1. Introduction
During the Mesozoic, Adelaide Island was situated on the western edge of the continental margin arc of Gondwana in a probable fore- or intra-arc position (Griffiths & Oglethorpe, Reference Griffiths and Oglethorpe1998). The area forms part of the geophysically and geologically defined Western Zone of the Central Domain of the Antarctic Peninsula (Fig. 1), a Mesozoic magmatic arc terrane that formed in response to subduction along the proto-Pacific margin of Gondwana (Vaughan & Storey, Reference Vaughan and Storey2000; Ferraccioli et al. Reference Ferraccioli, Jones, Vaughan and Leat2006).

Figure 1. Terrane map for the Antarctic Peninsula after Vaughan & Storey (Reference Vaughan and Storey2000) and Ferraccioli et al. (Reference Ferraccioli, Jones, Vaughan and Leat2006).
The fore-arc sequences (Fossil Bluff Group) of Alexander Island to the south of Adelaide Island have been well documented (e.g. Butterworth et al. Reference Butterworth, Crame, Howlett and Macdonald1988) and were considered by Vaughan & Storey (Reference Vaughan and Storey2000) to form a distinct tectonic unit (the Western Domain; Fig. 1) to that of the Central Domain. The correlation of volcano-sedimentary sequences across these two parts of the Mesozoic fore-arc will be used to verify the accuracy of terrane boundaries and the timing of accretion of the Antarctic Peninsula.
U–Pb (zircon) age data reported here, in conjunction with re-interpreted 40Ar–39Ar data and a complete revision of the lithostratigraphy has enabled a robust chronostratigraphy of Adelaide Island to be constructed. Adelaide Island sits in a geologically important position across the fore- to intra-arc sector of the Antarctic Peninsula, and correlations between its successions and those of Alexander Island and the Antarctic Peninsula should provide a test of terrane boundary models, timing of accretion and probability of the Western Domain being exotic (Vaughan & Storey, Reference Vaughan and Storey2000).
2. Lithostratigraphy of Adelaide Island
Adelaide Island is situated off the west coast of the Antarctic Peninsula, to the north of Alexander Island (Fig. 1). Adelaide Island can be divided into two distinct topographic areas: the Fuchs Ice Piedmont to the west and a mountainous area on the eastern part of the Island, including the massifs of Mount Liotard, Gaudry and Bouvier (Fig. 2).

Figure 2. Revised geological map of Adelaide Island.

