INTRODUCTION
It is often recognized that the consequence of applying certain fungicides to field-grown wheat is prolonged green leaf area duration (GLAD; Jones & Bryson Reference Jones and Bryson1998; Saunders & Salmon Reference Saunders and Salmon2000; Jones et al. Reference Jones, Shearman and Sylvester-Bradley2001), whether disease- or physiology-related. The application of fungicides could therefore be interpreted as resulting in the expansion of source relative to sink. However, the expansion of source does not always mean increased grain yield. Davies et al. (Reference Davies, Kettlewell, Green, Hocking and Jarai1984) observed a prolonged photosynthetic activity per unit area of the flag leaf at 40, 54 and 61 days after flag leaf emergence in response to propiconazole application at growth stage (GS) 39 (Zadoks et al. Reference Zadoks, Chang and Konzak1974) with no observed effect of fungicide application on either grain number or mean grain weight (MGW). They suspected that the delay in senescence was too late to contribute to the grain yield. However, in field experiments carried out in various sites, seasons and environments to investigate the effects of late-season applications of propiconazole and tridemorph on grain yield and bread-making quality, Gooding et al. (Reference Gooding, Smith, Davies and Kettlewell1994) observed a longer GLAD and an increased rate of grain filling following the application of propiconazole (125 g a.i.) plus tridemorph (250 g a.i.) at GS39 and GS59. Grain maturation was delayed by the fungicide application. When they considered all the field experiments conducted in their study, fungicide application increased grain yield by 7·6%, of which 5·5% was attributable to the increase in MGW.
Similar effects have been observed in crops treated with strobilurins. Gooding et al. (Reference Gooding, Dimmock, France and Jones2000) reported that azoxystrobin treated at or close to flag leaf emergence and ear emergence prolonged green flag leaf area duration (GFLAD) and increased mean grain mass. The degree of prolongation of GFLAD as well as that of the increase in mean grain mass was greater compared with the effects brought about by triazole and morpholines (Dimmock & Gooding Reference Dimmock and Gooding2002). Ruske et al. (Reference Ruske, Gooding and Jones2003a) tested the effects of adding either azoxystrobin or picoxystrobin to a triazole fungicide programme on five wheat cultivars (Hereward, Malacca, Charger, Consort and Shamrock) over 3 years. They observed, on average across the tested cultivars, an increase in grain yield of 0·5 t/ha (except for Hereward) and 0·9 t/ha for each week of delayed senescence in 2000 and 2001, respectively. Shamrock was more responsive than the other cultivars in 2002, showing a yield increase of 1·5 t/ha for each week of delayed senescence.
The effects of strobilurin fungicides on nitrogen (N) traits of wheat have also been documented. Gooding et al. (Reference Gooding, Gregory, Ford and Pepler2005) studied three wheat cultivars (Consort, Shamrock and Hereward) over 3 years and, following the application of a mixture of epoxiconazole and azoxystrobin at GS39 and GS59, consistently observed increased N in the above-ground biomass and net N remobilization from non-grain tissues and, consequently, improved N utilization efficiency compared with untreated plots. Ruske et al. (Reference Ruske, Gooding and Jones2003a) regarded improvements in N uptake from the soil, N harvest index (NHI), net remobilization of N from vegetative tissues after anthesis and an increase in the duration of N filling in the grain as a part of the main effects of strobilurin fungicides.
Although the main effects of strobilurin fungicides on dry matter (DM) and N aspects of winter wheat have been well reported (Gooding et al. Reference Gooding, Dimmock, France and Jones2000; Dimmock & Gooding Reference Dimmock and Gooding2002; Ruske et al. Reference Ruske, Gooding and Jones2003a), there have been few studies reporting on the interaction between strobilurin fungicides and different rates of N.
The objective of the present study was, therefore, to investigate the interactions between spray programmes containing strobilurin fungicide and fertilizer N with respect to yield and grain N.
MATERIALS AND METHODS
Field experiments
Four field experiments were conducted at Harper Adams University College: 1999/2000 (Expt I), 2001 (Expt II) and two in 2001/02 (Expts III-B and III-C). All the field experiments were designed as a factorial of two factors with either three (Expts I and II) or four replicates (Expts III-B and III-C). Experiments were randomized block designs with plot size of 1·75×10 m. Experimental plots were maintained according to standard farm husbandry practices, except for fungicide and N applications, which were the experimental treatments. Hereward, a bread-making cultivar, was used in each of the experiments. Seed rate was 350 seeds/m2 in all the field experiments.
