INTRODUCTION
The Sierra de Atapuerca is a great archaeo-palaeontological site with prehistoric occupations that have dates ranging from 1.3 Ma (Carbonell et al, Reference Carbonell, Bermúdez de Castro, Parés, Pérez-González, Cuenca-Bescós, Ollé and Mosquera2008) to the Holocene. Three caves, Gran Dolina, Galería, and Sima del Elefante, were dug systematically in the Railway Trench. All three were filled with sediment that has been dated to the end of the middle Pleistocene (Figs. 1 and 2). Because of the age of the deposits, no evidence of Neanderthal occupation has been found in these caves to complete the sequence in the Sierra de Atapuerca record.
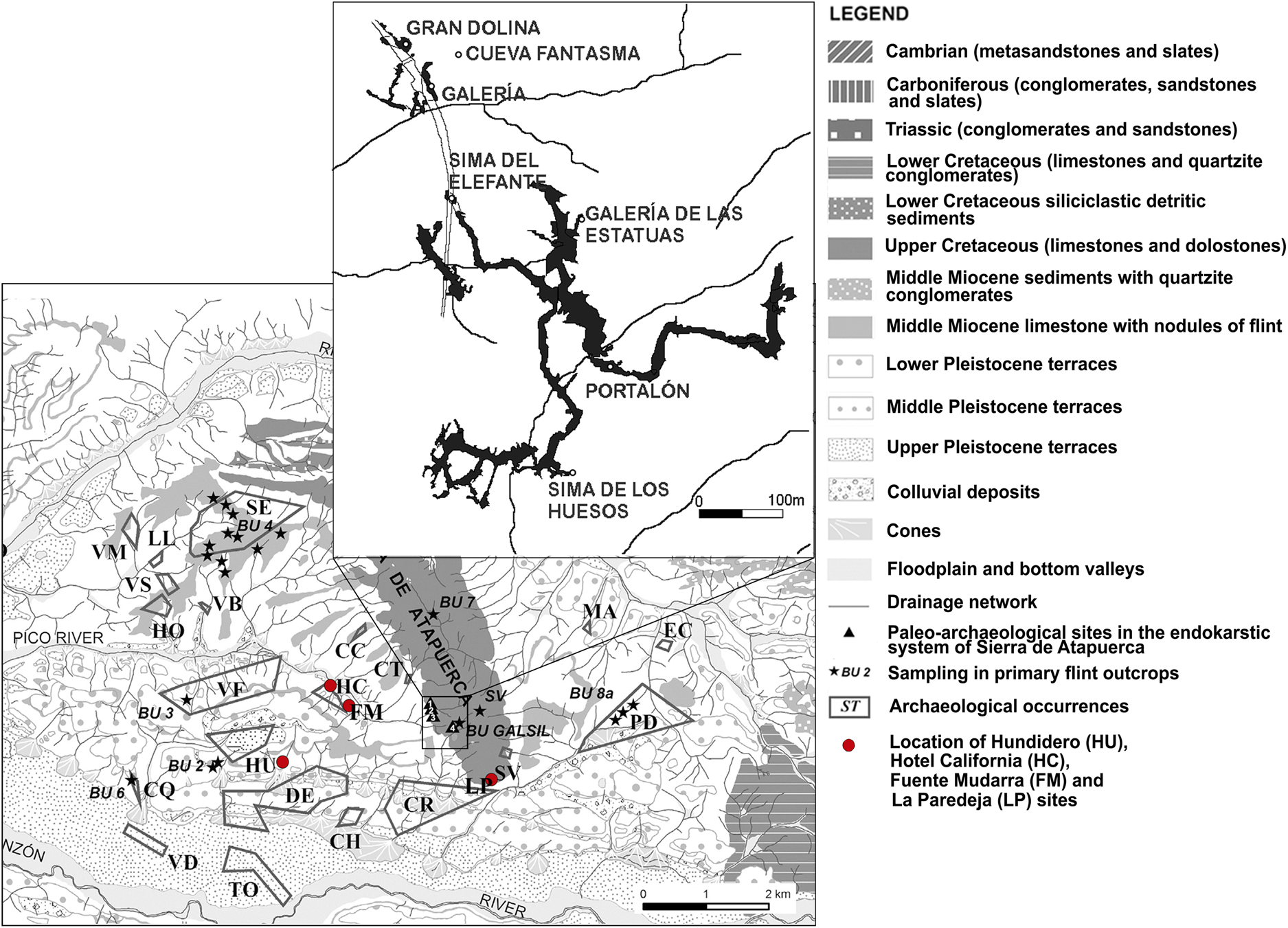
Figure 1. (color online) Map of the upper Pleistocene open-air sites of the Sierra de Atapuerca and archaeological sites in the Cueva Mayor karst system. Modified from Navazo et al. (Reference Navazo, Colina, Domínguez-Bella and Benito-Calvo2008).
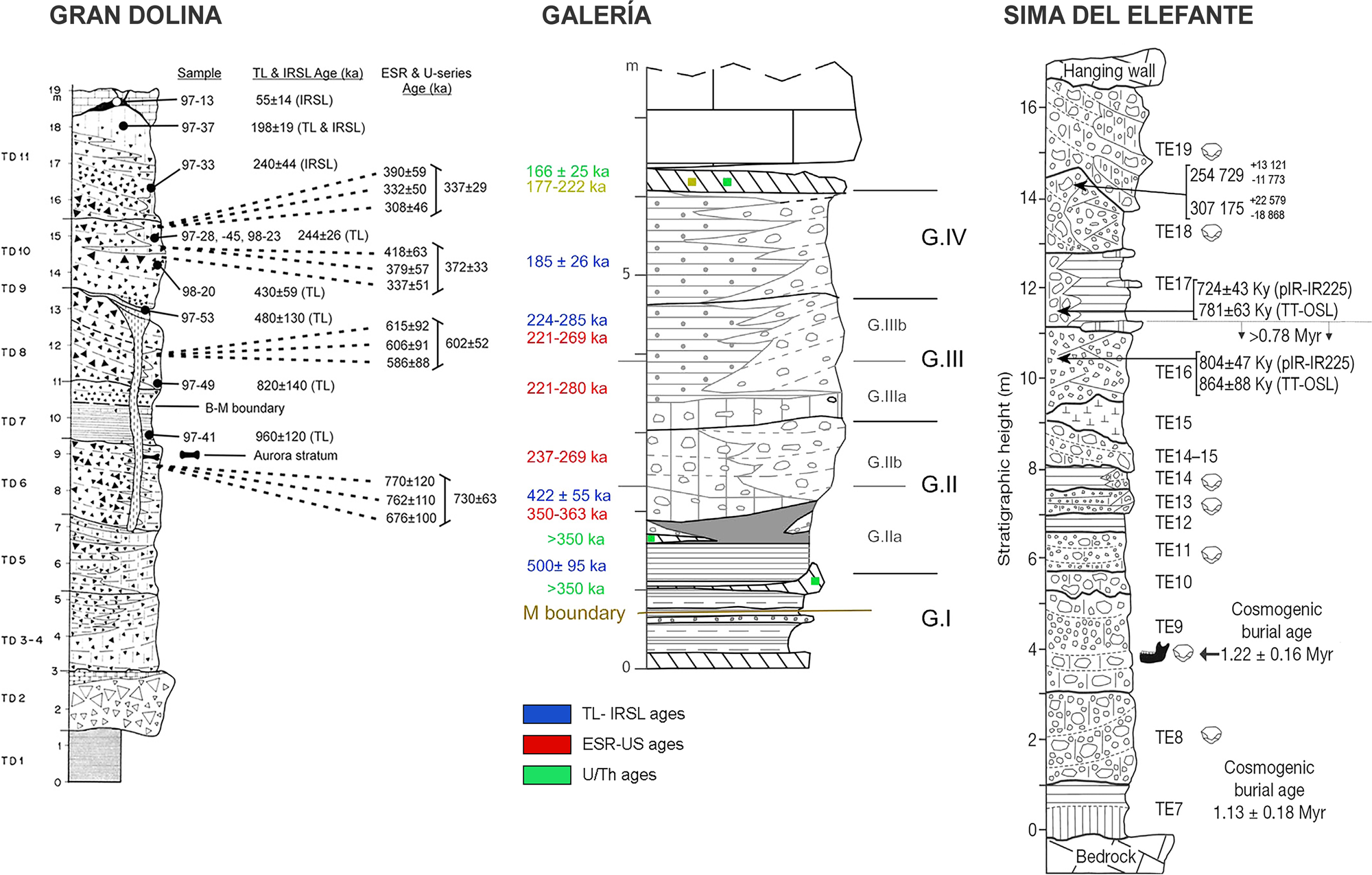
Figure 2. (color online) Gran Dolina stratigraphy and chronologies, from Berger et al. (Reference Berger, Pérez-González, Carbonell, Arsuaga, Bermúdez de Castro and Ku2008), Galería stratigraphy and chronologies from Falguères et al. (Reference Falguères, Bahain, Bischoff, Pérez-González, Ortega, Ollé and Quiles2013), and Sima del Elefante stratigraphy and chronologies from de Lombera-Hermida et al. (Reference de Lombera-Hermida, Bargalló, Terradillos-Bernal, Huguet, Vallverdú, García-Antón and Mosquera2015). TL-IRSL ages: thermoluminescence (TL) and infrared stimulated luminescence (IRSL) dating. ESR-US ages: Combined electron spin resonance (ESR) and uranium series (US) dating. U/Th ages: uranium–thorium dating. pIR-IR ages: post-infrared infrared stimulated luminescence.
In 1999, the Atapuerca Research Team began an archaeological survey in search of Neanderthal evidence (Navazo et al., Reference Navazo, Benito-Calvo, Alonso-Alcalde, Jordá Pardo and Carbonell2017). The archaeological surface survey documented almost 30 sites attributed to the Middle Palaeolithic (Navazo and Carbonell, Reference Navazo and Carbonell2014). Test pits were dug to ascertain the stratigraphic depths of these sites, and three of them were excavated: Hundidero (Navazo et al., Reference Navazo, Alonso-Alcalde, Benito-Calvo, Díez, Pérez-González and Carbonell2011), Hotel California (Arnold et al., Reference Arnold, Demuro, Navazo, Benito-Calvo and Pérez-González2013), and the Fuente Mudarra open-air site, which we present in this paper. Geological prospecting was carried out during the archaeological survey, and all outcrops and secondary deposits of the most representative material around the Sierra de Atapuerca lithic ensembles were documented (Navazo et al., Reference Navazo, Colina, Domínguez-Bella and Benito-Calvo2008).
