Introduction
Excess excitation energy that cannot be used for photosynthesis may damage the photosynthetic apparatus and result in reduced efficiency of photosynthesis. Reduced efficiency of photosynthesis caused by high light intensities is called photoinhibition, and maximal photosystem II efficiency (F v/F m) has become the standard measure of photoinhibition in many studies (e.g. Adams et al. Reference Adams, Muller, Cohu and Demmig-Adams2013). Photoinhibition can be divided into dynamic and chronic photoinhibition. Dynamic photoinhibition is related mainly to safe dissipation of excess excitation energy by the xanthophyll cycle whereas chronic photoinhibition is caused by damage, especially of the D1 protein in PSII (Osmond Reference Osmond1994). Photoinhibition in lichens also depends on their degree of hydration. Moist thalli can be more susceptible to photoinhibition because the cortex transmits more light when wet (Gauslaa & Solhaug Reference Gauslaa and Solhaug2001). However, moist thalli may recover by metabolic mechanisms during low light or dark periods (Gauslaa & Solhaug Reference Gauslaa and Solhaug1996). If thalli are exposed to intermediate light levels in the dry state for long periods they become photoinhibited (Gauslaa et al. Reference Gauslaa, Coxson and Solhaug2012). Recovery by metabolic mechanisms is not possible in the dry state, and intermediate intensity light over long periods thus causes severe, chronic photoinhibition (Gauslaa et al. Reference Gauslaa, Coxson and Solhaug2012). However, dry thalli have higher cortical screening than wet thalli and may also be protected by dissipation of energy in the photosystems, apparent as chlorophyll fluorescence quenching that does not seem to be associated with the xanthophyll cycle (Heber et al. Reference Heber, Bilger, Turk and Lange2010).
Chlorophyll a fluorescence is widely used for measuring photosystem II (PSII) efficiency, an indicator of stress caused by high light and other adverse environmental factors in photosynthetic organisms (e.g. Baker Reference Baker2008). Maximal photosystem II efficiency has apparently become the most popular activity parameter in lichens. For the measurement of F v/F m, the sample needs to be dark-adapted before measurement to ensure that all reaction centres in PSII are fully oxidized. The recommended dark adaptation time in the fluorimeter manuals is often 15–30 minutes, and it is also stated that relaxation of F m for accurate measurement of F v/F m is enhanced in moderate light intensities of 20–40 µmol m−2 s−1. In the field, pre-dawn measurements of F v/F m might be the best way to achieve this (Maxwell & Johnson Reference Maxwell and Johnson2000; Baker Reference Baker2008). However, if lichens are photoinhibited they might require a much longer time to recover from photoinhibition (e.g. Gauslaa & Solhaug Reference Gauslaa and Solhaug1996).
Photoinhibition measured as a decrease in F v/F m might have several causes. Conversion of violaxanthin to zeaxanthin in high light intensities will result in a decrease in F v/F m that would normally relax within seconds or minutes. However, irreversible damage to the D1 protein depends on D1 protein resynthesis in PSII which requires a longer time for relaxation (e.g. Maxwell & Johnson Reference Maxwell and Johnson2000; Nath et al. Reference Nath, Jajoo, Poudyal, Timilsina, Park, Aro, Nam and Lee2013). According to the model for D1 protein resynthesis, the damaged D1 protein must be degraded before the newly synthesized D1 protein is inserted into PSII (Nath et al. Reference Nath, Jajoo, Poudyal, Timilsina, Park, Aro, Nam and Lee2013). In the cyanobacterium Cynechocystis, recovery from photoinhibition is dependent on low light levels which seems to be important for a protease inhibitor which breaks down damaged protein before synthesis of new D1 protein (Singh et al. Reference Singh, Yamamoto, Satoh, Aro and Kanervo2005). In pea plants, D1 protein degradation depends on low light levels. Therefore, photoinhibition after high light intensity treatment relaxes faster in low light levels than in darkness (Aro et al. Reference Aro, McCaffery and Anderson1994). Demmig-Adams et al. (Reference Demmig-Adams, Máguas, Adams, Meyer, Kilian and Lange1990b ) showed that recovery from photoinhibition was faster in low light intensities (2–135 µmol photons m−2 s−1) than in darkness. However, the recovery decreased with increasing light intensity from 2 up to 135 µmol photons m−2 s−1. Low light levels are needed to recover F v/F m and oxygen production from photoinhibition in the red alga Phyllophora truncata (Hanelt et al. Reference Hanelt, Huppertz and Nultsch1992). In the cyanobacterium Spirulina platensis recovery was much faster in low light and the recovery was inhibited by chloramphenicol, indicating that recovery depends on resynthesis of proteins (Vonshak et al. Reference Vonshak, Torzillo and Tomaseli1994). However, the light requirement necessary for optimal relaxation from photoinhibition is not known for lichens. Most lichen studies take lichens directly from the field or from specific stress exposures and place them in complete darkness before measuring F v/F m. If their D1 protein is damaged, relaxation of F v/F m can be highly inefficient in darkness.
