Introduction
Giardia intestinalis is a parasite protozoan that inhabits its vertebrate hosts' upper small intestine and is the most common cause of waterborne diarrhoea worldwide. Giardiasis' clinical manifestations vary from an asymptomatic or mild infection to an acute or chronic disease associated with diarrhoea and malabsorption. Giardia infection initiates with the cyst form's ingestion, which excysts in the upper small intestine where Giardia stabilizes, and the parasite affects the host. Parasite–host relationships are of great importance, and how Giardia nourishes itself, causing adverse effects on the host, has been the object of interest in scientific research.
Giardia has two nuclei and a glycogen-rich cytoplasm. The membrane system of G. intestinalis is considered very simple, with a unique set of vesicles, named peripheral vesicles (PVs), the endoplasmic reticulum network and mitosomes. Recently, Acosta-Virgen et al. (Reference Acosta-Virgen, Chávez-Munguía, Talamás, Guillén, Martínez-Higuera, Lazcano, Martínez-Palomo and Espinosa-Cantellano2018) suggested, using several approaches, the presence of peroxisomes. The absence of a typical Golgi complex and a canonical lysosome is a matter of discussion (Kattenbach et al., Reference Kattenbach, Pimenta, de Souza and Pinto da Silva1991; Tai et al., Reference Tai, Ong, Chang and Su1993; Lanfredi-Rangel et al., Reference Lanfredi-Rangel, Attias, Carvalho, Kattenbach and De Souza1998, Reference Lanfredi-Rangel, Kattenbach, Diniz and de Souza1999; Wampfler et al., Reference Wampfler, Tosevski, Nanni, Spycher and Hehl2014).
The PVs are oval organelles 100–200 nm in size, located in the cell periphery, below the plasma membrane, and considered the endosomal/lysosomal system in Giardia (Feely and Dyer, Reference Feely and Dyer1987; Kattenbach et al., Reference Kattenbach, Pimenta, de Souza and Pinto da Silva1991; Rivero et al., Reference Rivero, Vranych, Bisbal, Maletto, Ropolo and Touz2010). The PVs participate in the endocytosis process, digestion and retrograde transport of the parasite (Lanfredi-Rangel et al., Reference Lanfredi-Rangel, Attias, Carvalho, Kattenbach and De Souza1998). Previous studies demonstrated the presence of resident enzymes such as acid phosphatases, sulphur-binding proteins (SH) and glucose-6-phosphatase in the PVs as well as the accumulation macromolecules such as albumin, peroxidase, transferrin, low-density lipoprotein, and accumulating fluid-phase and membrane-bound molecules and in PVs (Kattenbach et al., Reference Kattenbach, Pimenta, de Souza and Pinto da Silva1991; Tai et al., Reference Tai, Ong, Chang and Su1993; Lanfredi-Rangel et al., Reference Lanfredi-Rangel, Attias, Carvalho, Kattenbach and De Souza1998). The uptake of soluble material from the environment into PV is not selective, in contrast to further retrograde transport that allows some substances to rapidly cross over into the proximal endoplasmic reticulum (ER) (Abodeely et al., Reference Abodeely, DuBois, Hehl, Stefanic, Sajid, De Souza, Attias, Engel, Hsieh, Fetter and McKerrow2009). The presence of protein complexes associated with the endocytic machinery in Giardia has been reported, as clathrin heavy chain (GlCHC), subunits of the AP2 heterotetramer (GlAP2) and Giardia dynamin-related protein (GlDRP) with a discrete localization by fluorescence microscopy (Gaechter et al., Reference Gaechter, Schraner, Wild and Hehl2008; Rivero et al., Reference Rivero, Vranych, Bisbal, Maletto, Ropolo and Touz2010). Besides, a protein interactome of GlCHC revealed all of the conserved factors in addition to a novel protein, a putative clathrin light chain (Zumthor et al., Reference Zumthor, Cernikova, Rout, Kaech, Faso and Hehl2016).
The endoplasmic reticulum in Giardia is an extensive reticular system distributed from the nuclear envelopes throughout the cell body. The presence of a tubule-vesicular contiguous compartment in Giardia that functions as endoplasmic reticulum and endosomes/lysosome with connections with the PVs was proposed (Abodeely et al., Reference Abodeely, DuBois, Hehl, Stefanic, Sajid, De Souza, Attias, Engel, Hsieh, Fetter and McKerrow2009). The presence of some specific marker proteins in this organelle, similar to those of higher eukaryotic cells, such as glucose-6-phosphate, a resident ER enzyme, detected by electron microscopy cytochemistry was reported (Lanfredi-Rangel et al., Reference Lanfredi-Rangel, Attias, Carvalho, Kattenbach and De Souza1998). Previous studies demonstrated that although G. intestinalis has a conventional secretory trafficking, some elements, such as the post-translational modification machinery, are missing entirely. An extensive genomic and biochemical studies indicated that the parasite lacks several nucleotide sugar transporters (Banerjee et al., Reference Banerjee, Cui, Robbins and Samuelson2008). Thus, in G. intestinalis, the ER, in addition to protein synthesis, has different functions such as endocytic activities and extracellular material degradation, being a pluripotent compartment (Abodeely et al., Reference Abodeely, DuBois, Hehl, Stefanic, Sajid, De Souza, Attias, Engel, Hsieh, Fetter and McKerrow2009).