Three caves dug in recent years, Galería de las Estatuas in Cueva Mayor (Arsuaga et al., Reference Arsuaga, Gómez-Olivencia, Sala, Martínez-Pillado, Pablos, Bonmatí and Pantoja-Pérez2017; Demuro et al., Reference Demuro, Arnold, Aranburu, Gómez-Olivencia and Arsuaga2018; Pablos et al., Reference Pablos, Gómez-Olivencia and Arsuaga2019), Cueva Fantasma, and La Paredeja (see Fig. 1), complete the record from the late Pleistocene, which provides evidence of the presence of Homo neanderthalensis in Atapuerca.
With the Fuente Mudarra study, we intend to complete the occupational model of the Sierra de Atapuerca during the upper Pleistocene. Fuente Mudarra and the other open-air sites verify the Neanderthal presence in Atapuerca. These sites show that Neanderthal groups inhabited the Sierra de Atapuerca area from ~ 123 to 45 ka.
The most important objective is to identify, through the archaeological record in Fuente Mudarra, the different Neanderthal occupations, recognize the occupation locations, understand the uses of the sites, and understand the terms of the occupations and the repetition of activities in the same place, thus defining a land-use pattern.
The discovery and excavation of this upper Pleistocene chronology has made a major contribution to our understanding of the human occupational sequence in Sierra de Atapuerca. The analysed material facilitates explanations of how the Sierra was occupied throughout the entire Pleistocene. The Fuente Mudarra site in particular has yielded evidence of the longest continuous occupation in the Sierra de Atapuerca area during the upper Pleistocene.
GEOLOGICAL BACKGROUND
Fuente Mudarra is in the northeast sector of the Duero Basin (latitude: 42°21'7.37", longitude: 3°32'17.93", WGS84; elevation: 954 m asl), close to the southwest end of the Sierra de Atapuerca (Fig. 3A, 3B).
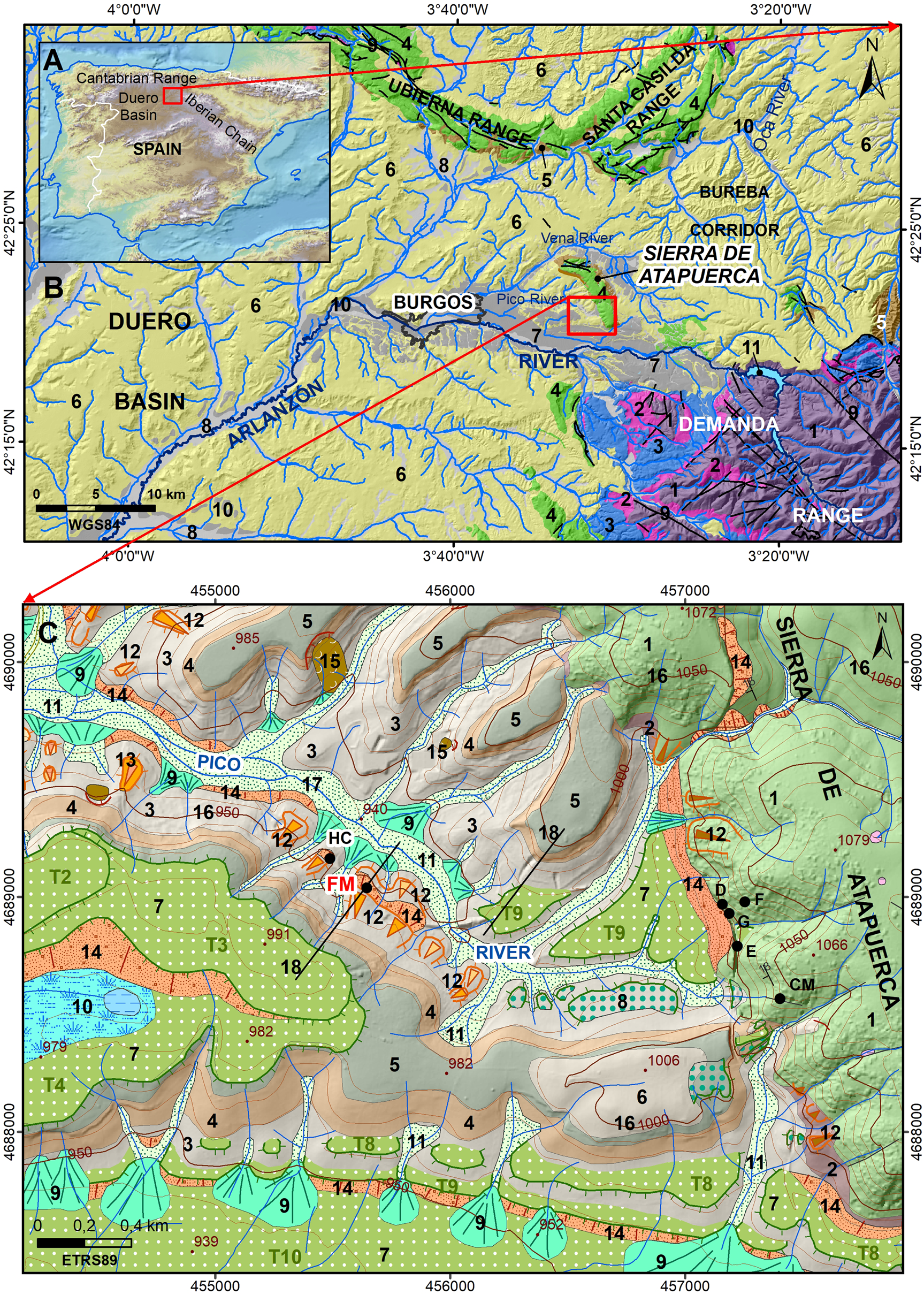
Figure 3. (color online) Geological and geomorphological context of the Fuente Mudarra site (simplified from Benito-Calvo and Pérez-González, Reference Benito-Calvo and Pérez González2015; Benito-Calvo et al., Reference Benito-Calvo, Ortega, Pérez-González, Campaña, Bermúdez de Castro and Carbonell2017). (A) Regional location on the Iberian Peninsula. (B) Sierra de Atapuerca location in the northeast Duero Basin (WGS84). Legend: 1, Palaeozoic; 2, Triassic; 3, Jurassic; 4, Cretaceous; 5, Oligocene-Early Miocene; 6, Miocene; 7, Pleistocene; 8, Holocene; 9, Faults; 10, Drainage network; 11, Reservoir. (C) Geological and geomorphological map of Sierra de Atapuerca (ETRS89). Legend: 1, limestone and dolostone (Late Cretaceous); 2, breccia, conglomerate, sandstone and siltstone (Oligocene–Early Miocene); 3, marl and clay (Early Miocene); 4, clay, marl, and sand (Middle Miocene); 5, limestone layer including flint nodules (Middle Miocene); 6, marl and limestone and local conglomerate; 7, river terraces (T2–T10); 8, rock-cut terraces; 9, cones; 10, semi-endohreic area and pool; 11, floodplain and valley beds; 12, erosive pediments; 13, mantled pediments; 14, colluvial deposits; 15, landslides; 16, contour lines and elevation (interval: 10 m asl); 17, drainage network; 18, archaeological sites: CM, Cueva Mayor; D, Dolina; E, Elefante; G, Galería; F, Fantasma; FM, Fuente Mudarra; HC, Hotel California 19, geomorphological cross-section (Fig. 4A).
The Sierra de Atapuerca is a gentle anticline ridge (Benito-Calvo and Pérez-González, Reference Benito-Calvo and Pérez-González2007, Reference Benito-Calvo and Pérez González2015) composed mainly of Late Cretaceous marl, limestone, and dolostone (Benito-Calvo and Pérez-González, Reference Benito-Calvo and Pérez González2015), which contain centimetric flint nodules (Navazo et al., Reference Navazo, Colina, Domínguez-Bella and Benito-Calvo2008). Mesozoic rock is overlain by Cenozoic alluvial and lacustrine sediments. The Fuente Mudarra bedrock is Early Miocene clay and marl (see Fig. 3C) overlain by Middle Miocene clay, marl, and sand, which are capped by a lacustrine-palustrine limestone layer containing flint nodules (Pineda, Reference Pineda and Arce1997; Navazo et al., Reference Navazo, Colina, Domínguez-Bella and Benito-Calvo2008). In this area, the top of the Cenozoic sedimentation layer mainly consists of Late Miocene marl and limestone (see Fig. 3C).
In the Quaternary, Mesozoic and Cenozoic materials were dissected under exorheic conditions by the Arlanzón River and its main tributaries in this area, the Vena and Pico Rivers (Zazo et al., Reference Zazo, Goy and Hoyos1983, Reference Zazo, Goy, Hoyos, Aguirre, Carbonell and Bermúdez de Castro1987; Pérez-González et al., Reference Pérez-González, Parés, Carbonell, Aleixandre, Ortega, Benito and Martín2001; Benito-Calvo et al., Reference Benito-Calvo, Pérez-González and Parés2008, Reference Benito-Calvo, Ortega, Navazo, Moreno, Pérez-Gonzalez, Parés, Bermúdez De Castro and Carbonell2018). The main river valley shows a sequence of fourteen 2–4−m-thick river terraces (Zazo et al., Reference Zazo, Goy, Hoyos, Aguirre, Carbonell and Bermúdez de Castro1987; Benito-Calvo et al., Reference Benito-Calvo, Pérez-González and Parés2008, Reference Benito-Calvo, Ortega, Navazo, Moreno, Pérez-Gonzalez, Parés, Bermúdez De Castro and Carbonell2018), which primarily contain quartzite gravel bars and, to a lesser degree, sand channels and fine overbank deposits. The river terraces are stratified from T1, 92–97 m above the modern Arlanzón River channel, to T14, located at +2–3 m. Electron spin resonance (ESR) dates indicate an Early Pleistocene age for terraces between T1 (+92–97 m) and T4 (+60–65 m), middle Pleistocene between T5 (+50–58 m) and T11 (+12–14 m), late Pleistocene for T12 (+10–11 m) and T13 (+5 m), and tentatively Holocene for T14 and the floodplain (Benito-Calvo et al., Reference Benito-Calvo, Pérez-González and Parés2008; Moreno et al., Reference Moreno, Falguères, Pérez-González, Duval, Voinchet, Benito-Calvo and Ortega2012). Arlanzón River downcutting during the Pleistocene has been related to the formation of the multilevel endokarst system where the Sierra de Atapuerca sites are located (Benito-Calvo et al., Reference Benito-Calvo, Ortega, Pérez-González, Campaña, Bermúdez de Castro and Carbonell2017, Reference Benito-Calvo, Ortega, Navazo, Moreno, Pérez-Gonzalez, Parés, Bermúdez De Castro and Carbonell2018).
