Successful human socialisation is heavily influenced by the abilities to detect and understand cognitive and emotional processes in others. These abilities are referred to as the Theory of Mind (ToM) and empathy (Gallese, Reference Gallese2003; Young et al. Reference Young, Camprodon, Hauser, Pascual-Leone and Saxe2010; Keuken et al. Reference Keuken, Hardie, Dorn, Dev, Paulus, Jonas, Van den Wildenberg and Pineda2011; Krall et al. Reference Krall, Volz, Oberwelland, Grefkes, Fink and Konrad2016). Clinicians and researchers use these terms interchangeably, but there is no universal consensus on their definitions and constructs. For example, some authors regard empathy as a two-component construct with affective and cognitive components (Reniers et al. Reference Reniers, Corcoran, Drake, Shryane and Völlm2011), while others (Blair, Reference Blair2005) have proposed a three-component construct by adding a motor component to reflect the act of mirroring the motor responses of the observed person (motor empathy). Some commentators view cognitive empathy as synonym to ToM, which is the ability to attribute mental states, such as desires, intentions, and beliefs, to others (Frith & Frith, Reference Frith and Frith1999). Some authors have favoured a ToM model with two distinct components, namely affective and cognitive (Kalbe et al. Reference Kalbe, Schlegel, Sack, Nowak, Dafotakis, Bangard, Brand, Shamay-Tsoory, Onur and Kessler2010). Others have suggested that empathy and ToM encompass similar underlying abilities that are discernible at the neural level (Reniers et al. Reference Reniers, Völlm, Elliott and Corcoran2014). More recently, Dvash & Shamay-Tsoory (Reference Dvash and Shamay-Tsoory2014) argued in favour of a two-component construct of empathy, namely emotional and cognitive empathy (also refered to as ToM), with distinct neuroanatomical underpinnings (Fig. 1). According to this model, cognitive empathy (ToM) has two distinct subcomponents, namely affective ToM and cognitive ToM.
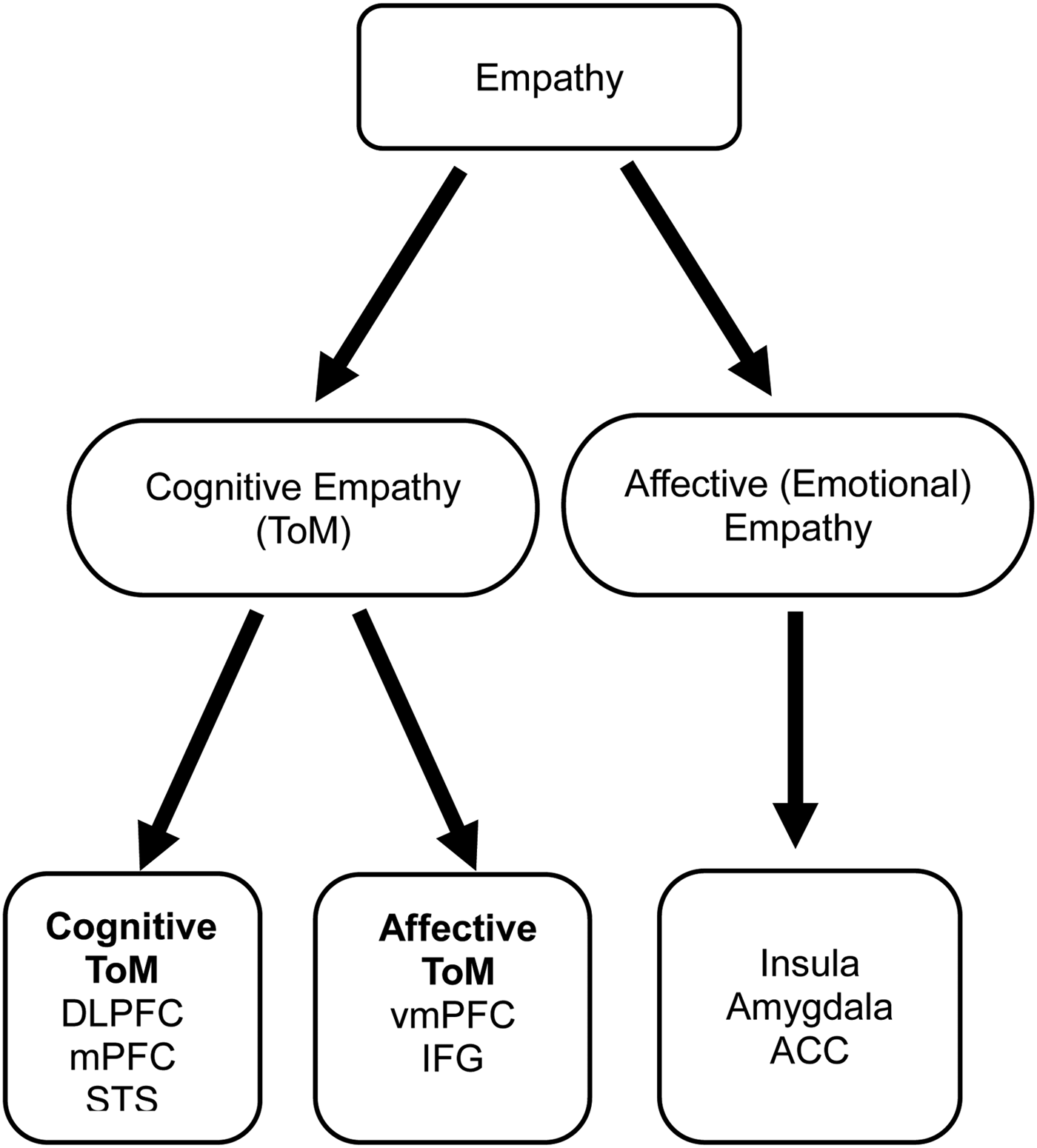
Fig. 1. Empathy system adapted from Dvash & Shamay-Tsoory (Reference Dvash and Shamay-Tsoory2014). ACC, anterior cingulate cortex; DLPFC, dorsolateral prefrontal cortex; IFG, inferior frontal gyrus; mPFC, medial prefrontal cortex; STS, superior temporal sulcus; ToM, Theory of Mind; TPJ, temporoparietal junction; vmPFC, ventromedial prefrontal cortex.
