INTRODUCTION
Until the 1980s, early human colonization of the New World was assumed to have taken place utilizing an interior (noncoastal) route. This was based partly on an assumption about the extent of late Pleistocene glacial ice in the Gulf of Alaska region, partly on a model of early New World colonization by way of Siberia, and partly on archaeological data suggesting that marine resources (sea mammals, sea birds, marine fish, and shellfish) were not used until late Pleistocene times (Yesner, Reference Yesner1980, Reference Yesner1987). Although some suggested that the earliest human occupants of the Aleutian Islands could have come from the south coast of Beringia as early as ~18,000 cal yr BP (Laughlin et al., Reference Laughlin, Jørgensen and Frølich1979), archaeological sites in the eastern Aleutian Islands, Kodiak Island, and the northern Gulf of Alaska (Fig. 1) proved to be younger than 9500 cal yr BP, with the possible exception of a single 11,000 cal yr BP date from Ugashik Narrows on the Alaska Peninsula (Henn, Reference Henn1978). It was assumed that Beringia was peopled by interior hunter-gatherers, possibly following the paleo–Yukon River and its tributaries (Hopkins, Reference Hopkins1979). From Beringia, it was assumed that an “Ice-Free Corridor” was sought between the Cordilleran and Laurentide ice sheets, allowing peopling of the Americas to the south.
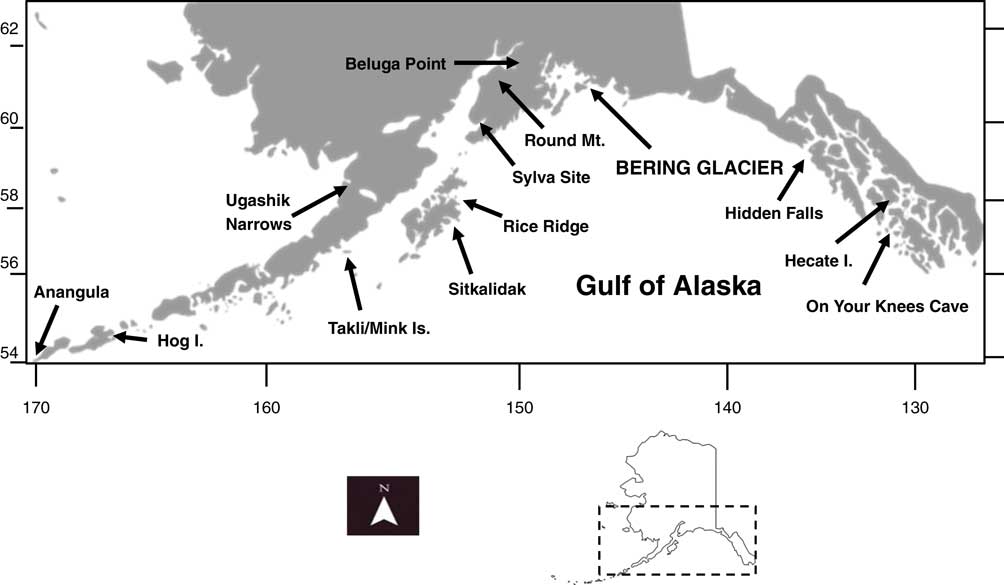
Figure 1 The Gulf of Alaska region, showing important archaeological sites discussed in the text. Inset map shows the location of the Gulf of Alaska region within the current boundaries of Alaska.
Beginning in the late 1970s, a number of investigators began to challenge this idea. The “Ice-Free Corridor” proved to have low ecological potential (Mandryk et al., Reference Mandryk, Josenhans, Fedje and Mathewes2001), and no human occupations in that region of western Canada proved to be as old as those in Beringia (before 13,000 cal yr BP; cf. Beaudoin et al., Reference Beaudoin, Wright and Ronaghan1996). However, it was Fladmark (Reference Fladmark1978, Reference Fladmark1979, Reference Fladmark1983) who offered the most substantial challenge, suggesting that late Pleistocene human populations could have migrated to the south via an ice-free strip of exposed coastal platform along what is now southeastern Alaska and western British Columbia. Did such populations migrate east from the Bering Land Bridge via the Gulf of Alaska?
Fladmark (Reference Fladmark1983) himself suggested that human coastal migration across the Gulf of Alaska to the west would have been much more problematic because of the presence of more extensive late Pleistocene glacial ice in that region. At that time, predominant geologic models for the last glacial maximum (LGM) in the Gulf of Alaska region involved an extensive ice sheet either grounded on or extending to the outer continental shelf (Hopkins, Reference Hopkins1979; Molnia, Reference Molnia1986; Mann and Peteet, Reference Mann and Peteet1994; Mann and Hamilton, Reference Mann and Hamilton1995; Molnia and Post, Reference Molnia and Post1995). Later work suggested that maximal late Pleistocene glaciation along the northern Gulf of Alaska coast was less extensive than in earlier cold phases, consisting of glacial lobes occupying a series of seven parallel troughs extending southward from the modern coastline to the edge of the continental shelf (Calkin et al., Reference Calkin, Wiles and Barclay2001; Kaufman et al., 2011; Molnia, Reference Molnia2008). Although the likely presence of ice-free refugia during the LGM was established for some islands of Prince William Sound (Detterman and Reed, Reference Detterman and Reed1973), a general view of a hostile environment for human coastal migration predominated. Clague et al. (Reference Clague, Matthewes and Ager2004, p. 91), noting that, even in summer, abundant icebergs would have been generated from calving fronts of tidewater glaciers many tens of kilometers wide, reaching to the edge of the continental shelf, concluded that “a coastal migration route along the southwestern tip of the Alaska Peninsula and on the coastal Gulf of Alaska … would have been nearly impossible at the last glacial maximum.”
