1. Introduction
Members of Deinonychosauria, here treated as the monophyletic grouping of Dromaeosauridae and Troodontidae (sensu Norell et al. Reference Norell, Clark, Makovicky, Gauthier and Gall2001), were functionally didactyl theropods with a characteristic sickle-shaped claw on their second pedal digit that was raised off the ground during normal locomotion (Ostrom, Reference Ostrom1969). Although forming a conspicuous component of many Late Jurassic – Late Cretaceous terrestrial ecosystems in Laurasia and Gondwana (Makovicky & Norell, Reference Makovicky, Norell, Weishampel, Dodson and Osmólska2004; Norell & Makovicky, Reference Norell, Makovicky, Weishampel, Dodson and Osmólska2004; Weishampel et al. Reference Weishampel, Barrett, Coria, Loeuff, Xing, Xijin, Sahni, Gomani, Noto, Weishampel, Dodson and Osmólska2004; Turner et al. Reference Turner, Makovicky and Norell2012), their tracks were undescribed until 1994. However, the rate at which new deinonychosaur tracks were discovered increased exponentially in the years that followed. While a recent review in 2016 demonstrated the occurrence of deinonychosaur tracks from at least 16 localities (Lockley et al. Reference Lockley, Harris, Li, Xing, van der Lubbe, Falkingham, Marty and Richter2016 a, table 11.1), a plethora of ongoing discoveries, especially from China, has since increased the global track record of these dinosaurs to at least 26 localities (Table 1). The morphology of these prints is variable but generally they are didactyl (representing digits III and IV), sometimes with a rudimentary impression of a third digit (digit II) depending on the degree of retraction of digit II or the substrate conditions (Xing et al. Reference Xing, Lockley, Yang, Xu, Cao, Klein, Persons, Shen and Zheng2015 a). Certain monodactyl prints, which may occur in association with didactyl tracks, have also been attributed to deinonychosaurs (Xing et al. Reference Xing, Lockley, Tang, Romilio, Xu, Li, Tang and Li2018 a, b). Digits III and IV may be conjoined by the presence of a heel pad or divided into distinct, typically parallel toe marks with no ‘heel’ impression (Xing et al. Reference Xing, Lockley, Yang, Xu, Cao, Klein, Persons, Shen and Zheng2015 a, fig. 5). Digital pads are often visibly defined in well-preserved specimens. As deinonychosaurs are predominantly known from the Cretaceous Period (Makovicky & Norell, Reference Makovicky, Norell, Weishampel, Dodson and Osmólska2004; Norell & Makovicky, Reference Norell, Makovicky, Weishampel, Dodson and Osmólska2004), almost all convincing tracks attributed to these dinosaurs are Cretaceous in age; the only exceptions are a possible Middle Jurassic occurrence from Argentina (Casamiquela, Reference Casamiquela1964; De Valais, Reference De Valais2011; Xing et al. Reference Xing, Lockley, Tang, Romilio, Xu, Li, Tang and Li2018 a), and latest Jurassic or earliest Cretaceous occurrences from China (Xing et al. Reference Xing, Harris, Sun and Zhao2009 a, Reference Xing, Zhang, Lockley, McCrea, Klein, Alcalá, Buckley, Burns, Kümmell and He2015 b, Reference Xing, Lockley, Du, Zhang, Klein, Romilio, Persons, Wang, Li and Wan2020; Table 1).
Table 1. Summary of reported deinonychosaur track occurrences, modified and expanded from Lockley et al. (Reference Lockley, Harris, Li, Xing, van der Lubbe, Falkingham, Marty and Richter2016 a, table 11.1) and Xing et al. (Reference Xing, Lockley, Tong, Peng, Romilio, Klein, Persons, Ye and Jiang2019, table 4).
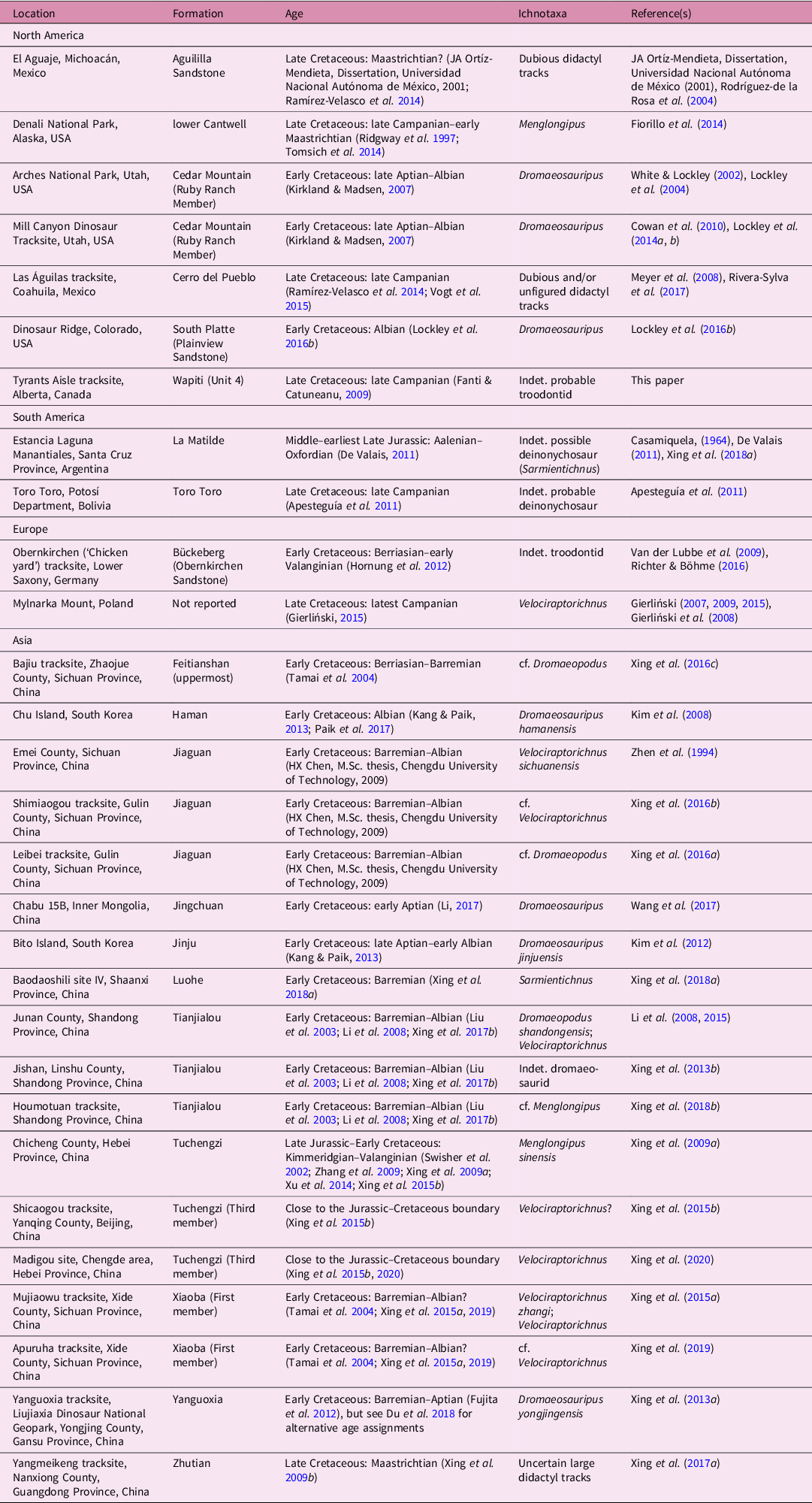
In 2018, the Boreal Alberta Dinosaur Project investigated a Late Cretaceous multi-taxic dinosaur-dominated tracksite along the Redwillow River in NW Alberta, Canada that preserves a didactyl footprint in possible trackway association with three monodactyl traces, as well as an additional isolated didactyl print located nearby on the same track layer. The purpose of this study is to document and identify these footprints, which contribute data to a poorly represented temporal and geographic interval in the deinonychosaurian track record.