Treatments
The fungicide programmes consisted of: epoxiconazole (Opus, BASF Ltd; 1 l/ha, 121 g a.i./kg product); epoxiconazole plus kresoxim-methyl (Landmark, BASF Ltd; 1 l/ha, 115 g a.i./kg product); and epoxiconazole plus trifloxystrobin (Twist, Novartis Crop Protection UK Ltd; 2 l/ha, 125 g a.i./kg product). The amount of epoxiconazole was the same for all the fungicide treatments. Fungicides were applied at GS31 and 39 (Zadoks et al. Reference Zadoks, Chang and Konzak1974). However, the fungicide programme of epoxiconazole plus kresoxim-methyl was not used in Expt III-B (Table 1). Fungicides were applied to each plot using a carbon dioxide powered hand-held knapsack sprayer. Fungicides were applied at 200 l/ha as a medium spray quality. Plant growth regulators, chlormequat chloride and trinexapac ethyl were applied to all the plots at GS30/31, mixed with fungicides where applicable. The rates of N fertilizer were determined in accordance with The Fertiliser Recommendations (RB209) (MAFF Reference Maff2000) for the individual experiments to follow commercial practice and were based on soil type, previous cropping and crop requirement. In addition, a variation from this was used to test the effects of N and the interaction with fungicide treatments. At GS30/31, the onset of stem elongation (Zadoks et al. Reference Zadoks, Chang and Konzak1974) and GS32, ammonium nitrate (0·345 N) was broadcast manually to each plot obtaining a uniform cover. The total amount of N corresponding to treatments for each experiment is presented in Table 1. Where N was applied to plots, it was split into two applications. The first application was of 40 kg/ha applied at GS 30/31 and the rest of the N at GS 32. The dates when each operation was conducted are presented in Table 2.
Table 1. Fungicide and N treatments used in each of the four experiments conducted on winter wheat cvar Hereward
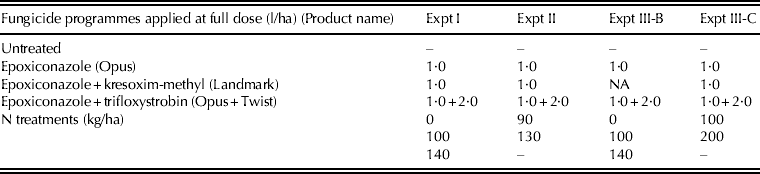
NA, not applicable.
Table 2. Dates of sowing, timing of N fertilizer and fungicide applications and harvest dates for four field experiments conducted on winter wheat cvar Hereward

Sampling and N analysis
At each sampling, plants were removed from an area of 0·525 m2 from each plot always excluding the border area except for Expt I, where 15 plants were randomly sampled from each plot. In Expt I, leaf area index (LAI) samples and pre-harvest samples were taken from a known area of 0·465 and 0·175 m2, respectively. Plant material was oven-dried at 80°C for 48 h. DM weight (DMW) was determined immediately after the removal of the samples from the oven. The dried plant material was then milled and passed through a 1 mm mesh using a Cyclotec 1092 sample mill (Tecator, Hillerød, Denmark). Total N concentration of plant samples was determined in duplicate by the Kjeldahl method (Bremner Reference Bremner and Black1965a) using a Kjeltec Auto 1030 and 1035 analyser (Tecator, Hillerød and Denmark) in 2000. For each sample, a portion of plant material was subjected to oven-drying at 100°C for 24 h and its moisture content determined. In 2001 and 2002, total N concentration of plant samples was determined in duplicate using an FP-528 (LECO, St. Joseph, MI, USA). Results of both methods were adjusted to 1000 g DM/kg.
LAI, GLAD and specific leaf area (SLA)
LAI is defined as the sum of the area of all leaves that are green per unit area of ground (Loomis & Connor Reference Loomis and Connor1992). For Expts I, II and III-B, leaf blades together with auricles were removed from the sample plants and leaf area was measured for a sub-sample using a Delta T Image Analysis System and WinDIAS (Delta-T Devices Ltd, Cambridge, UK). Fresh weight (FW), both of the total sample and the sub-sample, was measured and leaf area of the total sample was calculated from the ratio of the FW of the total sample and the sub-sample and the leaf area of the sub-sample. The leaf area of the total sample was divided by the area from which the plants were sampled and LAI was calculated.
In Expt III-B only, LAI measured at six samplings were linearly interpolated and the area under the LAI line between the two consecutive sampling dates was determined as the area of either a rectangle or a triangle. GLAD was calculated as the sum of rectangles/triangles. SLA was defined as the area per unit DMW of green leaf and was calculated by dividing LAI by DMW of leaf.
