Introduction
Internally stratified fossils from the Lower Devonian and divergence time studies based on fungal fossils have demonstrated that lichenization is an ancient mode of fungal nutrition (Lücking et al. Reference Lücking, Huhndorf, Pfister, Plata and Lumbsch2009; Honegger et al. Reference Honegger, Edwards and Axe2013; Prieto & Wedin Reference Prieto and Wedin2013; Beimforde et al. Reference Beimforde, Feldberg, Nylinder, Rikkinen, Tuovila, Dörfelt, Grube, Jackson, Reitner and Seyfullah2014). However, fossil records of extant lichen species are scarce. Most important discoveries are from amber, including Anzia Stitzenb. from 35–40 Myr-old Baltic amber and Parmelia Ach. and Phyllopsora Müll. Arg. from 15–45 Myr-old Dominican amber, which indicate a minimum age estimate for the family Parmeliaceae Zenker of c. 40 Myr (Poinar et al. Reference Poinar, Peterson and Platt2000; Rikkinen & Poinar Reference Rikkinen and Poinar2002, Reference Rikkinen and Poinar2008). However, these fossils were probably formed well after the origin of their family since a fossil-calibrated phylogeny has estimated Parmeliaceae to have diversified much earlier, around the Cretaceous-Paleogene boundary 58–74 Myr ago (Amo de Paz et al. Reference Amo de Paz, Cubas, Divakar, Lumbsch and Crespo2011). Fossils of crustose lichens from the genera Calicium Pers. and Chaenotheca (Th. Fr.) Th. Fr. have also been found in 35–55 Myr-old Baltic amber (Rikkinen Reference Rikkinen2003) and a fossil-calibrated phylogeny that includes different alternative positions of this Calicium fossil suggests that Caliciaceae Chevall. diversified 103–156 Myr ago in the early Cretaceous (Prieto & Wedin Reference Prieto and Wedin2016). Finally, amber inclusions of a small number of beard lichens have been found representing either Oropogon Th. Fr. or some alectorioid genera (Rikkinen & Poinar Reference Rikkinen and Poinar2002, Reference Rikkinen and Poinar2008; Rikkinen Reference Rikkinen2003; Kaasalainen et al. Reference Kaasalainen, Heinrichs, Krings, Myllys, Grabenhorst, Rikkinen and Schmidt2015).