Giardia does not present mitochondria; however, double-membrane small organelles, 200 nm in size, presenting some mitochondria characteristics named mitosomes, were described (Tovar et al., Reference Tovar, Fischer and Clark1999; Tovar et al., Reference Tovar, León-Avila, Sánchez, Sutak, Tachezy, van der Giezen, Hernández, Müller and Lucocq2003). The mitosomes are distributed over the cytoplasm and also placed between the flagellar axonemes. Mitochondrial characteristics such as biosynthesis of Fe-S clusters, a TOM and TIM protein family transport machinery were found in mitosomes (Tachezy and Smid, Reference Tachezy, Smid and Tachezy2008). However, mitosomes do not present several mitochondrial characteristics, such as ATP synthesis, the citric acid cycle, oxidative phosphorylation, heme biosynthesis, presence of DNA, lipid metabolism and the amino acid and urea cycles (Makiuchi and Nozaki, Reference Makiuchi and Nozaki2014). One important finding was the presence of an iron-sulphur complex (IscS and IscU proteins) (Tachezy et al., Reference Tachezy, Sanchez and Muller2001). Chaperones, such as Cnp60 and HSP70, are also present (Midlej et al., Reference Midlej, Penha, Silva, de Souza and Benchimol2016).
During the differentiation of trophozoite to cysts, two types of membrane-bounded vesicles appear, the encystation-specific vesicles (ESVs) (Faubert et al., Reference Faubert, Reiner and Gillin1991) and the encystation carbohydrate-positive vesicles (Midlej et al., Reference Midlej, Meinig, de Souza and Benchimol2013), and both act to build the cyst wall (CW), a constitutive and essential structure of cyst. The ESVs are large vesicles (1 μm of size), participate in CW formation and appear at the beginning of the encystation process (Faubert et al., Reference Faubert, Reiner and Gillin1991). The protein content of the ESVs is the cyst-wall proteins 1–3, which originate in the endoplasmic reticulum (Hehl and Marti, Reference Hehl and Marti2004).
The Golgi complex is an organelle characterized by stacked cisternae, often located around the nucleus and close to the endoplasmic reticulum. However, an organelle that fits with these criteria has not been identified in G. intestinalis. Some groups proposed a similarity between the Giardia's ESVs and the Golgi complex (Luján et al., Reference Luján, Marotta, Mowatt, Sciaky, Lippincottschwartz and Nash1995; Marti et al., Reference Marti, Li, Schraner, Wild, Kohler and Hehl2003; Stefanic et al., Reference Stefanic, Morf, Kulangara, Regös, Sonda, Schraner, Spycher, Wild and Hehl2009; Ebneter et al., Reference Ebneter, Heusser, Schraner, Hehl and Faso2016), but this is still very controversial. Besides, classical Golgi markers such as GM130, galactosyl transferases or the trans-Golgi network marker Rab6 were not detected in the parasite. Thus, a typical Golgi in G. intestinalis is not present (Dacks and Field, Reference Dacks and Field2007).
Because the endocytosis is an essential function in all cells and especially in parasites, investigations concerning the ingestion of molecules and other elements in the Giardia habitat and its traffic are necessary and still incomplete.
Giardia nutrition has been the subject of several works, mainly focusing on endocytosis by the parasite. In the literature, experimental designs start with Giardia growth in axenic culture media, which contains essential nutritional elements.
In vivo, Giardia inhabits the human gut, which contains 500–1000 microorganism's species, and its diversity is known as ‘microbiome’. Thus, in natural conditions in the intestine of a human, Giardia has the company of thousands of other organisms. The possibility of nourishing itself from this flora raises an important question: Do our laboratory experiments, where we grow Giardia in an axenic environment, answer the right questions to its mode of nutrition? Using the culture medium in the laboratory experiments, we can observe only fluid-phase endocytosis, since the medium only contains macromolecules. Thus, several questions regarding this endocytic pathway remain unanswered: would Giardia be able to phagocytize large particles or microorganisms present in its natural habitat? In the present study, we decided to evaluate Giardia's behaviour in an environment where microorganisms and large materials are present. Our observations show clearly that Giardia, once appropriately stimulated, can ingest large particles and even whole microorganisms.
Materials and methods
Cell culture
Trophozoites of G. intestinalis WB strain (ATTC, USA, No. 30957) were cultured for 48 h at 37°C in TYI-S-33 medium (Keister, Reference Keister1983) enriched with 10% fetal bovine serum and supplemented with 0.1% bovine bile.
Endocytic assays
Incubation with ferritin and albumin
Cells were washed and transferred to phosphate-buffered saline (PBS). They were incubated for periods varying from 1 to 60 min in a solution containing 5% native ferritin in PBS, pH 7.2 or 1 mg mL−1 bovine serum albumin (BSA; Sigma, USA), at 37°C. After incubation, the parasites were washed twice with PBS and fixed in 2.5% glutaraldehyde in 0.1 M cacodylate buffer, pH 7.2, and processed for scanning electron microscopy (SEM) and transmission electron microscopy (TEM) as described below.
