Introduction
The Colorado potato beetle, Leptinotarsa decemlineata (Say), is an oligophagous insect that feeds on plants of the family Solanaceae, primarily on cultivated potato, Solanum tuberosum L. (Hare, Reference Hare1990). Rapid food consumption, high fecundity and a remarkable adaptability to diverse environments make it one of the most destructive pests worldwide (Cingel et al., Reference Cingel, Savić, Lazarević, Ćosić, Raspor, Smigocki and Ninković2016). The use of chemical insecticides is still the main strategy for L. decemlineata management. Populations of L. decemlineata have developed resistance to most pesticide chemistries (Alyokhin et al., Reference Alyokhin, Baker, Mota-Sanchez, Dively and Grafius2008; Huseth et al., Reference Huseth, Groves, Chapman, Alyokhin, Kuhar, Macrae, Szendrei and Nault2014; Brevik et al., Reference Brevik, Schoville, Mota-Sanchez and Chen2018). Due to concerns about the development of insecticide resistance and the human health consequences of insecticide exposure (Pandian and Ramesh, Reference Pandian, Ramesh, Srivastava, Singh, Singh, Singh, Prasad, Tripathi and Chauhan2020; Upadhayay et al., Reference Upadhayay, Rana, Juyal, Bisht, Joshi, Srivastava, Singh, Singh, Singh, Prasad, Tripathi and Chauhan2020), the search for alternative, eco-friendly strategies for managing L. decemlineata populations has intensified (Sablon et al., Reference Sablon, Dickens, Haubruge and Verheggen2012).
One strategy relies on the use of plant secondary metabolites that have coevolved as a defence mechanism against herbivorous insects (Isman and Miresmailli, Reference Isman, Miresmailli and Paluch2011; Miresmailli and Isman, Reference Miresmailli and Isman2014). Secondary metabolites, including essential oils (EOs), can be extracted from aromatic plants belonging to Myrtaceae, Lauraceae, Lamiaceae and Asteraceae families, and represent complex mixtures of terpenes, terpenoids and aliphatic components (Isman and Miresmailli, Reference Isman, Miresmailli and Paluch2011; Ntalli and Menkissoglu-Spiroudi, Reference Ntalli, Menkissoglu-Spiroudi and Stoytcheva2011; Regnault-Roger et al., Reference Regnault-Roger, Vincent and Arnason2012; Pavela and Benelli, Reference Pavela and Benelli2016; Chaubey, Reference Chaubey2019). Many studies have reported repellent, antifeedant and insecticidal activities of EOs and other secondary metabolites, as well as their influence on various fitness and biochemical/physiological traits of insects (Szczepanik et al., Reference Szczepanik, Dams and Wawrzeńczyk2005; Isman, Reference Isman2006; Pavela et al., Reference Pavela, Sajfrtová, Sovová, Karban and Bárnet2009; Rattan, Reference Rattan2010; Kim and Lan, Reference Kim and Lan2011; Jumbo et al., Reference Jumbo, Faroni, Oliveira, Pimentel and Silva2014; Kiran et al., Reference Kiran, Kujur, Patel, Ramalakshmi and Prakash2017; Tak and Isman, Reference Tak and Isman2017). Insecticidal and antifeeding effects of plant extracts and EOs have been recorded in L. decemlineata (Kostić et al., Reference Kostić, Dražić, Popović, Stanković, Sivčev and Živanović2007; Gökçe et al., Reference Gökçe, Isaacs and Whalon2012; Bekircan et al., Reference Bekircan, Cüce, Bekircan and Tosun2015). Compared to conventional insecticides, the use of EOs in pest management has several advantages. EOs contain many components with diverse mechanisms of action that slow down the evolution of physiological and/or behavioural adaptations in target organisms. A very small number of EOs and their dominant components are toxic to mammals (Isman, Reference Isman2006). Due to the high volatility and low persistence and an outdoor half-life of less than 24 h, EOs are relatively safe for the environment (Regnault-Roger et al., Reference Regnault-Roger, Vincent and Arnason2012).