Whatever the situation during the LGM, it is now clear that deglaciation of the northern Gulf of Alaska region was underway between ~19,000 and 18,000 cal yr BP (Clague et al., Reference Clague, Matthewes and Ager2004; Molnia, Reference Molnia2008; Shennan, Reference Shennan2009; Molnia and Post, Reference Molnia and Post2010). The diatom record indicates a shift from sea ice–dominated assemblages to warmer water North Pacific species beginning ~17,000 cal yr BP (Katsuki and Takahashi, Reference Katsuki and Takahashi2005; Barron et al., Reference Barron, Bukry, Walter, Jason and Finney2009; Caissie et al., Reference Caissie, Brigham-Grette, Lawrence, Herbert and Cook2010). On land, many of the continental shelf areas in the eastern Gulf of Alaska were known to have been ice free by this time; ice-free areas had been noted at the mouth of the Copper River and near the mouth of Lituya Bay (Mann, Reference Mann1986), and shellfish remains dating to 10,500 cal yr BP were known from Harlequin Lake in the front range of the coastal mountains east of Yakutat Bay (Yehle, Reference Yehle1979). To the west, basal peat dates suggested that now-coastal areas of the Alaska Peninsula were deglaciated by 16,000 cal yr BP (Misarti et al., Reference Misarti, Finney, Jordan, Maschner, Addison, Shapley, Krumhardt and Beget2012), and marine invertebrates dated as early as ~17,000 cal yr BP in the Bootlegger Cove Formation of Cook Inlet (Schmidt, Reference Schmidt1963; Schmoll et al., Reference Schmoll, Szabo, Meyer and Dobrovolny1972; Reger and Pinney, Reference Reger and Pinney1996) suggested that local deglaciation had begun by that time. These data suggested that shore-based ice had begun its final retreat and that some shallow marine communities may have been established in the general region by ~17,000 cal yr BP. However, whether a coastline would support nearshore marine productivity in the northern Gulf of Alaska was unknown, and (until the present study) no invertebrate remains had been uncovered in that region.
In contrast to invertebrates, relatively little is known about sea mammal distributions on the southern coast of Alaska during late Pleistocene/early Holocene times. Late Pleistocene–aged specimens of walrus (Odobenus rosmarus) and bearded seal (Erignathus barbatus) have been retrieved from both the northern Bering Sea (Seward Peninsula, St. Lawrence Island) and from the southern Bering Sea (Nelson Island) (Kurtén and Anderson, Reference Kurtén and Anderson1980), but not from farther south.
Nevertheless, a prevailing model has been that there were significant changes in sea mammal distributions and migratory behavior resulting from closure of the Bering Strait. Whales and seals, which now migrate into the straits from the Pacific, must have been confined to the southern shore, while walrus and other species now limited to the zone of seasonal sea ice in the Bering and Chuckchi Seas were probably also displaced to the southern Beringian coast (Fladmark, Reference Fladmark1978, p. 123).
In this light, it is notable that remains of pagophilic taxa such as ringed seal (Phoca hispida) have been found in caves in southeastern Alaska dating from the LGM. On Your Knees Cave, for example, has provided 26 paleontological dates on ringed seal bones ranging from 24,150 to 13,700 14C BP (29,000 cal BP to 17,000 cal yr BP), indicating shorefast ice in that area until ~17,000 cal yr BP (Heaton et al., Reference Heaton, Talbot and Shields1996; Heaton and Grady, Reference Heaton and Grady2003). Specimens of walrus dated to the LGM are known from as far south as San Francisco Bay but appear to have retreated northward by the end of the Pleistocene (Harington, Reference Harington1984, Reference Harington2008).
Fedje et al. (Reference Fedje, Mackie, Dixon and Heaton2004, p. 137) concluded that, although there is “no archaeological evidence for [human] occupation [until] … 12.2 ka BP (10.3 14C ka BP),” “there is paleontological … evidence showing a viable and productive terrestrial and marine ecology back to at least 16 ka BP (13.5 14C ka BP)” in the Northwest Pacific. In that light, we report here on the discovery of important new data on marine invertebrate and sea mammal remains dating to the late Pleistocene/early Holocene period on the Bering Glacier foreland (Crossen et al., Reference Crossen, Pasch and Yesner2010; Pasch et al., Reference Pasch, Foster and Irvine2010). These data suggest that a highly productive marine environment was present in the northern Gulf of Alaska region during that time, one that could potentially have supported coastal human migration in the region.
GEOLOGIC HISTORY OF BERING GLACIER FORELAND
Modern environment
Bering Glacier drains into the Gulf of Alaska ~120 km southeast of Cordova, Alaska (Figs. 1 and 2). It is both the largest glacier in North America and the world’s largest temperate glacier, with an area of ~5175 km2 and containing ~15% of the glacial ice in Alaska (Molnia and Post, Reference Molnia and Post1995, Reference Molnia and Post2010). Bering Glacier trough, extending across the continental shelf, is more than 500 m deep, as much as 320 m below sea level, and extends inland ~80 km upglacier from the current terminus (Molnia and Post, Reference Molnia and Post2010; Schuchman et al., Reference Schuchman, Josberger, Jenkins, Payne, Hatt and Spaete2010).

Figure 2 (color online) Satellite image of Bering Glacier with place names and site locations. The Chugach Mountains are located to the north (top), and Vitus Lake lies between Bering Glacier and the Gulf of Alaska coastline. The Little Ice Age (LIA) margin is seen along the southern shore of Vitus Lake. Along the southeast and southwest moraine margins, LIA outwash plains prograde to the Gulf of Alaska. A series of beach ridges are seen along the coast both east and west of the Seal River. These may have prograded across the fiord mouth in the mid-Holocene, helping to change the Vitus Lake area from marine to freshwater. Locations cited in the text include Admiral Bogg (AB), Ancient Forest (AF), Ball Island (BI), and Weeping Peat (WP), as well as Long Island and Seal River.
An extensive glacial foreland (Fig. 2) extends from the current ice margin to the Gulf of Alaska (an area of ~550 km2 located between ~60–61°N and 142–144°W). This foreland, consisting of thick glacial deposits as well as littoral, fluvial, and lacustrine sediments, ranges from near sea level to 87 meters above sea level (m asl). It also contains a proglacial lake, Vitus Lake, where the 23-km-wide Bering Glacier margin calves into the water. Vitus Lake has increased in size with continuing ice retreat since the end of the Little Ice Age (LIA) in the late nineteenth century (Crossen, Reference Crossen2006; Crossen and Lowell, Reference Crossen and Lowell2010).