The purpose of measuring F v/F m may vary. One aim might be to measure photoinhibition for lichens in the field or to measure the short-term effect of some photoinhibitory treatment under controlled conditions. In such cases long-term repair of, for example, damaged D1 protein in PSII reaction centres is undesirable. However, sometimes we aim to use uniform lichen material with maximal F v/F m before various stress experiments. Knowledge of treatment effects on recovery from natural stress or from stress under controlled conditions is therefore important.
This study aims to quantify the effect of various low light levels on the relaxation of F v/F m after photoinhibitory treatment in four lichen species in order to clarify the treatment of lichens before chlorophyll a fluorescence and adjust the measuring protocol accordingly.
Materials and Methods
Mature and healthy thalli of Lobaria pulmonaria were collected from twigs of Picea abies in Selnes, Namsos, Nord-Trøndelag, W Norway (64°25'N, 11°25'E) in March 2008 and thalli of Lobaria scrobiculata were sampled on trunks of Salix caprea in open P. abies forests in Horka, Overhalla, Nord-Trøndelag, W Norway (64°26'N, 11°47'E) in May 2008. The material was air-dried and stored at −18°C until the experiment in September 2009. Well-developed thalli of Parmelia sulcata and Xanthoria parietina were collected in Ås, Norway (59°40'N, 10°45'E) in September 2009 from stems of S. caprea and Populus tremula, respectively. The material was air-dried and stored in the dark at room temperature until the start of the experiments a few days later.
All experiments were carried out at a constant temperature (18°C). After 24 h acclimation at 5 µmol photosynthetically active photons m−2 s−1 from standard warm white fluorescent tubes, the thalli were photoinhibited for 4 h beneath a high intensity LED light panel (custom made SL3500, Photon Systems Instruments, Brno, Czech Republic). Thalli of X. parietina and P. sulcata were exposed to 1000 µmol photons m−2 s−1, whereas L. pulmonaria and L. scrobiculata were exposed to 600 µmol photons m−2 s−1. The red (λmax=637 nm), green (λmax=537 nm) and blue (λmax = 463 nm) LEDs were set at equal irradiances during the photoinhibitory treatment.
Chlorophyll fluorescence
Maximal dark adapted PSII efficiency (F v/F m) was measured with a PAM 2000 fluorimeter (Heinz Walz, Effeltrich, Germany) after 15 min dark adaptation. F v/F m values were measured immediately before the photoinhibitory treatment and during recovery at 0, 1·5, 6, 18·5 and 20 h after the end of the photoinhibitory treatment. During recovery, half of the thalli were kept in total darkness and the remainder were kept under low light intensities of 0·2, 1, 5 or 30 µmol photons m−2 s−1 from standard warm white fluorescent tubes. The thalli maintained in total darkness during recovery were moved to 0·2, 1, 5 or 30 µmol photons m−2 s−1 for 1·25 h before a final measurement of F v/F m.