2. Geological setting and age
The tracks described here occur at the Tyrants Aisle locality – a c. 1400 m2, dinosaur-dominated tracksite – situated along the Redwillow River in NW Alberta, c. 65 km WSW of the city of Grande Prairie (Fig. 1). The site exposes rocks from lower Unit 4 of the Wapiti Formation, which is upper Campanian in age (Fanti & Catuneanu, Reference Fanti and Catuneanu2009) and temporally equivalent to the Drumheller Member of the lower Horseshoe Canyon Formation in southern Alberta (Eberth & Braman, Reference Eberth and Braman2012; Eberth & Bell, Reference Eberth and Bell2014; Eberth & Kamo, Reference Eberth and Kamo2019). More specifically, a bentonite layer exposed c. 16 km upstream and also within the lower part of Unit 4 yielded an Ar/Ar date of 72.58 ± 0.09 Ma (Bell et al. Reference Bell, Fanti, Currie and Arbour2014). This bentonite layer is roughly coeval or slightly lower in section than Tyrants Aisle, but provides an approximate age for the locality. Unit 4 is a coal-rich deposit composed of overbank, crevasse splay, levee and channel-fill sediments (Fanti & Catuneanu, Reference Fanti and Catuneanu2009) that were deposited at a palaeolatitude of c. 63° N (van Hinsbergen et al. Reference van Hinsbergen, de Groot, van Schaik, Spakman, Bijl, Sluijs, Langereis and Brinkhuis2015). In addition to the didactyl and monodactyl traces described here, other tracks at the Tyrants Aisle locality include those of hadrosaurids, and both large (track length > 40 cm) and small tridactyl theropods. These tracks will be described elsewhere within a broader synthesis of the palaeoecology of the site, although we provide comparative descriptions and discussion of some of these (such as the small tridactyl theropod tracks) that are pertinent for the interpretation of the didactyl tracks.

Fig. 1. Location of deinonychosaur tracks described here from the Tyrants Aisle locality in north-western Alberta. Scale as indicated. Grey shading represents the extent of the Wapiti Formation (after Fanti & Miyashita, Reference Fanti and Miyashita2009).
3. Methods
3.a. Photogrammetry
Tracks were photographed using a Nikon D810 SLR camera with 14–24 mm lens, set to 24 mm for all images. These images were imported into VisualSFM version 0.5.26 (http://www.cs.washington.edu/homes/ccwu/vsfm) to create sparse point cloud reconstructions of each track, before dense point cloud reconstructions were created using CMVS/PMVS (Furukawa & Ponce, Reference Furukawa and Ponce2010; Furukawa et al. Reference Furukawa, Curless, Seitz and Szeliski2010). Meshes were then cropped in MeshLab (version 2016.12; Cignoni et al. Reference Cignoni, Callieri, Corsini, Dellepiane, Ganovelli, Ranzuglia, Scarano and De Chiara2008) and Screened Poisson surface reconstructions of each were produced (Kazhdan & Hoppe, Reference Kazhdan and Hoppe2013). Digital elevation models of the Poisson meshes were created in CloudCompare version 2.9.1 (http://www.cloudcompare.org/), and final colour changes made in Paraview (version 5.5.2; Ahrens et al. Reference Ahrens, Berk, Charles, Hansen and Johnson2005). In accordance with current protocol (Matthews et al. Reference Matthews, Noble, Breithaupt, Falkingham, Marty and Richter2016; Falkingham et al. Reference Falkingham, Bates, Avanzini, Bennett, Bordy, Breithaupt, Castanera, Citton, Díaz-Martínez, Farlow, Fiorillo, Gatesy, Getty, Hatala, Hornung, Hyatt, Klein, Lallensack, Martin, Marty, Matthews, Meyer, Milàn, Minter, Razzolini, Romilio, Salisbury, Sciscio, Tanaka, Wiseman, Xing and Belvedere2018), these digital elevation models were used to better support the track descriptions/interpretations and are available for download in polygon file format (online Supplementary Figs S1–S7, available at http://journals.cambridge.org/geo).
3.b. Measurements
Track orientation and possible stride and pace lengths were measured on-site using a compass and tape measure, respectively. Track length, width and digit divarication were measured digitally in Inkscape version 0.92.3 (https://inkscape.org/) using screen captures of the digital elevation models to more accurately identify track contours.
3.c. Preservation assessment
Quality of track preservation was assessed using the grading system of Belvedere & Farlow (Reference Belvedere, Farlow, Falkingham, Marty and Richter2016) and Marchetti et al. (Reference Marchetti, Belvedere, Voigt, Klein, Castanera, Díaz-Martínez, Marty, Xing, Feola, Melchor and Farlow2019), which allows preservation quality to be quantified and more clearly interpreted and expressed. Each system grades track quality from 0 through to 3, where 0 represents the poorest quality and 3 the highest (for specific grading criteria, see Belvedere & Farlow, Reference Belvedere, Farlow, Falkingham, Marty and Richter2016, table 6.1; Marchetti et al. Reference Marchetti, Belvedere, Voigt, Klein, Castanera, Díaz-Martínez, Marty, Xing, Feola, Melchor and Farlow2019, table 1). These preservation quality designations influenced the track interpretations and trackmaker identifications expressed here.
4. Track descriptions
The possible trackway consists of an unusual series of four consecutive markings on the same bedding plane (Fig. 2a–h; online Supplementary Figs S1–S3). Each of the individual traces (here Di.Tw1.1.27D, Di.Tw1.2.27D, Di.Tw1.3.28D and Di.Tw1.4.29D) are eroded due to modern fluvial activity, although visible claw marks are still present on at least two of the four footprints. The first track in the sequence, Di.Tw1.1.27D (Fig. 2a–c, h, k; online Supplementary Fig. S1), is the only example from this sequence that is unequivocally didactyl (track length, 12.0 cm; track width, 6.9 cm; length/width ratio, 1.74). Two digit impressions are present, united by a shallowly impressed and rounded metatarsophalangeal area that is best visible when the track surface is wet (Fig. 2b). The left digit is shorter and shallower than the right digit and, following the interpretation that this track was produced by a deinonychosaur (see Section 5.d on ichnotaxonomic affinities), this suggests that the former is digit IV and the latter is digit III (i.e. the track was made by a left foot). Divarication between digits III and IV is 32°. The central portion of digit III is the most deeply impressed part of the track (Fig. 2c). Digit III is elliptical in overall shape and terminates in a small, sharp claw mark that is slightly medially in-turned. Digit IV is roughly two-thirds the length of digit III (length of digit IV/III measured from the heel base, 0.68) and terminates in a broad, subcircular impression that lacks any indication of a claw mark. No proximal pad of digit II is present. Overall, the general shape of the entire print remains visible (including distinguishable digit impressions), but because interphalangeal digital pad outlines, skin impressions and a claw mark on digit IV are absent, and the ‘heel’ area is particularly faint, a sub-optimal preservation grade of 1 is assigned to Di.Tw1.1.27D.