The piedmont lobe of Bering Glacier ramps up on the foreland, and because of its extraordinary volume and thickness (800 m), its flow path is not controlled by topography, so that ice is both extremely thick in the deep embayments and extremely thin over higher ridges. Below the modern ice, seismic and other methods have revealed bedrock highs, future islands, and peninsulas (Bruhn et al., Reference Bruhn, Forster, Ford, Pavlis and Vrkint2010; Molnia and Post, Reference Molnia and Post2010; Schuchman et al., Reference Schuchman, Josberger, Jenkins, Payne, Hatt and Spaete2010). A cross section of Vitus Lake has revealed parallel troughs and subaqueous ridges with relief of more than 200 m. The deepest portion of the lake shows water depths greater than 250 m, which are further underlain by a 110 m of lake basin sediment fill (Schuchman et al., Reference Schuchman, Josberger, Jenkins, Payne, Hatt and Spaete2010).
The seaward edge of Vitus Lake is dammed by an LIA moraine (Fig. 2) where Bering Glacier formerly terminated adjacent to the Gulf of Alaska coast, as seen on historic maps from AD 1880 and 1900 (Molnia and Post, Reference Molnia and Post2010). The lake currently drains into the Pacific Ocean via the 6-km-long Seal River (Figs. 2 and 3). Beyond the foreland is a relatively straight coastline, open to the Gulf of Alaska, and noted for its strong storms and large waves. Much of this Gulf of Alaska coast is inhospitable, lacking embayments and sheltered inlets. The coast adjacent to Seal River exhibits long sandy beaches covered with large logs and backed by parallel beach ridges, now partially eroded, but previously extending up to 30 km along the coast (Crossen and Lowell, Reference Crossen and Lowell2010) (Fig. 2).

Figure 3 (color online) The straight shoreline of the Gulf of Alaska at the southernmost edge of the Bering Glacier foreland.
Late Pleistocene/early Holocene marine environments
Submarine cores taken off Kayak Island (Ager, T., personal communication, 2007) indicated that ice retreated from the continental shelf ~18,300 cal yr BP. Along the eastern foreland of Bering Glacier, basal peats near Hanna Lake indicate deglaciation of that area had begun by 16,800 cal yr BP (Fleisher et al., Reference Fleisher, Muller, Peteet and Lachniet1999). It seems likely that Bering Glacier retreated up its fiord as a tidewater glacier by ~17,000 cal yr BP (Crossen and Lowell, Reference Crossen and Lowell2010). Shennan and Hamilton (Reference Shennan and Hamilton2010) suggest that relative sea level was 5 m higher at this time because of submergence linked to local tectonics. Rising sea levels and the depth of the Bering Trough likely engendered catastrophic retreat of a tidewater margin that may have receded inland of the current ice margin, and possibly to the grounding line, in the lengthening Bering Fiord. This would have a left an indented fiord deep within the mountain front and a series of islands and peninsulas along the mouth of the fiord, similar to the modern Prince William Sound coast today (Crossen and Lowell, Reference Crossen and Lowell2010) (Fig. 4).

Figure 4 (color online) Modern Prince William Sound fiord as an analog for late Pleistocene/early Holocene habitat at Bering Glacier. This model suggests that ice retreated to the fiord head, with protected shorelines adjacent to the fiord walls providing habitat for a variety of edible marine species. Subsequently, the late Holocene advance of Bering Glacier scraped Pleistocene sediment from the fiord floor and redeposited it on islands and shorelines around Vitus Lake.
Glacial retreat exposed new island and peninsula shorelines adjacent to the tidewater glacier, perhaps analogous to the fiords adjacent to Columbia Glacier or College Fiord today (Crossen and Lowell, Reference Crossen and Lowell2010). This would have allowed numerous intertidal and shallow marine invertebrates to populate these newly exposed shorelines, including the 6000+ specimens from Bering Glacier foreland that were collected and discussed here (Figs. 5 and 6). These invertebrate remains were left on exposed islands and ridges as the glacier retreated. The most likely scenario is that these marine sediments were deposited in areas currently below Bering Glacier and were overrun by ice advance. This would have carried the sometimes delicate shells within sediments blocks that were later transported by the ice onto terrestrial ridges where the shells subsequently eroded out of the sediment (Fig. 7). Samples have been found both lying on surfaces (Fig. 8) and embedded within deformed blocks of marine sediment (Pasch et al., Reference Pasch, Foster and Irvine2010). A few marine mammal bones were simultaneously deposited through the same process and are found in direct association with the invertebrate remains. In addition, at one location on the Taggland Peninsula, along the shore of Vitus Lake, articulated boring clams (Penitella) dated from 5430 to 9450 cal yr BP (Shennan and Hamilton, Reference Shennan and Hamilton2010) are found in situ in their original boreholes within glacially striated sandstones. These fossils show clear evidence of both the former marine environment and the later overriding of glacial ice. This landscape of narrow embayed marine fiords, lined with numerous elongated peninsulas and islands and populated by both marine invertebrates and vertebrates, would have made for a protected and productive coastline during the late Pleistocene/early Holocene period from approximately 15,000 to 5000 yr ago (Crossen et al., Reference Crossen, Pasch and Yesner2010).

Figure 5 (color online) Bivalve (Macoma lipara) in situ on the surface of the Bering Glacier melt-out till.

Figure 6 (color online) Samples of ancient edible shellfish from the Bering Glacier foreland. Bottom row: Macoma lipara; top row: carapace of Cancer magister (left) and leg fragments of Chionoecetes bairdi (right).

Figure 7 (color online) Irregular topography of the north side of Long Island, showing pyramidal blocks consisting of discontinuous beds of sand and silt.

Figure 8 (color online) Melt-out surface of the Taggland site showing irregular distribution of glacial sediments.