Several brain regions have been implicated in cognitive ToM, including medial prefrontal cortex (mPFC), dorsolateral prefrontal cortex (DLPFC), temporoparietal junction (TPJ), and temporal poles (Frith & Frith, Reference Frith and Frith1999; Völlm et al. Reference Völlm, Taylor, Richardson, Corcoran, Stirling, McKie, Deakin and Elliott2006; Carrington & Bailey, Reference Carrington and Bailey2009; Reniers et al. Reference Reniers, Völlm, Elliott and Corcoran2014). Brain areas implicated in the regulation of affective ToM include mPFC, particularly the ventral portion (Shamay-Tsoory & Aharon-Peretz, Reference Shamay-Tsoory and Aharon-Peretz2007; Shamay-Tsoory et al. Reference Shamay-Tsoory, Aharon-Peretz and Perry2009; Sebastian et al. Reference Sebastian, Fontaine, Bird, Blakemore, De Brito, McCrory and Viding2012), inferior frontal gyrus (IFG), anterior cingulate cortex, and amygdala (Shamay-Tsoory et al. Reference Shamay-Tsoory, Aharon-Peretz and Perry2009; Gonzalez-Liencres et al. Reference Gonzalez-Liencres, Shamay-Tsoory and Brune2013; Gentili et al. Reference Gentili, Cristea, Ricciardi, Costescu, David and Pietrini2015).
Self-report inventories commonly used to measure empathy include the Hogan Empathy Scale (Hogan, Reference Hogan1969), the Interpersonal Reactivity Index (IRI; Egger et al. Reference Egger, Smith, Schneider and Minder1997), the Balanced Emotional Empathy Scale (BEES; Mehrabian, Reference Mehrabian2000), the Empathy Quotient (EQ; Behan et al. Reference Behan, Stone and Garavan2015), and the Questionnaire of Cognitive and Affective Empathy (QCAE; Reniers et al. Reference Reniers, Corcoran, Drake, Shryane and Völlm2011). Behavioural measures of cognitive empathy (ToM) are primarily performance-based and include such tasks as first-order (Baron-Cohen et al. Reference Baron-Cohen, Leslie and Frith1985) and second-order false-belief (Baron-Cohen, Reference Baron-Cohen1989) tasks for assessing cognitive ToM, the Reading the Mind in the Eyes (RMET) for evaluating affective ToM (Baron-Cohen et al. Reference Baron-Cohen, Wheelwright, Hill, Raste and Plumb2001), and the Faux Pas Recognition (FPR) test (Stone et al. Reference Stone, Baron-Cohen and Knight1998) and the Yoni task (Shamay-Tsoory & Aharon-Peretz, Reference Shamay-Tsoory and Aharon-Peretz2007) for assessing both affective and cognitive ToM.
Impairment of social functioning consequent upon impaired empathy has been reported in a range of neuropsychiatric conditions, including psychopathy, antisocial personality disorder (Dolan & Fullam, Reference Dolan and Fullam2004), schizophrenia (Bragado-Jimenez & Taylor, Reference Bragado-Jimenez and Taylor2012), major depressive disorder (MDD; Schreiter et al. Reference Schreiter, Pijnenborg and Aan Het Rot2013), autistic spectrum disorder (ASD; Shimoni et al. Reference Shimoni, Weizman, Yoran and Raviv2012), temporal lobe epilepsy (Li et al. Reference Li, Chiu, Yeh, Liou, Cheng and Hua2013), Alzheimer's disease (Laisney et al. Reference Laisney, Bon, Guiziou, Daluzeau, Eustache and Desgranges2013), Parkinson's disease (Yu et al. Reference Yu, Wu, Chiu, Tai, Lin and Hua2012), and other neurodegenerative diseases (Poletti et al. Reference Poletti, Enrici and Adenzato2012). Empathy is highly correlated with violence (Jolliffe & Farrington, Reference Jolliffe and Farrington2004) and plays a pivotal role in the violence inhibition system (Blair et al. Reference Blair, Mitchell and Blair2005). Thus, enhancement of empathy has been regarded as a major treatment goal in criminogenic programmes (Day et al. Reference Day, Casey and Gerace2010; Reidy et al. Reference Reidy, Kearns and DeGue2013). However, conventional psychological interventions for empathy enhancement have proved less effective in certain offender groups, particularly those with psychopathy (Reidy et al. Reference Reidy, Kearns and DeGue2013), highlighting the need to develop alternative therapeutic interventions to enhance empathy, of which transcranial magnetic stimulation (TMS), especially its repetitive format (rTMS), is an example (Glenn & Raine, Reference Glenn and Raine2008; Glannon, Reference Glannon2014).