The main open-air sites are related to semi-endoreic areas preserved on river terraces (Hundidero site; Navazo et al., Reference Navazo, Alonso-Alcalde, Benito-Calvo, Díez, Pérez-González and Carbonell2011); other colluvial and alluvial landforms (Navazo et al., Reference Navazo, Benito-Calvo, Alonso-Alcalde, Jordá Pardo and Carbonell2017); and a doline (Valle de las Orquídeas site; Mosquera et al., Reference Mosquera, Ollé, Pérez-González, Rodríguez, Vaquero, Verges and Carbonell2007). The sites are primarily linked to raw material sources—the exhumation and erosion of the Middle Miocene limestone layer that includes flint nodules (Benito-Calvo et al., Reference Benito-Calvo, Ortega, Navazo, Moreno, Pérez-Gonzalez, Parés, Bermúdez De Castro and Carbonell2018). A relevant concentration of open-air occupations is distributed in the Pico River valley (see Fig. 3C), where two sites have recently been excavated: Hotel California (HC; Arnold et al., Reference Arnold, Demuro, Navazo, Benito-Calvo and Pérez-González2013), and Fuente Mudarra (FM; presented in this paper). Both sites are in a similar geomorphological context in the Pico valley (see Fig. 3C). During the final middle Pleistocene, a river terrace and a sequence of three nested pediments were formed in the Pico valley (see Fig. 3C; Benito-Calvo and Pérez-González, Reference Benito-Calvo and Pérez González2015). The river terrace is in the headwater of the Pico River. It has been dated at 348 ± 16 ka using optically stimulated luminescence (OSL) (Arnold et al., Reference Arnold, Demuro, Navazo, Benito-Calvo and Pérez-González2013) and correlated with Arlanzón terrace T9 (Benito-Calvo and Pérez-González, Reference Benito-Calvo and Pérez González2015; Arnold et al., Reference Arnold, Demuro, Navazo, Benito-Calvo and Pérez-González2013).
Pediments are mainly on the south side of the Pico valley, usually mantled by a thin veneer of alluvial-colluvial deposits generated by the erosion of the upper and middle Arlanzón river terraces and the Miocene materials that outcrop on the hillsides (see Fig. 3C). These pediments are erosive near the Hotel California and Fuente Mudarra sites. The oldest pediment level built up at the same base level as the Pico river terrace, +8–18 m above the current Pico River channel (see Figs. 3C and 4A). The colluvial deposits containing both of these sites are at the foot of this erosive pediment level. Hotel California, located to the west, consists of a 1–1.5−m-deep sequence containing up to seven stratigraphic units of poorly sorted silts and clays; a massive structure with locally occurring floating rounded quartzite gravels and subangular flint clasts sits unconformably on the Early Miocene clay and marl. OSL dating for Hotel California yielded ages of 71.0 ± 5.6 ka for Level I at the base, 57.6 ± 5.76 ka for Level II, and between 48.2 ± 3.3 and 48.2 ± 3.9 ka for Level V (Arnold et al., Reference Arnold, Demuro, Navazo, Benito-Calvo and Pérez-González2013). Fuente Mudarra shows a deeper sequence, which stratigraphy, age, and archaeological record are discussed below;).
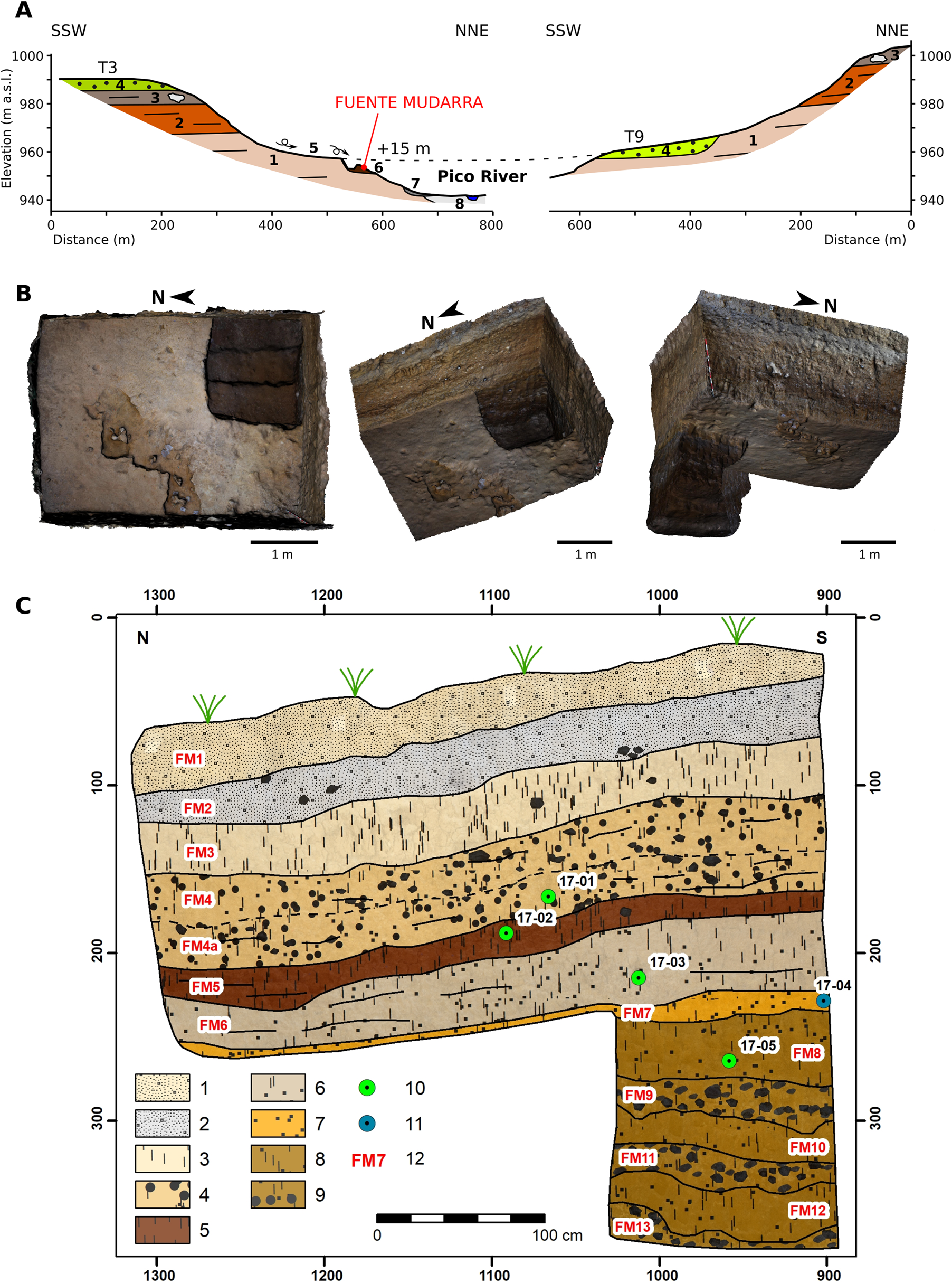
Figure 4. Fuente Mudarra stratigraphic sequence. (A) Pico River geomorphological cross-sections. Legend: 1, marl and clay (Early Miocene); 2, clay, marl, and sand (Middle Miocene); 3, limestone layer including flint nodules (Middle Miocene); 4, river terrace; 5, erosive pediment; 6, colluvial deposit; 7 cone; 8, floodplain. (B) 3D views of Fuente Mudarra trench. (C) Stratigraphic sequence, Section East. Legend: 1, landfill; 2, light grey sandy mud with gravel; 3, massive dark-beige silty clay; 4, massive and gravelly sandy silty clay; 5, massive dark brown silty clay; 7, sandy silty clay and silty sand, locally laminated; 8, massive brown sandy silty clay; 9, massive and gravelly brown sandy silty clay; 10, OSL samples collected from Section E; 11, OSL samples collected from Section S (projected on Section E); 12, stratigraphic unit. (For interpretation of the references to color in this figure legend, the reader is referred to the web version of this article.)
MATERIAL AND METHODS
Stratigraphical and sedimentological descriptions
A stratigraphic survey of Fuente Mudarra was done in combination with field descriptions and laboratory analysis. Terrestrial photogrammetry was used to generate 3D models of the site and orthophotos, which were used as a planimetric base for stratigraphy mapping (see Fig. 4B). Samples were collected for sedimentological analysis, which included granulometry (Robinson Pipeta) and composition (X-ray diffraction (XRD) and X-ray fluorescence (XRF)).
OSL dating
OSL ages were obtained from five samples from the two trench walls of Fuente Mudarra during the 2017 dig. Samples ATA 17 FM L4 OSL 17-01, ATA 17 FM L5 OSL 17-02, ATA 17 FM L6 OSL 17-03, ATA 17 FM L7 OSL 17-04, ATA 17 FM L8 OSL 17-05 (see Fig. 4C) were taken from five sedimentary units and sub-units, namely FM4a, FM5, FM6, FM7, and FM8. Sampling took place in the southeastern corner of the excavated trench, the deepest zone, where the units were more clearly defined. Four of the samples were taken from the eastern wall of the trench (Section E, see Fig. 4C), and one was taken from the southern wall (Section S, see Fig. 4C). Care was taken to avoid contact with areas with bioturbation or pedoturbation and smaller zones with greater textural heterogeneity.
All samples were collected using a low-speed automated rotation-percussion drill under a UV-resistant tarp to prevent light from harming the sediment. Each sample was placed in a light-resistant black zip bag. Gamma dose measurements were collected in situ using a CANBERRA Portable Gamma Spectrometer with a LaBr3 probe. Samples were taken from inside and around each borehole to analyse the light-sensitive sediment and were placed in tightly sealed bags. Each sample's water content and saturation levels were measured, along with precise laboratory beta and gamma dosimetry using a RISØ Beta Counter and CANBERRA High Precision Germanium Detector, respectively. The cosmic dose rate was calculated relative to the depth of each sample, taking into account the overburden density.
Conventional OSL dating was carried out at the CENIEH Luminescence Dating Laboratory in Burgos, Spain. Pure quartz separates (90–125 μm) were extracted from each sample using routine preparation procedures under low lighting (<2 μW/cm2; 600–690 nm). OSL signals were measured using a RISØ TL-DA-20 reader. Multiple-quartz grain aliquots (MGA) were stimulated with blue LED units (470 ± 20 nm, 90% power). Stimulation with infrared (IR) LEDs (875 nm) was also used to check for feldspar contamination at the end of each sample measurement. Samples were irradiated with a calibrated 90Sr/90Y beta source mounted on the reader. Prior to the final equivalent dose (DE) measurement, initial tests on over 60 2−mm MGA such as pre-heat plateau, thermal transfer test, dose recovery, and dose response curve were carried out on all samples. These tests allowed the team to check for various limiting factors inherent to quartz sensitivity, quality, and origin that could affect the samples.
