Introduction
As the global human population grows, farmers not only need to meet demands for increasing food quantity, but also for food quality and nutrition. Nutrient densities (concentrations of minerals, vitamins and proteins) of grains, fruits and vegetables produced in the USA and UK have been declining in the past 50–70 years (Jarrell and Beverly, Reference Jarrell and Beverly1981; Davis et al., Reference Davis, Epp and Riordan2004; Davis, Reference Davis2009). The seminal paper by Jarrell and Beverly (Reference Jarrell and Beverly1981) increased awareness of the negative consequences of a highly mechanized food production system. Davis (Reference Davis2009) clarified this work and suggested nutrient declines were driven by yield increases via varietal differences (new genetics, selected for economic characteristics) and to a lesser degree improved crop management practices, but admitted there were few well-designed comparative studies upon which to draw definitive conclusions. Conventional methods have often been associated with depletion of soil nutrients and decrease in soil organic carbon and soil biota, which can impact nutrient availability (Douds et al., Reference Douds, Janke and Peters1993; Douds et al., Reference Douds, Galvez, Janke and Wagoner1995; Hepperly et al., Reference Hepperly, Seidel, Pimentel, Hanson and Douds2007; Reganold et al., Reference Reganold, Andrews, Reeve, Carpenter-Boggs, Schadt, Alldredge, Ross, Davies and Zhou2010) even though extensive use of agro-chemicals in conventional farming has been credited for increased crop yields around the globe (Pang and Letey, Reference Pang and Letey2000). Lower nutrient density has the potential to impact population health and crop profitability. While several studies have shown higher mineral, vitamin, protein or phytonutrient concentrations in organic foods (Brandt and Mølgaard, Reference Brandt and Mølgaard2001; Heaton, Reference Heaton2001; Worthington, Reference Worthington2001; Williams, Reference Williams2002; Lairon, Reference Lairon2010; Reganold et al., Reference Reganold, Andrews, Reeve, Carpenter-Boggs, Schadt, Alldredge, Ross, Davies and Zhou2010; Benbrook et al., Reference Benbrook, Butler, Latif, Leifert and Davis2013; Barański et al., Reference Barański, Średnicka-Tober, Volakakis, Seal, Sanderson, Stewart, Benbrook, Biavati, Markellou and Giotis2014), this claim has also been challenged (Woese et al., Reference Woese, Lange, Boess and Bögl1997; Brandt and Mølgaard, Reference Brandt and Mølgaard2001; Williams, Reference Williams2002; Dangour et al., Reference Dangour, Dodhia, Hayter, Allen, Lock and Uauy2009; Barański et al., Reference Barański, Średnicka-Tober, Volakakis, Seal, Sanderson, Stewart, Benbrook, Biavati, Markellou and Giotis2014), either finding no difference in separate studies or questioning the methodology of the research. Until now, few studies have compared the nutritional quality of organic and conventional foods grown in the same soil under the same set of environmental conditions in a replicated, side-by-side trial (Mitchell et al., Reference Mitchell, Hong, Koh, Barrett, Bryant, Denison and Kaffka2007; Ren et al., Reference Ren, Reilly, Kerry, Gaffney, Hossain and Rai2017). Even fewer studies have simultaneously measured soil and nutritional quality on organic and conventional farms (Reganold et al., Reference Reganold, Andrews, Reeve, Carpenter-Boggs, Schadt, Alldredge, Ross, Davies and Zhou2010), and data on the relationship between soil health indicators and crop nutrient densities are scarce. As a shift is made from an emphasis on yield to an emphasis on producing nutritionally dense crops, there is a need to examine which indicators best predict soil health within the context of nutrient quality and the practices that will allow growers to reach this management goal.
Grains, including oats, constitute a major component of the US dietary recommendations that include six ounces of grains per day (McGuire, Reference McGuire2011; Chu, Reference Chu2013). Oats rank seventh as the most economically important cereal after corn, rice, wheat, barley, millet and sorghum and are both important as animal feed and human consumption (Zwer, Reference Zwer2004; Boczkowska et al., Reference Boczkowska, Podyma, Łapiński, Singh and Upadhyaya2016). It has been estimated that between 50 and 90% of the world oat production is used to feed animals, including horses, cattle, sheep and poultry (Zwer, Reference Zwer2004; Andersson and Börjesdotter, Reference Andersson and Börjesdotter2011; Boczkowska et al., Reference Boczkowska, Podyma, Łapiński, Singh and Upadhyaya2016). In human consumption, oat grains are used to produce many traditional, functional and medicinal products. Unlike other grains, the entire kernel after the hull is removed and utilized for many food products, such as oatmeal, hot cereals, bread, biscuits, infant food, and muesli or granola bars among others (Zwer, Reference Zwer2004; Andersson and Börjesdotter, Reference Andersson and Börjesdotter2011). Reported health benefits of oat grains include lowering blood cholesterol, normalizing blood glucose levels and reducing the risk of colorectal cancer (Zwer, Reference Zwer2004).
Oat grains have a high concentration of many valuable nutrients, including soluble fibers (β-glucans), proteins, unsaturated fatty acids, vitamins, minerals and antioxidants (Chu, Reference Chu2013; Rasane et al., Reference Rasane, Jha, Sabikhi, Kumar and Unnikrishnan2015). The protein quality and content of oat grains are substantially higher than in other cereal grains (Klose and Arendt, Reference Klose and Arendt2012; Chu, Reference Chu2013). While nitrogen fertilization can affect protein concentrations in most grains (Woese et al., Reference Woese, Lange, Boess and Bögl1997; Worthington, Reference Worthington2001; Mäder et al., Reference Mäder, Hahn, Dubois, Gunst, Alföldi, Bergmann, Oehme, Amadò, Schneider and Graf2007; Casagrande et al., Reference Casagrande, David, Valantin-Morison, Makowski and Jeuffroy2009), studies have shown that amino acid composition in oat grains is not as easily influenced by high nitrogen fertilization than other cereal grains (Lasztity, Reference Lasztity1998; Chu, Reference Chu2013). Compared to other cereal grains, oats have also been shown to have relatively higher fat content, containing linoleic and linolenic acid, both essential fatty acids (Lasztity, Reference Lasztity1998; Chu, Reference Chu2013). It has been reported that organically grown small grains in Europe have higher protein quality implying a higher concentration of essential amino acids (Worthington, Reference Worthington2001), but this was not corroborated by Mäder et al. (Reference Mäder, Hahn, Dubois, Gunst, Alföldi, Bergmann, Oehme, Amadò, Schneider and Graf2007), who found higher protein content in wheat grown in conventional farming systems compared to wheat from organic farming systems. We are, however, not aware of similar research results attributed specifically to oats reported in the mid-Atlantic region of the USA.