Fig. 2. Photographs, digital elevation models and interpretive line drawings of probable deinonychosaur tracks. Di.Tw1.1.27D photographs of (a) dry surface, (b) wet surface and (c) digital elevation model; Di.Tw1.3.28D (d) photograph and (e) digital elevation model; Di.Tw1.4.29D (f) photograph and (g) digital elevation model; (h) map of traces Di.Tw1.1.27D, Di.Tw1.2.27D, Di.Tw1.3.28D, Di.Tw1.4.29D and the tridactyl theropod undertrack Th.Tw3.1.27D, with inferred trackway associations as dashed lines (black – likely association; grey – tentative association); and Di.I.34E (i) photograph and (j) digital elevation model; (k) interpretive line drawings of specimens, scale as indicated. Scale bars for (a–g) and (i–j): 5 cm. Relative depth is indicated in the digital elevation models using colours, where cool colours represent topographic lows and warm colours are topographic highs. Three-dimensional meshes used to create the digital elevation models are available as online Supplementary Figures S1–S4.
The second track in the sequence is a small ovoid depression, Di.Tw1.2.27D (length, 6.5 cm; width, 3.7 cm), located 0.36 m to the SW of Di.Tw1.1.27D (Fig. 2h, k; online Supplementary Fig. S1). This depression is widest towards the posterior end and tapers distally to a blunt termination, although any recognizable digit morphology, such as pad impressions or claw marks, is absent. Accordingly, a poorest preservation grade of 0p is assigned, where ‘p’ indicates partial preservation (after Marchetti et al. Reference Marchetti, Belvedere, Voigt, Klein, Castanera, Díaz-Martínez, Marty, Xing, Feola, Melchor and Farlow2019). The third trace, Di.Tw1.3.28D, is located 1.15 m west of Di.Tw1.1.27D and displays one clear digit impression and possible evidence of a second (Fig. 2d–e, h, k; online Supplementary Fig. S2). The largest digit, presumably digit III, consists of a teardrop-shaped impression (length, 8.0 cm; width, 3.0 cm), tapering distally, lacking any obvious digital pads, and terminating in a prominent, narrow claw mark. A possible short, narrow trace (length, 2.1 cm) near the base and to the left of digit III may represent evidence of an adjacent digit, although it is uncertain if this mark is actually part of the track, as it shows only a slight depth difference to the surrounding substrate from the digital elevation model (Fig. 2e). Based on the single digit III impression that is recognizable, which possesses a prominent claw mark, the preservation grade of Di.Tw1.3.28D is 0.5p.
The final trace within this sequence, Di.Tw1.4.29D (Fig. 2f–h, k; online Supplementary Fig. S3), consists of a tear-drop shaped (tapering proximally) monodactyl impression with rounded margins (length, 9.0 cm; width, 3.2 cm). A possible sharp claw mark is present at the distal end, although this could also be exaggerated by a fracture in the rock. A distance of 0.83 m separates Di.Tw1.3.28D and Di.Tw1.4.29D (Fig. 2h), the latter of which is oriented in a slightly different direction (axis offset from that of Di.Tw1.3.28D by c. 14°). A poorest preservation grade of 0p is assigned to Di.Tw1.4.29D, given that no clear digit morphology is present.
In addition to the possible trackway, at least one other isolated didactyl trace was identified. The additional track, Di.I.34E (track length, 13.0 cm; track width, 7.7 cm; length/width ratio, 1.69), is located on the same bedding plane c. 6.5 m to the SW of Di.Tw1.1.27D (Fig. 2i–k; online Supplementary Fig. S4). Both prints are oriented in the same direction (bearing of 252–254°). However, Di.I.34E is laterally offset from the possible trackway by c. 2.5 m and is therefore unlikely to have been produced by the same individual. Assuming that the trackmaker was a deinonychosaur (see Section 5.d on ichnotaxonomic affinities), Di.I.34E is a left foot based on the relative size difference between the two digit impressions. The proximal portion of digit III is narrower than at its mid-length, with a rounded proximal margin that is deeper than the impression of the ‘heel’ area (Fig. 2i–j). Distally, digit III tapers to a sharp point. Digit IV is arcuate and, in comparison to digit III, much shorter (length of digit IV/III, 0.68–0.6, depending on whether a possible claw mark on digit IV is included), narrower (width of III, 4.5 cm; width of IV, 1.8 cm) and more shallowly impressed (Fig. 2j). Divarication of digits III–IV is 35–38°, dependent on the termination point of digit IV that is used. Interphalanageal pads (aside from the proximal-most part of digit III) are not visible on digits III or IV and there is no indication of digit II. As in Di.Tw1.1.27D, a raised ridge of sediment separates the impression of digit III from IV. The heel is rounded and deeply impressed into the substrate. A fair preservation grade of 1.5 is assigned to Di.I.34E, as the overall track shape is clearly discernible, claw marks are present and at least one interphalangeal pad outline (i.e. at the base of digit III) is visible. When comparing the morphology of Di.Tw1.1.27D and Di.I.34E, they differ in the shape of digit IV; digit IV is rounded in the former, but more narrow and arcuate in the latter. Similarly, the hypex between digits III and IV is located more anteriorly in Di.Tw1.1.27D. Both tracks nevertheless have a broad, robust digit III that terminates in a claw mark, a relatively short digit IV compared to digit III and rounded heel margins, and they are similar in overall track size (Table 2).
Table 2. Track and trackway measurements for prints described here. L – left foot; R – right foot; III – digit III; IV – digit IV. Preservation grade based on Belvedere & Farlow (Reference Belvedere, Farlow, Falkingham, Marty and Richter2016) and Marchetti et al. (Reference Marchetti, Belvedere, Voigt, Klein, Castanera, Díaz-Martínez, Marty, Xing, Feola, Melchor and Farlow2019).