For the lichen family Lobariaceae Chevall., only one fossil impression has been found in a 12–24 Myr-old Miocene deposit from northern California (MacGinitie Reference MacGinitie1937; Peterson Reference Peterson2000). The impression shows a thallus with the lower side facing upwards (Fig. 1, bottom photograph). The overall appearance of this fossil reveals strong similarities to extant species of Lobaria (Schreb.) Hoffm., including traits such as a thallus with elongated lobes, reticulated ridges and small pits along the lobe margins that Peterson (Reference Peterson2000) interpreted as impressions of broken soralia. With regard to these morphological traits, Peterson (Reference Peterson2000) placed emphasis on the resemblance to L. pulmonaria (L.) Hoffm. as well as other species of Lobariaceae with reticulated ridges. In fact, the fossil impression resembles three living species of Lobaria. The reticulated ridges and soralia at the lobe margin of the fossil are typical for L. pulmonaria. However, L. anomala (Brodo & Ahti) T. Sprib. & McCune also forms reticulated ridges and soralia, and L. retigera (Bory) Trevis. forms clusters of slender isidia which are difficult to distinguish from soralia if poorly developed or damaged on the fossil impression. These three species all grow along the western Pacific coast
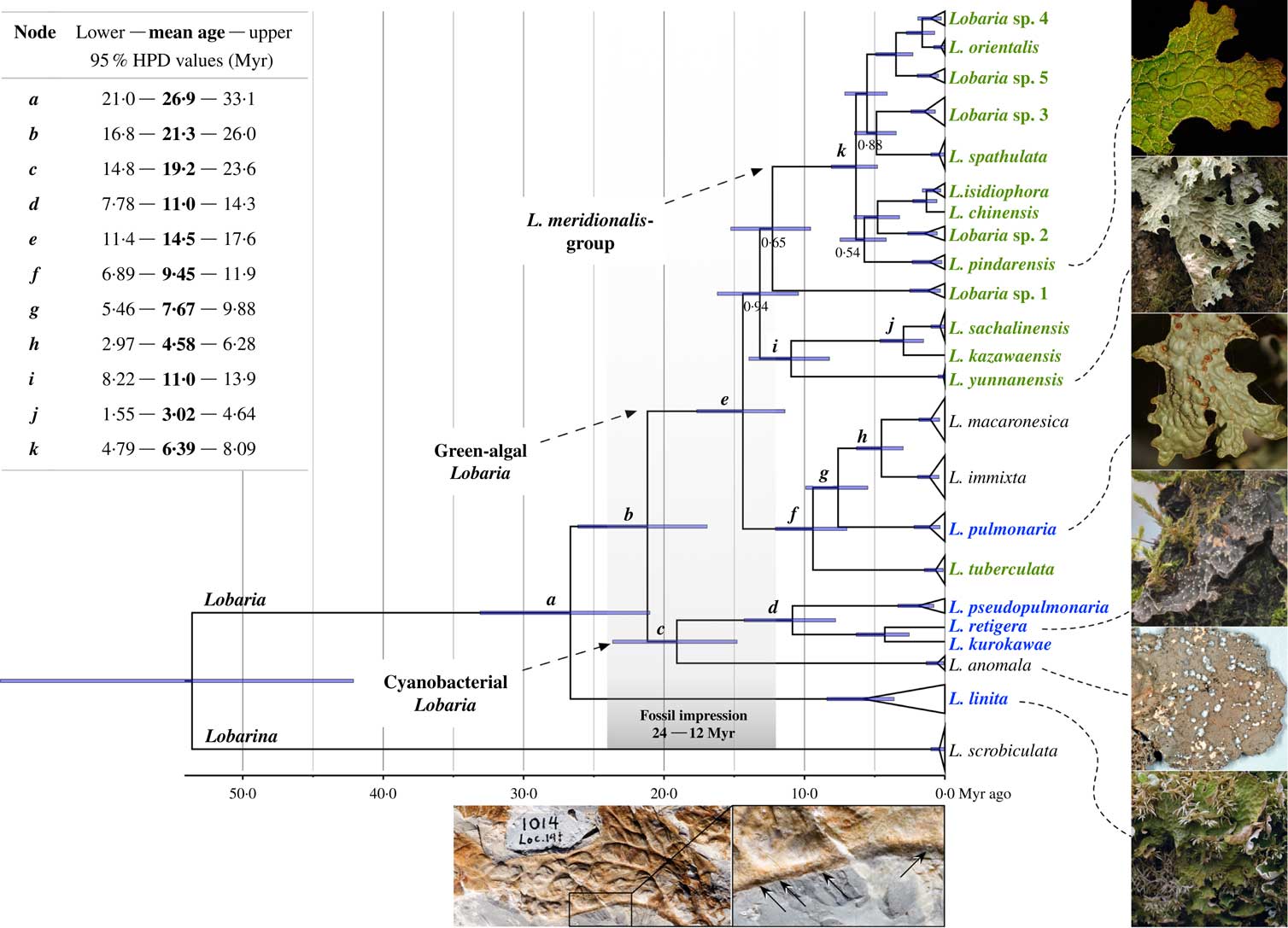
Fig. 1 Timetree of the genus Lobaria with images of modern Lobaria spp. (right) together with the fossil impression (bottom middle) for comparison. The timetree was obtained using Bayesian analysis with the mutation rate of nrITS as calibration reference. All branches obtained posterior probability values ≥0·98, except four with lower values indicated below the branches. Fossil impression age indicated by grey area. Mean ages of nodes a – k are given in the table (top left). Species in green are exclusively from East Asia, those in blue also grow in North America and other regions, leaving only L. anomala (West Pacific coast), L. immixta and L. macaronesica (both Macaronesian Islands in the North Atlantic) which grow outside of East Asia. Overview of the fossil impression UCMP No. 1014 (bottom middle left) and an enlargement of the area in the rectangle (bottom middle right). Arrows indicate small pits along the lobe margin (photograph courtesy of E. B. Peterson). Lobarina scrobiculata used as outgroup.
where the fossil impression was found. Furthermore, Cornejo & Scheidegger (Reference Cornejo and Scheidegger2015) have shown that L. anomala and L. retigera belong to a cyanobacterial lineage whereas L. pulmonaria belongs to a green-algal lineage, with both lineages originating from a common ancestral Lobaria species. Thus, another possibility is that the fossil impression represents an ancestral taxon.