TMS is a non-invasive technique used to deliver brief, high-intensity magnetic pulses to the brain inducing localised neuronal depolarisation to regulate cortical excitability that underlies the modulation of cortical networks (Luber & Lisanby, Reference Luber and Lisanby2014). In general, high-frequency (⩾5 Hz) rTMS and its newer version, intermittent θ burst stimulation, facilitate cortical excitability, whereas low-frequency (about 1 Hz) rTMS and continuous θ burst stimulation contribute to opposite effects (Pascual-Leone et al. Reference Pascual-Leone, Walsh and Rothwell2000; Huang et al. Reference Huang, Edwards, Rounis, Bhatia and Rothwell2005; Wassermann & Zimmermann, Reference Wassermann and Zimmermann2012). rTMS has been used to treat a variety of neurological and psychiatric diseases (see Wassermann & Zimmermann, Reference Wassermann and Zimmermann2012) and to enhance cognitive functions in healthy volunteers (see Hsu et al. Reference Hsu, Ku, Zanto and Gazzaley2015) and in people with MDD (Serafini et al. Reference Serafini, Pompili, Belvederi Murri, Respino, Ghio, Girardi, Fitzgerald and Amore2015). Online Supplementary Table S1 provides more information about the effects of TMS in clinical populations. Additionally, rTMS has been used to modulate empathy with some promising effects (see Hetu et al. Reference Hetu, Taschereau-Dumouchel and Jackson2012; Schuwerk et al. Reference Schuwerk, Langguth and Sommer2014a ). However, findings are inconsistent likely due to differences in the tasks used to measure empathy, experimental designs, targeted brain regions, and rTMS parameters, including the paradigms used (i.e. online or offline), stimulus intensity [measured as a percentage of resting motor threshold (rMT) or of maximum stimulator output (MSO)], frequency, and number of pulses.
We therefore aimed to conduct a systematic review and meta-analysis of the literature on the effects of rTMS on empathy in healthy and clinical populations to integrate the evidence base and to determine if certain TMS parameters or brain regions selected are associated with stronger effects on specific domains of empathy. While effective interventions involving healthy individuals could potentially be extended to clinical populations, as we shall describe later in this review, all the studies included in this review, bar one study, involved interventions in healthy groups. Due to the overlaps between the concepts of empathy and ToM, in this review we have conceptualised empathy in accordance with the model proposed by Dvash & Shamay-Tsoory (Reference Dvash and Shamay-Tsoory2014) as outlined above. We followed PRISMA-P guidelines (Moher et al. Reference Moher, Shamseer, Clarke, Ghersi, Liberati, Petticrew, Shekelle and Stewart2015; Shamseer et al. Reference Shamseer, Moher, Clarke, Ghersi, Liberati, Petticrew, Shekelle and Stewart2015) in the reporting of this review where applicable.
Method
Data sources
Using the terms ‘transcranial magnetic stimulation’ or ‘TMS’ combined with ‘theory of mind’, ‘ToM’, ‘empath$’, ‘mentali$’, ‘role taking’, or ‘perspective taking’, a systematic search of the literature on the effects of TMS on empathy was conducted on 25 May 2016 of seven electronic databases (AMED, Cochrane library, EMBASE, Medline, PsycInfo, Pubmed, Web of Science). The International Clinical Trials Registry Platform (World Health Organisation), Dissertation Abstracts, Google, and the library catalogues of the University of Nottingham were also searched to identify grey literature in the field. No filters were added regarding the age of study participants, publication time or language of publication (see online Supplementary Table S2 for search syntax). References of eligible articles were searched manually for potentially eligible studies missed by the electronic searches.
Study selection
Empirical studies were included in the review if they: (1) involved adult participants without dementia or other major neurological conditions; (2) used rTMS as an active intervention; (3) had a comparison group or control condition; and (4) used behavioural tasks to assess empathy. Of the 508 papers originally identified, 22 met the inclusion criteria (see online Supplementary Fig. S1 and Table S3) and were quality assessed using the quality assessment tool for quantitative studies (National Collaborating Centre for Methods & Tools, 2008) on the domains of selection bias, study design, confounders, blinding, data collection method, withdrawals and dropouts, intervention integrity, and statistical analyses.
Of the 22 studies included in the review, four (Uddin et al. Reference Uddin, Molnar-Szakacs, Zaidel and Iacoboni2006; Balconi et al. Reference Balconi, Crivelli and Bortolotti2010; Hoekert et al. Reference Hoekert, Vingerhoets and Aleman2010; Lev-Ran et al. Reference Lev-Ran, Shamay-Tsoory, Zangen and Levkovitz2012) were excluded from the meta-analyses due to lack of sufficient data to allow effect size calculation and only after exhausting attempts to obtain this information from the authors.
Data extraction and analyses
A standardised form was used to extract information concerning authors, study objectives, sample characteristics, inclusion/exclusion criteria, study design, experimental processes, rTMS protocols, outcome variables, and analytic strategy.