5. Discussion
5.a. Track preservation
Preservation level directly influences the accuracy, reliability and amount of anatomical data that can be obtained from tracks (Belvedere & Farlow, Reference Belvedere, Farlow, Falkingham, Marty and Richter2016; Marchetti et al. Reference Marchetti, Belvedere, Voigt, Klein, Castanera, Díaz-Martínez, Marty, Xing, Feola, Melchor and Farlow2019). There is considerable preservation variation at the Tyrants Aisle locality, with tracks ranging from clearly impressed with well-defined track margins and interphalangeal pads, through to highly eroded depressions representing former footprints, with no recognizable foot morphology remaining. This variation is almost certainly related to the uneven topography of the site and its position within an active riverbed, as the track-bearing layers are generally within the flow of the Redwillow River for much of the year. The most relevant portion of the tracksite for this work (i.e. the immediate area surrounding the described traces) demonstrates only a moderate level of track preservation (Fig. 3). For instance, a larger tridactyl theropod trackway partly overlaps the described didactyl sequence, and shows considerable intratrackway morphological variation (Fig. 3a–c, e). The first track from this sequence, Th.Tw3.1.27D, occurs less than half a metre from Di.Tw1.1.27D and Di.Tw1.2.27D (Fig. 3a–c). Th.Tw3.1.27D is preserved as a natural mould with well-defined track margins, but the track is shallow, no interphalangeal pad margins are discernible, and the overall shape of the print is highly irregular. The heel margin is almost straight, which makes the track very short relative to its width, and digit II is rounded, whereas digits III and IV are more pointed (Fig. 3b–c). In comparison, the fourth track from this sequence, Th.Tw3.4.24H, is preserved as an in situ natural cast (positive epirelief) that has been flattened by erosion, but the heel is more typically V-shaped (Fig. 3e), rather than straight as in Th.Tw3.1.27D. Although the natural cast preservation of Th.Tw3.4.24H differs from the preceding tracks in this sequence, which are preserved as natural moulds (Fig. 3a–c), a collective trackway association is inferred based on similar orientation, alignment and track spacing and a lack of evidence for additional mid-sized theropod trackmakers within this immediate vicinity (Fig. 3a). No interphalangeal pads are visible in Th.Tw3.4.24H. An isolated hadrosaurid track just over 1 m from Di.Tw1.1.27D (Fig. 3a, d) reveals the overall shape of the foot and short, rounded digits, but the print is considerably eroded and possesses short track walls, with no defined interphalangeal pad outlines. Considering the shallow preservation of the tracks within this area, the general lack of visible interphalangeal pads, absence of skin impressions and sometimes irregular foot morphologies, it is likely that these tracks (and the described didactyl tracks) represent eroded, shallow undertracks rather than ‘true’ tracks (sensu Milán & Bromley, Reference Milán and Bromley2006). Accordingly, the morphology of the described didactyl traces is treated cautiously, as undertracks often provide a poorer representation of the pedal anatomy of the trackmaker than true tracks (e.g. Milàn & Bromley, Reference Milán and Bromley2008, fig. 5).

Fig. 3. Tracks surrounding the possible didactyl trackway (in blue). (a) Outline map redrawn from field sketches. D – depression, question marks (?) – uncertain traces, probable trackway associations – black, long-dashed lines; less certain trackway associations – grey, short-dashed lines. Scale as indicated. (b) Photograph and (c) digital elevation model of theropod undertrack Th.Tw3.1.27D alongside didactyl traces Di.Tw1.1.27D and Di.Tw1.2.27D. Scale bar: 10 cm. (d) Photograph of hadrosaurid undertrack H.I.26D, which is naturally filled with water. Each grid square internal division represents 10 cm2. (e) Photograph of theropod track Th.Tw3.4.24H, preserved as an in situ natural cast. Image contrast, saturation and brightness have been adjusted for improved underwater visibility. Scale bar: 10 cm.
5.b. Comparisons with associated tridactyl theropod tracks
It is important to compare the morphology and preservation of the described didactyl traces with similarly sized tridactyl theropod tracks on the same layer, as it is possible that differences in digit counts may simply reflect preservational, substrate or kinematic-driven differences rather than anatomical difference. The clearest and most size-equivalent tridactyl theropod tracks on the same layer at Tyrants Aisle measure c. 18 cm in length and 13–18 cm in width (Fig. 4; online Supplementary Figs S5–S7). As for the described didactyl traces, these tracks are also heavily eroded; however, some evidence of all three digit impressions is consistently retained. In contrast, Di.Tw1.1.27D and Di.I.34E show no evidence of a third digit impression (Fig. 2). In particular, Di.I.34E is deeply impressed, making it unlikely that a third weight-bearing toe (if present) would fail to register on the substrate, as can sometimes occur in extant ‘pseudodidactyl’ bird tracks (Milán, Reference Milán2006, fig. 12C; Richter & Böhme, Reference Richter, Böhme, Falkingham, Marty and Richter2016, fig. 17.7). Where this phenomenon of pseudodidactyly was observed in extant birds, the substrate was firm and damp, and tracks were only shallowly impressed.

Fig. 4. Small, tridactyl theropod tracks from the same track layer as the described didactyl traces, shown here to demonstrate their morphological differences. Th.Tw5.3.72-A (a) digital elevation model and (b) line drawing; Th.Tw2.2.9C (c) digital elevation model and (d) line drawing; and Th.Tw4.4.70-A (e) digital elevation model and (f) line drawing. Dashed black lines indicate poorly visible track margins. All scales as indicated. Three-dimensional meshes used to create the digital elevation models are available as online Supplementary Figures S5–S7.
Digit III morphology in the small tridactyl theropod tracks at Tyrants Aisle also differs from that of Di.Tw1.1.27D and Di.I.34E. In the small tridactyl tracks, digit III is narrow, and the base of its free length is consistently the widest portion of that digit, which then tapers continuously towards the tip (Fig. 4). In contrast, digit III in both Di.Tw1.1.27D and Di.I.34E is more elliptical and the widest point occurs near the midpoint of its free length (Fig. 2). As previously discussed, Di.Tw1.1.27D and Di.I.34E likely represent shallow undertracks, so the possibility that this distinct digit III morphology is a preservation artefact cannot be ruled out. Nevertheless, given that some of the morphologically distinct tridactyl theropod tracks also appear to represent eroded undertracks, we suggest that digit III shape remains a reliable distinction between the two track types. We therefore do not consider a tridactyl trackmaker for Di.Tw1.1.27D and Di.I.34E to be the most likely scenario, and instead interpret that they were formed by a functionally didactyl trackmaker.
5.c. Monodactyly and trackway interpretations
The didactyl track Di.Tw1.1.27D occurs in possible trackway association with three monodactyl impressions: Di.Tw1.2.27D, Di.Tw1.3.28D and Di.Tw1.4.29D (Fig. 2h). A similar phenomenon occurs within four parallel trackways from the Lower Cretaceous Houmotuan tracksite of Shandong Province, China, where recognizably didactyl tracks become monodactyl impressions within the same trackways (Xing et al. Reference Xing, Lockley, Zhang, Klein, Persons, Romilio, Tang, Guo and Wang2018 b, fig. 5). The didactyl tracks within these sequences were used to infer a deinonychosaur maker for the trackways, as no non-avian or avian dinosaur is currently known to possess a monodactyl pes (Xing et al. Reference Xing, Lockley, Zhang, Klein, Persons, Romilio, Tang, Guo and Wang2018 b). However, the recent description of Vespersaurus from the early Late Cretaceous Epoch of Brazil indicates that at least some theropods may have been functionally monodactyl (Langer et al. Reference Langer, de Oliveira Martins, Manzig, de Souza Ferreira, de Almeida Marsola, Fortes, Lima, Sant’ana, da Silva Vidal, da Silva Lorençato and Ezcurra2019). It was speculated by Xing et al. (Reference Xing, Lockley, Zhang, Klein, Persons, Romilio, Tang, Guo and Wang2018 b) that the monodactyl impressions at the Houmotuan tracksite may have been produced if both digits III and IV were held together closely enough to produce a single depression on the substrate. However, the widths of the monodactyl traces at Tyrants Aisle are no greater than the width of digit III on its own in the two didactyl traces (Table 2; Fig. 2). We instead hypothesize that the monodactyl impressions at Tyrants Aisle (Di.Tw1.2.27D, Di.Tw1.3.28D and Di.Tw1.4.29D) represent more highly eroded, deeper undertracks than the didactyl traces, as only the most deeply impressed portion of the foot remains (i.e. the impression of digit III). This is supported by the fact that digit III is the most deeply impressed area of both Di.Tw1.1.27D and Di.I.34E (Fig. 2c, j). Digit III would therefore presumably deform the sediment at a greater depth than any other portion of the foot, and should also be the last part of the track to disappear from ongoing modern fluvial erosion. Experimental work with modern emu tracks has demonstrated a similar phenomenon, as the impression of digit III is all that remains beyond a certain depth, creating an apparently monodactyl track (Milán & Bromley, Reference Milán and Bromley2006, fig. 1H, I).