The primary objective of this project was to assess the effects of long-term organic and conventional grain cropping systems and tillage practices on oat grain nutrient profiles, including minerals, crude protein, β-glucans, and lipid-soluble (vitamin E and tocopherols) and water-soluble vitamins (thiamin, riboflavin, B6 and folic acid). The study also assessed soil minerals concentrations in order to determine potential connections between soil and grain nutrients.
Materials and methods
A study was established at Rodale Institute's FST research site in 2014 to assess the effect of long-term organic and conventional grain cropping systems and tillage practices on the nutrient density of oat grains. The Rodale Institute's Farming Systems Trial (FST) was established in 1981 to quantify the effects of long-term organic and conventional grain cropping systems and tillage practices. The FST is a rainfed (dryland) replicated 6.1-ha, 24-main-plot trial located at Rodale Institute, in Berks County, Pennsylvania (40°37′97″N and 40°75′98″W) with an average rainfall of 1198 mm, and mean temperature of 10.2°C. Soil is a moderately well-drained Comly silt loam with a neutral pH of 6.8. Between 1981 and 2007, eight replicates of each of the three cropping systems were managed by tillage (Liebhardt et al., Reference Liebhardt, Andrews, Culik, Harwood, Janke, Radke and Reiger-Schwartz1989; Lotter et al., Reference Lotter, Seidel and Liebhardt2003; Pimentel et al., Reference Pimentel, Hepperly, Hanson, Douds and Seidel2005; Ryan et al., Reference Ryan, Smith, Mortensen, Teasdale, Curran and Seidel2009). The three cropping system treatments included in the trial during this period included: (1) conventional system with synthetic nutrient sources (CNV), (2) organic system with legume cover crops (LEG), and (3) organic system that combined legume cover crops, perennial hay phase and periodic application of composted dairy manure (MNR). Major grain cash crops included in the rotation were corn (Zea mays), wheat (Triticum aestivum), soybean (Glycine max) and oat (Avena sativa). Mixed perennial orchardgrass (Dactylis glomerata) and alfalfa (Medicago sativa) were included in the MNR system. The most prevalent winter cover crops used included medium red clover (Trifolium pratense), hairy vetch (Vicia villosa) and cereal rye (Secale cereale). Consequently, a 3-year rotation (corn/rye − corn/rye − soybean/rye) was followed in the CNV system; a 4-year rotation (corn/rye − oats/clover and rye − soybean/wheat − wheat/vetch) followed in the LEG system, and an 8-year rotation (soybean/wheat − wheat/hay − hay − hay − corn silage/wheat − wheat/vetch − corn/rye − oat/rye) followed in the MNR system. In each system, N inputs were applied at equivalent available rates for corn. These inputs included: composted cattle manure and legume green manure and rolled down mulch in the MNR system; legume green manure and rolled down mulch in LEG system (red clove of hairy vetch) and ammoniated fertilizer in the CNV system (Hepperly et al., Reference Hepperly, Seidel, Pimentel, Hanson and Douds2007). About 168 kg N ha−1 were applied to corn in the CNV system to achieve a grain yield of 9.4 Mg ha−1. Mineral fertilizer was generally applied as a split application of starter fertilizer, including 33.6 kg ha−1 N and P, and 11.2 kg K ha−1 at planting, and 134.5 kg N ha−1 as side-dress application in the form of urea ammonium nitrate (UAN) when corn plants were about 30 cm tall. No fertilizer was applied to oats and soybeans in the CNV system, but 67 kg N ha−1 side-dress was applied to wheat as UAN. In the MNR system, composted manure was only applied every 2–3 years depending on crop rotation, typically prior to planting oats or corn silage only. Composted manure application rate was determined based on a target input of 89.7 kg N ha−1, and the assumption that there was at least 40% residual N in the soil provided by legume green manure or roll-down, and SOM accumulation over the long duration of the experiment.
In 2008, half of the replicates for each cropping system were converted to N.T. This study was a two-factor split-plot randomized complete block design with four replicates, each located within the FST's established NT or T replicates, accordingly. The smallest experimental unit, the split-plot, measured 6 m wide by 91 m long. The first factor (main) consisted of cropping systems with three levels: two organic and one conventional system. The two organic systems are the MNR and LEG. Due to proximity to the conventional systems, the two organic systems are not certified organic, but organically managed. Samples are collected from the middle rows of each plot, thereby minimizing any effects or contamination from the conventional plots. The CNV is a synthetic input-based system representative of nearly 90% of American farmland. The CNV is designed to exemplify the suite of recommended best crops and management practices adopted by the majority of grain growers in our region, including the use of synthetic fertilizers and pesticides and genetically modified crops.
The second factor (split) consisted of tillage practices with two levels: tilled (T) and no-till (NT). Tillage practices common to all three systems include plowing to a depth of 20 cm, disking and packing (or firming to increase seed to soil contact) before planting crops. Plowing is accomplished by an inversion moldboard in the MNR-T and LEG-T systems, and by a vertical chisel plow in the CNV-T system. During the cropping season, weeds are managed by cultivation (several passes a season) in MNR-T and LEG-T, while herbicides are used in CNV-T. No-till organic systems (MNR-NT and LEG-NT) utilize cover crops and the no-till roller/crimper, while CNV-NT uses the same cover crops terminated by synthetic herbicides prior to planting; these strategies also contribute to weed control. The NT organic systems utilize a roller/crimper implement to terminate established cover crops. This method allows cover crop roots to remain in the soil and compresses above-ground plant residue to create a mulch into which large-grained cash crops (corn and soybeans) are planted. Unlike the conventional no-till systems, organic no-till systems are not continuous no-till systems as fall-establishment of cover crops that are terminated in spring by the roller/crimper to create the mulch for weed control, as well as the establishment of other small grains in the rotation, still require tillage. For that reason, the system is more accurately referred to as rotational no-till (Mirsky et al., Reference Mirsky, Curran, Mortensen, Ryan and Shumway2009; Mirsky et al., Reference Mirsky, Curran, Mortenseny, Ryany and Shumway2011; Mirsky et al., Reference Mirsky, Ryan, Curran, Teasdale, Maul, Spargo, Moyer, Grantham, Weber, Way and Camargo2012; Mirsky et al., Reference Mirsky, Ryan, Teasdale, Curran, Reberg-Horton, Spargo and Moyer2013; Wallace et al., Reference Wallace, Williams, Liebert, Ackroyd, Vann, Curran, Keene, VanGessel, Ryan and Mirsky2017).