Mid-Holocene terrestrial environment
By approximately 4000 yr ago, this area changed from a largely marine to a terrestrial environment. Raised peat bogs at 5 m asl, with basal dates of ~3300 cal yr BP, cover the marine invertebrate boreholes and along with transported wood dating to 4050 cal yr BP indicate that the marine embayment had been uplifted and that forests and peat had replaced coastal habitats (Crossen and Lowell, Reference Crossen and Lowell2010). Tectonic uplift is common in this highly unstable area; this location experienced ~3 m of uplift during the AD 1964 Alaska Earthquake, and the adjacent Yakutat area experienced up to 16 m of uplift during the AD 1899 earthquake (Yehle, Reference Yehle1979; Hamilton and Shennan, Reference Hamilton and Shennan2005; Shennan, Reference Shennan2009). Subsequently, a series of seven well-dated buried forests as well as numerous buried peat bogs around the current Vitus Lake testify to the persistence of a terrestrial environment until 1025 cal yr BP. These forests included rooted and transported trees up to 1.6 m in diameter and containing up to 300 rings that in one location, the Ancient Forest site, occupied the area for as long as 800 yr. Forests were killed numerous times by burial in sandy lacustrine deposits. No glacial tills indicating ice advance are found interbedded in these nonglacial sediments (Crossen and Lowell, Reference Crossen and Lowell2010).
Late Holocene glacial environments
In the late Holocene, after AD ~1000, cross-bedded gravels, interpreted as glacial outwash, inundated the entire Bering Glacier foreland. At the Ancient Forest site, dating of the peats allowed for calculation of the rate of outwash aggradation. From AD ~600 to ~1000, 8 m of outwash was deposited for an average aggradation rate of 2 cm/yr. After AD 1000, the dating is less certain, but the outwash is coarser, and ~12 m of gravel was deposited below a single surface till. This thick package of outwash gravel is found across the entire foreland and even underlies the islands in the center of Vitus Lake (Crossen and Lowell, Reference Crossen and Lowell2010). This indicates that the former deep marine fiords, and in fact the entire marine ecosystem, were buried by the outwash deposited ahead of the advancing ice margin. This ice advance was the agent that cut the deep fiords in Vitus Lake and excavated the marine sediments containing the shells.
However, prior to the ice advancing to the coast during the LIA, the outwash remodeled the foreland. The outwash streams carried the gravels to the ocean, spreading broad outwash fans emanating from the proximal side of the advancing glacial moraine (Fig. 2). The fiords were filled, the forests overrun and buried, and a single till deposited above the gravel. The previously indented shoreline built seaward, and a smooth coastline formed at the distal edge of the outwash fan. Finer materials migrated along the shoreline forming multiple beach ridges, determined to be younger than 600 yr by Molnia and Post (Reference Molnia and Post2010). These ridges, which extend for more than 30 km along the coast, may have helped to block the previous Bering Fiord (Fig. 2). Today, the central area between two distal sets of beach ridges gives evidence to the erosion produced when the LIA outwash streams reached the ocean. These events built the current straight, unprotected coast along the southern Bering Glacier foreland (Fig. 3). Since AD 1900 Bering Glacier has been retreating north across Vitus Lake, with its recession punctuated by a series of surge events occurring in AD ~1900, 1920, 1938–1940, 1957–1967, 1993–1995, and 2010–2011.
RESEARCH METHODS
Collection of invertebrate remains and sea mammal bones
The University of Alaska Anchorage collected invertebrate remains and sea mammal bones from 15 localities within the glacial foreland along a 30 km transect adjacent to the active Bering lobe (Fig. 2). Marine organisms were found at each of these sites, suggesting that marine sediments must have been incorporated into the ice along its entire 30 km width. Sites with broad expanses of melt-out till surfaces were visited repeatedly (Pasch et al., Reference Pasch, Foster and Irvine2010). Invertebrate specimens were found on the ground surface and in buried glacial deposits, weathering out of eroding vertical exposures. However, specimens situated in buried sands and gravels were fragmentary and not identifiable to species. Vertebrate specimens were retrieved only from surface deposits.
A total of 6230 invertebrate specimens were collected from the 15 localities over a 9 yr period. The greatest number of invertebrates was found at four sites: Ancient Forest, Weeping Peat, Taggland, and Admiral Bogg Coast (Fig. 2). Together they accounted for 68% of the total number of invertebrate specimens. Of these, 2192 specimens were recovered from the Ancient Forest locale, 1336 specimens were recovered from the Weeping Peat locale, 513 specimens were recovered from the Taggland locale, and 201 specimens were recovered from the Admiral Bogg locale. These locales also provided 96% of the 605 best-preserved specimens found in the study area; another 11 less productive sites, along the margin of Vitus Lake and on small islands in the lake, accounted for 32% of the total number of specimens (Pasch et al., Reference Pasch, Foster and Irvine2010). Of these, one locale, Long Island, produced more than 600 specimens, but most were in the form of shell hash. Other important locales producing dated shells included Ball Island and Seal River (Fig. 2). Finally, vertebrate remains were found both at the Taggland and Long Island locales (Fig. 2). For that reason, both of these sites are described in detail subsequently.
The Taggland locale (60°8.99′N, 143°34.77′W) is a narrow headland between the east margin of Tashalich Arm and the main body of Vitus Lake at an elevation of 16–37 m asl. The site consists of ice margin deposits with irregular morainal ridges, swales, and gullies. The surface is dotted with small relic ponds, numerous erratic boulders, and pavements of lag gravels (Fig. 8).
The Long Island locale (60°6.91′N, 143°29.59′W) consists of a series of elongated, pyramidal-shaped hills up to ~5 m high and ranging from 2 to 20 m long (Fig. 8). This site is low in elevation (~6 m asl) and has relatively little relief. Invertebrate specimens were retrieved from distinct beds composed of silt and sand units up to 10 cm thick, found within weathered blocks of cohesive silt, believed to be chunks of seafloor deposited by basal ice during the 1993–1995 surge of Bering Glacier.
Taxonomic identification
Both invertebrate and vertebrate specimens were identified by reference to comparative collections at the University of Alaska Museum of the North. Vertebrate specimens were identified with the use of the Department of Mammalogy taxonomic osteological collections, designated as “UAM.” Lengths and weights were recorded for vertebrate specimens using standard metric units for comparison with modern specimens.