Monodactyl tracks could also potentially represent a swimming sequence, where the trackmaker was either fully or partially buoyant and did not consistently touch its entire foot onto the substrate. In general, the criteria used to identify swim traces are absent from the Tyrants Aisle specimens, which include the presence of raised sediment mounds at the posterior end of the track, digit reflectures resulting from directional changes within a single foot placement, kick-off scours and long claw drag marks that (in theropods) typically consist of parallel sets of one to three sinuous scratches (McAllister Reference McAllister, Gillette and Lockley1989; Milner & Lockley, Reference Milner, Lockley, Falkingham, Marty and Richter2016). Furthermore, in both Di.Tw1.1.27D and Di.I.34E, the entire foot appears to have contacted the sediment due to the presence of a heel impression and a normal weight-bearing stance is implied. We therefore conclude that a more deeply impressed digit III, in conjunction with greater erosion, is the most likely scenario for the formation of our monodactyl traces.
Close proximity and similar size, shape and general alignment provide the main basis for grouping Di.Tw1.1.27D, Di.Tw1.2.27D, Di.Tw1.3.28D and Di.Tw1.4.29D as traces potentially made by the same trackmaker, although the overall placement and spacing of each trace relative to each another is abnormal. As Di.Tw1.1.27D is interpreted as a left foot, the next right footfall would be expected to occur in front of and to the right of this track. However, the monodactyl impression Di.Tw1.2.27D instead occurs in front of and to the left of Di.Tw1.1.27D (Fig. 2h). This creates a problem if the trackmaker is then inferred to have produced Di.Tw1.3.28D as its third footfall. Di.Tw1.3.28D is considerably further away (0.85 m from Di.Tw1.2.27D), and once again on the wrong side of the trackway midline if Di.Tw1.2.27D was indeed created by a right foot (Fig. 2h). Changes in relative foot position (i.e. a pace angulation exceeding 180°) have been observed in modern ratites and the trackways of some non-avian theropods, which likely relates to the hindlimbs being positioned closely together and/or discontinuous locomotion, where the trackmaker briefly stopped in midstride (Breithaupt et al. Reference Breithaupt, Southwell and Matthews2006, Reference Breithaupt, Matthews, Green and Belvedere2019). In addition to the apparent crossover gait observed for the described Wapiti Formation tracks, a similar phenomenon is observable within the didactyl and monodactyl trackway T23 from the Houmotuan site (Xing et al. Reference Xing, Lockley, Zhang, Klein, Persons, Romilio, Tang, Guo and Wang2018 b, fig. 5). However, given the suboptimal undertrack preservation of Di.Tw1.1.27D, Di.Tw1.2.27D, Di.Tw1.3.28D and Di.Tw1.4.29D, and the abnormal spacing between each footfall (Fig. 2h; Table 2), it is likely that the apparent crossover gait observed here is partly explained by an absence of additional tracks (e.g. between Di.Tw1.2.27D and Di.Tw1.3.28D). It is also possible that the four traces correspond to at least two different individuals moving in close proximity, as was the case at the Houmotuan tracksite (Xing et al. Reference Xing, Lockley, Zhang, Klein, Persons, Romilio, Tang, Guo and Wang2018 b, fig. 5). Ultimately, the abnormal track positioning, poor preservation and probable absence of additional footprints precludes a confident interpretation of the Wapiti Formation sequence described here, and we therefore acknowledge that the collective trackway association of each trace is tentative rather than conclusive.
5.d. Ichnotaxonomic affinities
Small, didactyl tracks within dinosaur-bearing strata are most often referred to members of Deinonychosauria, as these dinosaurs are suitably sized, possess a functionally didactyl pes and have a body fossil record that spans Laurasia and Gondwana (Makovicky & Norell, Reference Makovicky, Norell, Weishampel, Dodson and Osmólska2004; Turner et al. Reference Turner, Makovicky and Norell2012). As Di.Tw1.1.27D and Di.I.34E are hypothesized to pertain to functionally didactyl trackmakers, and the presence of deinonychosaurs within Unit 4 of the Wapiti Formation has been confirmed by body fossils (Fanti et al. Reference Fanti, Currie and Burns2015), we mirror the rationale of Xing et al. (Reference Xing, Lockley, Tang, Romilio, Xu, Li, Tang and Li2018 a) and suggest that a deinonychosaur was probably responsible for producing the didactyl and monodactyl tracks described here. The aforementioned Vespersaurus – which may have been functionally monodactyl – belongs to the subfamily Noasaurinae, whose members are currently identified only from Gondwanan continents (Langer et al. Reference Langer, de Oliveira Martins, Manzig, de Souza Ferreira, de Almeida Marsola, Fortes, Lima, Sant’ana, da Silva Vidal, da Silva Lorençato and Ezcurra2019; Brougham et al. Reference Brougham, Smith and Bell2020) and are therefore unlikely to be responsible for producing the monodactyl traces described here.
Tracks that have been attributed to deinonychosaurs are assigned to five ichnogenera: Velociraptorichnus, Dromaeopodus, Dromaeosauripus, Menglongipus and Sarmientichnus (Lockley et al. Reference Lockley, Harris, Li, Xing, van der Lubbe, Falkingham, Marty and Richter2016 a; Xing et al. Reference Xing, Lockley, Tang, Romilio, Xu, Li, Tang and Li2018 a). The Middle Jurassic type material of Sarmientichnus (Casamiquela, Reference Casamiquela1964; De Valais, Reference De Valais2011) possibly pre-dates the first definitive deinonychosaurs identified from skeletal remains, and it therefore remains an open question whether the maker of these Middle Jurassic traces was a true deinonychosaur. Velociraptorichnus, Dromaeopodus and Dromaeosauripus have generally been treated as probable dromaeosaurid ichnotaxa in prior literature based on their usually subequal lengths of digits III and IV, following osteological observations that these two digits are typically of similar length (Lockley et al. Reference Lockley, Harris, Li, Xing, van der Lubbe, Falkingham, Marty and Richter2016 a). Troodontid pedal skeletons, by comparison, tend to possess a relatively shorter digit IV (van der Lubbe et al. Reference van der Lubbe, Richter and Böhme2009, Reference van der Lubbe, Richter, Böhme, Sullivan, Hübner, Richter and Reich2011; Lockley et al. Reference Lockley, Harris, Li, Xing, van der Lubbe, Falkingham, Marty and Richter2016 a). This distinction in digit IV length was the main criterion used to identify Menglongipus as a possible, albeit dubious, troodontid ichnotaxon (Lockley et al. Reference Lockley, Harris, Li, Xing, van der Lubbe, Falkingham, Marty and Richter2016 a). Similarly, a large collection of more than 86 unnamed didactyl tracks from at least 17 distinct trackways at the Obernkirchen track locality in Germany have been treated as the only conclusive examples of troodontid tracks based on a repeatedly shorter digit IV that is roughly 0.8 times the length of digit III (van der Lubbe et al. Reference van der Lubbe, Richter and Böhme2009, Reference van der Lubbe, Richter, Böhme, Sullivan, Hübner, Richter and Reich2011; Lockley et al. Reference Lockley, Harris, Li, Xing, van der Lubbe, Falkingham, Marty and Richter2016 a; Richter & Böhme, Reference Richter, Böhme, Falkingham, Marty and Richter2016). However, we note that the Velociraptorichnus sichuanensis holotype CFEC-B-1 possesses a digit IV/III length ratio of 0.84 (measured from the heel base; Lockley et al. Reference Lockley, Harris, Li, Xing, van der Lubbe, Falkingham, Marty and Richter2016 a, fig. 11.4A), very similar to that of the Obernkirchen tracks, and therefore agree that a troodontid maker for V. sichuanensis cannot be ruled out (Lockley et al. Reference Lockley, Harris, Li, Xing, van der Lubbe, Falkingham, Marty and Richter2016 a).