Fossils are frequently ambiguous because convergent evolution among extinct and living lineages is common (Wang & Mao Reference Wang and Mao2016). Therefore, caution should be employed when integrating ambiguous fossils as minimum age constraints, or integrating unambiguous fossils as maximum age constraints into molecular dating. In the present case, the unambiguous phylogenetic assignment of this fossil is difficult. Therefore, the current study uses a molecular clock-calibrated phylogeny to obtain a time estimate for species of Lobaria that can be contextualized against the age of the fossil impression. Here we propose the first age estimate for the genus and discuss whether the fossil impression can be assigned to an extant or an ancestral Lobaria species.
Material and Methods
For the present investigation we used the alignment from a previous study on evolutionary relationships within Lobaria (Cornejo & Scheidegger Reference Cornejo and Scheidegger2015), which is available in TreeBase (http://treebase.org) under the study identification number S18155. Three molecular markers (EF-1α, nrITS, RPB2) and 50 specimens from all major Lobaria clades were assembled and four outgroup specimens of Lobarina scrobiculata (Scop.) Nyl. completed the dataset. All calculations were run in BEAST v1.8.4 (Drummond & Rambaut Reference Drummond and Rambaut2007; Drummond et al. Reference Drummond, Suchard, Xie and Rambaut2012). First, three analyses using an uncorrelated lognormal relaxed clock were performed (each with a constant speciation rate per lineage (Yule speciation) and 12 million generations) to have an indication of how clock-like the genes behaved over the phylogeny. With branch rate standard deviations (ucld.stdev) of 0·618 for EF-1α, 0·461 for nrITS and 0·715 for RPB2 (mean of three runs), data were assumed to be quite clock-like (BEAST 1.8 guide; Drummond et al. Reference Drummond, Ho, Rawlence and Rambaut2007). Thus, a strict clock prior was used for all analyses. This is the basic model in BEAST which assumes a global clock rate with no variation among lineages in a tree.
Regarding several age estimates for genera of the Parmeliaceae and Lecanoraceae, we used the mutation rate calculated for 1) nrITS (2·4×10−9 substitutions per site per year (s s−1 y−1); Table 1) and 2) RPB2 (1·5×10−9 s s−1 y−1; Table 1) as secondary calibration points for two separate age estimates, and 3) both mutation rates in a combined calibration approach. The clock model was unlinked and divergence times were estimated under a strict molecular clock, implementing a Yule tree prior which assumes a constant speciation rate per lineage. The data matrix was partitioned by individual gene regions with a linked substitution model (HKY+I+Γ) across loci. Tree parameters were linked across partitions and all other priors and operators were allowed to auto-optimize. The analysis was run with 70 million generations, sampling every 2000th generation, with a discarded burn-in of 10%. Convergence and the consequent proportion of burn-in were assessed using Tracer v1.6 (Rambaut et al. Reference Rambaut, Suchard, Xie and Drummond2014), considering effective sample size values >200 as good indicators. A maximum clade credibility tree was generated by analyzing the BEAST trees in TreeAnnotator v1.8.4. This program determined the 95% highest posterior densities (HPD) and estimated the mean heights. The best resulting tree was graphically represented in FigTree v1.3.1 (Rambaut Reference Rambaut2009) and edited using OmniGraffle graphic software v6.0.5 (OmniGroup, Seattle, USA).
Table 1 Publication list of lichen studies that calculated the mean mutation rates for loci that are relevant to the present study. Dashes indicate missing information

* Derived from the mutation rates of RPB1 and nrLSU for Melanelixia (Amo de Paz et al. Reference Amo de Paz, Cubas, Divakar, Lumbsch and Crespo2011).
** Derived from the mutation rates of RPB1 for Melanohalea, as well as nrLSU and mrSSU for Parmeliaceae (Amo de Paz et al. Reference Amo de Paz, Cubas, Divakar, Lumbsch and Crespo2011).
*** Derived from the mutation rate of nrITS for Melanohalea (Leavitt et al. 2012 Reference Leavitt, Esslinger, Divakar and Lumbschb ).
† Derived from the mutation rate of nrITS for Melanelixia (Leavitt et al. 2012 Reference Leavitt, Esslinger, Divakar and Lumbscha ).
‡ Derived from the mutation rate of nrITS for M. fuliginosa/M. glabratula group (Leavitt et al. 2012 Reference Leavitt, Esslinger, Divakar and Lumbscha ).