Each cropping system by tillage practice is further subdivided into three equal sub-plots, referred to as points of entry, measuring 6 by 91 meters for a total of 72 sub-plots (from the original 24 main plots). Entry point sub-plots provide an opportunity to test three different crops within one system. Given that crop rotations in organic systems are longer and more diverse than in conventional systems, entry point sub-plots provide the flexibility to include at least one cash crop from time to time in all cropping systems by tillage treatments to facilitate statistical comparison between the systems. Samples for this study were collected from one of the three points of entry to be consistent with a rotation sequence unique to that sub-plot.
In 2014, all FST plots were planted to oats to update upcoming rotations to reflect current trends in agriculture. This provided an ideal opportunity to compare grain nutrient density differences between all three cropping systems by tillage practices (MNR, LEG and CNV between T and NT). The ‘Esker’ variety of oats were planted at 108 kg ha−1 using a John Deer (MaxEmergeTM 5e Row Unit, 1995) grain drill on March 20, 2014 in seedbeds prepared by tillage as described above for each system. Although weed sampling and weed data analysis were not undertaken, substantial weed pressure was observed in all plots in all systems. Soil and oat grain samples were taken in one representative entry point per system (split-plot) for a total of 24 samples for each soil or grain parameter tested. Oat grains were harvested on August 14 in 2014 from the middle two rows of each plot using a plot combine harvester (Massey Ferguson model 8XP, equipped with HarvestMaster high capacity GrainGage, HM 800 System, Juniper Systems Inc. Logan, UT, USA).
Oat grains were winnowed and cleaned using a 2-mm screen and divided equally into two subsamples for analysis. One oat subsample was sent to the Nutritional Biochemistry Laboratory of Drexel University's Department of Nutrition Sciences for vitamin and antioxidant analysis as follows. Fat-soluble vitamin (tocopherol vitamin E) was determined by high pressure liquid chromatography (HPLC) using a Phenomenex, Kinetex 2.6 μm F5 100 A, LC Column 150 × 4.6 mm, with a mobile phase of 1% isopropanol in hexane and a flow rate of 1 ml min−1. The vitamin E content (E-α, E-β, E-γ and E-δ) in oat grain samples was calculated in mg/100 g of sample, and E-total was calculated as the summation of all vitamin E content together. Water-soluble vitamins were also analyzed using HPLC mobile phase with a fluorescence detector (Shimadzu fluorescence detector, RF-20A).
The second oat grain subsample was sent to Cornell University Nutrient Analysis laboratory for mineral and protein (crude, soluble and digestible) analysis (G01 Bradfield Hall, Ithaca, NY 14853, USA). Minerals including Al, arsenic (As), beryllium (Be), Ca, cadmium (Cd), cobalt (Co), Cr, copper (Cu), Fe, potassium (K), lithium (Li), magnesium (Mg), manganese (Mn), molybdenum (Mo), sodium (Na), nickel (Ni), phosphorus (P), lead (Pb), S, selenium (Se), Sr, (titanium) Ti, vanadium (V) and Zn were analyzed using Inductively Coupled Plasma Spectroscopy method 6010 (USEPA, 1986), while total C, N and S were determined by combustion analysis (Matejovic, Reference Matejovic1993). The third set of oat samples was sent to Missouri State University (University of Missouri—Columbia, Office of the Missouri State Chemist, Analytical Services, Rm 4 Agriculture Bldg., 700 Hitt Street, Columbia, MO 65211) for crude protein and amino acid profile analyses.
Soil samples up to 100 cm depth were collected after crop harvest using the Giddings deep core soil probe (Giddings Machinery Co, Windsor, Colorado, USA). Four deep cores were taken per sample and split into 0–10, 10–20, 20–30, 30–60 and 60–100 cm depths. The four cores at each depth were then thoroughly mixed, clods broken and visible plant material was removed prior to dividing samples for analyses. For this study, a portion of 0–10 cm air-dried sample was sent to the Cornell University Nutrient Analysis Laboratory (G01 Bradfield Hall, Ithaca, NY 14853, USA) for total elemental analysis as well as total C, N and S. Minerals were extracted from the soil samples using the DTPA extraction method coupled with ICP spectrometry (Lindsay and Norvell, Reference Lindsay and Norvell1978) while SOM was determined using the ‘loss of weight on ignition’ method (Nelson and Sommers, Reference Nelson and Sommers1996) and soil moisture was determined by gravimetric method (Black, Reference Black1965).
Data analysis
Oat grain nutrient and soil quality parameters were subjected to analysis of variance (ANOVA) using the MIXED procedure of SAS (SAS 9.4, SAS Institute Inc., Cary NC, USA, 2014) at a 5% significance level, unless identified otherwise. Split-split plot factor was not considered in the analysis as only one of the three points of entry was sampled. The effect of tillage (T or NT) and cropping systems (CNV, MNR or LEG) were considered fixed effects, while blocks were considered as random effects. Significant differences between classification variables were analyzed using Tukey's adjusted multiple comparison procedure by Tukey-Kramer grouping in PROC GLIMMIX in SAS version 9.4 (SAS, 2014). Treatment differences were considered significant at P < 0.05. The figures were plotted using Sigmaplot version 14.0 (SigmaPlot, 2018). The systems were abbreviated in the figures and tables as following: organic manure (MNR), conventional (CNV), organic legume (LEG), till (T) and no-till (NT).
Results
There was a significant cropping system by tillage interaction (P < 0.05) for oat Mg, Zn, S and P; and a marginally significant system by tillage interaction (P ≤ 0.1) for Na and Mn in oat grains (Table 1). However, post-hoc separation (Tukey-Crammer) revealed that, (1) Na is not significant up to P = 0.167, and thus Na was removed from visual presentation, and (2) Mo was significant at P < 0.05 although overall ANOVA suggested differently (Table 1), and thus data for those minerals were analyzed, and presented separately by cropping system and tillage (Figs. 1A and 1B). It is not unusual to have a non-significant F-test and a significant pairwise comparison result, or vice versa. The possible reasons for causing this discrepancy could include multiple testing problem, insufficient sample size to detect a difference, unbalanced data or presence of covariates in the model. In other words, the P-value in the F-test and the multiple comparison results are based on different methodologies and can occasionally produce contradictory results. For example, it is possible that the F-test P-value can indicate that there are no differences between the means while the multiple comparisons output indicate that some means are different. In this case, it is generally agreeable to trust the multiple comparisons output. Although the field variables are limited to soil and oat grain samples, there are other covariates that may have been present during the experiment but not accounted for, including slope of the landscape, mechanical or chemical damage, seeding rate errors, other environmental variables, or micro-site conditions variability within field plots sampled. However, sample size was sufficient, and the data were balanced, confirming that, although a lack of some covariates was possible, the overall study was robust and provides a degree of confidence in the data.