In the current study, we evaluated the use of EOs from Tanacetum parthenium and Tanacetum vulgare (Asteraceae family) in L. decemlineata control. These two perennial species are native to Eurasia and naturally occur in the flora of Serbia (Ranđelović et al., Reference Ranđelović, Zlatković and Jušković2005). T. parthenium and T. vulgare EOs, as well as their dominant components, camphor and thujone, express strong biological activity against many harmful insects (Brewer and Ball, Reference Brewer and Ball1981; Hough-Goldstein and Hahn, Reference Hough-Goldstein and Hahn1992; Gabel and Thiery, Reference Gabel and Thiery1994; Tsao et al., Reference Tsao, Lee, Rice, Jensen, Coats, Baker, Fenyes and Basarab1995; Larocque et al., Reference Larocque, Vincent, Belanger and Bourassa1999; Riddick et al., Reference Riddick, Aldrich, De Milo and Davis2000; Pavlidou et al., Reference Pavlidou, Karpouhtsis, Franzios, Zambetaki, Scouras and Mavragani-Tsipidou2004; Pavela et al., Reference Pavela, Sajfrtová, Sovová, Bárnet and Karban2010; Szołyga et al., Reference Szołyga, Gniłka, Szczepanik and Szumny2014; Czerniewicz et al., Reference Czerniewicz, Chrzanowski, Sprawka and Sytykiewicz2018; Devrnja et al., Reference Devrnja, Kostić, Lazarević, Savić and Ćalić2020). Treating potato leaves with extracts of T. vulgare decreased survival and retarded the growth of L. decemlineata larvae (Ploomi et al., Reference Ploomi, Luik, Metspalu and Hiiesaar2006; Ertürk and Uslu, Reference Ertürk and Uslu2007).
In addition to the lethal effects of EOs, modification of behaviour at sublethal insecticide concentrations may also provide protection to crops (Hummelbrunner and Isman, Reference Hummelbrunner and Isman2001). For example, the push-pull control strategy uses repellent substances to move pests away from protected crops to traps and trap crops that can be treated with attractants (Cook et al., Reference Cook, Khan and Pickett2007). In a broad sense, repellency is related not only to movement away from an odour source, but also to the inability to find or recognize the host. According to this broad definition, repellent substances may provoke a behavioural response without direct contact or after direct contact with a pest (Deletre et al., Reference Deletre, Schatz, Bourguet, Chandre, Williams, Ratnadass and Martin2016). Antifeedants that disrupt feeding behaviour pre- or post-ingestively, as well as compounds that inhibit host attraction, are considered to be repellents.
Investigations of the behavioural responses of L. decemlineata to Tanacetum oils are scarce. The study of Panasiuk (Reference Panasiuk1984) pointed to avoidance behaviour of L. decemlineata adults in response to potato leaves treated with a single concentration of T. vulgare EO, while Kostich et al. (Reference Kostich, Ristich, Zabel, Sekeschan and Gaschich1993) confirmed an antifeedant effect of a single concentration of T. parthenium EO on second instar larvae. It has been previously demonstrated through olfactometer assays that starved female beetles will not feed on leaf discs treated with T. vulgare or T. parthenium EOs (Kostić et al., Reference Kostić, Stanković, Ristić, Jevđović and Rajković2003).
In the current study we examined the repellent effects of three concentrations of T. parthenium and T. vulgare EOs in multiple different assays, including direct contact of third instar larvae and adults with Tanacetum oils. Our factorial experimental design (two Tanacetum EOs × two developmental stages × three EO concentrations) allowed for the exploration of the main and interaction effects of the three factors and addressed the following questions, as follows: (1) Are the repellent effects of Tanacetum EO species-, developmental-stage- and/or concentration-specific? (2) Does the sensitivity of L. decemlineata to increasing EO concentrations depend on the Tanacetum species and/or the developmental stage? (3) Do larvae and adults have different responses to T. parthenium and T. vulgare EOs?
Materials and methods
Collecting plant material and extraction of EOs
T. parthenium and T. vulgare plants in the full-blossom stage were collected from the location of Brezovica (42°13′19″N, 21°0′17″E; 400 km south of Belgrade, Serbia) and Aleksinac (43°33′0″N, 21°42′0″E; 206 km southeast of Belgrade, Serbia), respectively. Tanacetum species were identified according to Josifović et al. (Reference Josifović, Stjepanović, Kojić, Nikolić and Josifović1975). Above-ground (herbaceous) plant parts were air-dried at room temperature (22–25°C) for 7 days and used to obtain the EOs in a Clevenger-type apparatus (European Directorate for the Quality of Medicines, 2002). The extracted EOs were transferred into dark glass flasks, filled to the top and kept at 4°C until use. The extracts were dissolved in 96% ethanol to concentrations of 0.125, 0.25 and 0.5%.