OSL dating results for the five samples are reported in Table 2. For each sample, OSL DE values were measured using the single-aliquot regenerative-dose (SAR) protocol (Murray and Wintle, Reference Murray and Wintle2000, Reference Murray and Wintle2003) on 24 2−mm MGA. A tested pre-heat temperature of 260° C was used for each measurement, and a fast component was observed for all aliquots. DE values and uncertainties were calculated using the Central Age Model (CAM) or the Minimum Age Model (MAM; Galbraith and Roberts, Reference Galbraith and Roberts2012). We were able to apply the CAM in three of the five samples dated by OSL because of the spread of the DE distribution (within 20% of unity; Duller, Reference Duller2008; López et al., Reference López, Goodman-Tchernov and Porat2018). For two of the samples, MAM was applied due to the over-dispersed nature of the DE distribution; these samples showed evidence of poor bleaching during transportation and deposition.
Technological study
The criteria employed in the Logical-Analytical System were applied to the Fuente Mudarra lithic industry analysis (Table 1) (Carbonell et al., Reference Carbonell, Guilbaud and Mora1983, Reference Carbonell, Mosquera, Ollé, Rodríguez, Sala, Vaquero and Vergés1992, Reference Carbonell, Rodríguez, Giralt, Mosquera, Ollé, Sala, Vaquero, Vergés, Bermudez de Castro, Arsuaga and Carbonell1995, Reference Carbonell, Márquez, Mosquera, Ollé, Rodríguez, Vaquero, Vergés, Carbonell, Rosas and Díez1999, Reference Carbonell, Mosquera, Ollé, Rodríguez, Sahnouni, Sala and Vergés2001; Carbonell and Mora Reference Carbonell and Mora1986; Carbonell and Rodríguez, Reference Carbonell and Rodríguez2002). In order to maximise the information gathered from the assemblage, we also used other technological criteria described by several authors (see Table 1) (Vaquero, Reference Vaquero1999; Vaquero and Carbonell, Reference Vaquero, Carbonell and Peresani2003; Menéndez, Reference Menéndez2009; Carmignani et al., Reference Carmignani, Moncel, Fernandes and Wilson2017).
Table 1. Logic analytical system categories (LAS). Adapted from Ollé et al. (Reference Ollé, Mosquera, Rodríguez, de Lombera-Hermida, García-Antón, García-Medrano and Peña2013).

Criteria proposed by Carmignani et al. (Reference Carmignani, Moncel, Fernandes and Wilson2017) for the study of systems employed in Bau de l´Aubesier and Payre were followed in the case of cores. The four technical parameters studied for each core were volumetric concept, platform organization, removal direction, and angle between preparation face and removal plane.
Recommendations by Vaquero (Reference Vaquero1999) were followed in the definition of variations within the centripetal operating systems; this approach takes into account criteria such as the principle of symmetry and hierarchization to define the amount of control in the reduction of core volumes (Vaquero and Carbonell, Reference Vaquero, Carbonell and Peresani2003). These authors focus on these factors in their explanation of variations in centripetal strategies in the Abric Romaní assemblages.
In the analysis of the positive bases (PBs) (see Table 1), the methodology proposed by Carmignani et al. (Reference Carmignani, Moncel, Fernandes and Wilson2017) was followed to assess several parameters and identify products derived from centripetal exploitations; this approach distinguishes between the results of exploitation of parallel and secant planes. Criteria included angles of removal from the dorsal surface, angle between butt and ventral surface, and direction of previous removals.
Exploitations with secant planes resulted in products with dorsal anterior centripetal removals and short, thick forms. Butts are generally angled, dorsal removals have a secant direction, and the resulting ridges have wide 40–60° angles. Two sub-types have been distinguished: products deriving from centripetal directions (A1) with continuous cutting edges, and those from the chordal direction (A2), with debordant flakes. Products of exploitations with parallel planes are generally flatter. The angle between the butt and the ventral surface varies between 95 and 115°. Dorsal removals are parallel or sub-parallel, and edges have angles about 15–40° thick. Categories B1 and B2 are differentiated in order to distinguish between centripetal and chordal products.
Bagolini's method (Reference Bagolini1968) was followed in the typometric analyses. The variables used in the Logical-Analytical System (LAS) (Carbonell et al., Reference Carbonell, Márquez, Mosquera, Ollé, Rodríguez, Vaquero, Vergés, Carbonell, Rosas and Díez1999; Rodríguez, Reference Rodríguez2004) were employed in the analysis of the retouched flakes and in their identification using the Laplace Analytical Typology (Laplace, Reference Laplace1972).
RESULTS
Excavation at the Fuente Mudarra site began in 2012. A 12−m2 test pit permitted the identification of 13 levels (FM1–FM13), 9 of which (FM1, FM2, FM3, FM4, FM5, FM7, FM8, FM9, FM10) were found to contain archaeological remains. Levels FM9–FM13 were dug in a small area of just over 1 m2 in a quadrat (J36) located in the southeast corner of the sample area (Fig. 4B).
The archaeological record consists exclusively of lithic remains. Unfortunately, there are no faunal remains. They may not have been preserved because the sedimentary environment is unfavourable, or it may be that the activities that occurred there did not involve fauna as a priority.
Stratigraphic sequence
The Fuente Mudarra sequence is at least 3.5 m thick, with a succession of locally gravelly muds. In this sequence, we distinguished 13 stratigraphic units separated by sharp and gradual contacts (see Fig. 4C). The lower part of the sequence comprises an alternation of mud and gravel (units FM13 to FM8). Muds are brown massive sandy silty clay, and gravel are matrix-supported pebbles and cobbles composed mainly of rounded and sub-rounded quartzite (see Fig 4C). Unit FM7 sits on these levels. It is characterized by the highest sand content (35%), which can appear locally laminated. Unit FM6 is a pale brown sandy silty clay layer with scarce gravel. Unit FM5 comprises dark brown silty clays, including some floating pebbles. The percentage of clasts increases in unit FM4a (around 15–20%), including rounded pebbles and cobbles of metamorphic rocks (mainly quartzite) and subangular clasts of limestone and flint, some of them reaching boulder size. Planar clasts are angled 8–10° toward the north. Clasts are matrix supported in a light brown and massive sandy silty clay matrix. Unit FM4 contains fewer clasts (<3–15%), especially toward the west, although the matrix maintains similar characteristics to unit 4a. Level FM3 consists of dark beige silty clay that are locally gravelly with floating metamorphic pebbles. Units FM13–FM3 contains abundant pedogenic cutans and gleyic colours. Unit FM2 is a light grey unit (see Fig. 4C) that includes sandy mud and gravel mixed in soil horizon A. FM1 is an anthropic landfill produced by recent earthworks.
OSL dating
We were able to obtain burial dose estimates from the majority (88%) of the MGA measured for each of the five samples dated in this study. Twenty-four aliquots (each containing ~100 grains) were used for the final DE estimation of each sample using the SAR protocol (see Table 2). Three of these samples yielded finite numerical OSL ages after applying the CAM (see material and methods section as their over-dispersion (OD) values were lower than 20%. The other two samples only yielded a terminus ante quem estimate. For these, the MAM age model best fitted the minimum age determination due to the wide range of DE value distribution (OD >20%). Nevertheless, all five samples are roughly in correct stratigraphical order. They are the second-youngest set of OSL ages obtained from the foothills and marginal valleys of the Arlanzón River and its tributaries around the Sierra de Atapuerca.
Table 2. Optically stimulated luminescence (OSL) age results. Ages referenced to 2018 (OSL measurement year). Confidence interval 1σ, including systemic uncertainties of doses, water content, and errors associated with equivalent dose (DE) measurement.

Lithic assemblages
In total, 2021 items (Table 3) from Levels 4, 5, 7, 8, 9, and 10 of the Fuente Mudarra site were analysed. The first three levels were excluded from the technological analysis because FM1 was a jumbled level due to disturbance by agricultural equipment and Levels FM2 and FM3 contained few remains and were heavily disturbed. Levels FM6, FM11, FM12, and FM13 were found to be archaeologically sterile. The main shared characteristics of all analysed levels are the preference for Neogene flint as raw material and the use of essentially centripetal operations aimed at producing small-sized PBs.
Table 3. Counts and percentages of lithic remains from Fuente Mudarra layers.

Level 4
In all, 1394 lithic remains were found in Level 4. The main problem at this level is the preservation of these archaeological remains; preservation quality has been heavily degraded due to the formation of patinas and fracturing and gelifraction scars (gelifraction is a type of fracture produced by the action of ice that, in our case, results in gelifraction domes and splinters; Fig 5). This is evidenced by the large proportion of indeterminate and fragmented items (frag, FPB, and Frag of PB) and gelifracts (Table 4).

Figure 5. (color online) (A) Fragment with gelifraction domes. (B–E) Examples of gelifraction splinters in different sizes.
Table 4. Composition of the lithic assemblages of Fuente Mudarra archaeological levels.

Neogene flint is the predominant raw material on this level, comprising 89.31% of all items. It is followed by quartzite (3.51%), quartz (2.36%), indeterminate flint (2.36%), Cretaceous flint (1.64%), and other little-used material such as sandstone and limestone. On Level 4, 11 natural bases and fragments of Neogene flint have been documented. In this archaeological context, many of the natural bases (nB) (see Table 4) made from Neogene flint are unworked, some are fractured, and others have been tested and then discarded after a couple of deliberate removals. We found 29 nBs made of quartzite river pebbles that may have been used as hammerstones, although no percussion scars were documented. They are therefore unlikely to have been part of the operative chain and were merely a result of the formation of the deposit. The cortical surfaces of these pebbles are degraded, making it impossible to distinguish the zones where the scars are located.
Only one Neogene flint core, a First Generation Negative Base of Exploitation (1GNBe), was found at this level. It was exploited on a large nB, with natural platforms (Fig. 6A). Exploitation of this large volume was begun but then abandoned, probably due to the low quality of the raw material since the flint contained intrusions and geodes. This initial tentative exploitation produced a well-defined centripetal surface and another slightly exploited surface in an orthogonal bipolar direction. This led us to define it as a centripetal peripheral exploitation of secant planes in an initial state of exploitation.