Results and Discussion
Molecular clock rate
All molecular clock analyses returned a topology that was fully consistent with the previous multilocus phylogeny of Lobaria (Cornejo & Scheidegger Reference Cornejo and Scheidegger2015). We first tested nrITS and RPB2 mutation rate priors separately and then in a combined approach. Both separately estimated posterior times differed substantially, placing the origin of Lobaria either at c. 27 Myr BP (nrITS; Fig. 1) or at c. 15 Myr BP (RPB2; see Supplementary Material Fig. S1A, available online). In the latter case, the fossil impression would just match the diversification of Lobaria, which is hypothetically possible. However, the parallel estimated mutation rates for both other loci were highly accelerated (EF-1α=1·92×10−9 s s−1 y−1; nrITS=4·44× 10−9 s s−1 y−1) compared with results for other lichen taxa (Table 1) and appeared less plausible for the slow-growing Lobaria species with rather long generation cycles (e.g. 10 years in L. pulmonaria; Scheidegger Reference Scheidegger1995).
From a statistical point of view, age estimates based on a single calibration are more prone to error than those in which multiple calibrations are applied. One approach to mitigate this error is to apply a combination of two secondary calibrations or, alternatively, of primary and secondary calibrations. Analysis 3, based on both mutation rates in a combined calculation, resulted in mean age estimates that are between both other analyses and placed the origin of Lobaria at 20·2 Myr (Supplementary Material Fig. S1B). However, it should be noted that dos Reis & Yang (Reference dos Reis and Yang2013) and Schenk (Reference Schenk2016) demonstrated that narrower minimum and maximum age estimates are accounted for by conflicting calibration references rather than more realistic divergence times. We thus assumed that the nrITS-calibrated analysis was more reliable than the RPB2 or combined estimate. The timetree obtained from analysis 1 is discussed below (Fig. 1), while the RPB2 and combined calibration trees may be consulted as online supplementary material (Fig. S1).
Along with the mutation rate for the nrITS (2·4×10−9 s s−1 y−1), we obtained rates for RPB2 (0·882×10−9 s s−1 y−1) and EF-1α (1·09×10−9 s s−1 y−1). Most bifurcations are maximally supported by posterior probabilities (PP)=0·98, except for four that were also poorly supported in the prior analysis (Cornejo & Scheidegger Reference Cornejo and Scheidegger2015) in which relationships between species of Lobaria were discussed. Consequently, these bifurcations are not considered for the age estimate. The following deals exclusively with the age estimate shown in Fig. 1.
Age estimates for Lobaria
The most recent common ancestor for species of Lobaria was dated to 26·9 Myr BP (node a ). Similar ages are known for some genera in the family Parmeliaceae, such as Flavoparmelia Hale and Xanthoparmelia (Vain.) Hale (Amo de Paz et al. Reference Amo de Paz, Cubas, Divakar, Lumbsch and Crespo2011), Montanelia Divakar et al. (Divakar et al. Reference Divakar, Del-Prado, Lumbsch, Wedin, Esslinger, Leavitt and Crespo2012) and Melanohalea O. Blanco et al. (Leavitt et al. 2012 Reference Leavitt, Esslinger, Divakar and Lumbscha ). Our analysis suggests that several major divergence events led to the evolution of extant taxa within Lobaria. In the early Miocene, an ancestral Lobaria split into two major lineages, one associated with cyanobacteria (Nostoc spp.) and the other comprising several lineages associated with green-algal strains of the genus Symbiochloris (Trebouxiaceae) (Škaloud et al. Reference Škaloud, Friedl, Hallmann, Beck and Dal Grande2016). Node c diversified 19·2 Myr BP early in the Miocene and contains four species in this analysis although, taking the missing species-pairs in our dataset into consideration, this clade contains six taxa. The basal radiation of the green-algal species was estimated to have taken place in the middle Miocene (node e , 14·5 Myr BP). The disjunct segregation of south- and north-eastern Asian lineages is noticeable in clade i . Lobaria yunnanensis Yoshim. is known from the mountainous Yunnan Province and Taiwan, while the species pair L. sachalinensis Asah. – L. kazawaensis (Asah.) Yoshim. grows in deciduous forests in north-eastern Asia. Node k , including species of the L. meridionalis-group, began diversifying in the late Miocene 6·39 Myr BP. This clade, which includes species exclusively from East Asia that are taxonomically still under dispute, will be discussed in detail elsewhere (C. Cornejo, S. Chabanenko & C. Scheidegger, unpublished data). Node f (9·45 Myr BP) includes the widely distributed L. pulmonaria, and L. tuberculata Yoshim. which is found in a restricted area of temperate and boreal forests in northern Japan, the Russian Primorsky Krai, and in the Sakhalin Oblast’ region. Also included are L. macaronesica Cornejo & Scheid. and L. immixta Vain. (node h , 4·58 Myr). These occur only in subtropical laurisilva habitats in the Macaronesian Islands.