The Obernkirchen didactyl tracks differ most notably from those from the Wapiti Formation described here in the shape of digit III: those from Tyrants Aisle are elliptical and broad, whereas the German tracks possess narrow digit III impressions (Fig. 5f). In addition, digit IV is relatively shorter in the Wapiti specimens (IV/III length ratio of 0.8 for the German tracks; 0.68–0.6 for Di.Tw1.1.27D and Di.I.34E) and the German specimens are also larger in overall size (track lengths from the Obernkirchen locality are typically > 20 cm, with a range of 13–23.3 cm; Lockley et al. Reference Lockley, Harris, Li, Xing, van der Lubbe, Falkingham, Marty and Richter2016 a). As a result, these tracks likely pertained to distinct trackmakers.

Fig. 5. Comparison of tracks described here with other select deinonychosaur tracks: (a) Di.Tw1.1.27D, with superimposed pedal skeleton of a troodontid (Talos sampsoni; adapted from Zanno et al. Reference Zanno, Varricchio, O’Connor, Titus and Knell2011); (b) Di.I.34E, with possible digit IV claw mark in grey; (c) cf. Menglongipus track HMT-T22-R6 from the Houmotuan tracksite in Shandong Province, China (reversed and redrawn from Xing et al. Reference Xing, Lockley, Zhang, Klein, Persons, Romilio, Tang, Guo and Wang2018 b, fig. 5); (d) Menglongipus track DMNH 21365 from Riley Creek, Denali National Park, Alaska, USA (after Fiorillo et al. Reference Fiorillo, Contessi, Kobayashi, McCarthy, Lockley and Lucas2014, fig. 7, excluding a possible trace of the proximal end of digit II); (e) track T.A.1 of the Menglongipus sinensis holotype trackway from Hebei Province, China (after Xing et al. Reference Xing, Harris, Sun and Zhao2009 a); (f) troodontid track A2 from the Obernkirchen track locality in Germany (after Lockley et al. Reference Lockley, Harris, Li, Xing, van der Lubbe, Falkingham, Marty and Richter2016 a, fig. 11.13); (g) Velociraptorichnus track from Shandong Province, China (after Li et al. Reference Li, Lockley, Makovicky, Matsukawa, Norell, Harris and Liu2008, fig. 2); (h) representative print from the Dromaeopodus shangdongensis holotype trackway CU 214.111, from Shandong Province, China (after Li et al. Reference Li, Lockley, Makovicky, Matsukawa, Norell, Harris and Liu2008, fig. 2); and (i) Dromaeosauripus track from the Mill Canyon Dinosaur Tracksite, Utah, USA (adapted from Lockley et al. Reference Lockley, Gierliński, Houck, Lim, Kim, Kim, Kim, Kang, Hunt Foster, Li, Chesser, Gay, Dubicka, Cart and Wright2014 b, fig. 6). Dashed lines denote the approximate location of a digit II proximal pad impression (when present). Scales as indicated.
The Wapiti Formation tracks differ from Chinese Velociraptorichnus sichuanensis prints in lacking a proximal pad impression of digit II and having a notably shorter digit IV (IV/III length ratio for the V. sichuanensis holotype, 0.84). Nevertheless, similarities include overall track size, where V. sichuanensis holotype and paratype tracks vary over the range 11–11.5 cm in length and 6–6.5 cm in width (Zhen et al. Reference Zhen, Li, Zhang, Chen and Zhu1994; see Table 2 for Wapiti track measurements), as well as relatively broad digit III impressions and a rounded heel. Additional tracks referred to Velociraptorichnus from China also display a number of similarities. One well-preserved specimen from Shandong Province (Fig. 5g; Li et al. Reference Li, Lockley, Makovicky, Matsukawa, Norell, Harris and Liu2008, fig. 2a, b) has a comparably rounded heel and an elliptical, broad digit III impression that is particularly reminiscent of that seen in Di.Tw1.1.27D and the monodactyl trace Di.Tw1.3.28D. However, this Chinese track differs from the Wapiti specimens in the relative length of digits III and IV, which are subequal, and by possessing a proximal pad impression of digit II. Another example from Sichuan Province (MJW-T2-R1; Xing et al. Reference Xing, Lockley, Yang, Xu, Cao, Klein, Persons, Shen and Zheng2015 a, fig. 3) possesses a relatively short digit IV comparable to that of Di.Tw1.1.27D and Di.I.34E, although its preceding track MJW-T2-L1 and others on the same bedding plane instead show subequal digit lengths, suggesting that the shortness of digit IV in MJW-T2-R1 may be a preservation artefact. These particular tracks, assigned to the ichnospecies Velociraptorichnus zhangi, also lack the broad digit impressions of the Wapiti specimens and represent a tridactyl deinonychosaur morphotype where digit II is fully impressed, either due to particular substrate conditions or a suitable amount of claw retraction (Xing et al. Reference Xing, Lockley, Yang, Xu, Cao, Klein, Persons, Shen and Zheng2015 a). The Wapiti tracks lack any such trace of digit II. We ultimately reject the referral of Di.Tw1.1.27D and Di.I.34E to Velociraptorichnus based on greater relative shortness of digit IV compared with III and the absence of a digit II proximal pad impression.
Dromaeopodus shandongensis was named by Li et al. (Reference Li, Lockley, Makovicky, Matsukawa, Norell, Harris and Liu2008) on the basis of well-preserved trackways of Early Cretaceous age in Shandong Province, China. These tracks display a proximal pad impression of digit II, subequal digit III and IV lengths, and are larger than the Wapiti specimens (length, 24–28.5 cm; Li et al. Reference Li, Lockley, Makovicky, Matsukawa, Norell, Harris and Liu2008, table 1). Additionally, digits III and IV of D. shandongensis are relatively narrow and both curve medially (Fig. 5h), while those of Di.Tw1.1.27D and Di.I.34E are either straight or, in the case of digit IV from Di.I.34E, curve laterally. A well-rounded heel impression that unites the proximal portions of digits III and IV is the only notable similarity between the two track morphotypes; we therefore reject assignment of the Wapiti tracks to D. shandongensis.