We originally intended to conduct separate meta-analyses of studies involving clinical populations and healthy individuals using the random-effects model and, where applicable, in accordance with the model proposed by Dvash & Shamay-Tsoory (Reference Dvash and Shamay-Tsoory2014) with its components: cognitive empathy (i.e. ToM, including cognitive ToM and affective ToM) and affective empathy. However, this has not been possible due to there being only one study in the field (Enticott et al. Reference Enticott, Fitzgibbon, Kennedy, Arnold, Elliot, Peachey, Zangen and Fitzgerald2014). Therefore, the meta-analyses presented in this review include only studies involving healthy subjects. Measures of cognitive ToM included the cognitive component of the Yoni task, moral judgement, false-belief tasks, and action-understanding tools. Measures of affective ToM included the RMET, tasks of facial expression recognition, the affective component of the Yoni task, affective go/no-go tasks, the faux pas test, and emotional egocentricity. While it can be argued that facial expression recognition is not a test of empathic abilities, the model proposed by Dvash & Shamay-Tsoory (Reference Dvash and Shamay-Tsoory2014) regards emotional recognition as a component of affective ToM. This view has been supported by other commentators (Poletti et al. Reference Poletti, Enrici and Adenzato2012). Therefore, tasks measuring emotional recognition, such as facial expression recognition taks, were included in the review.
Effect size was regarded as positive if the active rTMS effect was in the predicted direction and negative if it was in the opposite direction. Moreover, when a study entailed multiple stimulation sites, each trial of the different stimulation sites was used as the unit of analysis for the purpose of meta-analysis. A pooled effect size was used if a study provided multiple outcomes (e.g. accuracy and reaction time, score of each subscale, or short-term and long-term performance). Only the comparison between experimental and sham group (condition) was selected when a trial consisted of more than one control group or condition (e.g. one group receiving rTMS at a control site and another receiving sham stimulation). Effect sizes represented as Hedges’ g and 95% confident intervals (CI) were calculated according to the differences between experimental (real stimulation) and control (sham stimulation) conditions in post-stimulation evaluations or ‘online’ performance divided by pooled standard deviation.
The Q and I 2 statistics (Higgins & Thompson, Reference Higgins and Thompson2002; Higgins et al. Reference Higgins, Thompson, Deeks and Altman2003) were used to assess consistency between studies. The Q statistic represents the level of heterogeneity, while the I 2 index specifies the total variation from between-study variance. A p value ⩽0.05 and an I 2 value of >40% were deemed as indicative of moderate heterogeneity. Funnel plots (Egger & Smith, Reference Egger and Smith1995), the Egger test (Egger et al. Reference Egger, Smith, Schneider and Minder1997), and Begg and Mazumdar rank correlation tests (Begg & Mazumdar, Reference Begg and Mazumdar1994) were used to test for the presence of a potential publication bias. In cases where publication bias was evident, the Trim and Fill procedure (Egger & Smith, Reference Egger and Smith1995) was applied to correct it.
In order to identify variables that could contribute to alternation of empathy, pre-specified subgroup analyses were performed with the unit of trial by merging the data according to the rTMS parameters, including effect (‘excitatory’ v. ‘inhibitory’), stimulation paradigm (‘online’ v. ‘offline’), study design (‘randomised’ v. ‘non-randomised’), stimulation site, and task of outcome measurement.
Meta-regression was employed to examine the impact of between-study variation on study effect sizes. The effect size from each trial was set as the dependent variable while age, gender, intensity of stimulation, total pulses per condition, and weighted number of pulses (i.e. total number of rTMS pulses multiplied by intensity) were selected as predictor variables. All the quantitative analyses were performed using Stata 13.1 (StataCorp, 2013).
Results
Study characteristics
Table 1 summaries study characteristics. In summary, 22 studies involving 466 participants (82% males; mean age 24.45 years; range 18–59 years) were included in the review. For studies recruiting participants from clinical populations, there was only one study (Enticott et al. Reference Enticott, Fitzgibbon, Kennedy, Arnold, Elliot, Peachey, Zangen and Fitzgerald2014), recruiting patients with ASD as subjects. Sixteen of the included studies were conducted in Europe, three in North America (Uddin et al. Reference Uddin, Molnar-Szakacs, Zaidel and Iacoboni2006; Young et al. Reference Young, Camprodon, Hauser, Pascual-Leone and Saxe2010; Keuken et al. Reference Keuken, Hardie, Dorn, Dev, Paulus, Jonas, Van den Wildenberg and Pineda2011), two in Australia (Krause et al. Reference Krause, Enticott, Zangen and Fitzgerald2012; Enticott et al. Reference Enticott, Fitzgibbon, Kennedy, Arnold, Elliot, Peachey, Zangen and Fitzgerald2014), and one in Israel (Lev-Ran et al. Reference Lev-Ran, Shamay-Tsoory, Zangen and Levkovitz2012). The most common study design employed was non-randomised cross-over (n = 15), allocating the sequence of intervention conditions with counterbalancing (n = 10) or unspecified (n = 5) method. Of the six studies randomly allocating participants, two (Keuken et al. Reference Keuken, Hardie, Dorn, Dev, Paulus, Jonas, Van den Wildenberg and Pineda2011; Enticott et al. Reference Enticott, Fitzgibbon, Kennedy, Arnold, Elliot, Peachey, Zangen and Fitzgerald2014) were parallel randomised controlled trials and the other four (Costa et al. Reference Costa, Torriero, Oliveri and Caltagirone2008; Kalbe et al. Reference Kalbe, Schlegel, Sack, Nowak, Dafotakis, Bangard, Brand, Shamay-Tsoory, Onur and Kessler2010; Giardina et al. Reference Giardina, Caltagirone and Oliveri2011; Lev-Ran et al. Reference Lev-Ran, Shamay-Tsoory, Zangen and Levkovitz2012) were randomised cross-over trials. The remaining one between-subject study (Silani et al. Reference Silani, Lamm, Ruff and Singer2013) did not mention the method of participant allocation.