These age estimates are somewhat limited because we used a secondary calibration reference derived from the family Parmeliaceae. In addition, we cannot be certain that there is a linear relationship between distance and time of Parmeliaceae and Lobariaceae because molecular clock rates can vary greatly among genes and organisms (Ayala Reference Ayala1997; Takahata Reference Takahata2007; Wilke et al. Reference Wilke, Schultheiß and Albrecht2009). More widely used approaches for estimating mutation rates involve fossil and/or biogeographical data. However, fossils can only provide minimum age estimates given that a fossil is necessarily younger than the phylogenetic event that led to its existence (Wilke et al. Reference Wilke, Schultheiß and Albrecht2009). In the present study, the Lobaria fossil cannot be assigned unambiguously to branches in a phylogeny because it is possible that the fossil is an extinct taxon.
Another issue is the under-sampling problem. In many cases we do not know whether a phylogeny is complete or not because fossil evidence of extinct lichen species is extremely rare. Furthermore, the interpretation of the phylogenetic relevance of divergence events may be affected by missing taxa and thus subject to misinterpretation. With regard to this question, Cummings & Meyer (Reference Cummings and Meyer2005) point out that sampling manifested through the processes of speciation will tend to lead to short branches and the processes of extinction will tend to lead to longer branches in a phylogeny. For example, the single branching of L. anomala in node c and the very old age estimate of c. 19 Myr for this species suggest an under-sampling problem in our dataset. It is conceivable that taxa became extinct between nodes c and d . If this were the case, node c would represent the maximum age of cyanobacterial Lobaria species. Missing taxa could not lead to a greater age calculation for cyanobacterial Lobaria, but could lead to a lower age for L. anomala. The situation is similar for L. yunnanensis and Lobaria sp. 1, whose long branches indicate missing taxa. Both species are from understudied regions in East Asia and, in this case, it is also possible that missing taxa are undescribed but presently living species.
Fossil impression: living or extant taxon?
The tree topology in Fig. 1 shows the placement of the fossil close to the crown of Lobaria. According to the estimated ages, it is possible that the fossil represents L. anomala, which occurs from northern California to southern Alaska and appears to be an old species. Another possibility is that it represents L. linita (Ach.) Rabenh. or an ancestor of the cyanobacterial species in node d (L. retigera, L. kurokawae and L. pseudopulmonaria). Even though their mean ages were estimated at 26·9 Myr (node a ) and 11·0 Myr (node d ), respectively, the minimum age of node a and maximum age of node d are both estimated at c. 15 Myr (see Fig. 1). In any case, our time estimate rules out L. pulmonaria and L. retigera which also occur along the western Pacific coast but with much younger age estimates. The exclusion of L. pulmonaria is somewhat surprising, since the fossil has an elongated lobe that is common in green-algal species (node e ) such as L. pindarensis or L. yunnanensis (Fig. 1, images on the right). However, the lack of soralia and the distribution range of most green-algal Lobaria species are arguments against extant green-algal species. Although the Bering land bridge connected Eurasia and North America resulting in one supercontinent and allowed the interchange of terrestrial biota throughout most of the Cenozoic Era, most green-algal species are currently found in restricted areas in East Asia (Yoshimura Reference Yoshimura1971). Lobaria linita has a circumpolar distribution but completely lacks soredia or isidia. Only L. pulmonaria has a worldwide distribution and produces soralia. All other green-algal species grow on tree bark in deciduous forests in restricted regions in South-East Asian mountains, temperate rainforests in northern East Asia, and the Himalayan subalpine forests. The first opening of the Bering Strait temporarily impeded land migrations towards the end of the Miocene 5·32 Myr ago (Marincovich & Gladenkov Reference Marincovich and Gladenkov1999; Gladenkov et al. Reference Gladenkov, Oleinik, Marincovich and Barinov2002), indicating that most green-algal species evolved in East Asia after the initial separation of the supercontinent.
Considering all these arguments, we reject the hypothesis that the fossil impression belongs to L. anomala because the elongated lobe of the fossil differs from this species. Second, L. pulmonaria and L. retigera are ruled out due to their age, and L. linita due to the lack of vegetative propagules. Consequently, we hypothesize that the fossil impression belongs to an ancestral Lobaria species which formed elongated lobes and may have been associated with a green-alga or a cyanobacterium as the primary symbiont (node b or e ). Although it is quite likely that the ancestral Lobaria produced soredia, we cannot reliably exclude the possibility that this fossilized individual produced clusters of isidia, whose marks on the stone are hardly distinguishable from those of soralia (node d ).