Three ichnospecies of Dromaeosauripus are known from the Early Cretaceous Epoch. These are Dromaeosauripus hamanensis and Dromaeosauripus jinjuensis from South Korea (Kim et al. Reference Kim, Kim, Lockley, Yang, Seo and Choi2008, Reference Kim, Lockley, Woo and Kim2012), and Dromaeosauripus yongjingensis from China (Xing et al. Reference Xing, Li, Harris, Bell, Azuma, Fujita, Lee and Currie2013 a). Additional tracks referred to Dromaeosauripus are known from both Utah and Colorado (Lockley et al. Reference Lockley, Gierliński, Dubicka, Breithaupt and Matthews2014 a, b, Reference Lockley, Xing, Matthews and Breithaupt2016 b). All occurrences of Dromaeosauripus are characterized by narrow digit impressions that are subequal in length, which differ from the broader, unequal-length digits seen in Di.Tw1.1.27D and Di.I.34E. Dromaeosauripus tracks may also show a proximal pad impression of digit II, the presence of which is variable within a single trackway (Lockley et al. Reference Lockley, Huh and Kim2012; Xing et al. Reference Xing, Li, Harris, Bell, Azuma, Fujita, Lee and Currie2013 a). In Dromaeosauripus jinjuensis, the proximal pad of digit II is missing, while digits III and IV are represented by completely separate and parallel impressions, with no evidence of the heel pad (Kim et al. Reference Kim, Lockley, Woo and Kim2012). The separated toes and absence of a heel impression seen in Dromaeosauripus jinjuensis, as well as certain examples of both Dromaeosauripus hamanensis and Dromaeosauripus yongjingensis (Kim et al. Reference Kim, Kim, Lockley, Yang, Seo and Choi2008; Xing et al. Reference Xing, Li, Harris, Bell, Azuma, Fujita, Lee and Currie2013 a), is distinct from the rounded heel pad that unites digits III and IV in the Wapiti Formation tracks. In addition, both the Chinese and North American tracks assigned to Dromaeosauripus were produced by slender-toed trackmakers (Fig. 5i), while the didactyl tracks from the Wapiti Formation possess a more robust, broader digit morphology. Finally, digit IV in the Wapiti tracks is also notably shorter than in any of the three Dromaeosauripus ichnospecies. Collectively, these differences indicate that the Wapiti specimens cannot be referred to Dromaeosauripus.
The most comparable deinonychosaurian ichnotaxon to those from Tyrants Aisle, especially in the relative shortness of digit IV, is Menglongipus sinensis (Fig. 5e), an ichnospecies that was erected based on a trackway of four poorly preserved didactyl moulds (T.A.1–4; Xing et al. Reference Xing, Harris, Sun and Zhao2009 a) from the latest Jurassic or earliest Cretaceous period of Hebei Province, China. Average digit IV/III length of these prints is 0.62 (measured from heel to digit tip; Xing et al. Reference Xing, Harris, Sun and Zhao2009 a), consistent with ratios obtained for Di.Tw1.1.27D and Di.I.34E (Table 2). However, the overall size of each complete M. sinensis print is only 5.8–6.7 cm in length, suggestive of a smaller-bodied trackmaker. Additionally, three of the four M. sinensis holotype tracks show a proximal pad impression of digit II (Xing et al. Reference Xing, Harris, Sun and Zhao2009 a), a feature that is missing – albeit possibly due to erosion – from the Wapiti Formation tracks. Further occurrences of Menglongipus have since been reported from other localities both within and outside China. From the lower Cantwell Formation of Riley Creek, within Denali National Park in Alaska (upper Campanian – lower Maastrichtian), an isolated didactyl track, DMNH 21365, was referred to Menglongipus based on the relative shortness of digit IV in comparison to digit III (Fiorillo et al. Reference Fiorillo, Contessi, Kobayashi, McCarthy, Lockley and Lucas2014; Fig. 5d). This track is the most temporally and geographically comparable specimen to those from Tyrants Aisle and, as for the Tyrants Aisle specimens, the shortness of digit IV in DMNH 21365 suggests that it probably pertains to a troodontid rather than a dromaeosaurid (see the following section on referral to Troodontidae). However, DMNH 21365 differs from the Wapiti Formation tracks in possessing relatively narrower and less-rounded digits, especially that of digit III. The previously discussed collection of four parallel trackways containing didactyl and monodactyl tracks at the Houmotuan tracksite from the Dasheng Group of Shandong Province in China have also been tentatively referred to cf. Menglongipus (Xing et al. Reference Xing, Lockley, Zhang, Klein, Persons, Romilio, Tang, Guo and Wang2018 b; Fig. 5c). These Houmotuan tracks display a striking similarity to the Wapiti specimens in both the relative shortness of digit IV compared to digit III (IV/III length ratio for T22-R6 is 0.56; Xing et al. Reference Xing, Lockley, Zhang, Klein, Persons, Romilio, Tang, Guo and Wang2018 b, fig. 5; Fig. 5c) and also the broad, robust morphology of digit III in certain track examples (e.g. T22-L6; Xing et al. Reference Xing, Lockley, Zhang, Klein, Persons, Romilio, Tang, Guo and Wang2018 b, fig. 5). Furthermore, the presence of teardrop-shaped monodactyl impressions (Xing et al. Reference Xing, Lockley, Zhang, Klein, Persons, Romilio, Tang, Guo and Wang2018 b, fig. 5) in association with these didactyl tracks matches the co-occurrence of didactyl and similarly shaped monodactyl impressions observed at Tyrants Aisle. These cf. Menglongipus tracks from Shandong are therefore the most morphologically comparable tracks to our described specimens. However, we conservatively refrain from referring the Wapiti Formation tracks to Menglongipus due to the suboptimal preservation of the Wapiti tracks themselves, and the poor quality of the holotype trackway used to erect Menglongipus sinensis (Xing et al. Reference Xing, Harris, Sun and Zhao2009 a), which is therefore a potentially dubious ichnotaxon (Lockley et al. Reference Lockley, Harris, Li, Xing, van der Lubbe, Falkingham, Marty and Richter2016 a).
5.e. Referral to Troodontidae
Within Deinonychosauria, the relative digit proportions of the didactyl tracks at Tyrants Aisle are most consistent with that of a troodontid. Troodontids generally possess a digit IV that is up to 20% shorter than digit III and less anteriorly projected, whereas these two digits tend to be of subequal length in dromaeosaurids (van der Lubbe et al. Reference van der Lubbe, Richter, Böhme, Sullivan, Hübner, Richter and Reich2011; Lockley et al. Reference Lockley, Harris, Li, Xing, van der Lubbe, Falkingham, Marty and Richter2016 a). Among North American troodontids, complete pedes are known for Stenonychosaurus inequalis from the upper Campanian Dinosaur Park Formation of southern Alberta, Canada, (CMN 8537; Sternberg, Reference Sternberg1932) and Talos sampsoni from the upper Campanian Kaiparowits Formation of Utah, USA (UMNH VP 19479; Zanno et al. Reference Zanno, Varricchio, O’Connor, Titus and Knell2011), both of which have a digit IV/III length ratio of 0.8. Superimposing the foot skeleton of T. sampsoni onto Di.Tw1.1.27D results in an imperfect fit (Fig. 5a), as the relative digit IV length in the latter (and also Di.I.34E) is shorter than that of T. sampsoni. Nevertheless, a member of Troodontidae remains the most plausible trackmaker for these prints given the smaller discrepancy in relative digit IV length when compared to a typical dromaeosaurid. Within North America, isolated troodontid teeth commonly occur at many Campanian–Maastrichtian microvertebrate sites, although skeletal material is relatively rare and our understanding of their taxonomic diversity remains contentious (Evans et al. Reference Evans, Cullen, Larson and Rego2017; van der Reest & Currie, Reference van der Reest and Currie2017). From the Wapiti Formation specifically, troodontids are so far represented only by an isolated metatarsal, TMP 89.55.1008, and teeth referred to Troodon (Ryan & Russell, Reference Ryan, Russell, Tanke and Carpenter2001; Fanti & Miyashita, Reference Fanti and Miyashita2009; Fanti et al. Reference Fanti, Currie and Burns2015). Troodon teeth, along with those of indeterminate dromaeosaurids, were recovered within Unit 4 at the Wapiti River Pachyrhinosaurus bonebed (Fanti et al. Reference Fanti, Currie and Burns2015), confirming the presence of both deinonychosaur families within the same stratigraphic unit as the tracks described here. Additional Troodon teeth are known from close to the Unit 3–Unit 4 boundary, within the Pipestone Creek Pachyrhinosaurus bonebed (Currie et al. Reference Currie, Langston and Tanke2008), as well as at the Kleskun Hill microsites within Unit 3, where they are the most abundant small theropod tooth type (Fanti & Miyashita, Reference Fanti and Miyashita2009). The dromaeosaurids Boreonykus certekorum and teeth referred to Saurornitholestes sp. (including ‘Paronychodon’-type teeth; see Currie & Evans Reference Currie and Evans2020) and Richardoestesia are also known from Unit 3 (Currie et al. Reference Currie, Langston and Tanke2008; Fanti & Miyashita, Reference Fanti and Miyashita2009; Bell & Currie, Reference Bell and Currie2015). The discovery of probable troodontid footprints within the Wapiti Formation, although novel, is therefore not an unexpected find and further adds to a growing non-avian dinosaur ichnodiversity, which also includes hadrosaurids, tyrannosaurids, possible oviraptorosaurs, indeterminate tridactyl theropods and scarce ankylosaur and/or ceratopsian tracks (Fanti et al. Reference Fanti, Bell and Sissons2013; McCrea et al. Reference McCrea, Buckley, Plint, Currie, Haggart, Helm, Pemberton, Lockley and Lucas2014).