Fig. 1. (A) Mg, S, P; and (B) Mn, Zn, Mo concentrations in oat grains under organic and conventional grain cropping systems at the Farming Systems Trial. LEG, organic legume; MNR, organic manure; CNV, conventional; T or NT, tilled or no-till. (Please note that the scales are different for various minerals.)
Table 1. Analyses of variance (ANOVA) to test the effects of (i) cropping system (S), (ii) tillage (T) and their interaction on the concentrations of various minerals in oat grains grown under organic and conventional grain cropping systems at the Farming Systems Trial

The concentrations of significant or marginally significant grain minerals are presented in Figure 1, and the concentrations of all other oat grain minerals are listed in Supplemental Table 1. Tilled MNR and CNV systems had greater P and Mg concentrations than no-till CNV and LEG systems. However, there were no differences for P and Mg between tilled MNR and CNV systems and the other cropping systems by tillage treatments (Fig. 1). The concentration of S was lowest in CNV-NT system, but S was similar among the remaining treatments. The Zn concentration was highest in CNV-T system but not different among the other treatments. While there was no cropping system by tillage interaction for Mo, cropping systems had a significant effect on Mo (P < 0.0001). Although Mn was greater (P = 0.012) in CNV (45.72 mg kg−1) and LEG (46.20 mg kg−1) compared to MNR (44.1 mg kg−1), the opposite was true for Mo, where Mo concentration was greater in CNV (0.885 mg kg−1) and MNR (0.956 mg kg−1) compared to LEG (0.424 mg kg−1) (Fig. 1B). There were no statistical differences between cropping systems or tillage for all the other oat grain minerals analyzed.
There was a system by tillage interaction for soil moisture, Cd, Ni, S and Zn and marginally significant (P = 0.065) system by tillage interaction for soil Ti; hence data for these minerals were analyzed separately by system and tillage (Table 2). Once again, the LEG no-till system tended to have the greatest concentration of each of those elements, and CNV-NT the lowest (Table 2).
Table 2. Mineral concentration in soil under organic and conventional grain cropping systems under two tillage regimes at the FST in 2014

LEG, organic legume; MNR, organic manure; CNV, conventional; T or NT, tilled or no-till. Means within a column followed by the same letter are not significantly different (LSD, α = 0.05).
There was no system by tillage interaction for soil Al, Mg, P, Fe, Ca, B, Cr, Mo, Sr and Ti; hence, data were combined for analysis by system or tillage effect (Table 3). The LEG had greater concentrations of Al, Fe and to some extent, Cr. The MNR system also had greater concentrations of three more of these minerals (Ca, Bo and Sr). The CNV system had a significant effect on only Mg and Cr concentration, but the difference was not significant between the CNV system and MNR and LEG systems, respectively. The other soil minerals are presented in Supplemental Table 2.
Table 3. Soil mineral concentration in oat plots grown under organic and conventional grain cropping systems at the FST in 2014

LEG, organic legume; MNR, organic manure; CNV, conventional. Means within a column followed by the same letter are not significantly different (LSD, α = 0.05).
There was a cropping system by tillage interaction for all non-essential (Table 4) and essential amino acids (Table 5) (Heger, Reference Heger and D’Mello2003; Vilmane et al., Reference Vilmane, Zute, Straumīte and Galoburda2015) in oat grains, and thus data were separated by cropping system and tillage for analysis. Cropping systems had a highly significant effect (P < 0.02) in all amino acids analyzed. Tilled systems tended to have higher amino acids than no-till systems within each cropping system by tillage main plots. The concentration of all nine essential amino acids was greater in LEG-T and NT systems compared to all the other systems except lysine, histidine and methionine concentration that were not significantly different between the LEG systems and CNV-T system. Tilled organic legume system had the highest concentration of all amino acids, and conventional no-till had the lowest concentration of the same nutrients.
Table 4. Non-essential amino acids in oat grains grown under organic and conventional grain cropping systems at the Farming Systems Trial in 2014

LEG, organic legume; MNR, organic manure; CNV, conventional; T or NT, tilled or no-till. Means within a column followed by the same letter are not significantly different (LSD, α = 0.05).
Table 5. Essential amino acids in oat grains grown under organic and conventional grain cropping systems at the Farming Systems Trial in 2014

LEG, organic legume; MNR, organic manure; CNV, conventional; T or NT, tilled or no-till. Means within a column followed by the same letter are not significantly different (LSD, α = 0.05).
There was no cropping system by tillage interaction for oat vitamins (Table 6). Organic LEG had significantly greater vitamin B1 (0.50 mg kg−1) than MNR (0.43 mg kg−1) and CNV (0.42 mg kg−1). Given that cropping systems and tillage had a significant interaction effect on total amino acid (determined by summation of all the analyzed amino acids) and crude proteins, and marginally significant effects (P = 0.099) on vitamin B6; data for these nutrients were reported separately by tillage and cropping systems (Figs. 2 and 3). Although the LEG management system tended to have greater total amino acids and crude protein concentration than the other treatments, these nutrients were not significantly different between CNV-T and all organic treatments. However, total amino acids and crude proteins were greater in LEG system compared to CNV-NT system (Fig. 2). This trend was reflected in essential- (Table 5) and non-essential (Table 4) amino acids. Both T and NT LEG increased all essential amino acids in oat grains. However, the increase was not significant for tyrosine, lysine and arginine between LEG and CNV-T, and ornithine between LEG, CNV-T and MNR-NT (Table 5). Similarly, both LEG systems increased 12 out of the 13 non-essential amino acids analyzed in oat grains (Table 4). In each case, NT-CNV had the lowest essential and non-essential amino acids except for taurine, which was greater in CNV-T although the difference was not significant in comparison with LEG-T and CNV-NT. Taurine concentration was lowest in MNR-T and LEG-NT.

Fig. 2. The impact of cropping system by tillage on proteins and total amino acids in oat grains grown under organic and conventional grain cropping systems at the Farming Systems Trial in 2014. Means followed by the same letter are not significantly different (LSD, α = 0.05).

Fig. 3. The impact of cropping system by tillage on vitamin B1, B2 and B6 in oat grains grown under organic and conventional grain cropping systems at the Farming Systems Trial in 2014. Means followed by the same letter are not significantly different (LSD, α = 0.05).