We resorted to the analysis of the PBs to define the reduction sequences given the lack of other cores. In all, 16 complete PBs show origins in centripetal systems or chordal secant planes exploitations (14 of type A1 and 2 of type A2), and only two show origins in parallel planes (1 of type B1 and 1 of type B2) (Fig. 7A, 7B, 7J, 7L, 7M). PBs or flakes were prominent in the assemblage, accounting for 58.67% of the total material on this level, including complete (15.78%) and fractured bases (FPB: 5.95% and Frag of PB: 36.94%). This level predominantly contains small and micro-sized flakes, along with a large amount of knapping debris. In general, the flakes have non-cortical butts (Fig. 8), plain and unifaceted, with basically non-cortical dorsal surfaces and several removals in the dorsal surface.
There is a small proportion of retouched tools, not quite 2% of the items. There are various types of 2GNBcfrom Level 4, despite being a modestly represented category, with seven sidescrapers (types R11 [1], R21 [2], R22 [1], R23 [3]), five denticulates (D1), three notches (D21), two Tayac points (D24), one bec, and two abrupt retouches (A1 and A2). The features common to all these shaped items are unifacial retouch and a preference for simple and abrupt retouch that is not especially invasive with respect to either the ridge or the face. Sizes indicate that the largest items were usually chosen to be retouched (Fig. 9).
Level 5
Fuente Mudarra Level 5 yielded a total of 297 items (see Table 4). The proportion of fractured items is quite similar to Level 4; however, their surfaces are better preserved with a smaller percentage of gelifraction scars. Orange patinas are found on items from this level. Neogene flint is still the predominant raw material (91.24%). However, there is a significant increase in the number of Cretaceous flint items (6.06%). The other materials found on this level, quartzite, quartz, sandstone, and limestone, are marginal (less than 1%).
The nB of this level primarily consisted of 10 blocks of Neogene flint, in some cases showing signs of test removals. Three cores have been found with peripheral centripetal exploitation or chordal exploitation of secant planes, regardless of their original base.
Only one of the cores is exploited on an nB (1GNBe) (see Fig. 6B). The core is in an intermediate exploitation phase with a centripetal secant tendency and non-hierarchical exploitation. In this particular case, both surfaces seem to have been exploited at the same time with a lack of preparation. The two 2GNBe (cores on flake), show the same system employed in a similar way (see Fig. 6C, 6D). It should be noted that one was subsequently reused as a tool after retouching the distal part of the PB (see Fig. 6C).

Figure 6. (color online) Cores (1GNBe and 2GNBe) from level 4 (A), level 5 (B–D), and level 8 (E–H).
The PB reflects exploitations essentially involving centripetal systems with secant planes. Of the 16 PB whose exploitation could be defined, 100% were identified as type A1: the result of centripetal peripheral exploitation of secant planes (see Fig. 7C, 7D). PBs are widely represented at this level. They account for 60.6% of the total between PB, FPB, and Frag of PB. The dorsal surfaces are non-cortical, with three or more previous removals.
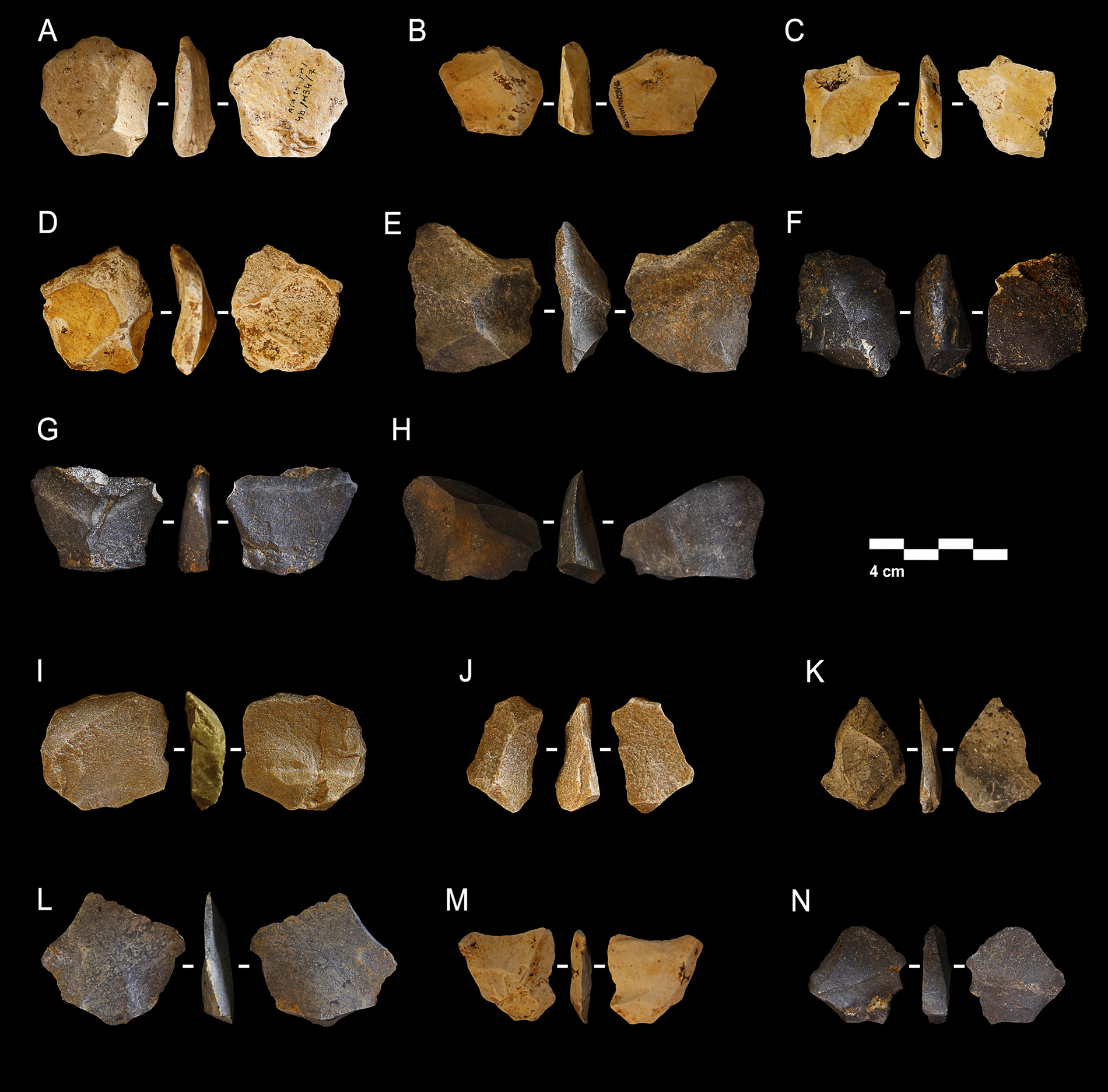
Figure 7. (color online) Examples of centripetal and chordal exploitation blanks. A1 type (A–H), A2 type (I, J), B1 type (K, L), B2 type (M, N).

Figure 8. Butts analysis scheme: (C) cortical, (NC) non-cortical, (CD-NC) cortical dominant, (NCD-C) non-cortical dominant, (PLA) plain, (LIN) linear, (PUN) punctiform, (NF) non-faceted, (UF) unifaceted, (BF) bifaceted, and (MF) multifaceted.
The percentage of retouched tools is smaller on Level 5 than Level 4. Only 6 retouched tools have been found (one of them fragmented). Three of these complete tools (sidescraper, denticulate, and retouched flake) are made on Neogene flint (R22, R21, and D1) (see Fig. 9G, 9H), and two are made on Cretaceous flint (D21 and D23) (see Fig. 9I, 9J). The common features include unifacial retouches and abrupt or semi-abrupt angles.
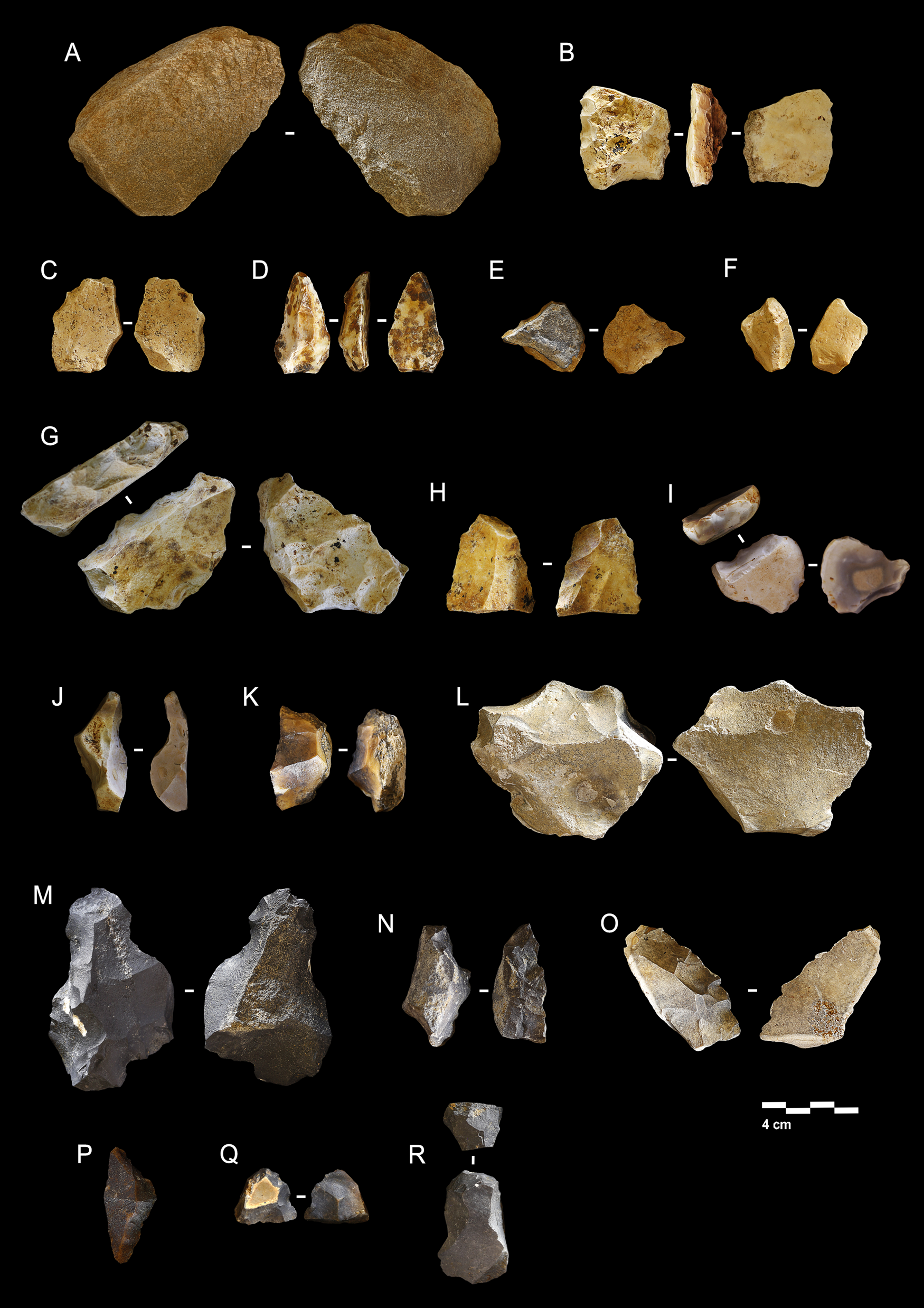
Figure 9. (color online) First Generation Negative Base of Configuration and Second Generation Negative Base of Configuration. Fuente Mudarra retouched items from level 4 (A–F), level 5 (G–J), level 7 (K–M), and level 8 (N–R).