Combine-harvest
In each of the experiments, ears were removed from the whole plot and threshed at the same time using either a ‘Haldrup’ (2000) or ‘Wintersteiger’ (2001, 2002) plot combine. Harvested grain weight was measured for each plot and yield was adjusted to 850 g DM/kg FW. A sample of 1 kg was retained from the combine-harvested grains of each plot for the determination of moisture content and MGW.
Soil sampling and soil mineral N
Soil samples of 0·90 m depth were taken using a set of semi-cylindrical gouge augers from each plot in Expts I and III-B. Samples were taken at three depths, 0–0·30, 0·30–0·60 and 0·60–0·90 m, from four positions in each plot. The four soil samples from each depth were bulked together, sealed in a plastic bag and kept in a freezer at −18°C. Soil samples were passed through a 5·6 mm sieve prior to analysis. Approximately 40 g of each soil sample was placed into a bottle together with 200 ml of 2m potassium chloride solution. Each bottle was tightly sealed and shaken for 2 h on a shaker set at 265 rpm. The extracted solution was filtered through a Whatman No. 40 filter paper. The filtrate was then stored in a fridge at 4°C until analysed. Total soil mineral N (NH4++NO3−) was determined by steam distillation of the filtered extract with magnesium oxide and Devardas alloy (Bremner Reference Bremner and Black1965b).
Statistical analysis
Results were analysed using the GENSTAT (5th and 6th Editions) and SPSS 13.0 (SPSS Japan, Tokyo, Japan) statistical programs. Analysis of variance and regression analysis were performed (P<0·05).
RESULTS
Foliar diseases
Septoria tritici Roberge was the dominant disease throughout the four field experiments, although Stagonospora nodorum (Berk.) may have been present at trace levels. The only other foliar disease observed in any of the experiments was powdery mildew (Erysiphe graminis DC. f.sp. tritici). The most powdery mildew seen on any individual leaf did not exceed 0·04 and for most assessments it was absent altogether. The severity of S. tritici on leaf 1 at the later phase of grain fill was 0·015–0·11, except for Expt III-C where levels were as high as 0·38 on untreated plots.
Leaf area assessment (LAI, GLAD and SLA of leaves below the flag leaf)
No significant interactions between fungicide programmes and N rates were observed for LAI, GLAD or SLA of leaves below the flag leaf in any of the experiments. No significant treatment effects were seen in Expt II (data not shown).
N had a consistent and almost always a significant effect on leaf area in Expts I and III-B. Values for LAI, GLAD and SLA all increased with an increasing amount of N applied (Table 3).
Table 3. LAI of leaves below flag leaf estimated at GS59 in Expt I and LAI of total leaf after anthesis, GLAD estimated from GS32 to maturity and SLA in Expt III-B for the winter wheat cvar Hereward

F, fungicide programmes; N, N rates; LBF, leaves below flag leaf; TL, total leaf; LGL, lower green leaf; LAI, leaf area index; GLAD, green leaf area duration; SLA, specific leaf area; GS, growth stage.
Fungicide had a significant effect on LAI, GLAD and SLA. LAI and GLAD tended to be greater for the plots treated with a mixture of fungicides, especially the mixture of epoxiconazole and trifloxystrobin. The largest difference in LAI between fungicide programmes was at the second assessment date where epoxiconazole resulted in an LAI of 1·75 while the mixture of epoxiconazole and trifloxystrobin produced an LAI of 2·38. The mixture of epoxiconazole and trifloxystrobin increased GLAD over epoxiconazole alone by 32 days, to 320 (Table 3). GLAD accounted for 0·87 (P<0·001) of the variability in above-ground DMW (ADMW) and 0·88 (P<0·001) of the variability in yield (data not shown). SLA was greater for untreated plots than the plots treated with fungicides before anthesis. After anthesis, however, SLA was greater for the plots treated with fungicides, especially the mixture of epoxiconazole and trifloxystrobin when compared with untreated plots and those treated with epoxiconazole alone (Table 3).
Above-ground DM and grain yield
Interactions between effects of fungicide programmes and N rates on DMW were only observed in Expts II and III-B. In Expt II, DMW of both above-ground and lower leaf increased as N increased from 90 to 130 kg/ha for all treatments except for epoxiconazole, where both above-ground and lower leaf dry weight decreased with increased N (Table 4). A similar interaction was observed with DMW of spike (P=0·041) (data not shown). In Expt III-B, above-ground DM increased as N increased from 0 to 100 kg/ha and then 140 kg/ha for both fungicide programmes and the untreated plot. However, the proportionate increase in above-ground DM was greater for the fungicide programme treatments than the untreated.
Table 4. DMW and grain yield (GY) for winter wheat cvar Hereward in experiments ExptsII and III-B where there were significant interactions between fungicide and N treatments

F, Fungicide programmes; N, N rates; DAS, days after sowing; GY, grain yield; DM, dry matter.