Table 6. Analyses of variance (ANOVA) to test effects of cropping system (S) and tillage (T) and their interaction on oat vitamins and proteins in oat grains grown under organic and conventional grain cropping systems at the Farming Systems Trial in 2014

While the CNV-NT system had greater vitamin B6 than all the other treatments, there was no statistical difference in vitamin B6 concentration between CNV-NT and MNR-NT (Fig. 3). Vitamin B1 concentration was highest in both LEG systems and lowest in CNV-NT system, but there was no significant difference in vitamin B1 between CNV-T, LEG and MNR systems (Fig. 3).
Tillage increased total amino acids and crude proteins but reduced vitamin B6. Tilled systems had 6.8% more crude protein and total amino acids than no-till systems, while no-till systems had 6.6% greater vitamin B6 than tilled systems (data not shown).
This study also found no interaction between cropping systems and tillage for total soil C and N primarily required for amino acid, therefore protein, synthesis and total sulfur, which also plays an important role in protein synthesis (Bromke, Reference Bromke2013). Data for the three minerals were thus combined for analysis (Table 7). The percent concentrations of total C, S and N were significantly greater in MNR system compared to the CNV systems (Fig. 4) but the difference was not significant in comparison with LEG system. Percent C, S and N also tended to be greater in LEG compared to CNV system, although the difference was not significant (Fig. 4).

Fig. 4. The impact of tillage on soil C, N and S in oat grains grown under organic and conventional grain cropping systems at the Farming Systems Trial in 2014. Means within a nutrient followed by the same letter are not significantly different (LSD, α = 0.05).
Table 7. Analyses of variance (ANOVA, P < 0.05) to test the effects of cropping system (S) and tillage (T) and their interaction on soil nitrogen (N), carbon (C) and sulfur (S) in oats grown under organic and conventional grain cropping systems at the Farming Systems Trial in 2014

Correlation coefficient analyses indicate that the correlation of soil N, C and S with amino acids and/or protein content in oat grains was greater in the organic systems compared to the conventional system (Table 8). Soil N positively correlated with only 20% total amino acids in CNV compared to 85 and 86% in the LEG and MNR, respectively. Soil C's correlation with total amino acids in CNV could not be detected by this analysis. However, soil C was 52 and 71% positively correlated to total amino acids in LEG and MNR respectively. Soil S was only 4% positively correlated to total amino acids in CNV compared to 74 and 67% in the LEG and MNR respectively. The relationship between total amino acids and protein content of oat grains is presented in Supplemental Figure 1.
Table 8. Correlation coefficients (r) between oat grain amino acids, protein and soil key elements
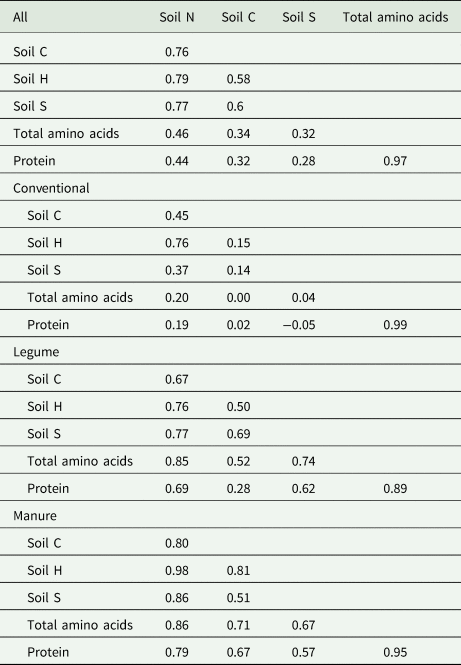
There was no statistical difference between treatments for soil organic matter. There was also no difference between treatments for soil moisture except for MNR-NT, which had the greatest soil moisture percentage (P = 0.0372) (Table 2). The other organic tilled plots and the CNV-NT plots lower soil moisture compared to MNR-NT.
In general, oat grain yields were very low compared to the country average, attributed to the extensive weed pressure observed in all plots in all systems during the study. Although the LEG system tended to have the greatest yield, yield differences between treatments were only marginally significant at P < 0.1 with CNV-NT having the lowest yield and no statistical difference between the other treatments.
Discussion
Soil and grain mineral chemical composition
Data on mineral concentration of oat grains are scarce because most studies have tended to focus on the nutritional quality of oat grain rather than its mineral composition (Pomeranz et al., Reference Pomeranz, Robbins and Briggle1971; Kim et al., Reference Kim, Kim, Abuel, Kwon, Shin and Ko2006; Micek et al., Reference Micek, Kulig, Woźnica and Sajdak2012; Gronle et al., Reference Gronle, Lux, Böhm, Schmidtke, Wild, Demmel, Brandhuber, Wilbois and Heß2015; Vilmane et al., Reference Vilmane, Zute, Straumīte and Galoburda2015). A novel aspect of the present study is that oat grain mineral concentrations were simultaneously evaluated and related to soil fertility and vital nutrients such as amino acids, proteins and vitamins in side by side comparisons of established organic and conventional management systems, each under both tilled and no-till treatment strategies.
The uptake and utilization of soil minerals by plants depend on many factors, including the plants’ morphological characteristics, environmental and climatic conditions, as well as soil biological, physical and chemical properties (Clarkson, Reference Clarkson1985; Sauerbeck and Helal, Reference Sauerbeck, Helal, Gabelman and Gabelman1990; Marschner, Reference Marschner2011). Thus, the mineral content of the soil does not always correlate directly with the mineral content of plant tissues (Marschner, Reference Marschner2011; Zu et al., Reference Zu, Li, Yang, Yu, Sun, Tang, Yost and Wu2014). Results from the current study are consistent with Worthington (Reference Worthington2001), who reported that only Fe, Mg and P concentrations of organically grown fruits, vegetables and grains differed from and were significantly greater than those grown under conventional management. Oat grain nutrient analyses of the current study revealed that most of the mineral concentrations were not significantly different between cropping systems or tillage treatments (Table 1, Supplemental Table 1), except for Mg, P, Mn, Zn, Mo and S (Fig. 1). The concentrations of these six minerals were either comparable between organic and conventional systems or significantly greater in organic systems. Greater oat grain mineral P and Mg in tilled MNR and CNV systems compared to no-till CNV and LEG systems, and greater concentration of Zn in CNV-T system suggest that tillage may have enhanced the uptake of these nutrients. However, periodic tillage in rotational no-till organic system renders this interpretation unlikely. More likely composted manure, known to have moderate to high levels of P, periodically added to the MNR system and mineral fertilizers regularly added to the CNV system, both incorporated into the soil by tillage may explain the higher concentration of these nutrients in oat grains (Fig. 1). Additional studies are required to determine the contribution of various fertilizer inputs combined with tillage on soil and tissue mineral availability.