Statistical analysis
F v/F m data were analyzed with a one-way ANOVA followed by Tukey’s test for pairwise comparisons using Minitab version 16.2.2.
Results
All lichens studied recovered completely from photoinhibition after 18·5 h recovery at 1, 5 or 30 µmol photosynthetically active photons m−2 s−1 (Fig. 1B, D, F & H). Even at 0·2 µmol photons m−2 s−1, Lobaria pulmonaria and Parmelia sulcata recovered completely, whereas L. scrobiculata and Xanthoria parietina did not (Fig. 1B, D, F & H). After 1·5 h under low light levels, recovery was incomplete in all four species (Fig. 1A, C, E & G). However, recovery in L. scrobiculata gradually improved with increasing light intensity and returned after 1·5 h to the initial value at the highest light intensity during recovery (30 µmol photons m−2 s−1; Fig. 1A). All light intensities between 0·2 and 30 µmol photons m−2 s−1 caused a similar level of recovery within both L. pulmonaria (Fig. 1E & F) and P. sulcata (Fig. 1C & D), whereas X. parietina required more than 0·2 µmol photons m−2 s−1 to recover (Fig. 1G & H). The cyanolichen L. scrobiculata did not recover in darkness while all green-algal lichens partially recovered in darkness and most of this recovery occurred within the first 1·5 h period (Figs 1 & 2). With respect to the kinetics in recovery after the high light exposure, the recovery was much weaker in all studied species kept in darkness (Fig. 2). However, at low light intensities (0·2–30 µmol photons m−2 s−1) for just 1·25 h after the 18·75 h recovery in darkness, all species rapidly resumed normal F v/F m values, at least at the higher light levels (Fig. 2).
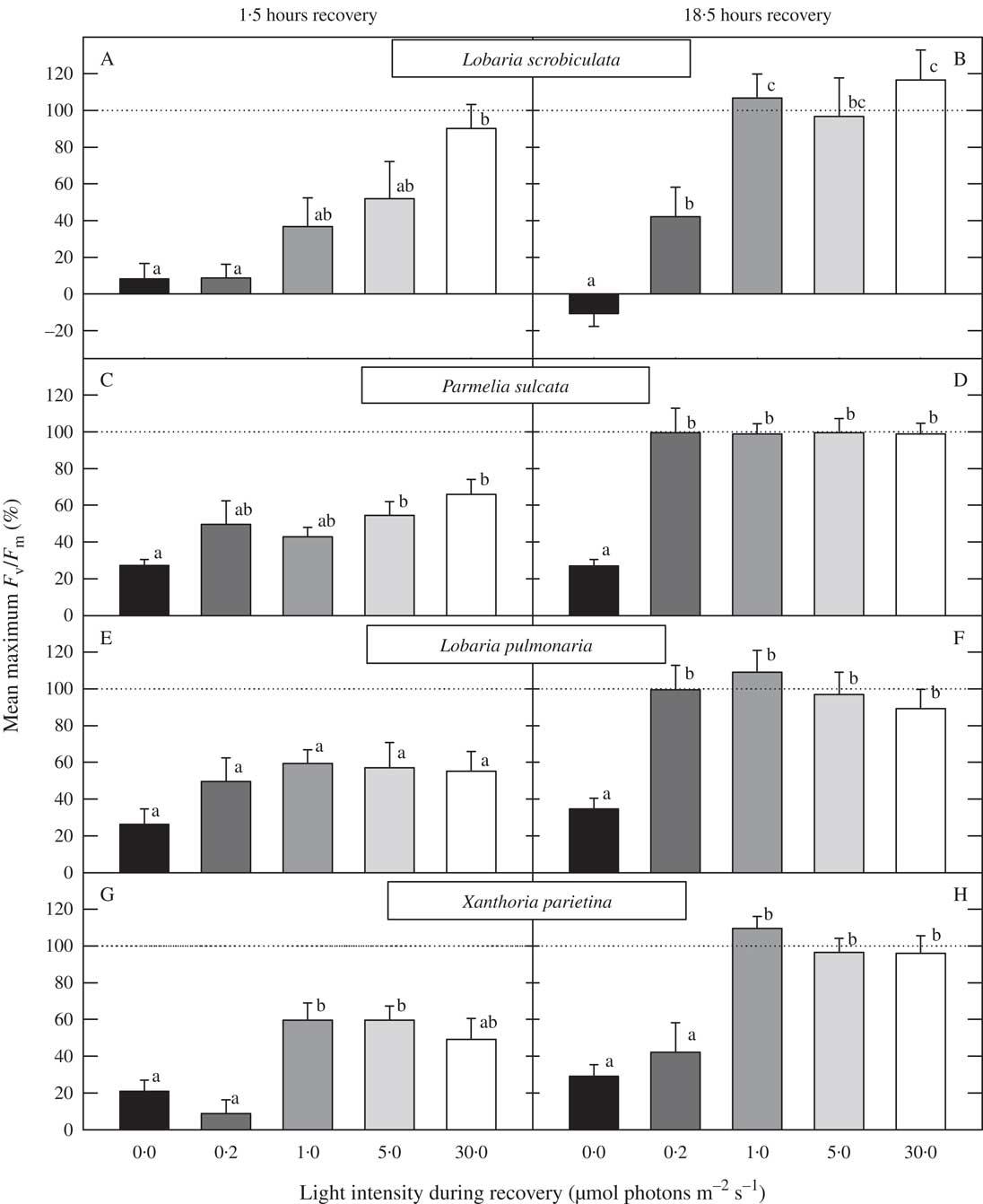
Fig. 1 Mean percentage recovery of F v/F m from photoinhibition after 1·5 h (A, C, E & G) and 18·5 h (B, D, F & H) in Lobaria scrobiculata (A & B), Parmelia sulcata (C & D), L. pulmonaria (E & F) and Xanthoria parietina (G & H) exposed to light intensities of 0, 0·2, 1·0, 5·0 and 30 µmol photons m−2 s−1. Values with the same letter above the columns are not significantly different (Tukey’s test for pairwise comparisons). Mean values (n=10) are plotted +1SEM.