Incubation with polystyrene microspheres
For TEM polystyrene microspheres, sizes of 10 nm, 110 nm or 0.9 μm in diameter were used (Polysciences, USA), according to a previously published protocol (Benchimol et al., Reference Benchimol, Batista and De Souza1990). They were suspended in PBS and allowed to interact with the parasites for 1, 5, 20, 30 and 60 min. Afterward, the cells were washed twice with PBS and fixed in 2.5% glutaraldehyde in 0.1 M cacodylate buffer, pH 7.2, plus 5 mm CaCl2, and postfixed in 1% OsO4, in 0.1 M cacodylate buffer plus 0.8% potassium ferrocyanide. After that, the cells were washed in PBS, dehydrated in acetone, and embedded in Epon. Besides, in some experiments, latex particles were coated with cationized ferritin (1 mg mL−1, final concentration of about 105 particles mL−1) or albumin (1 mg mL−1 BSA, Sigma) and used in interactions with the parasites.
Incubation with FluoSpheres
Living Giardia was incubated with FluoSpheresTM carboxylate-modified 100 nm, red (580/605), from Invitrogen (ThermoFisher Scientific), diluted 5000 times, and observed at 1, 5, 15, 30, 60, 120 min in medium without serum. The FluoSpheres were coated with BSA 1% before the incubation, sonicated and centrifuged to remove particle clusters. The samples were observed both alive and fixed for 10 min with 4% formaldehyde diluted in 0.1 M cacodylate buffer, pH 7.2. The fluorescence microscope used was Zeiss Elyra (Germany) in Confocal mode (LSM 710 system), Laser 543 nm, Objective Lens EC Plan-apochromat 63×/1,4.
Incubation with Saccharomyces cerevisiae
Saccharomyces cerevisiae was obtained from yeast powder (Fleischmann & Royal, Jundiaí, SP, Brazil), and diluted in warm water (37°C). Yeast cells were washed three times with warm 0.1 M sterile PBS (pH 7.2) for 5 min at 3000 g and suspended in fresh TYM medium without serum, the concentration of 5 × 107 cells mL−1. The yeast cells were used immediately for interaction assays following Pereira-Neves and Benchimol (Reference Pereira-Neves and Benchimol2007) protocol. Co-incubation of G. intestinalis (WB strain) with yeast cells was performed in medium without serum at parasite/yeast cell ratios of 1:10 and 1:40 for different times (1, 5, 20, 30, 60 min) at 37°C. Triplicate preparations were analysed by differential interference contrast (DIC) microscopy. After interaction, samples were processed for TEM and SEM.
Incubation with bacteria
Escherichia coli, strain 346, was grown at 37°C for 24 h in BT broth (Bacto-tryptone, Difco) medium. Giardia was collected by centrifugation at 6000 g for 5 min. Next, they were allowed to interact with the bacteria for periods varying from 1 to 60 min using bacteria: protozoa ratio of 10:1. After the interaction, the cells were fixed for 2 h at room temperature in a solution containing 2.5% glutaraldehyde in 0.1 M cacodylate buffer (pH 7.2) and processed for SEM and TEM as described below. In some experiments, a mixture of bacteria, yeasts and albumin was used.
Light microscopy
To evaluate the parasites' behaviour in contact with different materials after interaction, living cells were observed under light microscopy, in DIC and phase contrast mode (Zeiss Aphiovert light microscopy, Germany).
Scanning electron microscopy
Cells were allowed to adhere to Poly-L-lysine-coated glass coverslips and then fixed in 2.5% glutaraldehyde in 0.1 M cacodylate buffer, pH 7.2 for 60 min. After that, the cells were postfixed for 5 min in 1% OsO4, dehydrated in ethanol, critical point dried with CO2, sputter-coated with gold-palladium and examined in a Jeol 5800X SEM operating at 15 KV.
Transmission electron microscopy
Samples were fixed in 2.5% glutaraldehyde in 0.1 M cacodylate buffer (pH 7.2). They were then postfixed in 1% osmium tetroxide (OsO4) containing 0.8% potassium ferrocyanide in 0.1 M cacodylate buffer for 40 min, dehydrated in acetone and embedded in epoxy resin. Ultrathin sections (60–70 nm thick) were collected and stained with uranyl acetate and lead citrate. At the end of processing, the samples were observed using a Jeol 1210 (Jeol, Japan).
Cytochemistry for carbohydrate detection (Thiéry, 1967): Thiéry's technique (periodic acid-thiosemicarbazide-silver proteinate)
After a routine fixation and Epon embedding procedure, the ultrathin sections were collected on gold grids, treated for 20 min with 1% periodic acid, incubated for 48–72 h in 1% thiosemicarbazide, and exposed to 1% silver proteinate for 30 min. The sections were analysed using a JEOL 1210 TEM. The controls were performed by omitting the periodic acid step.