Results from this study revealed that the concentration of oat grain S was lowest in CNV-NT system but not significantly different among the other treatments (Fig. 1A; Table 2), while the concentration of soil S was also lower in both T and NT CNV systems compared to both organic systems under T or NT (Table 7). The main sources of S for plant uptake include S applied as fertilizer and mineralization of soil organic matter (Camberato and Casteel, Reference Camberato and Casteel2017). Elemental S and other forms of S present in soil organic matter and some fertilizers must first be converted to sulfate (SO4−2) for plant uptake (Hodges, Reference Hodges2010; Camberato and Casteel, Reference Camberato and Casteel2017). Therefore, lower soil organic matter content would likely result in S deficiency. Results from this study found no differences in soil organic matter between treatments, which could partly be attributed to smaller sample size as only one point of entry was sampled for analysis (24 out of 72 plots). However, long-term data from the FST have revealed that soil organic matter content in the organic systems has been historically greater than the conventional systems (Drinkwater et al., Reference Drinkwater, Wagoner and Sarrantonio1998; Pimentel et al., Reference Pimentel, Hepperly, Hanson, Douds and Seidel2005; Seidel et al., Reference Seidel, Moyer, Nichols and Bhosekar2017). Drinkwater et al. (Reference Drinkwater, Wagoner and Sarrantonio1998) reported soil C levels at the FST increased by ~1000 g m−2 over a 14-year period, while increases in soil C in CNV systems were not observed for that same period. Soil water-holding capacity, infiltration rate and water capture efficiency were also significantly greater in organic LEG compared to CNV system (Drinkwater et al., Reference Drinkwater, Wagoner and Sarrantonio1998). In spite of the smaller sample size in the current study, soil moisture content was greater in MNR-NT compared to the two tilled organic systems (MNR-T and LEG-T) and CNV-NT (Table 2). Higher soil moisture in MNR-NT, which was not statistically different from LEG-NT and CNV-T, partly explains the suitable soil conditions that likely helped to increase the availability of S in the organic systems. Conversion of organically bound S to sulfate involves a biological oxidation process (mineralization) facilitated by Thiobacillus bacteria (Hodges, Reference Hodges2010) suggesting that soil conditions need to be warm, moist and well-drained to facilitate the process. Microbial activity can be reduced by cold and excessively wet or dry conditions, thus reducing S availability from soil organic matter (Camberato and Casteel, Reference Camberato and Casteel2017). Increased adoption of no-till practices and heavy residue from high-yielding crops has been implicated as important factors exacerbating S deficiency (Camberato and Casteel, Reference Camberato and Casteel2017). Significantly lower S in CNV-NT, therefore, is attributed to continuous no-till practice in CNV-NT compared to rotational NT in organic systems and lower soil organic matter in studied CNV system. This might also explain current study results that illustrated the tendency for CNV-NT to have lower concentrations of soil micronutrients that were negatively impacted by significant tillage and cropping systems interaction effects (Table 2). These tillage and cropping system interaction effects were significant in only Ni, S, Zn and Ti, with LEG-NT tending to have the greatest concentration of these micronutrients and NT-CNV tending to have the lowest (Table 2).
In theory, increasing soil nutrient content would generally result in an increase in crop nutrient uptake. However, the interrelationships between nutrients in the plant system are complex and interdependent (Marschner, Reference Marschner2011). Plants require balanced nutrition analogous to the human requirement for a balanced diet with adequate proportions of carbohydrates, proteins, vitamins, minerals, fats and water (Ranade-Malvi, Reference Ranade-Malvi2011). Efficient uptake and utilization of minerals by plants involve specific synergistic and antagonistic pathways among nutrients (Marschner, Reference Marschner2011). For example, sufficient levels of Ca and Zn improve the uptake of P and K, while excessive levels of P and N can reduce the uptake of micronutrients such as Fe, Zn, Mn and Cu (Ranade-Malvi, Reference Ranade-Malvi2011). In the current study, Mn concentration in oat grains was significantly greater in CNV and LEG compared to MNR, and oat Mo concentration was significantly greater in CNV and MNR compared with LEG (Figs. 1A and 1B), which suggests mineral interactions between the two trace elements.
Soil mineral concentrations in this study were similar between cropping systems and between tillage treatments (Supplemental Table 3). For ten of these minerals, concentrations were influenced by significant cropping systems effects, with no-tillage effects (ANOVA, Table 3). Organic systems had greater concentrations of six of those, including Al, Fe and Cr which were greatest in LEG; and Ca, B and Sr which were greater in MNR compared to CNV (Table 3). Given that the influence of CNV was observed only for Mg and Cr, and that this influence was not significant between CNV and either organic system, these combined results suggest that organic systems increased the bioavailability of more minerals compared to the conventional system. Thus, while our study revealed that organic systems improved the availability of key minerals, further research is required to elucidate the complicated relationships among essential minerals, and how management systems affect those relationships.
Oat grain proteins and soil carbon, nitrogen and sulfur
The concentration of total amino acids ranging between 7.5 and 10 g 100 g−1 and crude protein ranging between 8.65 and 11.12 g 100 g−1 in oat grain in the current study (Fig. 2) were within the range of a recent study that reported amino acid and protein concentration in oat grain ranging between 9.3–13.2 and 11.3–17.7 g 100 g−1 respectively (Vilmane et al., Reference Vilmane, Zute, Straumīte and Galoburda2015). The organic LEG system tended to increase total essential and non-essential amino acids as well as crude proteins. While trending higher than organic MNR system, the CNV-T and both T and NT LEG also had the highest total amino acids and crude proteins in oat grains and CNV-NT had the lowest concentration of those nutrients (Fig. 2). The LEG systems T and NT increased most of the essential and non-essential amino acids analyzed in oat grains (Tables 4 and 5). Taurine was the only non-essential amino acid whose concentration was lowest in organic systems (MNR-T and LEG-NT). These results suggest that organic management systems in general, and the organic legume system in particular, were more effective in enhancing the protein quality and quantity of oat grains than CNV systems.