Dating
Conventional radiocarbon ages for 29 bivalve specimens were determined, 9 by Beta Analytic Inc. (standard dates) and 20 by the University of California, Irvine (accelerator mass spectrometry dates). A single date was obtained from pinniped (vertebrate) remains in order to minimize destruction of the four samples available. An updated calibration was performed using the program CALIB 7.1 and the Marine 13.14C data set (Stuiver and Reimer, Reference Stuiver and Reimer1993; Stuiver et al., Reference Stuiver, Reimer and Reimer2015) to calculate age ranges associated with the conventional dates. A δ13C marine isotopic correction was applied to both invertebrate and vertebrate remains; in addition, a local marine reservoir age correction (δr) of 390 yr was applied, intermediate between values from Orca Bay and Middleton Island in Prince William Sound, Alaska (McNeely et al., Reference McNeely, Dyke and Southon2006).
ANALYTICAL RESULTS
Invertebrate remains
The overall invertebrate assemblage from the Bering Glacier foreland represented 110 species, 75 genera, and 46 families; by comparison, only 43 species of invertebrates are known for the area today (Foster, Reference Foster2000). The four major locales (Ancient Forest, Weeping Peat, Taggland, and Admiral Bogg) produced a range of 17 to 86 species per site, whereas the remaining locales produced only 2 to12 species per site (Pasch et al., Reference Pasch, Foster and Irvine2010). The Long Island locale was dominated by Nucula pernula. Otherwise, bivalves were the most numerous among the specimens, comprising 70% of the total number of invertebrate specimens, while gastropods were the most diverse, comprising 44% of the total number of species.
As noted previously, 29 large bivalve specimens were selected for radiocarbon dating: 9 Macoma specimens from the Admiral Bogg locale; 6 Macoma specimens from the Ancient Forest locale; a Clinocardium specimen from the Ball Island locale; a Saxidomus specimen from the Seal River locale; and 12 specimens including a variety of taxa from the Weeping Peat locale (Fig. 2, Table 1). Conventional radiocarbon ages on these specimens ranged from 7590±140 to 13,230±25 BP, with associated calibrated ages from ~7600 to ~14,700 cal yr BP (Table 1). Of the four collection sites, the Admiral Bogg locality produced the oldest dates, with ages as early as ~14,700 cal yr BP; the Seal River locale produced a date of ~14,000 cal yr BP. Although a variety of taphonomic processes are responsible for the precise location of these sites, it is worth noting that the sites associated with the oldest dates (Admiral Bogg and Seal River) are located closest to the modern shoreline, with other sites more than 5 km farther inland. However, dates from the Long Island locale were produced only on sea mammal bones (see under Vertebrate Remains: Dating).
Table 1 Conventional radiocarbon and calibrated dates for 29 invertebrates collected across the Bering Glacier foreland.

Note: Oldest specimen (from Ancient Forest locale) shown in bold.
a Analysis by Beta Analytic Inc. All other analyses by Keck Carbon Cycle Accelerator Mass Spectrometry Facility, University of California, Irvine.
The ancient species reflected by the invertebrate remains were apparently adapted to a variety of conditions, such as water depth, turbidity, and substrates (including various mixtures of mud, sand, gravel, and boulders, as well as bedrock). They also demonstrate a variety of life modes and feeding types, including bivalves, gastropods, barnacles, and bryozoans (Table 2). In terms of water depth, the invertebrate remains reflect a wide range of intertidal to subtidal habitats. The four most productive sites produced a range of 9.1–42.4% intertidal invertebrates (Table 3), those potentially most easily collectable by ancient human populations.
Table 2 Types of ancient invertebrates found at major collection locales in the Bering Glacier foreland.

Table 3 Environmental parameters of ancient invertebrates found in the Bering Glacier foreland.

When the species assemblages from these four most productive sites were compared in detail in terms of ecological attributes (depth preference, habit, and feeding type), it became apparent that the faunas represent the presence of somewhat distinct habitats or paleoenvironments along the ancient shorelines (Pasch et al., Reference Pasch, Foster and Irvine2010). For the Ancient Forest habitat, higher numbers of grazers, including several limpets, topshells, and sea urchins indicate a rock/boulder substrate swept by currents carrying particulate organics and algal debris. However, the presence of deposit feeders and subtidal infauna also suggests the presence of an enriched soft substrate offshore of a rocky intertidal nearshore setting. The Weeping Peat assemblage includes a mix of intertidal and shallow subtidal deposit feeders composed mostly of infaunal bivalves, along with some suspension feeders and intertidal predatory gastropods. This shelled fauna suggests a mixed substrate consisting of boulders scattered on mud. For the Admiral Bogg habitat, most of the specimens represent infaunal and epifaunal subtidal deposit feeders including clams and gastropods, suggesting muddy sediments in a bay or fiord. For the Taggland habitat, the predominance of boring clams, complete valves of nesting clams, and worm tubes suggests a substrate of stiff clay or sedimentary rock reflecting a protected outer coast in a low intertidal to subtidal setting (Lees, Reference Lees1978; Feder, Reference Feder1979; Hood and Zimmerman, Reference Hood and Zimmerman1987; Gordillo and Aitken, Reference Gordillo and Aitken2000).
As noted previously, these marine shells were apparently released from blocks of frozen marine floor sediment eroded by the glacier and transported to its terminus. This indicates that the area currently occupied by the piedmont lobe was previously a marine embayment. Current topography suggests that the paleoshoreline had to lie ~35–40 km farther inland at the base of the mountain front, with seawater flooding troughs lying below sea level (Crossen and Lowell, Reference Crossen and Lowell2010). This would have resulted in a shoreline similar to that seen in Prince William Sound today, with deep embayments, rocky headlands, islands, and beaches (Fig. 4). This is corroborated by the fact that the ancient marine invertebrates represent distinct paleoenvironments not all available in the area today. They include exposed coasts, sheltered coasts, mudflats, and beaches with different energy levels.
These invertebrates would have provided an important source of food for pinnipeds (see below) but also potentially for humans traveling along the coast. Of the 110 ancient invertebrate species, 34 are known to be edible by humans (Table 4). By comparison, only 14 edible invertebrate species are found in the area today (Foster, Reference Foster2000).
Table 4 Edible species of ancient invertebrates found in the Bering Glacier foreland.