Characterization of the EOs
Oil analysis was accomplished using gas chromatography (GC) and mass spectrometry (MS) analyses, as described by Block et al. (Reference Block, Flamini, Brkić, Morelli and Quetin-Leclercq2006). GC analyses were performed using an HP-5890 Series II gas chromatograph with a split/splitless injector, fused silica capillary column (25 m × 0.32 mm), coated with non-polar stationary phase HP-1 (cross-linked methyl silicone, 0.5 μl film thickness) and a flame ionization detector. GC/MS analyses were performed on a Hewlett-Packard 5890 gas chromatograph directly coupled to a Hewlett-Packard HP 5971 A (70 eV) mass selective detector. Component identification in the tested samples was carried out with GCD ChemStation Software G1701BA version B.00.00 (Agilent Technologies, www.chem.agilent.com) using the probability merge search engine along with a Wiley 275 L mass spectrum database library (www.onlinelibrary.wiley.com), by comparing the MS of constituents with those from the Agilent MS library.
Collecting and rearing insects
L. decemlineata adults were collected at the location of Dobanovci (25 km west of the centre of Belgrade) in potato fields not treated with pesticides. In the laboratory, adults were placed in glass cylinders in which potato was grown under conditions optimal for the development of L. decemlineata (T = 27 ± 1°C, RH = 60 ± 5%, neon diffuse light of intensity 30,159.29 cd under a 16:8 h light:dark regime). After eggs were laid on the potato leaf mass, the adult individuals were removed and egg hatching, larval moulting and eclosion of L. decemlineata adults were monitored. Identification of life stages was carried out according to Boiteau and Le Blanc (Reference Boiteau and Le Blanc1992). After the moulting, larvae were transferred to new cylinders. Bioassays were performed on third instar larvae (L3) 1 day after moulting and in adults 4 days after eclosion.
Antifeedant bioassay
Potato plants (cultivar Desiree) used in the bioassay were 6- to 7-week-old and 25–30 cm in height. The potato leaf mass was treated with ethanol solutions of EOs at three concentrations: 0.125, 0.25 and 0.5%; control potato plants were treated with 96% ethanol. Treatments were carried out by spraying with a TLC sprayer (Sigma-Aldrich). A total of 40 ml of solution per m2 was used for the potato treatments. After a 15-min air-drying of plants at room temperature, six third instar larvae or six adults (three females and three males) that had been starved for 24 h were placed on leaves, and the plants with insects were covered with glass cylinders and transferred to a microclimate chamber (Danfoss EKH 20 operational system; T = 27 ± 1°C, RH = 60 ± 5%, neon diffuse light of 30,159.29 cd intensity under a 16:8 h light:dark regime). After 48, 72 and 96 h of continuous exposure to treated leaves, leaf mass damage was estimated using a 0–10 scale (with 0 = 0% and 10 = 100% leaf mass damage). The bioassay was set up in five replicates per experimental group (one control and six groups treated with three concentrations of the two EOs). The antifeedant index (AFI) was calculated according to the formula:

where C is the consumption of control leaves and T is the consumption of treated leaves (López-Olguín et al., Reference López-Olguín, de la Torre, Ortego, Castañera and Rodríguez1999).
Data were tested for normality of distribution by the Shapiro–Wilk test and for homogeneity of variances by Levene's test. Since leaf damage did not have a normal distribution, data comparisons between experimental groups were carried out by Kruskal–Wallis analysis of variance (ANOVA). To reveal whether the treatment groups differed significantly from the control group, the Kruskal–Wallis test was followed by the Bonferroni correction (McDonald, Reference McDonald2014). To estimate the main and interaction effects of the examined factors, non-parametric two- and three-way ANOVAs were performed according to Brunner and Puri (Reference Brunner and Puri2001), followed by the Bonferroni test.