There was an interaction between fungicide and N for yield of combine-harvested grains at harvest in Expt III-B. There was no difference in yield between fungicide programmes and the untreated plots in the absence of N fertilization. However, at 100 kg/ha and then 140 kg/ha, fungicide-treated plots gave progressively more yield over the untreated. At 140 kg/ha, untreated plots yielded 8·49 t/ha while epoxiconazole alone and those treated with a mixture of epoxiconazole and trifloxystrobin yielded 9·72 and 10·04 t/ha, respectively.
For a few sampling occasions in both Expts I and III-B, DMW of green leaf was found to be greater for the plots treated with fungicides, especially those treated with a mixture of epoxiconazole and trifloxystrobin (Table 5). In Expt III-B, DMW of senesced leaf was smaller for the plots treated with a mixture of epoxiconazole and trifloxystrobin than untreated plots and those treated with epoxiconazole alone.
Table 5. DMW of different leaf layers for winter wheat cvar Hereward in experiments Expts I and III-B where there were no significant interactions between fungicide and N treatments

LBF, leaves below flag leaf; LGL, lower green leaf; LSL, lower senesced leaf; DAS, days after sowing.
No significant interactions occurred between fungicide programmes and N application for pre-harvest DM assessments (ADMW), grain yield or HI. No difference in pre-harvest samples for ADMW was observed between fungicide programmes in Expts I, II or III-B. In Expt III-C, untreated plots showed a smaller ADMW before harvest to those treated with fungicides (Table 6). No difference in ADMW was observed at other samplings in any of the field experiments (data not shown).
Table 6. ADMW, MGW and HI at pre-harvest and GY (@850 g DM/kg) at harvest winter wheat cvar Hereward in experiments Expts I, II, III-B and III-C where there were no interactions between fungicide and N treatments

* Manually harvested grains at pre-harvest.
F, fungicide programmes; N, N rates.
No significant difference in grain yield was observed between fungicide programmes in Expt I or Expt II (Table 6). In Expt III-C, untreated plots showed a lower yield than those treated with fungicides (P=0·001). There was no difference in HI between fungicide programmes in Expts I, II or III-B (data not shown). In Expt III-C, untreated plots showed a smaller HI than those treated with fungicides (P=0·003) (Table 6).
No significant interactions occurred between fungicide programmes and N application for ear number per area (ENA), grain number per area (GNA) or MGW in any of the field experiments (data not shown). In addition, no difference in ENA and GNA between fungicide programmes was observed in any of the experiments (data not shown). In Expts I, II and III-C, MGW tended to be greater for the plots treated with fungicides than untreated plots (Table 6).
Leaf N yield
No significant differences in leaf N yield were seen in Expts II or III-C (data not shown). No significant interaction between N and fungicide treatment was observed in Expts I or III-B. Greater leaf N was observed in the plots where a greater rate of N had been applied (data not shown). In these two experiments, however, a greater N content of green leaf as well as smaller N content of senesced leaf were often observed during grain fill in the plots treated with fungicides, especially those treated with a mixture of epoxiconazole and trifloxystrobin (Table 7).
Table 7. Leaf N yield for different leaf layers of winter wheat cvar Hereward in experiments Expts I and III-B where there were no interactions between fungicide and N treatments

GL, green leaf; SL, senesced leaf; TL, total leaf; LGL, lower green leaf; DAS, days after sowing.
Above-ground N yield, grain N yield and NHI
There were significant interactions between fungicide programmes and N rates for above-ground N yield and grain N yield in Expt III-B (Table 8). The interactions between N and fungicide treatment appear to be caused by the greater response to increased N application from the mixture of epoxiconazole and trifloxystrobin. The plots treated with epoxiconazole alone showed a greater above-ground N yield than untreated plots at 100 kg/ha of N, while above-ground N yield was smaller for this fungicide programme when no N was applied (P=0·007). When trifloxystrobin was applied in mixture with epoxiconazole, a smaller and a similar above-ground N yield compared with untreated plots was observed at 0 and 100 kg/ha of N, respectively, at 295–302 days after sowing. When N was applied at 140 kg/ha, however, plots treated with this strobilurin fungicide programme showed a greater above-ground N yield than untreated plots and those treated with epoxiconazole alone (P=0·007). At this sampling time, a greater response in grain N yield with increasing N application was also observed from the application of a mixture of epoxiconazole and trifloxystrobin (P=0·038). At harvest, the plots treated with epoxiconazole alone and a mixture of epoxiconazole and trifloxystrobin showed a greater grain N yield than untreated plots, by 18 and 29 kg/ha, respectively, at 140 kg N/ha, while there was no difference in grain N yield between fungicide programmes when no N was applied (P=0·040). In addition, the plots treated with a mixture of epoxiconazole and trifloxystrobin showed a greater grain N yield than untreated plots at 100 kg/ha.