Vertebrate remains
Four well-preserved bones of pinnipeds (seals and walruses) were recovered from the Bering Glacier foreland. These are the first reports of sea mammal remains from the Gulf of Alaska region from the late Pleistocene/early Holocene period. All four specimens were located on glacial drift recently deposited on the melt-out surface (Fig. 8) between the retreating ice front and the terminal moraine of the 1993–1995 surge of Bering Glacier (Molnia and Post, Reference Molnia and Post1995; Crossen and Lowell, Reference Crossen and Lowell2010). Three of the four specimens were recovered from the Taggland locale (Pasch et al., Reference Pasch, Foster and Irvine2010) in association with more than 500 invertebrate remains; the fourth was recovered from the Long Island locale, in association with more than 600 invertebrate remains (Fig. 2).
Taxonomic analysis
One of the Taggland specimens (05-AP-20; Fig. 9, bottom) is a complete left first metatarsal from a juvenile, probably male, bearded seal (Erignathus barbatus), with a fused distal but unfused proximal epiphysis. Specimen weight is 27.9 g. The specimen is a close match for UAM 16621 (SUV-31-77), a male measuring 215.7 cm (85 in.) in total length and weighing 242.7 kg (535 lb.) (see also specimen UAM 11455, an adult male measuring 228.6 cm [90 in.] in total length and weighing 289.4 kg [638 lb.]; University of Idaho Museum, 2016). The metatarsal is 9.10 cm in length, while UAM 16621 is 9.20 cm in length. Other critical measurements helping to define this metapodial include the thickness of the proximal end (3.10 cm vs. 2.78 cm), the thickness of the distal end (2.28 cm vs. 1.89 cm), and the “waisting” of the distal diaphysis (1.30 cm vs. 1.28 cm). Those features help to differentiate this specimen from other large pinnipeds such as walrus or Steller sea lions (Eumetopias jubatus). Additional diagnostic features include the rotation and flatness of the proximal end; the porosity of the proximal diaphysis; and the rotation, roundedness, and muscle scars associated with the distal condyles.

Figure 9 (color online) Two pinniped specimens collected from the Taggland locale. Top: right first phalanx of a juvenile Pacific walrus (Odobenus rosmarus). Bottom: complete left first metatarsal of a juvenile bearded seal (Erignathus barbatus).
The second Taggland specimen (06-AP-20B; Fig. 9, top) is a right first phalanx from a juvenile, probably female, Pacific walrus (Odobenus rosmarus), missing a portion of the distal end. Specimen weight is 26.6 g. This specimen is a close match for UAM 11637 (GLA-1-71), a female measuring 266 cm (104.7 in.) in total length and weighing 623.7 kg (1375 lb.). It is 9.61 cm in length, while UAM 11637 is 7.57 cm in length. Although similar to Steller sea lion (Eumetopias jubatus), it can be differentiated by the following diagnostic features: the flatness, pattern of angulation, and porosity of the proximal end; the flatness of the diaphysis; the U-shaped intercondylar groove; and the flatness, rotation, and muscle scars associated with the distal condyles.
The third Taggland specimen (06-989-TL/20; Fig. 10) is a complete right medial phalanx of the hind limb (pes) of an adult ringed seal (Phoca hispida) based on epiphyseal fusion (Stora, Reference Stora2000); gender is indeterminate. It is 2.82 cm in length and weighs 7.85 g. Although it is similar to phalanges of other, closely related phocids (harbor seal [Phoca vitulina], spotted seal [Phoca largha], and ribbon seal [Histriophoca fasciata]), there are sufficiently distinctive features, particularly lateral rotation of the distal condyles, to identify the specimen as ringed seal (e.g., see harbor seal [Phoca vitulina] UWBM-81073; University of Idaho Museum, 2016). However, in deference to common practice related to the difficulty of definitively identifying such phalangeal specimens to species, we refer to it as Phoca cf. hispida.

Figure 10 (color online) Third pinniped specimen collected from the Taggland locale: complete right medial phalanx of the hind limb (pes) of an adult ringed seal (Phoca cf. hispida).
The sea mammal specimen from Long Island (O5-AP-14) was an additional pinniped metapodial, tentatively identified as an additional walrus specimen. The specimen was incomplete, representing the proximal portion of a left third metatarsal. The preserved distal portion suggests an adult specimen. Specimen weight was 35.2 g. This specimen was sent to Beta Analytic Inc. for radiocarbon dating.
Dating
Specimen 05-AP-14 received an uncalibrated but δ13C isotopically corrected date of 13,980±70 14C yr BP (Beta-228899). Utilizing the 13.14C marine database and the 390 yr marine reservoir effect described previously results in a 1σ calibrated date range of 15,730–15,967 cal yr BP or a 2σ calibrated date range of 15,601–16,085 cal yr BP. Therefore, this specimen can be securely dated at ~16,000 cal yr BP. This constitutes the first late Pleistocene record of sea mammal remains from the Gulf of Alaska region. Although there are no shellfish dates from the Taggland locale, the sea mammal remains were directly associated with the invertebrate remains, so that the bone date may represent a maximum date for this locale. The date is similar to but still older than the oldest date from the shellfish specimens recovered from the Admiral Bogg locale (~15,000 cal yr BP). It therefore implies that pagophilic marine mammals (see under Ecology) inhabited this area by ~16,000 BP, shortly after deglaciation, and perhaps even earlier than the establishment of intertidal shellfish communities. It is possible that the date also applies to the walrus specimen from the Beringia Nova locale which, because of the uniqueness of that specimen, we were unwilling to sacrifice for radiocarbon dating.
Ecology
All three of these pinniped taxa are pagophilic, associated with pack ice or shorefast ice, and distributed today primarily in the northern Bering Sea and Arctic Ocean. The walrus has inhabited the northern Pacific Ocean during the last million years, having derived from earlier Miocene populations (Harington, Reference Harington2008). It is an inhabitant of moving pack ice, although in summer it may utilize isolated coastal beaches and rocky islets, such as at haulouts on Round Island in southwestern Alaska today. Ice is preferred for rookeries, but land may be used in the absence of ice. Archaeological evidence from Margaret Bay on Unalaska Island in the eastern Aleutian Islands (Davis, Reference Davis2001) suggests that walrus habitat expanded, at least seasonally, during the mid-Holocene Neoglacial period (~6500–4000 BP) to include the southern Bering Sea, and isolated walrus elements are known from mid-Holocene archaeological sites on Yukon Island in Cook Inlet, southcentral Alaska (Workman and Workman, Reference Workman and Workman1988; Workman Reference Workman1996). The walrus is a migratory animal, covering up to 3000 km in annual movements. It was used for food, fuel, tools, clothing, shelter, boats, and sleds by Alaska Native peoples (Nowak, Reference Nowak1991).