Avoidance of EO volatiles in non-contact and contact bioassays
A two-choice bioassay for evaluating the influence of EOs on host attraction was performed in an olfactometer. At the beginning of the bioassay L. decemlineata female adults were not in direct contact with treated leaf discs. The olfactometer was made of thick glass. The overall dimensions of the usable space in the olfactometer were 28 × 15 × 5 cm (length × width × height). The air flowed from two entrances, through the expanded part of the olfactometer (15 × 7 cm) and three tunnels (16.5 cm long and 3 cm wide), to two exits on the opposite side. The expanded part of the olfactometer prevents air turbulence. The olfactometer uses an air pump and rubber-coated tubes (9 mm in diameter) for the inlet air to the manifold, the air flow regulator, the rotameter and the glass air hub with activated charcoal, which served to neutralize the odouriferous substances in the air. Leaf discs of potato were cut with a cork-borer (20 mm in diameter) and immersed for 3 s in ethanolic solutions of EOs at concentrations of 0.125, 0.25 and 0.5%. Control leaf discs were immersed in 96% ethanol. After air drying for 15 min, control and treated leaf discs were placed in the right and left tunnels, respectively. For the control group, leaf discs treated with ethanol were placed in both tunnels. Third instar larvae 1 day after moulting and 4-day-old females starved for 24 h were used in the experiment. In each trial, one individual was put in the olfactometer so that the distance between the potato leaf discs and female adults was 21 cm, while third instar larvae were 2 cm away from the disc. Choice time was recorded as the time needed to ‘make the decision’ and to move towards the control disc.
In the contact bioassay, the treated discs were placed in Petri dishes (d = 9 cm), and then one third instar larva that was starved for 24 h was placed on each leaf disc. The retention of larvae on the treated or control discs was monitored for 5 min and expressed as escape time, i.e. the time in s before a larva left the disc. None of the control larvae left the leaf disc within the observation period.
Both bioassays were set at 10 individuals per experimental group. Log-transformed values of adult choice times and larval escape times had a normal distribution (Shapiro–Wilk test) and homogeneous variances (Levene's test) and thereby satisfied the assumptions for parametric analyses. These data were analysed by classic one- and two-way ANOVAs followed by a Bonferroni test. For comparison of the control with the treatment groups, the parametric Dunnett test was used.