Table 8. Above-ground N yield (ANY) and grain N yield (GNY) for winter wheat cvar Hereward in Expt III-B where there were significant interactions between fungicide and N treatments
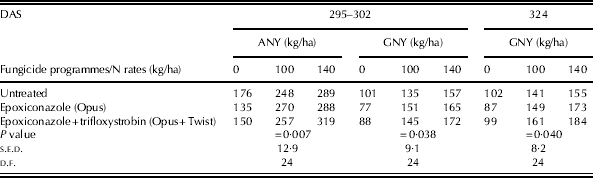
ANY, above-ground N yield; GNY, grain N yield; DAS, days after sowing.
There were no significant interactions between fungicide and N application in Expts I, II, III-B or III-C for above-ground N yield, Expts I, II or III-C for grain N yield or Expts II, III-B or III-C for NHI. Above-ground N yield, grain N yield and NHI are presented in Table 9.
Table 9. ANY and NHI at pre-harvest and GNY at harvest for winter wheat cvar Hereward in experiments Expts I, II, III-B and III-C where there were no interactions between fungicide and N treatments

* Manually harvested grains at pre-harvest.
F, fungicide programmes; N, N rates.
Above-ground N yield was increased significantly by increased N application in Expts I, III-B and III-C, whereas there was no significant effect of N in Expt II. Fungicide treatment, however, had no significant effect on above-ground N yield in any experiment. The greater the amount of N applied, the greater the above-ground N yield in Expts I, II and III-B. In Expt I, the plots treated with 140 kg N/ha appeared to have continued to take up N during the later phase of grain fill, while those treated with the lower amounts of N stopped taking up N (data not shown).
Grain N yield was significantly increased by both increased N application and fungicide treatment in each of the experiments with the exception of fungicide in Expt II. In Expt I, the plots treated with a mixture of epoxiconazole and trifloxystrobin showed a greater grain N yield than untreated plots, those treated with epoxiconazole alone and those treated with a mixture of epoxiconazole and kresoxim-methyl. In Expt III-C, untreated plots showed a lower grain N yield than those treated with fungicide programmes.
There was a significant effect of N on NHI in Expt III-C where NHI was reduced at the higher rate (200 kg/ha) of N. There was no effect of N on NHI in the other experiments. Fungicide treatment did have a significant effect on NHI in Expts III-B and III-C only. In Expt III-B, plots without fungicide showed a smaller NHI than the plots treated with epoxiconazole alone or those treated with a mixture of epoxiconazole and trifloxystrobin (P<0·001). A similar result was seen in Expt III-C, where untreated plots showed the lowest NHI compared with the plots treated with fungicides (P<0·001).
In Expt II, there was no difference in the ratio of NHI to HI between fungicide programmes (data not shown). In Expt III-B, untreated plots showed a smaller ratio of NHI to HI than those treated with epoxiconazole alone and those treated with a mixture of epoxiconazole and trifloxystrobin (P<0·001) (Table 10). In Expt III-C, untreated plots showed a smaller ratio of NHI to HI compared with the plots treated with fungicides (P<0·001) (Table 10).
Table 10. The ratios of NHI to HI in experiments Expts III-B and III-C and ANC at pre-harvest and grain N concentration at harvest in Expt I for winter wheat cvar Hereward
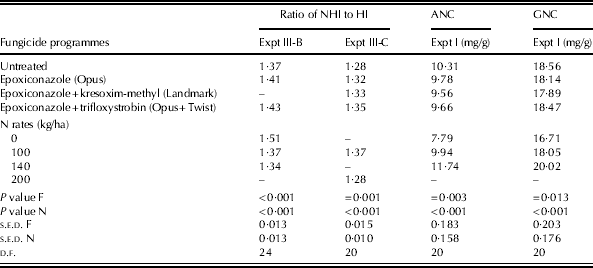
F, fungicide programmes; N, N rates; NHI, N harvest index; HI, harvest index; ANC, above-ground N concentration; GNC, grain N concentration.
Above-ground and Grain N concentration
In Expts II, III-B and III-C, there were no differences in above-ground N concentration at pre-harvest between fungicide programmes or grain N concentration (data not shown). In Expt I, there were no interactions between N and fungicides for above-ground N concentration or grain N concentration. Plots treated with fungicides, however, showed a smaller above-ground N concentration than untreated plots (Table 10), whereas untreated plots showed a greater grain N concentration compared with those treated with epoxiconazole alone and those treated with a mixture of epoxiconazole and kresoxim-methyl by 0·5 and 0·7 mg/g, respectively. The plots treated with a mixture of epoxiconazole and trifloxystrobin showed a greater grain N concentration than those treated with a mixture of epoxiconazole and kresoxim-methyl, by 0·6 mg/g (Table 10).