Level 7
This level yielded a relatively small number of lithic items (only 98), but they are in a very good state of preservation. There is no evidence of gelifraction here, and there is considerably less fracturing. All items from this level have completely black/grey patinas, which are uniform on all surfaces (see Table 4).
As in the upper levels, Neogene flint is the predominant material (75.51%) in Level 7. The 16.32% of undetermined flint is due to the difficulty of identifying the material de visu, given the dense patinas on material at this level. The nB found at this level are principally six quartzite pebbles and one block of fractured Neogene flint. It shows a couple of test removals. It was probably discarded because of its poor quality since it has intrusions, coarse grains, and geodes inside the block.
It is difficult to define the exploitation systems used on Level 7, because we found no cores. In 12 of the 32 complete PBs (37.5% of PBs), the origin could be attributed to centripetal exploitation systems. Peripheral exploitation of secant planes was identified in 10 of the products (seven type A1 and three type A2) (see Fig. 7E, 7F), and two cases of peripheral exploitation of parallel planes were identified (one type B1 and one type B2) (see Fig. 7K).
The flake categories (PB, FPB, and Frag of PB) form 64.2% of the total. Among the PBs are knapping debris and small, medium, and large flakes (Fig. 10). The butt types are unifaceted and non-cortical. In all cases, the dorsal surfaces are non-cortical and there are more than three removals, generally centripetal.
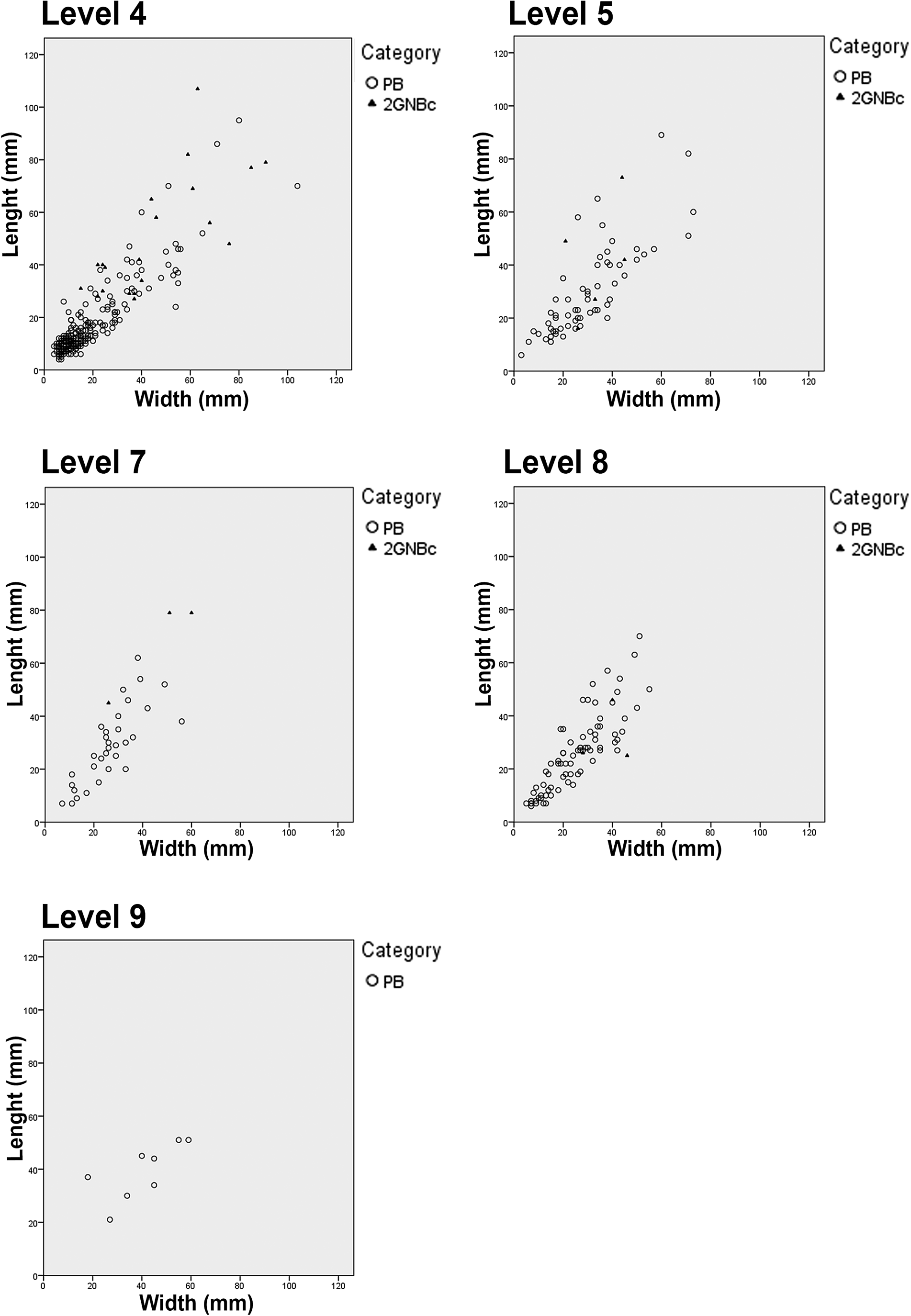
Figure 10. Typometry of Positive Bases and Second Generation Negative Base of Configuration of Fuente Mudarra (by levels).
Only three retouched items were found on this level. One of the items (see Fig. 9M) is made on a fragment with two opposing notches (D21), one is a large denticulate (D3) (see Fig. 9L), and one is an abrupt deep retouch on a fragment (see Fig. 9K).
Level 8
Level 8 contains 209 stone technology items, with similar features to those on Level 7 (see Table 4). There are no remains with gelifraction, and there is a smaller proportion of fractured items than in Levels 4 and 5. Like in Level 7, intense greyish patinas were observed on all the pieces.
We found 22 quartzite nBs and 4 Neogene flint nBs in different sizes. In some cases, the flint nB seems to have been tested. The exploitation systems are somewhat more varied on this level, with a predominance of centripetal exploitation systems on secant planes. All cores are in the 1GNBe category, that is, exploitation directly on blocks of Neogene flint.
In three cases, bifacial centripetal systems of secant planes were applied to Neogene flint nodules of different sizes. Only one of the cores reached an advanced stage of exploitation in which a third surface was generated as a result of the exhaustion of angles and striking platforms surfaces, resulting in a trifacial core. The change of type from bifacial to trifacial centripetal is due to the knapper's search for volume on the third surface at an advanced point in its exploitation, when the size of the core was quite small. Another core is on a medium-sized nB with cortical surfaces. The exploitation system employed on the base is bipolar orthogonal.
Analysis of the complete PB showed centripetal systems employed in this level (see Fig. 7), primarily represented by the results of exploitations of secant planes (17 type A1 and 2 type A2), and only 3 from the exploitation of parallel planes, type B1. The PB on Level 8 repeat the same patterns found on the previous levels, with non-cortical, unifaceted, and dorsal surfaces with no cortex and several previous removals.
Retouched products (First Generation Negative Base of Configuration [1GNBc] and Second Generation Negative Base of Configuration [2GNBc]) include denticulates (D2), denticulate sidescraper and sidescraper (D23 and frag of R2), endscraper (G11), and Tayac point (D24). A noteworthy aspect is that several of these tools were shaped directly on fragments (see Fig. 9N–R).
Level 9
Work on this level only involved a roughly 1−m2 test area in the southeastern corner of the main Fuente Mudarra test pit (see Fig. 4B). Nineteen lithic technology items were found, 13 of Neogene flint, with patinas in different shades of white, grey, and black.
The distinctive feature of the nBs from this level is their large size. Neogene flint nBs include large cobble nodules (one of them was tested). There are also large quartzite nB. PB is the largest category of material from Level 9. The butts are non-cortical, and the dorsal surfaces have removals (between 2 and 5), two of which were from centripetal exploitation systems. The remaining undescribed items are fragments of uncertain origin. Abrasion is detected on some items, along with post-depositional fracturing.
Level 10
Four lithic industry items were found in the test pit on Level 10 (one nB, one fragment, one FPB, and one PB), all in Neogene flint, which simply served to confirm occupation at this level.
DISCUSSION
Fuente Mudarra is 1 of 30 open-air sites with Neanderthal occupations in the Sierra de Atapuerca. It was visited repeatedly during Marine Isotope Stage (MIS) 5–3. The technological features found at the different levels indicate that it resembles the rest of the documented sites in this area.
Previous geomorphological studies have revealed that the Fuente Mudarra and Hotel California sites are in a colluvial landform produced by the dismantling of a middle Pleistocene mantled pediment level (Benito-Calvo, Reference Benito-Calvo2004; Benito-Calvo and Pérez-González, Reference Benito-Calvo and Pérez González2015). The latter was formed around 348 ka BP (Arnold et al., Reference Arnold, Demuro, Navazo, Benito-Calvo and Pérez-González2013) by the erosion of the T3 Arlanzón river terrace (which generated a large amount of rounded metamorphic gravel) and the Miocene stratigraphic units (large amounts of mud, limestone, and subangular flint clasts). This pediment is usually mantled by these materials in other parts of the Pico River, and is the source material of the colluvial landform where Fuente Mudarra and Hotel California are located. Previous studies at the Hotel California site have suggested that the dismantling process mainly took place between 71 and 48 ka (Arnold et al., Reference Arnold, Demuro, Navazo, Benito-Calvo and Pérez-González2013), in the MIS 3–4 transition. Nevertheless, new OSL ages presented in this work suggest that pediment dismantling started earlier, around 123 ka (MIS 5). At Fuente Mudarra, the erosion of this pediment generated the build-up of a predominantly sandy mud sequence, mainly massive in structure on an 8–10° north-facing slope. These characteristics and geometry suggest that the sediments were transported and sedimented by mud flows. This would be the case for stratigraphic units FM12, FM10, FM8, FM6, FM5, and FM3. In contrast, other units such as FM4, FM4a, FM9, FM11, and FM13 contain a significant percentage of clasts. The clasts in these units are basically pebbles, cobbles and, to a lesser degree, boulders, ranging from rounded to subangular in shape, depending on the source material. The clasts are mainly matrix supported, floating in a sandy muddy matrix. Altogether, these characteristics point to an unsorted or very poorly sorted sedimentary process such as debris flow. Water-current lamination was observed locally in unit FM7, suggesting run-off processes were a factor in the formation of the sequence as well. All these units contain abundant cutans and gleyic features, indicating illuviation pedogenetic processes and seasonal water saturation after sedimentation. These geomorphological and sedimentological characteristics suggest that the Fuente Mudarra sediments are from a nearby source in the dismantled pediment level, 15–20 m from the site.