Results
Light microscopy
To observe whether there would be interaction and possible ingestion of large materials at the light microscopy level, living Giardia were incubated with BSA-coated FluoSpheresTM carboxylate-modified 100 nm, red (580/605) and observed at different periods. Video microscopy, phase contrast microscopy, DIC mode and confocal laser scanning microscopy were used (Fig. 1). The samples were observed either alive (Fig. 1a and c) or fixed for 10 min with 4% formaldehyde (Fig. 1b, d and e). Different focal planes were analysed (Z-level) to check if the beads were outside or inside the cell. After a few min, we observed red spots outside the cells and on the cell's surface. Living cells showed an intense beating of ventral flagella (not shown), and beads were also found in the ventral flagella exit region. After 30 min, several cells' compartments exhibited bright fluorescence, some of them around the nucleus (Fig. 1b).
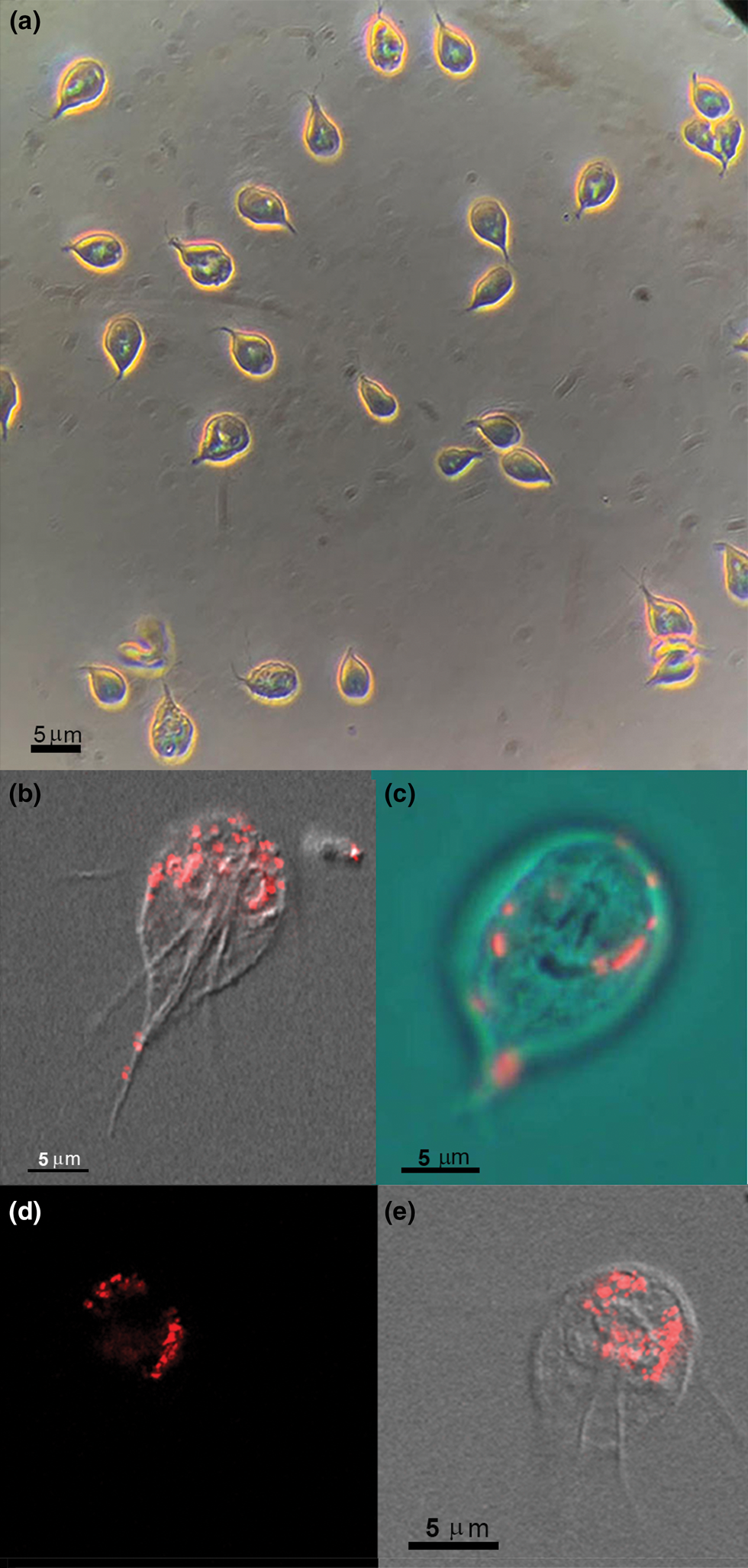
Fig. 1. Incubation with 100 nm red FluoSpheres BSA coated for 30–60 min and observation by phase contrast (a) or confocal laser-scanning microscope and DIC (b–e). (a) General field view at low magnification to observe the living trophozoites during FluoSpheres interaction for 30 min using phase-contrast microscopy. Vacuoles containing the fluorescent beads are seen in the Giardia cytosol as bright spots. (b–e) Overlay of DIC and confocal fluorescence images after Giardia interaction with 100 nm red FluoSpheres. In (b, d, e), Giardia was allowed to interact for 60 min, fixed and observed, whereas (c) is a living cell after 30 min interaction, and the image is presented in RGB overlay. The 100 nm beads sizes are below the light microscopy resolution; thus, the red spots are vacuoles with beads concentration. Virtual sections were made to check if the beads are on or in the cell. (d, e) Fluorescence (d) and DIC overlay (e) of the same section.