5.f. Importance within the fossil record of Deinonychosauria
While skeletal material and/or teeth from deinonychosaurs have been found on all seven continents (Makovicky & Norell, Reference Makovicky, Norell, Weishampel, Dodson and Osmólska2004; Norell & Makovicky, Reference Norell, Makovicky, Weishampel, Dodson and Osmólska2004; Weishampel et al. Reference Weishampel, Barrett, Coria, Loeuff, Xing, Xijin, Sahni, Gomani, Noto, Weishampel, Dodson and Osmólska2004; Turner et al. Reference Turner, Makovicky and Norell2012), their track record is currently limited to North America, South America, Europe and Asia (Fig. 6). In particular, the majority of track occurrences are from the Early Cretaceous Epoch of China (Table 1).

Fig. 6. Temporal comparison of deinonychosaur tracks and body fossils for continents where deinonychosaur tracks have been reported. Body fossils are coloured with black bars and tracks with red bars. Thinner grey bars indicate a possible occurrence, either due to inconclusive taxonomy or poorly resolved dating of host strata. An asterisk (*) denotes the temporal position of the presently described tracks. Dromaeosaurid silhouette copyright of Emily Willoughby, available under Creative Commons licence (CC BY-SA 3.0). Track silhouette adapted from Lockley et al. (Reference Lockley, Harris, Li, Xing, van der Lubbe, Falkingham, Marty and Richter2016 a, fig. 11.4.A).
Deinonychosaur tracks from North America were first identified from Aptian–Albian strata in eastern Utah, within the Ruby Ranch Member of the Cedar Mountain Formation at Arches National Park (White & Lockley, Reference White and Lockley2002; Lockley et al. Reference Lockley, White, Kirkland and Santucci2004) and at the nearby Mill Canyon Dinosaur Tracksite (Cowan et al. Reference Cowan, Lockley and Gierliński2010). The Mill Canyon tracks are referred to the ichnogenus Dromaeosauripus on the basis of their slender-toed morphology and the presence of a slight impression of the proximal portion of digit II (Fig. 5i; Lockley et al. Reference Lockley, Gierliński, Dubicka, Breithaupt and Matthews2014 a, b). Dromaeosauripus tracks have also been identified from the Albian Plainview Sandstone of Dinosaur Ridge in Colorado (Lockley et al. Reference Lockley, Harris, Li, Xing, van der Lubbe, Falkingham, Marty and Richter2016 a, b), and a single track referred to Menglongipus is known from the Late Cretaceous Epoch of Alaska within Denali National Park (Fiorillo et al. Reference Fiorillo, Contessi, Kobayashi, McCarthy, Lockley and Lucas2014). Other reports of North American deinonychosaur tracks are more dubious. Alleged Late Cretaceous dromaeosaurid tracks from El Aguaje in Michoacán, Mexico have been reported (JA Ortíz-Mendieta, Dissertation, Universidad Nacional Autónoma de México, 2001), although these require re-evaluation as they may represent a superficially didactyl variation of a tridactyl track morphotype (Rodríguez-de la Rosa et al. Reference Rodríguez-de la Rosa, Aguillón-Martínez, López-Espinoza and Eberth2004). Similarly, an alleged large dromaeosaurid trackway from near the Las Águilas tracksite in Coahuilia, Mexico (Meyer et al. Reference Meyer, Eberhard, Thüering and Krempaská2008) is regarded as a poorly preserved ornithopod sequence by some workers (Bravo-Cuevas & Rodríguez-de la Rosa, Reference Bravo-Cuevas, Rodríguez-de la Rosa, Rivera-Sylva, Carpenter and Frey2014). Additional deinonychosaur tracks from Las Águilas have since been reported (Rivera-Sylva et al. Reference Rivera-Sylva, Frey, Schulp, Meyer, Thuring, Stinnesbeck and Vanhecke2017), but have not been figured or described. North American deinonychosaur ichnodiversity is therefore restricted to only two definitive morphotypes, comprising three occurrences of Dromaeosauripus from the Early Cretaceous Epoch of Utah and Colorado, and one occurrence of Menglongipus from the Late Cretaceous Epoch of Alaska. We add to this record probable troodontid prints described here from the Late Cretaceous Epoch of Canada, which establishes the presence of at least three distinct deinonychosaur track morphotypes from North America. These findings demonstrate that there is considerable scope for further increasing the deinonychosaur ichnodiversity from North America, as has occurred in Asia, through ongoing exploration of dinosaur track-bearing strata in the coming years.
Conflict of interest
None.
Acknowledgements
We thank RT McCrea and LG Buckley for their on-site assistance with track documentation; D Larson for providing field equipment belonging to the Philip J Currie Dinosaur Museum; G Behuniak, C Coy, S Kagan and W Yan-yin for helping with on-site track documentation during the Boreal Alberta Dinosaur Project (BADP) 2018 field season; and the Grande Prairie Regional College (GPRC) for providing lodging and vehicle use during BADP fieldwork. We would also like to thank an anonymous reviewer for their constructive comments on the manuscript, Bas Van de Schootbrugge and Susie Cox (handling editors) and Elaine Rowan (copy-editor). This work was supported by: an RTP scholarship from the Australian Government, provided to NJ Enriquez; funds given by the River of Death and Discovery Dinosaur Museum Society in support of the Philip J Currie Professorship in Vertebrate Palaeontology at the University of Alberta, Canada; a Natural Sciences and Engineering Research Council of Canada Discovery Grant (RGPIN-2017-06246) and start-up funding awarded by the University of Alberta to C Sullivan; and grants given to NE Campione and MJ Vavrek by the Dinosaur Research Institute, Alberta, Canada.
Supplementary material
To view supplementary material for this article, please visit https://doi.org/10.1017/S0016756820001247