OSL sample ATA 17 L5 OSL 17-02 and, to a lesser extent, samples ATA 17 FM L6 OSL 17-03 and ATA FM L8 OSL 17-05 showed positively skewed tails. Coincidentally, all three samples were from three units characterized as mud flows (i.e., FM5, FM6, and FM8, respectively, see Fig. 4C). With incomplete bleaching, an increase in the viscosity and turbidity of a flow implies a decrease in the capacity of effective solar resetting of the grains transported by this medium, resulting in a wider DE distribution (López et al., Reference López, Goodman-Tchernov and Porat2018). Interestingly, sample ATA 17 FM L4 OSL 17-01 showed strong multi-modality with a greater concentration of the DE population at higher values. This sample was associated with a much thicker and stratigraphically younger deposit of debris flow (unit FM4, see Fig. 4C). The main difference between these types of distribution may mark the difference in the depositional environment. When one flow is denser than the other, it is easier to find quartz grains in “darker conditions” than the other, allowing for a lower concentration of residual OSL signals that do not reflect the most realistic deposition date (or last burial).
With regard to raw material, the most commonly used in all levels is Neogene flint, collected around the site. As mentioned above, there is a secondary deposit of this material in the area. Exactly the same pattern has been found at 27 of the 30 documented open-air sites in the Sierra de Atapuerca area, indicating the knappers were thoroughly familiar with the area where they lived, as they occupied zones with secondary deposits of this flint. This may have been one of the major factors for deciding to settle in the Pico River valley, which contains primary outcrops with - nodules of this material and also secondary deposits generated by erosion of the former material in gravitational and alluvial processes (Navazo et al., Reference Navazo, Colina, Domínguez-Bella and Benito-Calvo2008).
In most of the sites of these chronologies, as in our case, the raw material is usually within a radius of 5 km around the site. Several exceptions attract attention such as the Axlor flint (Ríos, Reference Rios-Garaizar2017) and the Jarama VI flint (Navazo et al., Reference Navazo, Jordá Pardo, Burow, Kehl, Pastoors, Weniger and Wood2020). In both sites this material is introduced in the form of finished tools, and quartz and local flint are the predominant raw materials in these assemblages.
Neogene flint shows characteristic superficial alterations (abrasion, gelifraction, and patina, among others) and internal irregularities. The quality of the blocks from the outcrops and crumbled material found in secondary deposits is quite uneven; some blocks have different granular textures and inclusions, and others have a regular, crystalline texture. (García Antón, Reference García Antón2016). This is the reason for the numerous cases of fracture during knapping.
In all levels of Fuente Mudarra we have seen siret fractures (Siret, Reference Siret1928), flexion fractures, and also fractures due to the material's lines of weakness. We also observed alterations to the surface of the archaeological material in this type of open-air context, including a large amount of gelifraction (see Fig. 5) on Levels 4 and 5, probably because the material was exposed to cold conditions on the surface for a long time. There are intense patinas on the Neogene flint items, with a variety of colors in the different strata, but not on the other raw materials found at Fuente Mudarra. These patinas were probably caused by illuviation and seasonal saturation with water.
Large nodules and natural fragments of this material were found at all levels of the site. The lack of cortex removal spall or cortical elements in the assemblages is due to the nature of the Neogene flint supply sources on secondary deposits (Navazo et al., Reference Navazo, Colina, Domínguez-Bella and Benito-Calvo2008), in which the matrices broke off the outcrop into more accessible sizes and lost their cortex naturally (Navazo et al., Reference Navazo, Alonso-Alcalde, Benito-Calvo, Díez, Pérez-González and Carbonell2011).
The flint nodules found at Fuente Mudarra are no more than 220 mm long, and somewhat smaller sizes (87×64×39 on average) are more frequent. The size and weight of these nBs may therefore have been a criterion for their selection, along with their lack of cortex. Several of these selected nodules were tested for the quality of the raw material, normally by means of a few removals. These tested nBs were then discarded in some cases, when the flint exhibited intrusions, geodes, granulation, etc.
The exploitation systems shared several features throughout the analyzed levels. The cores throughout the sequence follow the same pattern in different intensities on differently sized nBs and PBs. In the reduction systems, the common denominators are the exploitation of the largest base surfaces and the use of bifacial secant planes in centripetal directions, which we interpret as a variation within the discoid exploitation system.
In these cores, no evidence is found of prior surface preparation, and in some of these bifacial patterns there is more exploitation on one of the surfaces, without being preferential in any item. This centripetal method with secant planes (discoidal) is ideal for the production of the largest number of flakes from the initial core volume, since it does not require much reconditioning and generates little wasted raw material (Vaquero et al., Reference Vaquero, Chacon, Cuartero, García-Anton, Gomez de Soler, Martínez and Carbonell2012). In these cases, exploitation is based on quantitative criteria in which the lack of hierarchization permits more productive cores (Vaquero and Carbonell, Reference Vaquero, Carbonell and Peresani2003).
This method is not demanding in terms of the criteria applied to the reduction sequence, in which the requirements for continued recurrent exploitation are reduced to the maintenance of the angle between the core platforms and a peripheral convexity, in contrast to the more stringent requirements of other methods such as Levallois (Vaquero et al., Reference Vaquero, Chacon, Cuartero, García-Anton, Gomez de Soler, Martínez and Carbonell2012).
There was little variation in the centripetal or discoid patterns detected at Fuente Mudarra. There are no clearly hierarchical cores, and most cores tend to be symmetrical as the exploitation process advanced, leaving behind the initial volume of the natural base (Fig. 11).

Figure 11. (color online) Scheme of the reduction sequence (centripetal system of secant planes at different stages of exploitation).
Volume is the prime factor in this variability—for example, it influences the asymmetries that result from the initial shapes of the bases used for exploitation or the exhaustion of angles produced by changes in the exploitation system (in the case of a change from discoid to trifacial). Nevertheless, the premise for deciding about core reduction seems to always have been the same, and the end products help to confirm this. The majority of the complete PBs were not definable, but those that were showed signs of being the result of centripetal/chordal exploitations.
The PBs in which it was possible to define the production method (66) were mostly A1 or A2, in congruence with the described exploitation systems. Type Aa, the result of centripetal exploitation of secant planes, accounted for 89% of the identified PBs (n=59), while only 7 PBs were type Bs, from centripetal exploitation of parallel planes.
Exploitation was aimed at producing flakes for direct use, without stringent shape or size requirements. The proportion of retouched tools was very low at all levels (less than 3%)— a clear indication that this was not the main purpose of the knapping process.
In short, it is appreciated that the exploitation almost exclusively involved a single method employed throughout the sequence. We found a core with opposite bipolar removals and a few flakes that may have come from centripetal cores of parallel planes, but none of the latter were found. The proportion of cores in the assemblage is also very low (only seven complete cores), and all were discarded at different stages of exploitation—initial and full—without any of them having been exhausted.
This exploitation method has been found at sites from the Lower Palaeolithic to the Neolithic (Vaquero and Carbonell, Reference Vaquero, Carbonell and Peresani2003). In the present case, it was the central thrust of tool production throughout the entire Fuente Mudarra sequence over the course of almost 70 ka.
Delagnes and Meignen (Reference Delagnes, Meignen, Hovers and Kuhn2006) write about various systems of exploitation in France during the Middle Palaeolithic and state that their geographical and chronological distribution shows general trends such as an increased use of the systems with a low degree of predetermination at the end of MIS 4. The authors state that this “can be attributed to groups with different technical traditions who kept their own fundamental technical identity and who also adopted similar mobility patterns.”(Delagnes and Meignen, Reference Delagnes, Meignen, Hovers and Kuhn2006: 85) This is how we interpret the occupations in Atapuerca: groups with a strong cultural tradition repeated a stable land-use pattern during the Middle Palaeolithic.
The purpose of the exploitation was to produce small (2–4 cm according to Bagolini [Reference Bagolini1968]) and medium-sized PBs (4–6 cm according to Bagolini [Reference Bagolini1968]) (see Fig. 10), which seem to have been functionalised directly. The first functional analysis of Level 4 at Fuente Mudarra (study in progress) employed a SZX10 stereoscope and an Olympus LEXT OLS3100 confocal microscope, both monitored Seven items were studied, all of them in Neogene flint, and they were selected on account of the differential characteristics of their ridges. The tools from this level could not be exhaustively inspected due to the poor state of the flint and the presence of patinas in almost the entire assemblage, which is a hindrance to this type of analysis. The uses of three of these tools could not be defined. The other four tools showed diagnostic traces of use on different materials: one for hide scraping, two for wood scraping, and one for which we could only define the hardness of the material it was used on: soft or semi-soft material.
Open-air sites are of great importance in understanding different aspects of the everyday lives of prehistoric human groups. Our knowledge of their activities would be biased if it were based solely on information from cave sites (Arrizabalaga et al., Reference Arrizabalaga, Rios-Garaizar and Alvarez-Alonso2014). The site poses an added difficulty for interpretation since it lacks a finite container space such as a cave, where occupations can be spatially delimited. In the case of Fuente Mudarra, where only 12 m2 have been explored to date, the complete site probably spread across a large part of the Pico River valley, and the occupation layers that have been found are extensions of recurrent occupations that ultimately formed large palimpsests across the area.
The Fuente Mudarra lithic industry showed features similar to those of the other open-air sites, with centripetal and orthogonal exploitations focused on the production of bases, very rarely retouched, with a preference for small-sized PBs (Navazo and Carbonell, Reference Navazo and Carbonell2014). At these sites, the cores were reused as tools in a few cases, although the abundance of raw material made this recycling unnecessary. At Fuente Mudarra, one example of this was found (see Fig. 6C), a Second Generation Negative Base of Exploitation retouched at one end.