Scanning electron microscopy
Since by light microscopy we observed an intense flagella movement and preferential interaction of the large particles at the region where the ventral flagella exit, we decided to check using SEM (Figs 2, 3 and 6a). The other flagella also contact the large materials, as seen by SEM (Fig. 2a and c). Giardia's whole-cell surface also interacted with all material used here (Fig. 2f), even the bare zone (Fig. 3f) of the ventral disc. Large materials as yeasts, which measures 4–7 μm, were also seen in the process of interaction (Figs 2 and 3e), and the anterior flagella seemed to participate in this process (Fig. 2a and c). In the first 5 min of incubation, we noted plasma membrane ripples (Fig. 1, asterisks; Fig. 3b and c) and large cell projections (Fig. 4a–c). All materials tested, large or small, such as bacteria, yeasts, coated latex beads, albumin and ferritin, were taken by the cells. We did not observe the ingestion of uncoated latex beads. When we used short periods of incubation, smaller markers were preferentially seen in the region where the ventral flagella exit (Figs 3 and 6a). Large extensions and invaginations on Giardia's cell surface were observed on the parasite's dorsal region after incubation with cells such as bacteria, yeasts or polystyrene microspheres (Figs 4, 6–8). An interesting observation was the change in the Giardia cell surface: cells grown in axenic culture medium presented a smooth and uniform texture (Fig. 5a) when observed by routine SEM whereas, after endocytosis, Giardia's surface showed clear signs of irregularities and wrinkles, probably due to the intake of large materials as observed after 20 min of incubation with E. coli (Fig. 5b).

Fig. 2. Giardia SEM after incubation with live yeasts (S. cerevisiae) (a–f) and also together with latex beads (a) or E. coli (b) for 5 min. Notice that flagella adhere to all these materials. Figures (e, f) show that the parasite's plasma membrane exhibits projections (arrows) in the areas of contact with the yeasts. Y, yeast; B, bacteria; L, latex beads. Artificially coloured.

Fig. 3. Giardia trophozoites observed by SEM after incubations for 10 min with different materials: (a, b) with ferritin-coated latex beads presenting two different sizes (LF); (c) with E. coli (B) and uncoated latex beads (L); (d) with albumin-coated latex beads; (e) with a mixture of ferritin-coated latex beads (L), bacteria (B) and yeasts (Y); (f) with ferritin-coated latex beads (L). Notice in (a) that there is adhesion of the bead with one flagellum. The small particles over the cell are free ferritin. In (b), the arrowhead points to an opening below the ventral disc, whereas the arrows indicate a large compartment below the plasma membrane in images (b, c). In (d, e), the uncoated latex beads (L) are in the process of internalization precisely in the region of the emergence of the ventral flagella (arrows). In (f), two uncoated latex beads have adhered to the ventral disc bare zone. Images were artificially coloured.

Fig. 4. SEM. After 5 min of incubation with E. coli (B) and free ferritin (spots on the membrane), Giardia trophozoites' cell surface. Significant changes on the cell surface were observed in the parasite's dorsal region, similar to the formation of large pseudopods (arrows). The bacteria were artificially coloured.

Fig. 5. Scanning electron microscopy of G. intestinalis under two different conditions: in (a) a cell grown in an axenic culture medium and (b) a cell after 20 min of incubation with E. coli (B) and with free ferritin. There is a significant change in the cell surface, which is much wrinkled after the materials' internalization.
Transmission electron microscope
After different incubation times with Giardia trophozoites, ultrathin sections were analysed using a TEM. Small markers, such as free albumin and ferritin, were found in the PVs. We observed markers' entry through all cell membrane places, especially where the ventral flagella emerge (Fig. 6), confirming observations made by light microscopy and SEM. After 5 min of co-incubation, membrane's expansions, similar to pseudopods, were observed when coated latex beads or bacteria were used (Figs 7a–c, 8b and c, 9a and b). A process of adhesion of ferritin or albumin-coated latex beads with these projections were noted (Fig. 7b and inset). After incubation with yeasts, a sharp interaction between Giardia and yeast was observed (Fig. 7d). After 30 min of incubation, incorporated elements such as coated latex beads were found in a vacuole (Fig. 7e). When we carried out simultaneous incubations with bacteria and yeasts, or bacteria and latex beads (Figs 8 and 9), we noticed that the parasites present drastic changes in shape, and membrane projections appeared. In Fig. 8c, we show a latex bead already incorporated while the parasite presents a dramatic change in shape, apparently trying to reach a bacterium, forming a pseudopod-like extension (Figs 8b and 9). One of these projections clearly shows filamentous structures (Fig. 9b). In some situations, Giardia has changed its shape profoundly, assuming an amoeboid form (Figs 8c and 9).

Fig. 6. (a) Scanning electron microscopy of small latex beads coated with BSA when in interaction with G. intestinalis for 5 min Notice that the beads are located in the area of the ventral flagella (VF) exit (arrow). D, ventral disc. (b) Transmission electron microscope (TEM). Giardia trophozoite after incubation for 5 min with yeasts (Y). Note the contact of the ventral flagella (VF) with the microorganism and the yeast location in the region of exit of the ventral flagella, just below the area of the ventral disc. N, nucleus; F, flange; Ax, axonemes.