Data on the larval choice time had nonhomogeneous variances and were analysed by Welch's ANOVA. Significant differences between the treatment groups and the control were revealed by the Games-Howell test followed by the Bonferroni correction (McDonald, Reference McDonald2014). Two-way and three-way non-parametric ANOVAs followed by the Bonferroni test (Brunner and Puri, Reference Brunner and Puri2001) were carried out to test the main and interaction effects of Tanacetum species, developmental stage and EO concentration.
Results
Composition of Tanacetum essential oils
The composition of the two Tanacetum oils is given in table 1. Thirteen compounds are present in both oils but in different concentrations. The major constituent of the T. parthenium oil was camphor while β-thujone was estimated at the highest concentration in T. vulgare. Comparison of constituents with concentrations greater than 1% in T. parthenium oil revealed that T. parthenium had a higher content of camphor, camphene, trans-chrysanthenyl acetate, bornyl acetate and α-pinene. The content of camphene, bornyl acetate and α-pinene in T. vulgare oil was below 0.5% but it contained β-thujone and 1,8-cineole that were not present in T. parthenium.
Table 1. Chemical composition of T. parthenium and T. vulgare EOs
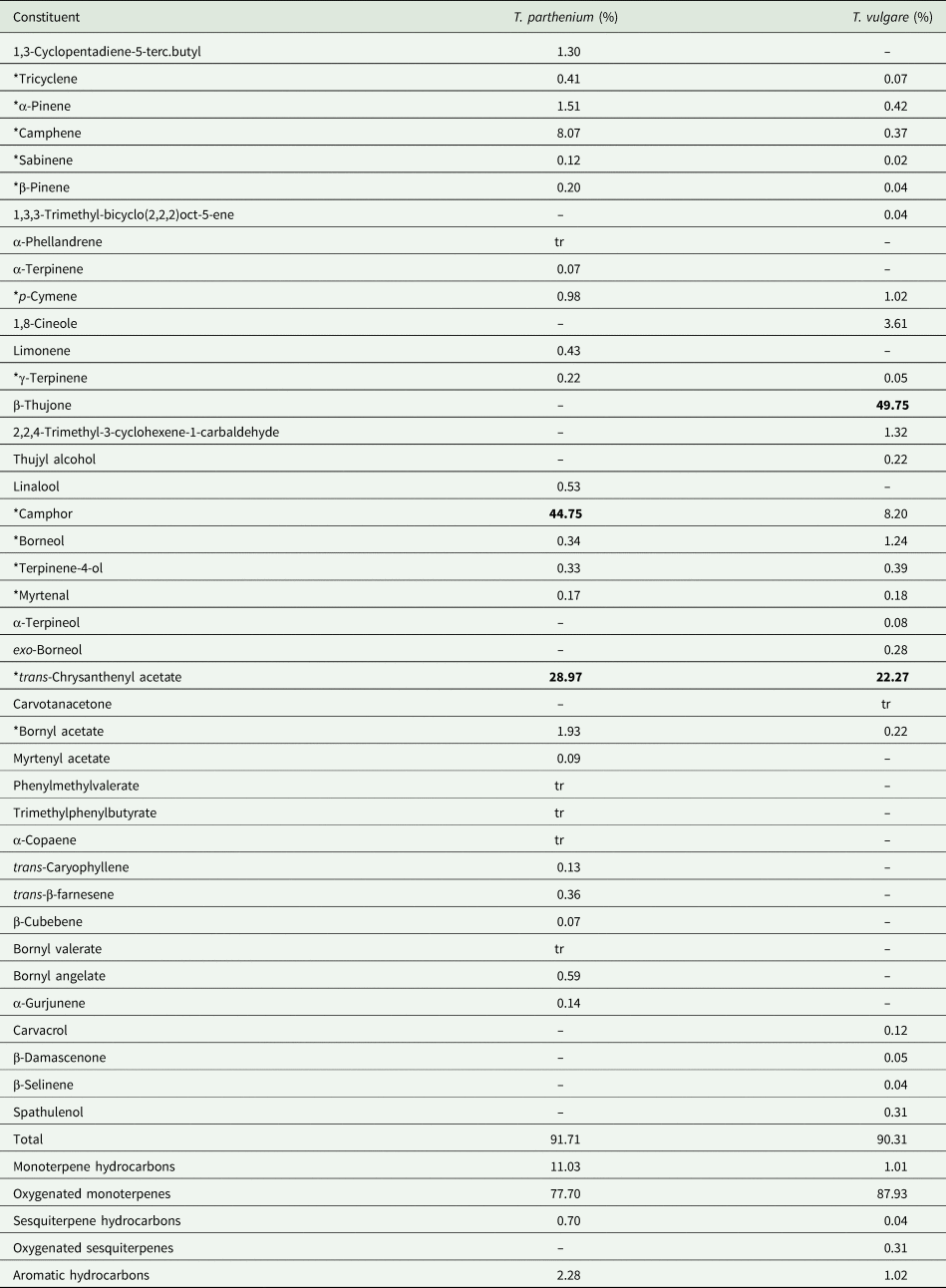
Major components (above 20%) are marked in bold. Components present in both oils are marked with an asterisk. Components present in traces are marked with tr.
Antifeeding effects of Tanacetum EOs
Defoliation was significantly decreased in EO-treated groups when leaf tissue was exposed to third instar larvae, indicating a phagodeterrent effect of the applied Tanacetum oils (fig. 1). Significant differences among the experimental groups at the end of the observation period (96 h) were revealed (H 6,35 = 23.79, P = 0.0006). After 96 h, both examined Tanacetum EOs provoked a significant reduction in leaf damage which was twofold or fourfold lower in the treatment groups than in the control group (fig. 1). Leaf damage strongly depended on Tanacetum species and on the concentration of the EO (table 2). On average, higher EO efficacy was recorded in T. parthenium than in T. vulgare (df = 24, t = 7.15, P < 0.0001), and at higher EO concentrations (0.125 vs. 0.25%: df = 24, t = 3.76, P = 0.0029; 0.125 vs. 0.5%: df = 24, t = 4.99, P = 0.0001).

Figure 1. The percentage of the damage of potato leaves treated with different concentrations of T. parthenium and T. vulgare EOs after 2, 3 and 4 days of consumption by L. decemlineata third instar larvae and adults. Significant differences (P < 0.05) of treatment groups from the control in the level of leaf damage after 4 days are marked with an asterisk.
Table 2. Two-way non-parametric ANOVA testing effects of Tanacetum EOs, oil concentrations and their interaction at 96 h of leaf damage made by third instar larvae and adults of L. decemlineata

Significant P values are presented in bold.