The bearded seal is found along coasts and ice floes, is restricted to waters of the continental shelf near coastlines, and is generally associated with sea ice. It will, however, come ashore on gravel beaches during summer to rest and molt. It was used traditionally by Alaska Native peoples for food, oil for lamps, clothing (especially intestines), boot soles, boat covers, and tools such as heavy ropes and dog harnesses (Nowak, Reference Nowak1991). In Alaska, the distribution of the bearded seal is today limited to areas north of the Alaska Peninsula in the Bering Sea. Archaeological evidence from the Amaknak Bridge site on Unalaska Island (Crockford and Frederick, Reference Crockford and Frederick2007; Crockford, Reference Crockford2008) suggests that, as for the walrus, the habitat of the bearded seal expanded in the southern Bering Sea during the Neoglacial period in response to the expansion of seasonal sea ice (Kelly, Reference Kelly2001; Rigor and Wallace, Reference Rigor and Wallace2004; Fisher et al., Reference Fisher, Dyke, Koerner, Bourgeois, Kinnard, Zdanowicz, de Vernal, Hillaire-Marcel, Savelle and Rochon2006). Polar bear habitat appears to have expanded in the southern Bering Sea at the same time (Veltre et al., 2008). The diet of the bearded seal consists of fish, shrimp, crabs, and mollusks, with the latter including both clams and gastropods. The ringed seal (Phoca hispida) is usually associated with ice floes, and breeds on the ice (Wiig et al., Reference Wiig, Derocher and Belikov1999). However, it may move to the shoreline during the summer. The ringed seal eats fish, cephalopods, and crustaceans and was used extensively for food, oil, clothing, and tools throughout the Arctic (Nowak, Reference Nowak1991). In Alaska, the distribution of ringed seal today is generally limited to the northern Bering Sea region. In contrast, paleontological evidence suggests that ringed seal habitat expanded southeastward during the LGM (Heaton et al., Reference Heaton, Talbot and Shields1996; Heaton and Grady, Reference Heaton and Grady2003). Oceanic warming during the early Holocene subsequently resulted in the replacement of the ringed seal by the harbor seal (Phoca vitulina) throughout southern Alaska; harbor seals also utilize sea ice for haulouts and breeding, particularly for predator avoidance, but do not require either. At the same time, the Steller sea lion (Eumetopias jubatus) and sea otter (Enhydra lutris) became established throughout that area (Baichtal and Crockford, Reference Baichtal and Crockford2011). However, archaeological evidence suggests that ringed seal habitat may have expanded southwestward into the eastern Aleutian Islands during the Neoglacial period (Davis, Reference Davis2001). Neoglacial sea ice expansion appears to have affected some aspects of ringed seal adaptations and life history dynamics (Crockford and Frederick, Reference Crockford and Frederick2007).
ARCHAEOLOGY OF EARLY COASTAL SITES
In spite of the presence of marine resources in the Gulf of Alaska region in the late Pleistocene/early Holocene period, all known archaeological sites in the region, demonstrating the definitive presence of humans, postdate ~8000 cal yr BP. The nearest sites in the region that may be late Pleistocene or (more likely) early Holocene in date are those with microblade technology in the eastern Cook Inlet/Kenai Peninsula region, such as Beluga Point (Reger, Reference Reger1981) and Round Mountain (Reger and Pipkin, Reference Reger and Pipkin1996) (Fig. 1). However, the antiquity of these sites has not been established, and they may well be derived from earlier microblade sites in the Susitna Valley to the north (Wygal and Goebel, Reference Wygal and Goebel2012), and ultimately from so-called Denali Complex sites of interior Alaska, north of the Alaska Range (Yesner, Reference Yesner2001, Reference Yesner2006). The earliest securely dated sites in the region are those such as the Sylva site on Kachemak Bay, eastern Cook Inlet (Workman, Reference Workman1996), which postdate 8000 cal yr BP and may represent regional extensions of the Early Ocean Bay culture of Kodiak Island and the Alaska Peninsula (Clark, Reference Clark1979), including the Sitkalidak Island sites (Fitzhugh, Reference Fitzhugh2003) and Rice Ridge site (Kopperl, Reference Kopperl2003) on eastern Kodiak Island, and the Mink Island (Hirons et al., Reference Hirons, Murray and Schaaf2003) and Takli Island sites (Clark, Reference Clark1977) on the eastern Alaska Peninsula (Fig. 1). The well-preserved faunal remains from Rice Ridge and Mink Island do, in fact, demonstrate utilization of sea mammals and shellfish as early as ~8000 cal yr BP.
No early coastal sites have been found in the Bering Glacier area or the general Gulf of Alaska/Prince William Sound region (Mann et al., Reference Mann, Crowell and Hamilton1998; Jangala, Reference Jangala2010). For the western Gulf of Alaska/Prince William Sound, this may largely be explained as the result of both Holocene sea level rise and tectonic submergence (Mann et al., Reference Mann, Crowell and Hamilton1998; Hamilton and Shennan, Reference Hamilton and Shennan2005; Shennan and Hamilton, Reference Shennan and Hamilton2006, Reference Shennan and Hamilton2010). However, no early sites have been recovered from the eastern Gulf of Alaska/Prince William Sound region, characterized by numerous uplifted surfaces, although the area remains relatively unexplored archaeologically.