Fig. 7. Images obtained with the transmission electron microscope. In (a, b), albumin-coated latex beads (L) are observed adhered to on Giardia's cell surface. Expansions of the membrane (arrows), similar to pseudopods, and even a contact with one of the latex beads are noted (b). An adhesion point between the Giardia membrane and a latex bead's surface is observed in the inset. In figure (c), an endocytosis experiment was performed where tiny latex beads were coated with ferritin. There are evident expansions of the membrane (arrows) forming pseudopods and even a closure of one of these expansions keeping the material inside. Notice a compartment (C) in the cytosol. In figure (d), a large yeast (Y) is in phagocytosis by Giardia. In figure (e), an incubation with latex beads coated with ferritin was made, and one of the particles (L) is observed in a large internal vacuole of the trophozoite. N, nucleus.

Fig. 8. TEM of several different incubations of G. intestinalis with bacteria (a, b, c) coated BSA latex beads (c), and yeasts (a, e). In (d), incubation was performed only with albumin, and it is observed that the peripheral vesicles (PV) present this protein inside. A high concentration of albumin in the process of endocytosis is seen in the region of the disc (D) where the ventral flagella exit (arrows) and the peripheral vesicles containing albumin are present. In (a, e), the experiments were carried out with yeasts (Y), and the presence of three yeasts is already observed inside a vacuole in (e). B, bacteria; D, ventral disc; N, nucleus.
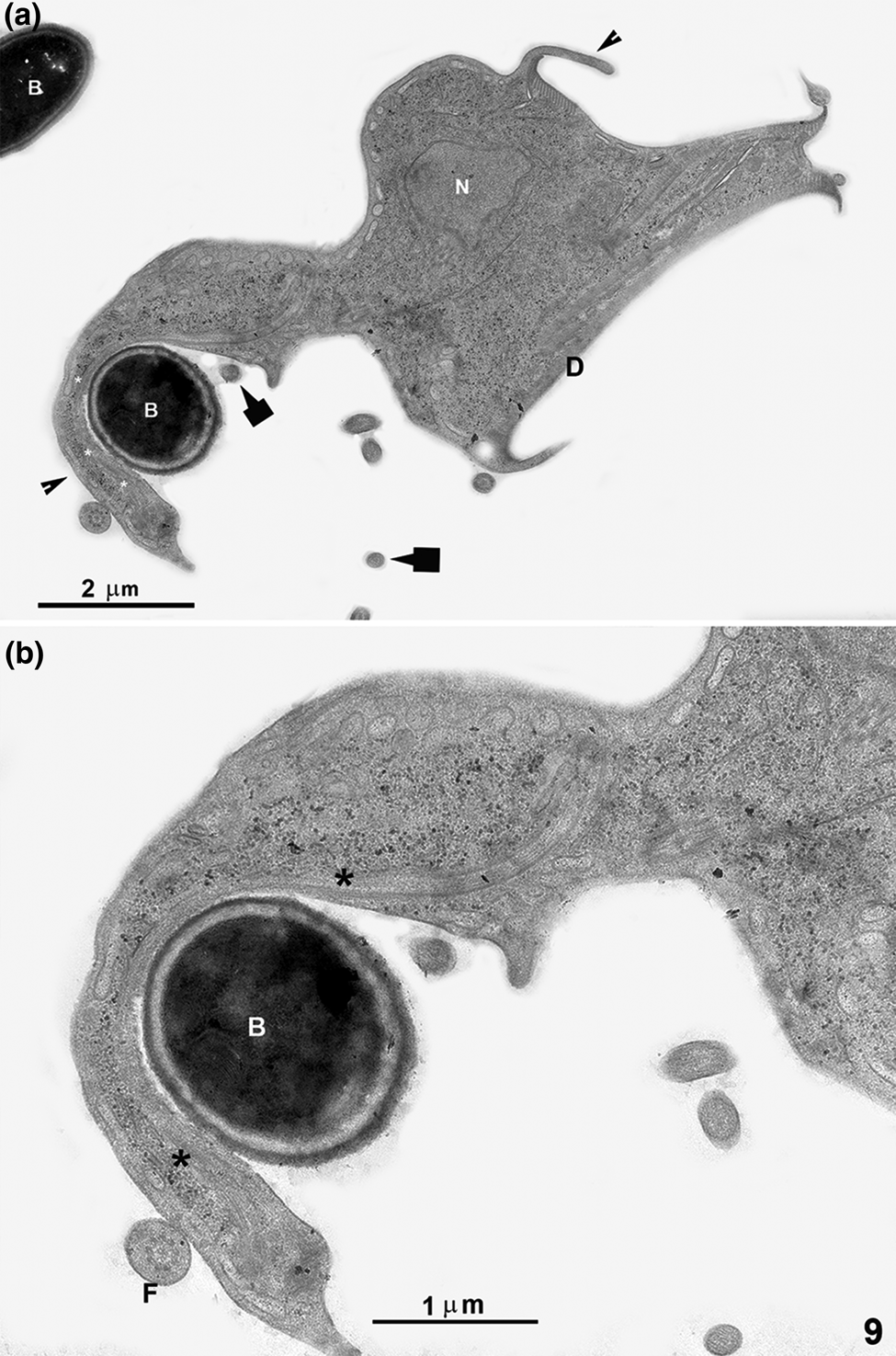
Fig. 9. TEM of G. intestinalis after interaction with bacteria and small latex beads for 5 min. The parasite has drastically changed its shape, presenting an amoeboid form with short and long projections (arrowheads). One long membrane projection, similar to a pseudopod, is seen in contact with a bacterium (B). In this membrane projection, filamentous structures (asterisks) can be noted. The arrows point to 100 nm latex beads. Figure (b) is a higher magnification of (a). D, ventral disc; F, flagellum; N, nucleus.