Soil mineral N
Soil mineral N at a depth of 0·9 m after harvest in Expt I is presented in Table 11. Soil mineral N was found to be higher after harvest than in spring for most of the plots (data not shown). No interaction was observed between fungicide programmes and N rates for soil mineral N. The plots treated with a mixture of epoxiconazole and trifloxystrobin showed a lower soil mineral N after harvest than untreated plots and those treated with epoxiconazole alone by 13 and 9 kg/ha, respectively (P=0·011). Soil mineral N after harvest was greater for untreated plots than the plots treated with epoxiconazole and kresoxim-methyl by 7 kg/ha (P=0·011). No significant differences in soil mineral N were observed in Expt III-B (data not presented).
Table 11. Soil mineral N at 0·9 m post-harvest in Expt I

F, fungicide programmes; N, N rates.
DISCUSSION
The objective of the present study was to investigate the interactions between N and strobilurin-fungicide-containing spray programmes with respect to yield and grain N. Overall, there was no consistent interaction between N and fungicide spray programme across the study, although some interactions were observed in individual experiments. As would be expected, the accumulation of DM and N increased with increased rates of N application. Fungicide programmes also increased grain yield and grain N but the epoxiconazole plus trifloxystrobin treatment appeared to have a greater effect than the other fungicide treatments: this was most pronounced at high N rates. This response to the epoxiconazole plus trifloxystrobin treatment at high rates of N appears to explain the significant interactions that occurred between N and fungicide.
Above-ground DM
Interactions between fungicide programmes and N rates were observed in two of the four experiments for above-ground DM accumulation. In Expt II, plots treated with a mixture of epoxiconazole and kresoxim-methyl showed an increase in ADMW, observed immediately after anthesis, to the highest rate of N (130 kg/ha) compared with that of 90 kg/ha. No similar effect was observed in plots treated with other fungicide programmes. There was, however, no evidence that the observed effect from this particular fungicide programme was beneficial to final grain yield, as no interaction in DM accumulation was observed between fungicide programmes and N rates at pre-harvest. Results were slightly different in Expt III-B, where an interaction was observed with respect to DM accumulation at a more advanced stage of grain fill, i.e. at c. 6 weeks after anthesis. A greater increase in ADMW was observed with the highest N application of 140 kg/ha from plots treated with a mixture of epoxiconazole and trifloxystrobin. The implication is that the application of this particular fungicide programme might have improved the way the crop utilized the N in carbon assimilation. Another interesting point to make here is that ADMW and grain yield were smaller on the plots treated with fungicides, than untreated plots where no N was applied, suggesting that the benefit of applying fungicides could only be exploited in the presence of N fertilization.
The effects of the fungicide programme containing a combination of triazole and strobilurin on ADMW at the highest N rate of 140 kg/ha observed in Expt III-B, however, did not seem to affect grain yield as no difference in yield was seen in plots treated with 100 kg/ha of N. If the increased ADMW of the plots treated with the combination of triazole and strobilurin was not fully utilized in forming the yield, there might have been a possibility of sink limitation as suspected by Ruske et al. (Reference Ruske, Gooding and Jones2003a) with cvar Hereward, the same cultivar used in the present study. The low responsiveness of Hereward to strobilurin fungicides was also observed by Bayles (Reference Bayles1999) in her study of the effects of strobilurin fungicides on the grain yields of 10 winter wheat cultivars. It should be noted that the plots treated with triazole alone showed a significant increase in yield in response to the N rate of 140 kg/ha compared with that of 100 kg/ha, although such an effect was not observed with ADMW or HI at pre-harvest. This might support the previous suggestion that the benefit of applying fungicides could be exploited better with N fertilization and may be enhanced at higher rates of N fertilization whether the fungicide is a strobilurin or not.
Above-ground N
There was no evidence in any of the experiments, from above-ground N yield measurements at pre-harvest, of an interaction between fungicide programmes and N rates with respect to above-ground N uptake. Grain N yield, however, measured at harvest in Expt III-B, indicated a significantly greater N accumulation in grains at the higher N rate (140 kg/ha) when plots were treated with fungicides, both triazole alone and a combination of triazole and strobilurin. Such an increase in grain N yield with increased rate of N was, however, absent for untreated plots. It is natural to suspect a possible interaction between fungicide programmes and N rates on NHI, considering that only grain N yield was increased as the consequence of the interaction between fungicide programmes and N rates, while above-ground N yield was not. However, this was not the case in Expt III-B.