Biogeographical context of the fossil
The hypothesis proposed here implies that the ancestral Lobaria may have been able to migrate through the Bering land bridge from North America to East Asia, or vice versa. According to Wen et al. (Reference Wen, Nie and Ickert-Bond2016), beside the land bridge that joined both continents, floristic and faunal migration was primarily controlled by climate with 1) warm-temperate migration groups associated with the boreotropical-mixed mesophytic forests during the late Cretaceous to Paleogene (70–20 Myr BP), 2) boreal groups associated with taiga-coniferous forests in the Neogene (20–3 Myr BP), and 3) Arctic groups associated with tundra vegetation relatively recently during the Quaternary (3–0 Myr BP). There is considerable literature that demonstrates a close biogeographical connection between eastern Asia and western North America from the late Cretaceous to the late Neogene in major lineages of vascular plants (for a review, see Wen et al. Reference Wen, Zhang, Nie, Zhong and Sun2014, Reference Wen, Nie and Ickert-Bond2016). Here, we wish only to highlight the genus Picea A. Dietr. which contains important substratum species for Lobaria taxa. Extensive studies of this genus show a disjunction similar to that in Lobaria with the highest species diversity found in the western Cordillera of North America and the Qinghai-Tibetan Plateau (QTP). The diversification of the QTP-clade has been dated back to 19·8 Myr ago at the early Miocene. However, similar to the situation presented here, the ancestral area of the QTP-clade is still highly debated and may be interpreted as having a QTP or a North American origin (Ran et al. Reference Ran, Wei and Wang2006; Bouillé et al. Reference Bouillé, Senneville and Bousquet2011; Lockwood et al. Reference Lockwood, Aleksić, Zou, Wang, Liu and Renner2013).
Lobaria s. lat.
An issue that was not addressed in this study is whether species of closely related genera with similar characteristics might also fit the age of the fossil impression. The phylogeny presented in this paper follows the taxonomic revision by Moncada et al. (Reference Moncada, Lücking and Betancourt-Macuase2013) that circumscribed Lobaria in a strict sense, separating the genera Lobariella Yoshim., Lobarina Nyl. ex Cromb., Ricasolia De Not. and Yoshimuriella B. Moncada & Lücking from Lobaria. Within these genera, there are many species that remain phylogenetically unverified and, thus, nomenclatorially unrevised. In what follows, we treat them as Lobaria s. lat. For example, L. hallii (Tuck.) Zahlbr. and L. oregana (Tuck.) Müll. Arg. are both present along the Pacific coast of North America and both have reticulated ridges. Of these two species, only L. hallii produces abundant soredia along ridges and lobe margins (and may possibly be related to the fossil impression) while L. oregana has lobulated vegetative propagules. Further work needs to be done to formalize the taxonomic positions of L. hallii and L. oregana within the genus Lobarina and to estimate the divergence age for this genus. Furthermore, a time analysis including all lineages of the family Lobariaceae could shed light onto the area of origin of the different genera and migration pathways.
Conclusions
Using a secondary calibration reference from Parmeliaceae, we have obtained the first dated phylogeny of Lobaria and estimated the ages of divergence of the mostly well-resolved species. The timetree gave a diversification estimate for Lobaria in the late Oligocene. This timetree fits with the only known fossil from Lobariaceae, which is hypothesized here to be an ancestral Lobaria species. Analogous to East Asian-North American disjunct plants, the age estimates indicate that the paleoclimate and the closing or opening of the Bering Strait played a major role in shaping the current distribution of most Lobaria species. The ancestral Lobaria species must have successfully migrated along the Bering land bridge which acted as a major highway during warm-temperate climate periods but as a barrier during Arctic climate times. However, the time estimates presented here must be interpreted with caution owing to the lack of certainty regarding a linear relationship between mutation rates of Parmeliaceae and Lobariaceae. In addition, single long branches of some Lobaria species (L. anomala, L. yunnanensis and Lobaria sp. 1) suggest an under-sampling problem. While it can be assumed that some missing taxa are extinct, undescribed species from understudied regions are also conceivable.
The authors thank Toby Spribille and two anonymous reviewers for comments on the manuscript. Curtis Gautschi corrected the English text.
Supplementary Material
For supplementary material accompanying this paper visit: https://doi.org/10.1017/S0024282917000676