We decided to compare fluid-phase endocytosis with large particle phagocytosis and then incubated the cells with albumin (Fig. 8d). The difference was striking since albumin incorporation occurred only in PVs. Besides, we noticed an intense concentration of albumin in the ventral flagella exit region, where many PVs containing albumin were also seen (Fig. 8d). After 10 min of incubation, yeasts were found inside a vacuole (Fig. 8e).
When we searched for intracellular localization of materials tested here, we were able to find BSA-coated latex beads in vacuoles, which are not the PVs by its size and localization (Fig. 10a–f and h). Single large beads were seen in a large vacuole (Fig. 10a–e), whereas several small beads were clustered in other vacuoles (Fig. 10f–h).

Fig. 10. Intracellular localization of BSA-coated latex beads. Giardia intestinalis were incubated with polystyrene microspheres (L), sizes of 10 nm, 110 nm or 0.9 μm and fixed after 30 min (a–f, h). Some cells presented beads internalization (L). The large beads were seen alone in a large vacuole (a–e), whereas several small beads are together in a vacuolar structure (f, arrow). Figure (h) is a higher magnification of (f). Figure (g) is an image of the parasite after incubation with bacteria for 30 min using cytochemistry [periodic acid-thiosemicarbazide-silver proteinate (Thiéry, Reference Thiéry1967) for carbohydrates detection]. A positive reaction is observed in one large vacuole where a possible biological material degradation is observed (arrow). Ax, flagella axonemes; D, ventral disc; N, nucleus; F, flagellum.
Cytochemistry
Using the periodic acid-thiosemicarbazide-silver proteinate (Thiéry, Reference Thiéry1967), a cytochemical technique associated with electron microscopy, carbohydrates were detected in a large vacuole. After incubation with bacteria, there was material in a vacuole under an apparent process of degradation (Fig. 10g).
Discussion
Giardia intestinalis is a parasite whose endocytic pathway has been previously studied. There is consensus that the PVs found below the plasma membrane of this parasite forms an interconnected network, which includes part of the endoplasmic reticulum, correspond to Giardia's early and late endosomes and the lysosomal system (Feely and Dyer, Reference Feely and Dyer1987; Kattenbach et al., Reference Kattenbach, Pimenta, de Souza and Pinto da Silva1991; Rivero et al., Reference Rivero, Vranych, Bisbal, Maletto, Ropolo and Touz2010). In all these studies, trophozoites were grown in an axenic medium, where essential macromolecules are supplied to the parasite during its in vitro growth. All markers used until now were restricted to follow the fluid-phase or receptor-mediated endocytosis.
It is essential to consider that in vivo, Giardia is found in the small intestine where there is an enormous population of other microorganisms that are part of the intestinal microbiota. In this way, it was missing an analysis of this parasite's behaviour in experimental conditions closer to those found in vivo.
In 1979, Reference Nemanic, Owen, Stevens and MuellerNemanic et al. reported bacterial-like structures, considered as endosymbionts, in the cytoplasm of Giardia muris and G. lamblia collected from mice intestines. The endosymbionts found by the authors were both trophozoites and cysts. Subsequently, Sogayar and Gregorio (Reference Sogayar and Gregorio1989) showed internalized bacteria in Giardia duodenalis found in rat intestine (Rattus rattus). The authors did not characterize either the species of Giardia or the bacteria found in the gut. They published that the bacteria were in phagocytic vacuoles without signs of bacteria digestion. The authors also claimed that the bacteria were observed attached to the trophozoite membrane until their total incorporation by phagocytosis. Here, three essential points caught my attention: (1) there is no mention of the participation of PVs in the endocytic mechanism described; (2) Giardia were collected from a naturally infected animal, which means that the parasites were found in a natural community with several microorganisms; (3) the work indicated the capacity of ingestion of a large material by Giardia. These observations lead us to explore further, using a more controlled experimental system, G. intestinalis trophozoites' ability to ingest large particles.
Preferential place for uptake
Our present observations showed that Giardia uptake of ferritin and other materials occurred throughout the cell surface, but preferentially through the ventral flagella's exit, just below the ventral disc. Abodeely et al. (Reference Abodeely, DuBois, Hehl, Stefanic, Sajid, De Souza, Attias, Engel, Hsieh, Fetter and McKerrow2009) made a similar observation when they used 40 nm biotin-conjugated FluoSpheres. The authors also found that clathrin, an essential protein involved in receptor-mediated endocytosis, was abundant near the ventral flagella (Abodeely et al., Reference Abodeely, DuBois, Hehl, Stefanic, Sajid, De Souza, Attias, Engel, Hsieh, Fetter and McKerrow2009). We also noted the same preferential place, the ventral flagella exit, although the whole Giardia cell surface showed contact with all materials tested.