Fig. 2 Kinetics of maximal PSII efficiency (F v/F m) recovery after 4 h strong photoinhibitory light (600 µmol m−2 s−1 for Lobaria scrobiculata and L. pulmonaria, and 1000 µmol m−2 s−1 for Parmelia sulcata and Xanthoria parietina). F v/F m values were measured before high light treatment (−4 h), immediately after (0 h), and then after 1·5, 6 and 18·5 h during recovery in darkness (closed symbols) or under low light intensities of 0·2, 1·0, 5·0 and 30 µmol photons m−2 s−1 (open symbols). Before the last measurement (20 h) all thalli were kept under low light intensities for 1·25 h. Mean values (n=10) are plotted ±1SEM.
Discussion
Complete relaxation from photoinhibition in lichens clearly depends on low light, as shown previously for red algae (Hanelt et al. Reference Hanelt, Huppertz and Nultsch1992), cyanobacteria (Vonshak et al. Reference Vonshak, Torzillo and Tomaseli1994; Singh et al. Reference Singh, Satoh, Yamamoto, Kanervo and Aro2008) and higher plants (Greer et al. Reference Greer, Berry and Bjorkman1986; Aro et al. Reference Aro, McCaffery and Anderson1994). Efficient relaxation at as low a light level as just 0·2 µmol photons m−2 s−1 (Fig. 1) suggests that light during the recovery acts as a signal for relaxation and not as an energy source during the relaxation period. Singh et al. (Reference Singh, Satoh, Yamamoto, Kanervo and Aro2008) argue that recovery is linked to plastid gene regulation. The green-algal lichens recovered partially from photoinhibition in darkness whereas the cyanolichen L. scrobiculata did not recover at all. The fast partial relaxation of F v/F m in green-algal lichens probably depends on relaxation of dynamic photoinhibition by conversion of zeaxanthin to violaxanthin in darkness (e.g. Maxwell & Johnson Reference Maxwell and Johnson2000), whereas rapid relaxation does not occur in the cyanolichen L. scrobiculata because cyanobacteria lack the xanthophyll cycle (Demmig-Adams et al. Reference Demmig-Adams, Adams, Czygan, Schreiber and Lange1990a ).