Table 1. Characteristics of included rTMS studies on empathy

ACC, anterior cingulate cortex; ASD, autistic spectrum disorder; CCR, counterbalanced cross-over design; CCT, clinical controlled trial; cTBS, continuous θ burst stimulation; DLPFC, dorsolateral prefrontal cortex; Exp: experiment; IFG, inferior frontal gyrus; IPL, inferior parietal lobule; IRI, Interpersonal Reactivity Index; M1, primary motor cortex; mPFC, medial prefrontal cortex; MSO, maximum of stimulator output; NA, not available; RCR, randomised cross-over design; RCT, randomised controlled trial; RMET, Reading the Mind in the Eye Test; rMT, resting motor threshold; rTMS, repetitive transcranial magnetic stimulation; S1, primary somatosensory area; SMG, supramarginal gyrus; TPJ, temporoparietal junction; UCR, cross-over design with unknown allocation.
a Presented as number of participants included in final analysis and the number of participants in subgroups in the parenthesis.
b Not included for meta-analysis.
c No randomisation method reported.
d Presented as the original sex ratio.
e Presented as the sex ratio of participants in the whole study.
Various tasks were used to assess empathy, including facial expression recognition tasks with materials derived from Ekman & Friesen (Reference Ekman and Friesen1976), the RMET or its modified version, the Yoni task, scenarios using video clips assessing individuals’ capability of social judgement or action understanding, the false belief task and the faux pas task. With regard to published self-report instruments, only one study (Enticott et al. Reference Enticott, Fitzgibbon, Kennedy, Arnold, Elliot, Peachey, Zangen and Fitzgerald2014) selected a self-report measure, the IRI, as the empathy measure. The number of pulses within each experimental session ranged from 120 to 3000. The majority of the reviewed studies (n = 15) set the intensity of the pulses to 100% or more of rMT, while other four studies used subthreshold intensity (Costa et al. Reference Costa, Torriero, Oliveri and Caltagirone2008; Hoekert et al. Reference Hoekert, Vingerhoets and Aleman2010; Giardina et al. Reference Giardina, Caltagirone and Oliveri2011; Michael et al. Reference Michael, Sandberg, Skewes, Wolf, Blicher, Overgaard and Frith2014). The remaining three studies (Young et al. Reference Young, Camprodon, Hauser, Pascual-Leone and Saxe2010; Keuken et al. Reference Keuken, Hardie, Dorn, Dev, Paulus, Jonas, Van den Wildenberg and Pineda2011; Krall et al. Reference Krall, Volz, Oberwelland, Grefkes, Fink and Konrad2016) selected MSO as the index of intensity. The DLPFC, mPFC (ventral or dorsal portion), TPJ, and IFG were targeted as the main sites for stimulation. The most common control condition was vertex stimulation (n = 11). Five studies did not report the detail of their sham protocol.
Quality assessment
Of the twenty-two studies included, only one study (Enticott et al. Reference Enticott, Fitzgibbon, Kennedy, Arnold, Elliot, Peachey, Zangen and Fitzgerald2014) attracted a rating of ‘strong’, 19 studies were rated as ‘moderate’, and two studies as ‘weak’ (online Supplementary Table S4). Poor rating on selection bias was the most common reason for not reaching the ‘strong’ quality threshold. The two weak ratings were due to vulnerability to confounders (Silani et al. Reference Silani, Lamm, Ruff and Singer2013) and poor description of the reliability and validity of the outcome measures used (Michael et al. Reference Michael, Sandberg, Skewes, Wolf, Blicher, Overgaard and Frith2014). For rTMS reproducibility, most of the reviewed studies (n = 16) provided all necessary parameters, but two studies (Balconi et al. Reference Balconi, Crivelli and Bortolotti2010; Silani et al. Reference Silani, Lamm, Ruff and Singer2013) failed to provide information in relation to the type of coil utilised, and four studies (Pobric & Hamilton, Reference Pobric and Hamilton2006; Costa et al. Reference Costa, Torriero, Oliveri and Caltagirone2008; Balconi et al. Reference Balconi, Bortolotti and Gonzaga2011; Balconi & Bortolotti, Reference Balconi and Bortolotti2012) lacked comprehensive information about the duration of the intervention. Only three studies described adverse effects relating to the administration of rTMS, with one study indicating no adverse effects observed (Young et al. Reference Young, Camprodon, Hauser, Pascual-Leone and Saxe2010) and the other two studies reporting minor post-rTMS side effects (Enticott et al. Reference Enticott, Fitzgibbon, Kennedy, Arnold, Elliot, Peachey, Zangen and Fitzgerald2014) and one syncope event (Kalbe et al. Reference Kalbe, Schlegel, Sack, Nowak, Dafotakis, Bangard, Brand, Shamay-Tsoory, Onur and Kessler2010).