Our results also confirmed the phagodeterrent effects of Tanacetum oils on L. decemlineata adults. Similar to the results obtained in larvae, adult consumption differed significantly among the experimental groups after 96 h of exposure (H 6,35 = 22.11, P = 0.0012), and the phagodeterrent effects strongly depended on the Tanacetum species (df = 24, t = 6.82, P < 0.0001) and EO concentration (0.125 vs. 0.25%: df = 24, t = 5.59, P = 0.0029; 0.125 vs. 0.5%: df = 24, t = 7.84, P = 0.0001) (table 2). It is clear that Tanacetum oils effectively reduced leaf consumption by adults. The values of leaf damage were significantly lower than in the control group, except in the group treated with the lowest concentration of T. parthenium EO (fig. 1).
The results presented in table 3 clearly demonstrate that leaf damage and antifeedant effects of Tanacetum oils were stage-specific. On average, during 96 h the adults consumed more leaves than the third instar larvae (fig. 1; significant developmental stage effect in table 3). Also, the EOs exhibited different effects depending on the developmental stage (significant D × EO interaction effect). T. parthenium EO was more effective at reducing leaf damage by larvae, and T. vulgare EO was more effective in adults. The same pattern was also observed from the AFI (fig. 2). Another difference between developmental stages refers to the opposite trends of changes in the AFI with time. In larvae, antifeedant activity increased during the time of observation, while in adults it decreased.

Figure 2. AFI for L. decemlineata larvae and adults after 2, 3 and 4 days of consumption of potato leaves treated with T. parthenium and T. vulgare EOs at three different concentrations (0.125, 0.25 and 0.5%).
Table 3. Three-way non-parametric ANOVA testing effects of development stage, Tanacetum EOs, oil concentrations and their interaction at 96 h of leaf damage made by L. decemlineata

Significant P values are presented in bold.
Behavioural responses to Tanacetum EO volatiles in the olfactometer
Tanacetum EOs altered the responses of both developmental stages of L. decemlineata to host plant odour in the olfactometer in which contact cues with a stimulus were absent (table 4). The time needed to choose the control disc was prolonged when an alternative leaf disc was treated with the EO. On average, the two EOs did not differ in effectiveness (non-significant EO effect in table 5) while EO concentration significantly influenced movement of L. decemlineata larvae (0.125 vs. 0.5%: df = 24, t = 4.02, P = 0.0005) and adults (0.125 vs. 0.5%: df = 24, t = 3.41, P = 0.0037) (table 5). These patterns were similar in both the larvae and adults, i.e. the developmental stage (F 1,77.1 = 0.01, P = 0.9103) and D × EO effects were not significant (F 1,77.1 = 0.07, P = 0.7971). Larvae exposed to discs treated with the highest doses of both EOs, and adults exposed to the highest dose of T. vulgare EO moved more rapidly towards the control disc and were as fast as individuals that were presented with two control discs (table 4).
Table 4. Behavioural responses of L. decemlineata to T. parthenium and T. vulgare EOs in non-contact (choice time in s) and contact (escape time in s) bioassays

F, P values – results of Welch's one-way ANOVA for larval choice time and results of classic one-way ANOVA for adult choice time and larval escape time. Significant differences (P < 0.05) from the control group are marked in bold.
Table 5. Results of two-way ANOVA testing of the effects of Tanacetum oils, EO concentrations and their interaction on larval choice time (non-parametric ANOVA), adult choice time and larval escape time (classic ANOVA)

Significant effects are marked in bold.
Behavioural responses to Tanacetum EO volatiles after contact with a stimulus
After direct contact with Tanacetum EOs, L. decemlineata larvae rapidly moved away from the stimulus. On average, such a behavioural response was provoked more rapidly by T. parthenium than by T. vulgare EO (table 4; significant EO effect in table 5), and by higher than lower EO concentrations (0.125 vs. 0.5%: df = 24, t = 5.91, P < 0.0001; 0.25 vs. 0.5%: df = 24, t = 4.46, P = 0.0001) (table 4; significant concentration effect in table 5). The effect of the concentration of EO depended on the Tanacetum species (significant EO × C interaction in table 5). It can be seen in table 4 that a significant decrease in escape time with an increasing concentration of EO was observed only in T. parthenium oil (0.125 vs. 0.5: df = 54, t = 6.87, P < 0.0001).