In relaxed leaves of higher plants the F v/F m is remarkably stable at 0·80–0·83 (e.g. Baker Reference Baker2008) but F v/F m values of ≥0·80 rarely occur in lichens sampled from their natural habitats. This suggests that slight photoinhibition is very common in lichens. However, severely photoinhibited L. pulmonaria thalli which were stored for 48 h at 5 µmol photons m−2 s−1 in a hydrated state did not fully recover from photoinhibition (Gauslaa & Solhaug Reference Gauslaa and Solhaug1996). It seems that variable levels of lasting photoinhibition are common for lichens under natural conditions (e.g. Gauslaa et al. Reference Gauslaa, Coxson and Solhaug2012; Färber et al. Reference Färber, Solhaug, Esseen, Bilger and Gauslaa2014) and that it varies with season (Vráblíková et al. Reference Vráblíková, McEvoy, Solhaug, Barták and Gauslaa2006). In order to start experiments with more uniform and relaxed lichen material, it is important to acclimate lichens in low light, and not in darkness, for as long as 1–2 days to recover from natural photoinhibition before experiments. In cyanolichens, maximal F v/F m values are rarely higher than 0·6. These low values in cyanolichens may be caused by fluorescence from phycobilisomes contributing to increased F o (Campbell et al. Reference Campbell, Hurry, Clarke, Gustafsson and Öquist1998). In addition, several compounds are shared between the photosynthetic and respiratory electron transport chains in cyanobacteria resulting in PSII not being fully oxidized in darkness (e.g. Binder Reference Binder1982). Higher PSII yields than when dark-adapted can be achieved by exposure to low blue light that excites mainly PSI, resulting in more oxidized PSII (Solhaug et al. Reference Solhaug, Xie and Gauslaa2014).
Both the reduction in the effective quantum yield of PSII and F v/F m under photoinhibitory light and the recovery of these two parameters are similar in L. pulmonaria (Barták et al. Reference Barták, Solhaug, Vráblíková and Gauslaa2006). Rapid recovery from photoinhibition under low light, as shown in Fig. 2, might be ecologically important. On a clear day, a lichen may become photoinhibited and it will desiccate during the day. Photoinhibition will stay constant or increase during the period in the dry state, depending on light exposure. The lichen thallus will regain moisture due to condensation during the night with a photosynthetic active period during the first part of the day (e.g. Green et al. Reference Green, Nash and Lange2008). The low light period at dawn might therefore be important for fast recovery of F v/F m and effective quantum yield of PSII before the period with photosynthesis in the early part of the day.
It seems that most green-algal lichens can gain F v/F m values close to 0·8 (Gauslaa & Solhaug Reference Gauslaa and Solhaug1996). However, F v/F m is often considerably lower. F v/F m values in the range of 0·45–0·65 have often been considered as normal under non-stressed conditions (Bačkor et al. Reference Bačkor, Swanson and Fahselt2006 and references therein). Such low F v/F m values are probably a result of long-term photoinhibition that slowly recovers. Lichens may have a more long-term downregulation of PSII efficiency similar to the sustained downregulation of PSII efficiency common in conifers during winter (Adams III et al. Reference Adams, Demmig-Adams, Rosenstiel and Ebbert2001). The ecological function of this sustained downregulation of PSII may be protection against high light during rapid changes in light levels.
In conclusion, this study shows that it is necessary to allow lichens to recover under low light intensities to optimize relaxation from photoinhibition. In darkness, relaxation is incomplete. If the purpose of an experiment is to measure natural photoinhibition, only dark adaptation should be used before F v/F m measurement, whereas if standardized lichen thalli without photoinhibition are needed, a long pretreatment under low light intensities is recommended.
Professor Yngvar Gauslaa is thanked for his comments on the manuscript.