Meta-analysis
Effects of rTMS on empathy in clinical populations
Since there was only one trial (Enticott et al. Reference Enticott, Fitzgibbon, Kennedy, Arnold, Elliot, Peachey, Zangen and Fitzgerald2014) involving participants with a mental disorder, it was not possible to conduct a meta-analysis to examine the rTMS effect on empathy in clinical populations. This study (Enticott et al. Reference Enticott, Fitzgibbon, Kennedy, Arnold, Elliot, Peachey, Zangen and Fitzgerald2014) showed that deep high-frequency rTMS applied bilaterally to the dorsal mPFC in patients with ASD did not have a statistically significant facilitatory effects on empathy (g = −0.22; 95% CI −1.55 to −0.01, p = 0.016), cognitive empathy (g = −0.32; 95% CI −1.07 to 0.44, p = 0.41), or affective empathy (g = 0.08; 95% CI −0.66 to 0.82, p = 0.21).
Effects of rTMS on empathy in healthy volunteers
Twenty-four trials extracted from reports of 17 studies were included for the meta-analysis of the effects of rTMS on empathy. This revealed a significant small overall effect size (g = 0.29; 95% CI 0.10 to 0.48, p = 0.003) as plotted in Fig. 2a . A moderate level of heterogeneity was observed across the studies (Q 23 = 39.22, p = 0.019; I 2 = 41.4%). Separate meta-analyses were conducted for trials involving cognitive empathy with its two components; cognitive and affective ToM. However, it was not possible to conduct a meta-analysis on the effects of rTMS on affective empathy due to lack of studies in the field.

Fig. 2. (a) Statistical summary and forest plot of effect sizes for empathy. (b) Statistical summary and forest plot of effect sizes for cognitive ToM. (c) Statistical summary and forest plot of effect sizes for affective ToM. DLPFC, dorsolateral prefrontal cortex; ES, effect size; IFG, inferior frontal gyrus; mPFC, medial prefrontal cortex; PMC, primary motor cortex; S1, primary somatosensory area; SMG, supramarginal gyrus; TPJ, temporoparietal junction TBS, theta burst stimulation; TPJ, temporoparietal junction.
Effects of rTMS on cognitive ToM
The meta-analysis of findings from 16 trials on the effects of rTMS on cognitive ToM showed a non-significant mean effect (g = 0.12, 95% CI −0.15 to 0.40, p = 0.39; see also Fig. 2b ). The trim and fill procedure applied suggested an estimated mean effect size of −0.13 after imputing five missing trials (online Supplementary Fig. S2b). A moderate heterogeneity was found across trials (Q 16 = 30.64, p = 0.01; I 2 = 51.0%). The funnel plot was asymmetrical by visual inspection (online Supplementary Fig. S2a), but neither the Begg's test (z = 0.95, p = 0.34) nor the Egger's test (intercept16 = 2.42, t = 1.18, two-tailed p = 0.26) suggested publication bias.
The subgroup analyses (Table 2) revealed a non-significant mean effect for inhibitory rTMS (g = 0.03, 95% CI −0.27 to 0.33, p = 0.83) but a significant one for excitatory rTMS (g = 0.58, 95% CI 0.05 to 1.10, p = 0.03). For the stimulation paradigm, since all trials with offline paradigms applied inhibitory rTMS and all trials with online paradigms applied excitatory rTMS, the results of the subgroup analysis were the same (offline: g = 0.03, 95% CI −0.27 to 0.33, p = 0.83; online: g = 0.58, 95% CI 0.05 to 1.10, p = 0.03). Moreover, the subgroup analysis for study designs revealed a non-significant mean effect size for trials with randomised design (g = −0.16, 95% CI −0.56 to 0.25, p = 0.45) but a significant one for trials with non-randomised design (g = 0.40, 95% CI 0.13–0.67, p = 0.004). Furthermore, the subgroup analysis for stimulation sites revealed non-significant mean effect sizes for all stimulation sites, including TPJ (g = 0.26, 95% CI −0.04 to 0.56, p = 0.09), DLPFC (including IFG) (g = −0.09, 95% CI −0.71 to 0.53, p = 0.79), and mPFC (g = 0.04, 95% CI −0.44 to 0.52, p = 0.87). Finally, the subgroup analysis for the nature of outcome measure tasks revealed non-significant mean effect sizes for false-belief tasks (g = 0.10, 95% CI −0.21 to 0.41, p = 0.51) and intention attribution tasks (g = −0.10, 95% CI −0.57 to 0.37, p = 0.69) but a significant large mean effect size for action-understanding tasks (g = 0.82, 95% CI 0.34–1.30, p = 0.001).
Table 2. Subgroup analyses

CI, confidence interval; DLPFC, dorsolateral prefrontal cortex; IFG, inferior frontal gyrus; mPFC, medial prefrontal cortex; ToM, Theory of Mind; TPJ, temporoparietal junction.
*p < 0.05.
The meta-regression analysis across trials showed that none of between-study variables significantly predicted the effects of rTMS (mean age of participants: β = 0.08, p = 0.55; gender ratio: β = −1.01, p = 0.11; intensity of stimulation: β = −0.03, p = 0.26; number of pulses per condition: β = −0.005, p = 0.45; weighted number of pulses: β = 0.005, p = 0.48).
Effects of rTMS on affective ToM
The meta-analysis of results from 15 trials on the effects of rTMS on affective ToM showed a significant small mean effect (g = 0.26, 95% CI 0.02–0.50, p = 0.03) with a moderate heterogeneity (Q 14 = 25.98, p = 0.03; I 2 = 46.1%; see also Fig. 2c ). The funnel plot (online Supplementary Fig. S3a) and the Egger's test (intercept17 = −4.39, t = −2.55, two-tailed p = 0.02) showed evidence of publication bias. However, the Begg's test (z = 1.48, p = 0.14) and the trim and fill procedure did not show evidence of publication bias.