Coastal archaeological sites from the southern Alaskan Pacific region (Fig. 1) are somewhat earlier both west and east of the Gulf of Alaska. To the west, in the eastern Aleutian Islands, coastal sites such as Anangula and Hog Island are somewhat earlier but still postdate 9500 cal yr BP (Laughlin et al., Reference Laughlin, Jørgensen and Frølich1979; Knecht and Davis, Reference Knecht and Davis2001). This coincides with an increased spike in oceanic productivity at this time (Caissie et al., Reference Caissie, Brigham-Grette, Lawrence, Herbert and Cook2010). Although faunal remains are not preserved in these sites, their location and known available resources suggest that sea mammal hunting would have been the focus at these early sites. Lack of evidence for shellfish collection in eastern Aleutian sites until the mid-Holocene period suggests that rapidly rising sea levels during the early Holocene may have prevented development of robust shellfish populations until that time (Yesner, Reference Yesner1977, Reference Yesner1980).
In the southeast Alaskan region, coastal archaeological sites are somewhat older, dating back to 12,500 cal yr BP (Ackerman, Reference Ackerman1996). Similar limiting dates have been found to the south on the British Columbia coast, even from submerged or intertidal sites (Josenhans et al., Reference Josenhans, Fedje, Conway and Barrie1995, Reference Josenhans, Fedje, Pienitz and Southon1997; Fedje et al., Reference Fedje, Mackie, Dixon and Heaton2004; Mackie et al., Reference Mackie, Davis, Fedje, McLaren and Gusick2013). In southeastern Alaska, early components at sites such as Hidden Falls (Davis, Reference Davis1989) (Fig. 1) have demonstrated salmonid remains, but definitive evidence of shellfish use is somewhat later, both at Hidden Falls (Erlandson, Reference Erlandson1989) and at Hecate Island, where they are dated to ~9500 cal yr BP (Ackerman, Reference Ackerman1985). The earliest human remains discovered on the Alaskan coast, from On Your Knees Cave on Prince of Wales Island (Dixon, Reference Dixon2001), have demonstrated dietary dependence on marine foods, based on values of stable carbon and nitrogen isotopes at ~11,000 cal yr BP. However, this date is 3500 yr later than the evidence for ringed seals in the paleontological record of southeast Alaska, and there is no definitive evidence that these earliest occupants of southeast Alaska were sea mammal hunters.
DISCUSSION
Both marine invertebrate and pinniped remains have been retrieved from the Bering Glacier foreland. These remains were entrained in the Bering Glacier ice when it excavated an ancient seafloor at the base of the mountain front. The forelands were flooded between ~16,000 and 5500 cal yr BP, creating a shoreline 40–55 km inland (northward) from the present one (Crossen and Lowell, Reference Crossen and Lowell2010; Pasch et al., Reference Pasch, Foster and Irvine2010; Shennan and Hamilton, Reference Shennan and Hamilton2010). Incursion of salt water on this deglaciated topography would have formed an irregular shoreline with fiords, bays, beaches, and rocky headlands. This may well have been a highly productive, complex coastline on which substantial edible and economically useful invertebrate and sea mammal populations existed (Crossen and Lowell, Reference Crossen and Lowell2010).
The shellfish and pinniped remains from the Bering Glacier foreland show that a variety of marine resources would have been available to any late Pleistocene humans colonizing coastal Alaska. A shoreline with sheltered bays would have provided areas for hunting, camping, and protection from storms to humans in boats (Fig. 4). This shoreline would have been distinctly different from the dangerous coast open to storm waves that exists in the vicinity of Bering Glacier today (Fig. 3).
CONCLUSION
The evidence for late Pleistocene/early Holocene sea mammal and shellfish remains from the Bering Glacier foreland, some as early as 16,000 cal yr BP, described here suggests that the coastal environment of the northern Gulf of Alaska could have provided food as well as shoreline conditions favorable for early colonists. If so, the nearest ice-free refugia would likely have been near the mouths of the Copper River and Lituya Bay (Mann, Reference Mann1986) in the eastern Gulf of Alaska, as well as portions of some islands of Prince William Sound (Detterman and Reed, Reference Detterman and Reed1973). Farther west, extensive areas of ice-free refugia may have been available on southwest Kodiak Island during the LGM (Karlstrom and Ball, Reference Karlstrom and Ball1969) and on the Shumagin Islands by 16,000 cal yr BP (Misarti et al., Reference Misarti, Finney, Jordan, Maschner, Addison, Shapley, Krumhardt and Beget2012). A portion of the Cook Inlet region was not covered by glacial deposits; some upland areas, such as the Caribou Hills of the Kenai Peninsula, were clearly ice free even at the LGM (Reger, Reference Reger1983; Reger and Pinney, Reference Reger and Pinney1996). Tusk fragments of woolly mammoth (Mammuthus primigenius) from the Kenai Peninsula have recently been dated to just prior to the LGM, at 27,400–28,200 BP (Klein et al., Reference Klein, Reger and Berg2014).
However, no evidence has yet appeared proving human occupation of the Gulf of Alaska coastline (or surrounding regions) during that time (or prior to 12,500 cal yr BP). A number of possible scenarios emerge from these data. Marine resources, including both sea mammals and edible shellfish, may have been present during the late Pleistocene/early Holocene period but were unexploited by human populations who were not yet present in the region. It is possible that they did not use a specifically coastal route, perhaps migrating from interior Alaska to the coast somewhere east of the Copper River delta. Alternatively, human populations may have been present on the coast but were not utilizing these marine resources. It is also possible that archaeological sites relating to early coastal migrants may simply not yet have been discovered. Finally, early coastal sites may have been destroyed by Holocene sea level rise, but there are parts of the Gulf of Alaska where such evidence should be preserved—particularly on tectonically uplifted surfaces in the eastern Gulf of Alaska region (Plafker and Rubin, Reference Plafker and Rubin1978)—yet to date no such evidence has been found. Nevertheless, the data presented here suggest that a variety of invertebrate and sea mammal resources were likely available to any late Pleistocene humans interested in colonizing the Gulf of Alaska region.
ACKNOWLEDGMENTS
We would like to thank the Alaska Regional Office of the U.S. Bureau of Land Management for field support, and the Department of Mammalogy, University of Alaska Fairbanks Museum of the North for the use of comparative osteological collections. Funds for radiocarbon dating of marine mammal specimens were provided by a University of Alaska Anchorage Faculty Research Grant to KJC. Aron Crowell, director of the Anchorage office of the Arctic Studies Center, Smithsonian Institution, assisted in the drafting of Figure 1.