas Filho de Amparo à Pesquisa do Estado do Rio de Janeiro, E-26/110.138/2014 and E26/203.019/2016) for the financial support. This study was financed in part by the Coordenação de Aperfeiçoamento de Pessoal de Nível Superior - Brasil (CAPES) - Finance Code 001. The authors thank the team of the National Museum of the Universidade Federal do Rio de Janeiro, especially Maria Dulce Gaspar, coordinator of the project “Sambaquis Médios, Grandes e Monumentais.” We thank the reviewers and editors for their valuable comments and suggestions.