While this study did not measure soil enzymes or soil amino acids, the results suggest that oats may have had greater access to free amino acids in the LEG system. The LEG system relies entirely on leguminous cover crops and cash crops in rotation to provide N fertilizer needs of crops grown in this system. Nitrogen is required for amino acid synthesis. The rhizobia-mediated N fixation by legumes as well as mineralization of organic matter supplied by soil-incorporated legume green manure can both provide free amino acids as well as nitrate-N and ammonium-N for plant uptake (Weigelt et al., Reference Weigelt, Bol and Bardgett2005; Valadares et al., Reference Valadares, de Ávila-Silva, da Silva Teixeira, de Sousa, Vergütz, Larramendy and Soloneski2016; Solangi et al., Reference Solangi, Bai, Gao, Yang, Zhou and Cao2019). Studies by Solangi et al. (Reference Solangi, Bai, Gao, Yang, Zhou and Cao2019) to evaluate P and K uptake efficiencies of various winter leguminous cover crop species reported that phosphatase and leucine-aminopeptidase (enzyme involved in the synthesis of leucine amino acids) as well as β-glucosidase and N-acetyl-glucosaminidase were significantly increased by different species of hairy vetch green manures. Weigelt et al. (Reference Weigelt, Bol and Bardgett2005) assessed the potential of five temperate grass species to preferentially take up different chemical forms of N, including inorganic N and a range of amino acids common in temperate grassland soil. The authors reported that all grass species evaluated were able to directly take up soil amino acids of varying complexities. Schobert et al. (Reference Schobert, Köckenberger and Komor1988) also demonstrated that castor bean plants directly absorbed proline amino acids through their root systems into their xylem vessels without metabolic conversion of the amino acid, suggesting that plant roots can successfully compete with microorganisms for free amino acids in the soil. Although soil organic matter is also known to contain amino acids, only a small proportion of those are free amino acids (Wang et al., Reference Wang, Ye, Perez, Tang and Huang2013; Cao et al., Reference Cao, Ma, Zhong, Yang, Zhu, Zhang, Jin and Wu2016). Studies have shown that amino acids can be adsorbed on soil and soil organic matter, and therefore not available for plant uptake, can be five times greater than free amino acids (Cao et al., Reference Cao, Ma, Zhong, Yang, Zhu, Zhang, Jin and Wu2016). Opportunities for incorporating cover crops into the soil as green manure or rolled-crimped on soil surface as decomposable mulch are limited by the much longer rotations in the MNR system, which includes up to 4 years of a perennial hay phase, compared to the shorter rotations of organic LEG system where these cover crops are more frequently incorporated. These constraints further emphasize the need for comparative systems studies to determine the role of organic inputs in free amino acid availability and uptake by crops.
This study showed that total soil C and N were significantly greater in both organic systems compared to the conventional and S was significantly greater in MNR compared to CNV and LEG with no statistical difference in total S concentration between CNV and LEG (Figs. 3 and 4). These results suggest that organic systems played a role in greater oat grain protein synthesis. This is because all three elements are important components of amino acids—the building blocks of proteins, with the most critical of the three elements in protein synthesis being N (Virtanen and Peltonen, Reference Virtanen and Peltonen1996; Delin, Reference Delin2004). This is further supported by results from this study showing that all three key soil elements were well correlated with both total amino acid and crude protein content in oat grain (Table 8). While soil N was poorly correlated with both amino acids (r = 0.20) and protein content (r = 0.19) of oat grains under CNV system, soil N was highly correlated with LEG (r = 0.85) and MNR (r = 0.86). Similarly, soil C and S were poorly correlated with CNV (r = 0 and 0.04, respectively) compared to a much higher correlation with total amino acids in oat grains in LEG and MNR [r ranging from 0.52 to 0.74 (Table 8)]. A straightforward regression analysis indicated that the total amino acid content of the studied oat grain was 98% of the measured crude protein content (R 2 = 0.95, Supplemental Fig. 1). Given that the CNV system relied mostly on mineral fertilizers to supply C, N and S for oat uptake, we propose that organic inputs including soil organic matter, composted manure and green manures were more important sources of those elements. These observed trends confirm that (i) a strong relationship exists between concentrations of key soil and grain elements (C, N, S) and organically synthesized nutrients (amino acids, protein), and (ii) oat grain proteins benefitted more from organic sources of C, N and S needed for protein synthesis than the more readily available mineral sources in inorganic fertilizers in the CNV system. However, given that inorganic and organic fertilizer inputs were not an experimental factor in this study, further studies are required to specifically evaluate the role of different input sources in supplying minerals required for protein synthesis.
Oat grain vitamins
Vitamin B1 (also called thiamine) is an essential water-soluble organic compound required for carbohydrate metabolism in both plants and animals (Raschke et al., Reference Raschke, Bürkle, Müller, Nunes-Nesi, Fernie, Arigoni, Amrhein and Fitzpatrick2007; Fattal-Valevski, Reference Fattal-Valevski2011). Occurring more abundantly in cereal grains, vitamin B1 deficiency is associated with beriberi, a disease manifested by multiple neuritis, general incumbrance and, in extreme cases, heart failure (Fattal-Valevski, Reference Fattal-Valevski2011). Consisting of thiazole and pyrimidine heterocycle, vitamin B1 can be synthesized only by fungi prokaryotes (bacteria and cyanobacteria) and plants (Fattal-Valevski, Reference Fattal-Valevski2011), necessitating consumption from plants and other dietary sources. Fattal-Valevski (Reference Fattal-Valevski2011) demonstrated that biosynthesis of vitamin B1 in plants requires ThiC, an iron–sulfur cluster protein (Martinez-Gomez and Downs, Reference Martinez-Gomez and Downs2008; Fattal-Valevski, Reference Fattal-Valevski2011). Therefore, higher vitamin B1 concentration in the LEG system compared to the CNV system (Fig. 3) can partly be explained by the greatest concentration of total amino acids and crude proteins in the LEG system compared to the other systems (Tables 4 and 5, and Fig. 3). Given that vitamin B1 is a sulfur-containing compound, higher soil and tissue S concentration in the organic systems in general may also have played a role in increasing vitamin B1 in LEG system (Table 7 and Fig. 4).