Discussion
Our results showed that the two examined Tanacetum oils produced repellent effects on L. decemlineata. Treatment with the EOs reduced potato leaf damage, slowed down movement towards the untreated leaf disc in the olfactometer and increased movement away from the treated leaf disc. The following patterns were revealed: (1) The efficacy of Tanacetum EOs in modifying L. decemlineata behaviour was developmental-stage-specific and depended on Tanacetum species and EO concentration. (2) Sensitivity of leaf damage and choice time to increasing concentrations of EOs did not differ significantly between Tanacetum species and developmental stages. (3) T. parthenium and T. vulgare EOs were not equally effective at reducing leaf damage by larvae and adults.
Many studies have shown antifeeding and avoidance behaviours of L. decemlineata in response to different plant extracts and EOs (González-Coloma et al., Reference González-Coloma, Martin-Benito, Mohamed, Garcia-Vallejo and Soria2006; Pavela, Reference Pavela2010; Gökçe et al., Reference Gökçe, Isaacs and Whalon2012; Bekircan et al., Reference Bekircan, Cüce, Bekircan and Tosun2015). These types of behaviours are induced by compounds that hinder recognition of host-indicating odours and thus host acceptance for oviposition and feeding (Schröder and Hilker, Reference Schröder and Hilker2008). Panasiuk (Reference Panasiuk1984) recorded movement of L. decemlineata adults away from filter paper treated with a single concentration of thujone-rich T. vulgare EO, and also described adult behaviour in short-term choice tests as avoidance, i.e. beetles exclusively chose untreated leaves and fed on them. This is in accordance with our previous finding that L. decemlineata adults never choose leaves treated with thujone-rich T. vulgare EO (Kostić et al., Reference Kostić, Stanković, Ristić, Jevđović and Rajković2003). In contrast, a repellence index below 100% was recorded with EOs from bornyl acetate and camphor-umbellulone T. vulgare chemotypes (Schearer, Reference Schearer1984). Herein, we used choice time to quantify the repellent behaviour of L. decemlineata in an olfactometer choice test and found that both the larvae and adults respond to EO treatment by choice time prolongation in a dose-dependent manner.
When there was no choice, within the first minutes of the contact, L. decemlineata rapidly moved away from the treated leaves. However, after 2–4 days, potato leaves treated with Tanacetum oils were consumed although at a lower rate than the control potato leaves. Again, these behavioural responses depended on EO concentration. Similarly, a dose-dependent antifeedant activity in L. decemlineata was recorded for water and organic solvent extracts of T. vulgare (Hough-Goldstein, Reference Hough-Goldstein1990; Kutas and Nádasy, Reference Kutas and Nádasy2005) and other plant species (Gökçe et al., Reference Gökçe, Isaacs and Whalon2012; Alkan et al., Reference Alkan, Gökçe and Kara2015; Rusin et al., Reference Rusin, Gospodarek and Biniaś2016), and for the EOs of Satureja hortensis, Thymus transcaucasicus, T. pseudopulegioides and T. leucotrichus (Pavela et al., Reference Pavela, Sajfrtová, Sovová, Karban and Bárnet2009; Bekircan et al., Reference Bekircan, Cüce, Bekircan and Tosun2015). Antifeedant activity of EOs was achieved at lower doses when compared to water and methanolic extracts, which can be explained by differences in composition and the concentrations of active ingredients. Comparing our results on the AFI obtained after 3 days of L. decemlineata exposure to 0.5% concentration of Tanacetum EOs with the results of other authors revealed that Tanacetum EOs were stronger antifeedants than T. leucotrichus EO and the methanolic extract of Humulus lupulus (Gökçe et al., Reference Gökçe, Isaacs and Whalon2012; Bekircan et al., Reference Bekircan, Cüce, Bekircan and Tosun2015), while the hexane-ethylacetate-methanolic extracts of Achillea millefolium and H. lupulus induced similar antifeedant response (Alkan et al., Reference Alkan, Gökçe and Kara2015).
Our results obtained in behavioural assays, which include direct contact between L. decemlineata and EOs show that the repellent responses differed between T. vulgare and T. parthenium. Namely, the antifeedant bioassay revealed that the T. parthenium EO was more effective at reducing leaf damage by larvae, and that the T. vulgare EO was more effective in adults. Also, the T. parthenium EO produced more rapid movement away from the treated leaf disc than T. vulgare. Differences in oil composition may account for significant differences in efficacy between the two Tanacetum oils.