Fuente Mudarra is a few metres from Hotel California, another of the Sierra de Atapuerca open-air sites. The final OSL ages for Hotel California show that the sediments associated with the in situ Mousterian lithics were laid down over an ~23 ka period between 71 and 48 ka (Arnold et al., Reference Arnold, Demuro, Navazo, Benito-Calvo and Pérez-González2013). This chronology limits the Middle Palaeolithic occupation of the Hotel California site to between the early MIS 4 (ca. 57–71 ka) and the middle of MIS 3 (ca. 29–57 ka) at or prior to the Heinrich Event 4 at ca. 45 ka (Hemming Reference Hemming2004; Lisiecki and Raymo, Reference Lisiecki and Raymo2005). Luminescence dating of quartz grains from several Hundidero site levels at the Dating and Radiochemistry Laboratory, Autonomous University of Madrid (Spain), has revealed a chronology of 70,556 ± 11,011 yr BP (Thermoluminescence [TL]) for the top of Level H4, and 56,157 ± 4389 yr BP (OSL) for Level H3. Dating at H2 has yielded diverse chronologies: 58,788 ± 4907 yr BP (TL) at the base and 30,221 ± 3636 yr (OSL) at the top (Navazo et al., Reference Navazo, Alonso-Alcalde, Benito-Calvo, Díez, Pérez-González and Carbonell2011). Fuente Mudarra is the largest and best-preserved upper Pleistocene site that has been dug to date in the Sierra de Atapuerca area.
Surveys, test pits, and excavations at several of the 30 documented Middle Palaeolithic sites show that they are enormous areas that were visited recurrently by Neanderthal groups for tens of thousands of years. These groups used all the environments, hillsides, valleys, and moors in the Pico River valley, which seems to have been the focus of these occupations.
The continuous presence of human groups in the Sierra de Atapuerca indicates that this has been an enclave rich in resources useful for the survival of the different groups that inhabited it (Carbonell et al., Reference Carbonell, Huguet, Cáceres, Lorenzo, Mosquera, Ollé, Rodríguez and Sala Ramos2014). In the archaeological record we observe similarities between the technological assemblages of the sites attributed to the lower levels of the middle and lower Pleistocene. In GIII, TD10.1, TD10.2 (see Fig. 2), and Fuente Mudarra levels (from level 8 to level 4) there is a tendency to standardize exploitation systems intended for predetermination. Centripetal knapping tends to dominate these assemblages (Ollé et al., Reference Ollé, Mosquera, Rodríguez, de Lombera-Hermida, García-Antón, García-Medrano and Peña2013).
Standardization is also observed in the retouched flakes, finding, above all, simple retouch that generate dihedral edges such as sidescrapers, denticulates, and points. And above all, uniformity is observed in the raw materials used; the Neogenous flint from the vicinity of the Sierra is almost always the most frequently used. ICretaceous flint (except in TD10.2, where is used more), quartzite, and sandstone are used in a more residual way.
Reviewing the upper Pleistocene sites on the Iberian Peninsula, we found caves such as Axlor (Rios-Garaizar, Reference Rios-Garaizar2017; Gómez-Olivencia et al., Reference Gómez-Olivencia, Sala, Núñez-lahuerta, Sanchis, Arlegi and Rios-Garaizar2018), El Castillo (Bernaldo et al., Reference Bernaldo, Neira, Maillo and Sala Ramos2014), Cueva Morín (Maillo-Fernández et al., Reference Maillo-Fernández, Arteaga, Iriarte-Chiapusso, Fernández, Wood, Bernaldo de Quiros and Sala Ramos2014), Esquilleu (Cuartero et al., Reference Cuartero, Alcaraz-Castaño, López-Recio, Carrión-Santafé and Baena-Preysler2015; Baena-Preysler et al., Reference Baena Preysler, Carrión Santafé, Torres Navas and Vaquero Rodríguez2019), Prado Vargas (Navazo and Díez, Reference Navazo and Díez2008), Arrillor (Iriarte-Chiapusso et al., Reference Iriarte-Chiapusso, Wood and Sáenz de Buruaga2019), Jarama VI (Jordá-Pardo et al., Reference Jordá-Pardo, Navazo, Díez and Sala Ramos2014), Gabasa (Montes and Utrilla, Reference Montes, Utrilla and Sala Ramos2014), Abric Romaní (Marín et al., Reference Marín, Saladié, Rodríguez-Hidalgo and Carbonell2017), La Arbreda (Lloveras et al., Reference Lloveras, Garcia, Maroto, Soler and Soler2018), Teixoneres (Rosell et al., Reference Rosell, Blasco, Rivals, Chacón, Arilla, Camarós and Rufà2017), Abric del Pastor (Machado et al., Reference Machado, Mayor, Hernández and Galván2019), Bolomor (Sañudo et al., Reference Sañudo, Blasco and Fernández Peris2016), Cova Negra (Martínez Valle et al., Reference Martínez Valle, Guillem Calatayud and Villaverde Bonilla2016), El Salt (Leierer et al., Reference Leierer, Jambrina-Enríquez, Herrera-Herrera, Connolly, Hernández, Galván and Mallol2019), Bajondillo (Cortés-Sánchez et al., Reference Cortés-Sánchez, Simón-Vallejo, Jiménez-Espejo, Lozano Francisco, Vera-Peláez, Maestro González and Morales-Muñiz2019), and Boquete de Zafarraya (Barroso et al., Reference Barroso, Caparrós, Barsky, Moigne, Monclova and Sala Ramos2014), among others, have shown several levels of Neanderthal occupation. However, most of them have been interpreted as short-term occupations separated by unoccupied gaps during which many of these caves were occupied bycarnivores. An excellent recent publication about Salt (Leierer et al., Reference Leierer, Jambrina-Enríquez, Herrera-Herrera, Connolly, Hernández, Galván and Mallol2019) goes so far as to state that sites in Europe and the Near East reflect short-term occupations. There are even authors who see occupation hiatuses in northern France and begin to talk about regional extinctions and recolonisations in western Europe (Roebroeks et al., Reference Roebroeks, Hublin, Macdonald, Ashton, Lewis and Stringer2011), backed by differences in the assemblages before and after MIS 4. This reality is different from the one seen in the Sierra de Atapuerca, which has a continuous occupation.
More similar to our case are the few Iberian examples of a single territory containing several sites with the same chronologies; this includes El Habario and El Esquilleu (Cuartero et al., Reference Cuartero, Alcaraz-Castaño, López-Recio, Carrión-Santafé and Baena-Preysler2015), the Pinilla del Valle sites (Márquez et al., Reference Márquez, Baquedano, Pérez-González and Arsuaga2016; Sánchez-Romero et al., Reference Sánchez-Romero, Canals, Pérez-González, Márquez, Mosquera, Karampaglidis, Arsuaga and Baquedano2017), Roca del Bous together with Cova Gran (Mora et al., Reference Mora, Martínez-Moreno, Roy Sunyer, Benito Calvo, Polo-Díaz and Samper Carro2018) and Cova de L'estret (Casanova et al., Reference Casanova, Mora, Roda, Martínez-Moreno, Roy, Vega and Sala Ramos2014), and Fuente Mudarra and the other Sierra de Atapuerca sites. Occupations at all these sites were short but recurrent and were generally associated with seasonal cycles and other nearby locations that are exactly the same. The land-use and occupation pattern of these groups consisted of a large, more or less invariable network of sites in their territory that were used for tens of thousands of years. These patterns suggest that the users were groups with close links to a certain territory, generating what are regarded as landscapes of habit (Gamble, Reference Gamble1999), but which in fact is the cultural heritage of successive generations who used the same space for many millennia.
CONCLUSIONS
The presence of Neanderthals in different contexts of the Sierra de Atapuerca area has been confirmed on the basis of the information gathered to date, both at open-air sites (Hundidero, Hotel California, Fuente Mudarra) and, with less impact, in cave sites (Galería de las Estatutas and Cueva Fantasma). Chronologies range from MIS 5 to 3, implying different environments that could be reflected in the sedimentary deposits.
The archaeological material associated with each of the levels at Fuente Mudarra shows that the raw material supply was stable throughout the entire sequence. Locally collected Neogene flint was the most frequently used material.
Technological analysis also found technological stability in the Fuente Mudarra sequence, without detectable diachronic variations. Knapping strategies were non-hierarchical and had one main objective: the recurrent production of secant removals from an intersection plane of two surfaces. The products were small and medium-sized, with anterior centripetal and chordal removals. The extremely infrequent retouched products tend to be sidescrapers and denticulates, varying in scarcely significant percentages, with Tayac points in Levels 4 and 8.
The Fuente Mudarra record is representative of Neanderthal occupational sequences. The entire Valhondo area around the Atapuerca karst zone benefited from the water sources and streams that flooded the Sierra slopes. This situation generated biotopes with powerful trophic gradients that were exploited recurrently by upper Pleistocene hunter-gatherers. The dynamics seem to have included visits and alternating short- and medium-duration occupations in areas that became part of the seasonal cycles and points of recurrent usage by these human populations, who regularly occupied these spaces. This suggests a humanized landscape in which the cave entrances that were not blocked during the upper Pleistocene also acted as an inducement to settle in the Pico River valley.
The occupational network produced horizontal palimpsests, with a high-resolution occupational model through the combination of two complementary types of occupation(in open-air and cave sites). This type of occupation was probably a continuation of the trend at the end of the middle Pleistocene that has been recorded in the upper levels of the Gran Dolina, Galería, and Sima del Elefante sites.
We therefore propose a continuous occupation with many similar features between the late middle Pleistocene and upper Pleistocene populations.
ACKNOWLEDGMENTS
This work was supported by the Spanish Ministry projects CGL2012-38434-C03-02, CGL2015-65387-C3-3-P, and PGC2018-093925-B-C31. The field excavation work was supported by the Junta de Castilla y León and Fundación Atapuerca. Marta Santamaría is the beneficiary of a predoctoral grant from the University of Burgos. Hardware and software (Agisoft Photoscan) at the Digital Mapping and 3D Analysis Laboratory (CENIEH) were used for the terrestrial photogrammetry. Textural analysis was done by Leticia Miguèns at the Geology Laboratory. X-Ray Diffraction (XRD) and X-Ray Fluorescence (XRF) analysis was done by Ana Álvaro Gallo at the Archaeometry Laboratory (CENIEH).
SUPPLEMENTARY MATERIAL
The supplementary material for this article can be found at https://doi.org/10.1017/qua.2020.65