Further subgroup analyses showed that the mean effect size of inhibitory rTMS trials failed to reach statistical significance (g = 0.25, 95% CI −0.00 to 0.51, p = 0.052). It was not possible to calculate the mean effect size for excitatory rTMS since there was only one trial (Balconi & Canavesio, Reference Balconi and Canavesio2013) in this subgroup that showed a positive effect (g = 0.33). For stimulation paradigms, trials with ‘offline’ paradigms revealed a non-significant mean effect (g = 0.10, 95% CI −0.12 to 0.32, p = 0.35), while trials with ‘online’ paradigm showed a significant moderate effect (g = 0.52, 95% CI 0.05 to 1.00, p = 0.03). The subgroup analysis for study design revealed a non-significant mean effect size for trials with randomised design (g = −0.06, 95% CI −0.36 to 0.24, p = 0.71) but a significant one for trials with non-randomised design (g = 0.43, 95% CI 0.123 to 0.73, p = 0.006). Regarding the sites of stimulation, all three locations revealed non-significant mean effect sizes [TPJ: g = −0.14, 95% CI −0.74 to 0.46, p = 0.65; DLPFC (including IFG): g = 0.28, 95% CI −0.35 to 0.91, p = 0.39; mPFC: g = 0.22, 95% CI −0.07 to 0.52, p = 0.14]. For type of measurement, the mean effect sizes for trials using emotion recognition tasks (g = 0.32, 95% CI −0.06 to 0.69, p = 0.10) and faux-pas recognition tasks (g = −0.08, 95% CI −0.50 to 0.35, p = 0.73) were not significant.
The meta-regression analysis across trials showed that none of between-study variables significantly predicted the effects of rTMS (mean age of participants: β = 0.07, p = 0.44; gender ratio: β = −0.68, p = 0.22; intensity of stimulation: β = 0.15, p = 0.07; number of pulses per condition: β = 0.02, p = 0.11; weighted number of pulses: β = −0.02, p = 0.11).
Discussion
This study aimed to examine the literature on the effects of rTMS on empathy and, where relevant, to determine which intervention parameters were associated with stronger effects. Our findings show that rTMS has a significant but small overall effect on empathy in healthy participants and that this effect varied according to empathy domains, cognitive or affective ToM. It has not been possible to draw valid conclusions regarding the effect of rTMS on empathy in clinical population as there was only one study conducted in the field.
The meta-analysis of rTMS studies relating to cognitive ToM revealed a non-significant effect size indicating that rTMS may not be effective in modulating cognitive ToM. Moreover, the results suggested that there might be five unpublished trials investigating this issue with negative findings. In contrast, a significant effect size was found on the meta-analysis of rTMS studies for affective ToM though the magnitude of effect was small. These findings of dissimilar effects of rTMS support the idea of examining subcomponents of empathy separately as they are associated with distinct brain regions (Dvash & Shamay-Tsoory, Reference Dvash and Shamay-Tsoory2014).
Our subgroup analyses further identified parameters associated with a positive effect of rTMS, including excitatory v. inhibitory rTMS and online v. offline paradigms. However, these finding should be interpreted with caution due to the relatively small number of trials, particularly for excitatory rTMS. Although previous studies (Robertson et al. Reference Robertson, Theoret and Pascual-Leone2003) suggest that the duration of the rTMS after-effect only persists for half of the stimulation time, physiological evidence indicates that the rTMS after-effect decays gradually with time (Eisenegger et al. Reference Eisenegger, Treyer, Fehr and Knoch2008). Nevertheless, given that completion of conventional tasks measuring empathy is time-consuming, it is less likely to detect significant rTMS effect on empathy from experiments with offline paradigm.
Surprisingly, the subgroup analysis by stimulation site did not reveal statistically significant mean effects across different brain regions pertaining to specific empathetic components. The literature suggests differential roles of specific brain regions: the dorsal part of mPFC and TPJ (particularly the right side) for cognitive ToM (Denny et al. Reference Denny, Kober, Wager and Ochsner2012) and the ventral part of mPFC and IFG for affective ToM (Sebastian et al. Reference Sebastian, Fontaine, Bird, Blakemore, De Brito, McCrory and Viding2012; Dal Monte et al. Reference Dal Monte, Schintu, Pardini, Berti, Wassermann, Grafman and Krueger2014). It would thus be expected to find significant effects if rTMS is administered to these regions, but not to other regions. However, we found no significant effect applying rTMS to TPJ for cognitive ToM or IFG for affective ToM and only one included trial (Keuken et al. Reference Keuken, Hardie, Dorn, Dev, Paulus, Jonas, Van den Wildenberg and Pineda2011) explored affective ToM targeting at these crucial regions (e.g. IFG), a firm conclusion cannot be drawn at this stage. It is worth noting here that t he issue of spatial resolution is an inherent limitation of TMS research. The issue may be further compromised when non-imaging-guided techniques are utilised to localise the stimulation sites. With this in mind, and since a considerable number of studies included in this review (Balconi et al. Reference Balconi, Crivelli and Bortolotti2010; Balconi & Bortolotti, Reference Balconi and Bortolotti2012; Krause et al. Reference Krause, Enticott, Zangen and Fitzgerald2012; Schuwerk et al. Reference Schuwerk, Langguth and Sommer2014b ) did not utilise imaging-guided techniques, we have categorised the studies according to the effects of TMS on relatively large regions of the brain rather than smaller ones while performing subgroup analyses. Nevertheless, the results need to be interpreted with caution.