With regards to a major terpene, more than 30 different chemotypes can be distinguished in T. vulgare, while T. parthenium was less diverse (Kleine and Müller, Reference Kleine and Müller2011). β-Thujone-chrysanthenyl acetate chemotype of T. vulgare in the current paper, is similar to samples from Estonia (Raal et al., Reference Raal, Orav and Gretchushnikova2014), and the camphor-chrysanthenyl acetate T. parthenium chemotype corresponds to individuals collected in Belgium (De Pooter et al., Reference De Pooter, Vermeesch and Schamp1989) and the Netherlands (Hendriks et al., Reference Hendriks, Bos and Woerdenbag1996). Compounds such as camphor, β-thujone, camphene, bornyl acetate, α-pinene, 1,8-cineole, p-cymene that are known for repellent effects against L. decemlineata (Panasiuk, Reference Panasiuk1984; Schearer, Reference Schearer1984), are present at concentrations above 1% at least in one of the two examined Tanacetum EOs. Dominant components of the EOs belong to oxygenated monoterpenes, which are thought to contribute to antifeedant activity in insects (Koul, Reference Koul2004). Kostić et al. (Reference Kostić, Dražić, Popović, Stanković, Sivčev and Živanović2007) recorded antifeedant effects of the thujone-rich fraction of Salvia officinalis EO, and of camphor in larvae and adults of L. decemlineata. The higher percentage of oxygenated monoterpenes in the T. vulgare EO than in the T. parthenium EO possibly contributed to its higher efficacy in adults.
Interestingly, opposite results were obtained for larvae, so that the T. parthenium EO was more effective at reducing feeding than the EO of T. vulgare. It has been suggested by Hough-Goldstein (Reference Hough-Goldstein1990) that adults, as primary host finders, are more sensitive to deterrents than larvae. However, our results, as well as the results of other authors (Szczepanik et al., Reference Szczepanik, Dams and Wawrzeńczyk2005; Kutas et al., Reference Kutas, Nádasy, Gráf and Asbóth2009; Rusin et al., Reference Rusin, Gospodarek and Biniaś2016), revealed that larva-adult differences in repellent behaviour depended on the botanical type and concentration.
Chemical insecticides are effective in potato protection as they cause high mortality of L. decemlineata larvae and adults, and significantly reduce leaf damage in the field (Alyokhin et al., Reference Alyokhin, Dively, Patterson, Castaldo, Rogers, Mahoney and Wollam2007; Bassi et al., Reference Bassi, Rison and Wiles2009). However, eco-friendly botanical insecticides such as neem extracts, pyrethrin and stilbenes could be equally or more successful, inducing 2.5–8-fold lower leaf damage (Igrc Barčić et al., Reference Igrc Barčić, Bažok, Bezjak, Gotlin Čuljak and Barčić2006; Gabaston et al., Reference Gabaston, El Khawand, Waffo-Teguo, Decendit, Richard, Merillon and Pavela2018). Our laboratory assays showed that after 3 days of exposure to the highest applied concentrations of EOs, leaf damage was about fourfold lower in T. parthenium EO in larvae, and in T. vulgare EO in adults. The antifeedant effects of Tanacetum oils were apparently stronger than the effects obtained in laboratory assays after 3 days of exposure of L. decemlineata to sublethal concentrations of the chemical insecticides imidacloprid and cyromazine (Furlong and Groden, Reference Furlong and Groden2001). On the other hand, a 3-day assay with neem-based botanical insecticides showed that Tanacetum EOs were more effective for adults than for larvae (Zehnder and Warthen, Reference Zehnder and Warthen1988; Hiiesaar et al., Reference Hiiesaar, Švilponis, Metspalu, Jõgar, Mänd, Luik and Karise2009).
In conclusion, these results point to the potential for the development of a Tanacetum EO-based repellent that could be included in integrated pest management programmes against L. decemlineata. Such a repellent would not be toxic to humans since it is known that the acceptable daily intake of camphor and thujone is relatively high (Zuccarini, Reference Zuccarini2009; Lachenmeier and Uebelacker, Reference Lachenmeier and Uebelacker2010). However, before its commercial application, several issues should be addressed, such as risks for non-target organisms, provision of increased EO stability, water solubility and persistence in the environment, as well as efficacy against the target L. decemlineata under field conditions.
Acknowledgements
This work was supported by the Ministry of Education, Science and Technological Development of Republic of Serbia, Grant nos. III43010 and 451-03-68/2020-14/200007.