In Expt III-B, complex interactions were found between fungicide programmes and N rates with respect to the traits measured in the present study related to DM accumulation and N accumulation, although the general trend was that positive effects resulted from a higher rate of N where strobilurin-based fungicides were applied. There were interactions observed even in Expt II, where the magnitude of the differences between fungicide programmes was small. It can be speculated that physiological effects of fungicides may have been present in the present study even though they were not always statistically significant, and that the performance of crops with respect to grain yield and grain N is most likely to be affected by fungicides where N fertilization is not lacking.
Fungicides affected grain yield, MGW and grain N yield in some of the experiments; however, above-ground DM accumulation and above-ground N yield were hardly affected by fungicides in any experiment. Inhibited translocation of N by foliar diseases such as S. tritici, documented by other workers (Leitch & Jenkins Reference Leitch and Jenkins1995; Bancal et al. Reference Bancal, Robert and Ney2007), has been recognized in the present study as a reduced NHI of untreated plots in comparison with the plots treated with fungicides. A reduction in HI was not evident, except in Expt III-C where a particularly severe level of S. tritici was observed. This observation is supported by the findings of Serrago et al. (Reference Serrago, Carretero, Bancal and Miralles2009) who showed that yield in cereal grains was reduced by diseases mainly through reductions in above-ground biomass but not HI. The reduced ratio of NHI to HI for untreated plots in Expts III-B and III-C is additional support for this point.
Mechanism for increased grain N
In the present study, no interaction was observed between fungicide programmes and N rates with respect to LAI and GLAD. These are the traits related to the size and the persistence of green leaf. Therefore, the different fungicides did not appear to affect the way the crops utilized the applied N, in forming and maintaining green leaf area, as the rate of N increased. Effects of fungicide programmes alone on LAI and GLAD were recognized in some of the experiments where the performance of plots treated with a mixture of epoxiconazole and trifloxystrobin was good, in particular at the later phase of grain fill. The greater N contained in green leaves and less N in senesced leaves following treatment with epoxiconazole and trifloxystrobin indicates a better optimization of N concentration throughout the whole canopy.
Delayed senescence of the flag leaf following application of strobilurin fungicides has been reported by Gooding et al. (Reference Gooding, Dimmock, France and Jones2000) and Ruske et al. (Reference Ruske, Gooding and Jones2003a, Reference Ruske, Gooding and Jonesb). The present study, however, showed that the LAI of leaves beneath the flag leaf was also maintained for a longer period following the application of strobilurin fungicides, and this effect was most notable for trifloxystrobin. SLA was found to be greater for the plots treated with the combination of triazole and strobilurin, which may indicate slightly more efficient utilization of DM assimilates and account for the maintenance of lower green leaf area. Unfortunately, the observations of SLA are from a single-year experiment and only allow for speculation. However, microscopic assessment of leaf structure may be useful, as Benton & Cobb (Reference Benton and Cobb1995) observed a morphological change in cleavers (Galium aparine L.) following the application of epoxiconazole and there was some evidence from the present study of transverse thickening of the lamella, from the epoxiconazole and trifloxystrobin treatment, under the light microscope (data not shown). The growth regulatory activity by triazole compounds was noted on oilseed rape by Berry & Spink (Reference Berry and Spink2009), who reported that rooting of this crop was improved following the application of metconazole, one of the triazole-type compounds that are used in Europe for both their fungicidal and growth regulatory properties. The increased rooting was estimated to contributed to a yield increase by 0·2–0·3 t/ha in a dry season.
It is known that most assimilates that contribute to grain yield are derived from carbon assimilation in the flag leaf during grain fill (Evans et al. Reference Evans, Wardlaw, Fischer and Evans1975) and that lower leaves will only have a small effect on the total amount of assimilates produced. Conversely, however, although the importance of post-anthesis N uptake from the soil has been highlighted and discussed by Bancal et al. (Reference Bancal, Roche and Bancal2008), most grain N usually comes from the remobilization and translocation of N from the stem and leaves previously taken up during the vegetative phase of growth (Kettlewell Reference Kettlewell, Henry and Kettlewell1996). The maintenance of lower leaf layers might therefore be advantageous if, for example, it contributes to extra uptake of translocation of N during this time. The advantage of delaying leaf senescence of the lower part of the canopy was shown by Ottman & Welch (Reference Ottman and Welch1988) with respect to DM and N accumulation in maize. As lower leaf layers do not contribute significantly to carbon assimilation, a net accumulation of N in the grain following translocation of N from lower leaves could occur, or at least compensate for any grain dilution effect caused by extended GLAD of the flag leaf. Of course, the lower leaves would need to senesce before the end of grain fill for remobilization and translocation of N to be possible. Monaghan et al. (Reference Monaghan, Snape, Chojecki and Kettlewell2001) used grain protein deviation (GPD) to measure the ability of a cultivar to achieve a higher grain protein than that expected from its grain yield. They noted the importance of both post-anthesis uptake and remobilization of pre-anthesis uptake of N, with respect to grain protein concentration, and found that two cultivars, Hereward and Pastiche, had high GPD. It is therefore possible that the cultivar used in the present study, Hereward, may well be more responsive to the effects of lower leaf area duration and subsequent grain N yield than other cultivars.