Meta-regression revealed no differential effects in relation to participant characteristics (age, gender) or stimulation parameters (intensity, number of pulses, weighted number of pulses). This may be due to the low heterogeneity detected in relation to participants’ age and gender ratio. Contrary to the findings of other meta-analytic studies (Chou et al. Reference Chou, Hickey, Sundman, Song and Chen2015), rTMS parameters did not contribute significantly to effect sizes. A number of explanations exist as to why these findings were not replicated in this review. First, the number of studies included in this review was slightly higher than 10, the minimum number required to attain sufficient statistical power (Borenstein et al. Reference Borenstein, Hedges, Higgins, Rothstein, Borenstein, Hedges, Higgins and Rothstein2009). Second, the impact of the rTMS parameters may only be evident when rTMS is applied to the brain region corresponding to the task measured. Third, empathy is a multi-faceted construct involving a network of brain regions, and since the effects of TMS are dose-dependent, a larger number of sessions and pulses per session may be required to modulate empathy.
Future research should examine a number of pertinent issues. For example, some of the included studies (Balconi & Bortolotti, Reference Balconi and Bortolotti2013; Balconi & Canavesio, Reference Balconi and Canavesio2016) suggested that baseline level of empathy can moderate the inhibitory effect of low-frequency rTMS on facial emotional recognition. Interestingly, they found people with higher levels of empathy performed better under control conditions than those with lower levels of empathy when the activity of the dorsal mPFC was inhibited. However, for the effect of facilitatory rTMS for enhancing empathetic ability, the role of baseline empathy level has not yet been investigated, which is obviously a crucial issue for rTMS in clinical application. In addition, as speculated in a number of included studies, the behavioural tasks selected might not be appropriate for outcome measures due to their low sensitivity to detect rTMS-induced effects (Keuken et al. Reference Keuken, Hardie, Dorn, Dev, Paulus, Jonas, Van den Wildenberg and Pineda2011; Krause et al. Reference Krause, Enticott, Zangen and Fitzgerald2012; Lev-Ran et al. Reference Lev-Ran, Shamay-Tsoory, Zangen and Levkovitz2012; Enticott et al. Reference Enticott, Fitzgibbon, Kennedy, Arnold, Elliot, Peachey, Zangen and Fitzgerald2014; Schuwerk et al. Reference Schuwerk, Schecklmann, Langguth, Dohnel, Sodian and Sommer2014b ). Finally, it might be too simplistic to expect that increased excitability contributes to behavioural improvement and decreased excitability to deterioration as others have also suggested (Sandrini et al. Reference Sandrini, Umilta and Rusconi2011).
Strengths and limitations
A major strength of this study is that some of the studies included were relatively well designed with low dropouts rates and high reproducibility of rTMS protocols. However, the study suffered a number of limitations in relation to selection bias, reflected by restricted participants’ age range, recruitment resources and reporting adverse of effects, which is essential in TMS studies (Rossi et al. Reference Rossi, Hallett, Rossini and Pascual-Leone2009). Further, the subgroup analysis of study design showed that more significant effects were found in non-randomised than randomised trials. This raises the question whether the results of the current study may be vulnerable to some methodological limitations. However, since a majority of included studies were rated as equivalently moderate in quality assessment, the source of heterogeneity is less likely from allocation bias and needs further investigation. While the research on rTMS application into alteration of empathy is still in its infancy, this systematic review with meta-analysis applied a broad range of search terms to enrol eligible studies with variant outcome measures and different rTMS protocols. We included both randomised and non-randomised trials as a considerable number of studies in this field used non-randomised design. Multiple databases were thoroughly searched to minimise potential publication bias. However, a number of studies could not be included in the meta-analysis due to not reporting effect sizes, outcome measures not matching our inclusion criteria, and the presence of possible publication bias. The majority of included studies applied empathy tasks providing multiple outcomes, such as accuracy and reaction time. We dealt with these multiple outcomes by averaging the effect sizes though this may have underestimated the size of effect. The number of studies included in the meta-analysis is relatively small, and this in conjunction with considerable levels of heterogeneity across the studies may have affected the power of the study. Finally, only one study involving interventions in a clinical population was included in the review and no meta-analytic data could therefore be provided for clinical samples. This highlights the urgent need to conduct clinical trials in the field.
Conclusion
The present review with meta-analysis demonstrated that rTMS has a discernible contribution to the alteration in different components of empathy although the effect sizes may not be as favourable as expected. The most encouraging finding for clinical implications is the effect of excitatory rTMS on enhancing affective ToM. Therefore, this review may help researchers having an interest in exploring rTMS impacts on empathy tailor their rTMS protocols to maximise its effect. Future studies in the field can potentially examine the effects of excitatory rTMS in clinical populations with impaired empathetic capabilities, such as those with ASD, psychopathy, and schizophrenia. However, we do not currently know whether the same effects will be observed in these populations. rTMS parameters may have to be refined further to maximise the effects on crucial brain regions, and there is a need to develop ecologically validated and sensitive empathy tasks for rTMS experiments.
Supplementary material
The supplementary material for this article can be found at https://doi.org/10.1017/S003329171700232X.
Acknowledgements
This research received no specific grant from any funding agency, commercial or not-for-profit sectors.
Declaration of Interest
None.