An essential metabolite in living organisms, vitamin B6 is widely recognized as a critical co-factor for diverse biochemical reactions, which regulates a greater proportion of cellular metabolism in humans and animals than any other single nutrient (Tambasco-Studart et al., Reference Tambasco-Studart, Titiz, Raschle, Forster, Amrhein and Fitzpatrick2005; Parra et al., Reference Parra, Stahl and Hellmann2018). Existing in various forms including pyridoxal, pyridoxine, pyridoxamine and phosphorylated derivatives, the vitamin can act as an essential cofactor for several metabolic enzymes, including amino acid metabolism and antibiotic biosynthesis (Tambasco-Studart et al., Reference Tambasco-Studart, Titiz, Raschle, Forster, Amrhein and Fitzpatrick2005). Recent studies have revealed vitamin B6 to be a potent antioxidant capable of quenching reactive oxygen species such as singlet oxygen and superoxide (Ehrenshaft et al., Reference Ehrenshaft, Bilski, Li, Chignell and Daub1999; Jain and Lim, Reference Jain and Lim2001; Tambasco-Studart et al., Reference Tambasco-Studart, Titiz, Raschle, Forster, Amrhein and Fitzpatrick2005). It is interesting that CNV-NT and MNR-NT treatment, in the current study, that tended to have the lowest concentration of total amino acids and crude proteins (Fig. 2) had the highest vitamin B6 concentration (Fig. 4). Conversely, cropping systems by tillage management treatments that tended to have higher total amino acids and crude proteins (Fig. 2) tended to also have lower vitamin B6 concentration (Fig. 3). Given that vitamin B6 is involved in the synthesis of several amino acids including leucine, isoleucine and valine (Amorim Franco et al., Reference Amorim Franco, Hegde and Blanchard2016; Parra et al., Reference Parra, Stahl and Hellmann2018), we propose that vitamin B6 metabolic functions may have been inhibited in the cropping system by tillage practice treatments that had lower amino acids and/or proteins. In this study, tillage increased total and crude proteins by 6.8% but reduced vitamin B6 by 6.6%, which suggests tillage may have enhanced vitamin B6 mediated metabolic processes leading to amino acid and protein synthesis, and thereby reducing the concentration of vitamin B6 in tilled systems. However, this study did not quantify soil enzymes and vitamins, nor did it establish a relationship between soil and grain oat grain vitamin concentration, calling for further studies to assess these relationships. While the role of tillage in enhancing mineralization and bioavailability of many soil nutrients is widely documented (e.g., Kladivko et al., Reference Kladivko, Griffith and Mannering1986; Álvaro-Fuentes et al., Reference Álvaro-Fuentes, Morell, Madejón, Lampurlanés, Arrúe and Cantero-Martínez2013; Willekens et al., Reference Willekens, Vandecasteele, Buchan and De Neve2014; Lehman et al., Reference Lehman, Cambardella, Stott, Acosta-Martinez, Manter, Buyer, Maul, Smith, Collins and Halvorson2015; Asenso et al., Reference Asenso, Li, Hu, Issaka, Tian, Zhang, Zhang and Chen2018), the negative impacts of tillage on soil biological, physical and chemical properties are also widely documented (e.g., Kladivko et al., Reference Kladivko, Griffith and Mannering1986; Álvaro-Fuentes et al., Reference Álvaro-Fuentes, Morell, Madejón, Lampurlanés, Arrúe and Cantero-Martínez2013; Willekens et al., Reference Willekens, Vandecasteele, Buchan and De Neve2014; Lehman et al., Reference Lehman, Cambardella, Stott, Acosta-Martinez, Manter, Buyer, Maul, Smith, Collins and Halvorson2015; Asenso et al., Reference Asenso, Li, Hu, Issaka, Tian, Zhang, Zhang and Chen2018). Further studies should, therefore, establish a positive balance between reducing tillage and increasing oat grain nutrients.
In this study, vitamin B6 concertation did not statistically differ between all the organic and conventional management treatments except in CNV-NT, which suggests that amino acid synthesis was not negatively impacted by vitamin B6 content in any of the systems. Neither was the biosynthesis of vitamin B6 substantially impacted by the different management systems. These results are in conformity with other studies that reported no major differences in vitamin contents under different fertilizer dosages in both natural and agricultural environments (Brandt et al., Reference Brandt, Leifert, Sanderson and Seal2011; Miret and Munné-Bosch, Reference Miret and Munné-Bosch2014) and further explain why only two of the vitamins in oat grain were significant. However, in general, organic systems enhanced the availability of nutrients in oat grains more than conventional systems. Oat grain yields between the systems were only marginally significant with the LEG systems trending higher yields than the other systems. This suggests that nutrient concentration differences between the systems were likely due to the different and historic management practices and not due to yield dilution effects.
Summary
Data from the current study clearly indicate that while organic systems significantly improved key nutrients, not all oat grain inorganic and organic nutrient compositions were similarly affected. Essential minerals (C, N and S) and organic (total amino acids and protein) nutrients in oat grain were highly correlated under organic systems than conventional systems suggesting a strong linkage between supplied soil mineral nutrients and synthesis of organic nutrients. While a higher level of S in the MNR systems than in CNV systems may be attributed to the addition of S in composted manure, S concentration in the LEG system was also comparable to that in the MNR systems, suggesting that there may have been a redistribution of S from deeper soil layers. Additional studies are, therefore, required to confirm the sources of these nutrients. Crude proteins and total amino acid levels were significantly greater under organic legume, followed by manure system while the same was significantly decreased under conventional no-tilled system suggesting the ability of organic systems to supply higher levels of the key soil nutrients to synthesize essential and non-essential amino acids, and proteins in oat grains. This study also revealed a strong relationship between total amino acids and crude protein content in oat grain. Organically synthesized nutrient levels such as protein and various vitamins in oat grains under contrasting farming management practices are identified as a further future researchable priority. This study is especially important as it responds to a serious global challenge of declining nutrient concentration of crop produce and proposes best management farming methods that can address the challenge. The study adds important findings to a basket of scientific knowledge on the role of organic farming in enhancing the nutrient quality of grains. Oats are not only economically important for human consumption and livestock feed but gaining increasing recognition as a source of high value compounds with industrial applications due to the many unique properties of the oat grain (Marshall et al., Reference Marshall, Cowan, Edwards, Griffiths, Howarth, Langdon and White2013). The presence of β-glucan, a major endospermic cell wall polysaccharide, has enhanced the recognition of oat grains as a health food source leading to an increase in its use and a broadening of oat-based products (Marshall et al., Reference Marshall, Cowan, Edwards, Griffiths, Howarth, Langdon and White2013). This study not only highlights the increasing value of oats in crop nutrition and human health, but also of the potential of best management farming approaches to enhance human and planetary health.
Supplementary material
The supplementary material for this article can be found at https://doi.org/10.1017/S1742170521000387
Acknowledgements
The authors would like to acknowledge Dr Stella Volpe (PhD), Dr Juan Muniz (PhD) and Dr Brandy-Joe Milliron (PhD) for their support and invaluable advice to Marisa Wagner during her research and compilation of data that constituted this manuscript.
Conflict of interest
None.