Agronomical implications
Obviously, strobilurins are not the first group of fungicides for which non-target effects, i.e. the extra effects other than curative and protective effects against plant diseases (Vyas Reference Vyas1988), have been claimed. The distinction between target effects and non-target effects is not always clear. In the present study, for example, in Expt III-C the severity of S. tritici was much higher on untreated plots compared with those treated with fungicides. The significantly lower MGW and HI observed on untreated plots compared with those treated with fungicides is likely to be a result of the amount of S. tritici on untreated plots. Therefore, from an agronomic point of view, it appears sensible not to lose sight of the importance of fungicide efficacy and, for example, pathogen resistance to fungicides (Russell Reference Russell2005), rather than concentrating on possible non-target effects in isolation.
The data from Expt I suggests that N uptake continued during grain fill for the plots treated with the highest N rate of 140 kg/ha, which was set in accordance with commercial practice following the Fertiliser Recommendations (RB209) (MAFF Reference Maff2000), while for the plots treated with the less N (0 and 100 kg/ha) appeared to have stopped N uptake before grain fill. Although 101 kg/ha of N was recovered in the above-ground part of the crop, even on the plots that received no N fertilizer in Expt I (Tables 8 and 9), the fact that a possible dilution of grain N occurred in the plots treated with fungicides (Table 10) leads to the possibility that this experiment could have suffered from some level of N deficiency. The observation that N uptake estimated in Expts III-B and III-C was greater than that estimated in Expt I by c. 50 and 80 kg/ha, respectively, (Table 9) may confirm the possibility that N application was not sufficient in Expt I in producing grains of good protein concentration. In such a situation, the increased grain N yield observed on the plots treated with a mixture of epoxiconazole and trifloxystrobin (Tables 8 and 9) might have a significant meaning to growers. Apart from soil sampling prior to fertilization, measuring canopy N might help determine optimum N fertilization rates in a similar way to that suggested by Henke et al. (Reference Henke, Sieling, Sauermann and Kage2009) for oilseed rape.
Another common but important observation made in the present study was that the wheat crop recovered much greater N than that applied to the soil as fertilizer N (Zhu et al. Reference Zhu, Midmore, Yule and Radford2006). Even supposing that only grain N is taken away from the field, the N budget would be negative especially when several years of cereal production occurred. In order to use the field in the long term, the removed N should be returned to the field preferably together with other nutrients in some way such as manure application or planting legumes (Ryan et al. Reference Ryan, Singh, Pala, Makhboul, Masri, Harris and Sommer2010).
CONCLUSION
The present study has provided evidence to confirm the main non-fungicide in-field crop effects of strobilurin fungicides such as increased soil N uptake (Bryson Reference Bryson2000), longer GLAD, increased grain yield and improved N use efficiency (Ruske et al. Reference Ruske, Gooding and Jones2003a), which have been reported by other workers. In addition it has revealed the possibility that these effects may only be exploited fully with sufficient levels of N fertilization. Most interestingly, however, the present study provides new evidence to suggest that strobilurins, and in particular trifloxystrobin, can affect the duration of leaves below the flag leaf and that the N within these layers of the canopy is important and can contribute to grain N uptake and increased grain N concentration.
The objective of the present study was to investigate the interactions between N and strobilurin-fungicide-containing spray programmes with respect to yield and grain N. Overall, there was no consistent interaction between N and fungicide spray programme across the study. Some interactions, however, were observed in individual experiments. As would be expected, the accumulation of DM and N increased with increased rates of N application. Fungicide programmes also increased grain yield and grain N but the epoxiconazole plus trifloxystrobin treatment appeared to have a greater effect than the other fungicide treatments and this was most pronounced at high N rates. This response to the epoxiconazole plus trifloxystrobin treatment appears to explain the significant interactions that occurred between N and fungicide.
The authors would like to thank the many members of Harper Adams staff who contributed much to this work. Support given by K. Okada and Prof. T. Horie is gratefully acknowledged. This work was financially supported by a PhD studentship from Harper Adams University College.