Despite extensive EM studies of the Giardia endocytic system, a detailed morphological characterization of the incorporated molecules' destination and its digestive process was not carried out. This parasite has been described as presenting an endomembrane system restricted to a few structures such as the endoplasmic reticulum and PVs, a contiguous collaborative compartment working in harmony (Abodeely et al., Reference Abodeely, DuBois, Hehl, Stefanic, Sajid, De Souza, Attias, Engel, Hsieh, Fetter and McKerrow2009). Although numerous articles have been published with excellent results on endocytosis mechanisms in Giardia, they focused on using small markers and their incorporation by fluid-phase endocytosis or/and clathrin-mediated receptor (Hernandez et al., Reference Hernandez, Castillo, Roychowdhury, Hehl, Aley and Das2007; Zumthor et al., Reference Zumthor, Cernikova, Rout, Kaech, Faso and Hehl2016). The previous studies are unanimous in describing the participation and importance of PVs as sites for incorporating and possibly digestion of the ingested material. Based on the localization of lysosomal enzymes such as acid phosphatase and cysteine proteases, PVs have been described as having lysosomal properties (Feely and Dyer, Reference Feely and Dyer1987; Lindmark, Reference Lindmark1988; McCaffery and Gillin, Reference McCaffery and Gillin1994), which means they acidify and behave as digestive organelles. Cytochemical, biochemical or molecular-tag analyses using the search for acid phosphatase has been carried out by several authors, who invariably found this enzyme in some PVs and also in the endoplasmic reticulum (Lindmark, Reference Lindmark1988; Kattenbach et al., Reference Kattenbach, Pimenta, de Souza and Pinto da Silva1991; Lanfredi-Rangel et al., Reference Lanfredi-Rangel, Attias, Carvalho, Kattenbach and De Souza1998; Touz et al., Reference Touz, Kulakova and Nash2004; Rivero et al., Reference Rivero, Miras, Feliziani, Zamponi, Quiroga, Hayes, Rópolo and Touz2012). A previous work raised the possibility of an alternative endosomal compartment that lies adjacent to the ER or in direct contact with it (Abodeely et al., Reference Abodeely, DuBois, Hehl, Stefanic, Sajid, De Souza, Attias, Engel, Hsieh, Fetter and McKerrow2009).
In the present work, occasional cells with membrane projections resembling pseudopods-like structures were noticed, when trophozoites were incubated in the presence of large materials such as coated latex beads, bacteria and yeasts. One interesting observation was the drastic parasite change in shape and an extensive projection towards one bacterium. This dramatic change in shape has not been described previously and suggests that the protozoan can detect signals indicating the necessity to internalize a microorganism. The presence of filaments in these projections corroborates the idea that they correspond to a true pseudopod involved in a phagocytic process, a fact not yet described for Giardia.
Also, we were able to find several images of the large beads in cellular structures that do not correspond to the PVs, either by size or location. These vacuoles have not yet been described in the Giardia literature. They may represent either a new organelle with a lysosomal function or a path that has not yet been fully elucidated in Giardia. These vacuoles were found in different places in the cell, but preferably close to the nucleus. The presence of a perinuclear structure as an alternative place of incorporating external materials from PV had already been suggested in Abodeely et al.'s work (Abodeely et al., Reference Abodeely, DuBois, Hehl, Stefanic, Sajid, De Souza, Attias, Engel, Hsieh, Fetter and McKerrow2009). Further studies are needed to follow a probable endocytic alternative pathway in Giardia, and new experiments are underway to add new information in this regard.
Several studies have shown the relationship between bacteria and E. histolytica growth and virulence. They have noted that the bacterial microbiota may influence the behaviour and virulence of pathogens, their immune response, and lead to variability in the outcome of parasitic infections (Marie and Petri, Reference Marie and Petri2014; Bär et al., Reference Bär, Phukan, Pinheiro and Simoes-Barbosa2015). Thus, it would be important to study further the relationships between Giardia and other microorganisms in the same habitat to see if it affects the parasite's virulence. A greater understanding of Giardia's eating habits and its relationship with intestinal microbiota may provide knowledge for new treatments and expand knowledge in the field of intestinal parasitism.
In conclusion, our observations indicate that in addition to a classic fluid-phase and receptor-mediated endocytosis, Giardia is also able, when properly stimulated, to ingest large particles and microorganisms through a phagocytic-like process, as previously shown for other pathogenic protozoa such as Trichomonas vaginalis (Pereira-Neves and Benchimol, Reference Pereira-Neves and Benchimol2007) and Entamoeba histolytica (Orozco et al., Reference Orozco, Guarneros, Martinez-Palomo and Sánchez1983). Ingestion takes place, preferably at the ventral flagella exit. The ingested material most probably follows another pathway and is accumulated into large vacuoles.
Financial support
This work was supported by the Conselho Nacional de Desenvolvimento Científico e Tecnológico (CNPq) and Fundação Carlos Chagas Filho de Amparo à Pesquisa do Estado do Rio de Janeiro (FAPERJ), Grant number E-26/202.824/2017.
Conflict of interest
None.
Ethical standards
Not applicable.