Figure 2. Legend for geological map of Adelaide Island.
Adelaide Island preserves a 2–3 km thick succession of sedimentary, volcaniclastic and volcanic rocks, which are interpreted to be late Mesozoic in age and represent sedimentation and volcanism in a fore- to intra-arc position. Later plutonism is exposed across the Island and probably forms part of the Antarctic Peninsula batholith (Leat, Scarrow & Millar, Reference Leat, Scarrow and Millar1995).
The geology of Adelaide Island was first described in detail by G. J. Dewar (unpub. Ph.D. thesis, Univ. Birmingham, 1965; Reference Dewar1970) and later by Griffiths & Oglethorpe (Reference Griffiths and Oglethorpe1998) who were not able to place too much chronological control on the stratigraphy they proposed, but they did assign a probable Late Jurassic age to the stratified rocks of Adelaide Island based on similarities to rocks from elsewhere on the west coast of the Antarctic Peninsula.
A revised stratigraphy of Adelaide Island is proposed here, based on recent geological mapping and the previous field studies of C. J. Griffiths (e.g. Griffiths & Oglethorpe, Reference Griffiths and Oglethorpe1998) and G. J. Dewar (e.g. Dewar, Reference Dewar1970) and spectral mapping (Haselwimmer, Riley & Liu, Reference Haselwimmer, Riley and Liu2010). The new formations proposed here are supported by newly acquired geochronology.
2.a. Buchia Buttress Formation
The Buchia Buttress Formation crops out at its type locality of Buchia Buttress and extends south to the Shambles Glacier region, along the Sheldon Glacier region, with minor outcrop on the western side of Leonie Island (Fig. 2). The extent of the Buchia Buttress Formation to the north of Buchia Buttress is less certain given the paucity of outcrop, but it has been tentatively extrapolated to the north and west of Bond Nunatak (Fig. 2) based on aeromagnetic data (British Antarctic Survey, unpub. data).
At Buchia Buttress, the succession is characterized by volcanic breccias, silicic crystal tuffs, immature volcaniclastic rocks and coarse-grained volcaniclastic sandstone units with interbedded cobble/boulder conglomerates. An alternating fining-upwards sequence is observed at Buchia Buttress consisting of sandstones, interbedded with pebble/boulder conglomerates, cross-bedded medium–fine-grained sandstones and ripple cross-laminated sandstones. Within this sequence, cherty silicic crystal tuffs occur, which are interpreted to have been emplaced into water. The tuffs are green-grey in colour, medium–fine grained and include rare lithic fragments and oblate, porphyritic pumice. The sandstone beds are locally fossiliferous, particularly at Buchia Buttress where marine invertebrates are common, including bivalves (Buchia sp., Inoceramus sp.), perisphinctid ammonites and belemnites (Hibolithes sp.). Thomson (Reference Thomson1972) favoured a Kimmeridgian–Tithonian age (likely Early Tithonian) based on the comparison of the fauna to elsewhere on the Antarctic Peninsula.
The succession at Buchia Buttress and southern Mount Bouvier is estimated at 830 m in thickness. Elsewhere, the succession is not as complete and the exposures south of the Shambles Glacier are characterized by sandstone and conglomerate units, with far less abundant volcanic material.
The lower part of the succession, to the south of Mount Bouvier, is intruded by tonalite of the Mount Bouvier intrusive suite.
2.b. Milestone Bluff Formation
The thick (at least 1.5 km) sequence of sedimentary, volcaniclastic and volcanic lithologies dominate the geology of the central escarpment of Adelaide Island. The sequences exposed from Fletcher Bluff, Milestone Bluff and south towards Window Buttress can all be correlated with a high degree of confidence, although the exposures at the southern end of the range at Cape Alexandra are deformed and have a greater volcanic component and are only tentatively assigned to the Milestone Bluff Formation.
The sequences at Fletcher Bluff, Milestone Bluff and Window Buttress are dominated by sandy turbiditic sediments with subordinate ash flow-, crystal- and vitric tuffs. Rare ignimbrite beds are also present with strongly flattened (6:1) pumices (Fig. 3a). The ignimbrites are typically feldspar porphyritic and together with crystal tuffs can form beds up to 30 m in thickness. Cobble and boulder conglomerates are prominent horizons throughout the succession (Fig. 3b), with individual beds up to greater than 20 m in thickness. The conglomerates are typically poorly sorted, dominantly clast-supported, with individual clasts up to 0.8 m in diameter and are the result of deposition from high density turbidity currents or debris flows in an alluvial fan environment.
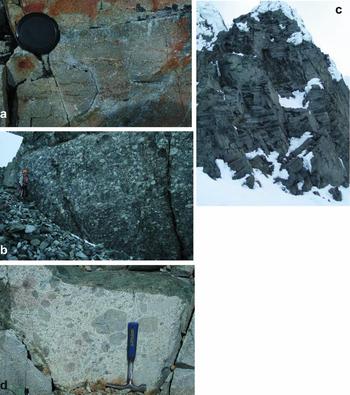
Figure 3. (a) Rhyolitic ignimbrite from the Milestone Bluff Formation, Milestone Bluff. Lens cap is 58 mm diameter; (b) cobble/boulder conglomerate from Fletcher Bluff, Milestone Bluff Formation; (c) columnar jointing in basaltic andesite lavas, Sighing Peak, Bond Nunatak Formation, ~ 40 m height; (d) dioritic ‘blebs’ in granodiorite host, Rothera Point, Adelaide Island Intrusive Suite.
The sequences dip shallowly (~ 10–15°) to the east (85–105°) and are offset by numerous, minor, reverse faults. The conglomerate clast orientations indicate a general source direction from the east to the southeast (Griffiths & Oglethorpe, Reference Griffiths and Oglethorpe1998; this study), which is consistent with the possible source of the plutonic clasts (NW Palmer Land; Fig. 1), which have been dated at ~ 140 Ma (Griffiths & Oglethorpe, Reference Griffiths and Oglethorpe1998).
Belemnite, bivalve and plant fossil fragments have all been identified (Thomson & Griffiths, Reference Thomson, Griffiths, Moyes, Willan and Thomson1994; Griffiths & Oglethorpe, Reference Griffiths and Oglethorpe1998; this study) from the loose debris of Milestone Bluff and Window Buttress and indicate that at least part of the succession was deposited in a shallow marine environment. The fossil fragments have tentatively been assigned a Late Jurassic age based on similarities to elsewhere on Adelaide Island and the Antarctic Peninsula (Thomson & Griffiths, Reference Thomson, Griffiths, Moyes, Willan and Thomson1994).
2.c. Mount Liotard Formation
The Mount Liotard Formation is restricted to parts of southern Adelaide Island, principally the area around Mount Liotard and Mount Ditte, west to Avian Island and east to Jenny Island (Fig. 2).
Mount Liotard (2225 m) and the surrounding area are composed of at least 1800 m of basaltic andesite and andesite multiple lava flows. Individual, complete lava units are difficult to distinguish, but where possible, individual lavas of 30–40 m thickness have been identified within the succession. The units are typically feldspar porphyritic and are cut by rare basaltic sills. Erosional surfaces were not observed, but many flows are seen to have rubbly flow tops and intercalated autoclastic lava breccias and rare hyaloclastite beds.
A section close to the summit of Mount Liotard preserves well-exposed sequences ~ 80 m thick of andesite lavas and monomict breccias.
2.d. Bond Nunatak Formation
The Bond Nunatak Formation is exposed across parts of northern and eastern Adelaide Island and possibly extending to Liard Island and Wyatt Island (Fig. 2). At Bond Nunatak, the succession is composed of thickly bedded sandstones and cobble/boulder conglomerates overlying andesite lavas and coarse breccias. The sequence is shallowly dipping (~ 15°) to the southeast.
The volcanic part of the succession at Bond Nunatak is at least 400 m thick, with multiple porphyritic andesitic lava flows interbedded with breccias and immature volcaniclastic rocks. A continuation of this succession is observed at Mount Vélain in the northern part of Adelaide Island. At Mount Vélain, steeply dipping (35° to the northeast) basaltic andesite breccias and aphanitic andesitic lavas are exposed. They have a total thickness of at least 300 m and the lavas/breccias are interbedded with minor (typically < 2 m thickness) units of coarse-grained immature volcaniclastic sediments.
At Bond Nunatak, the succession is intruded near its base by tonalite and gabbro, but no plutonic rocks are observed at Mount Vélain.
The basic–intermediate rocks identified on Lagoon Island (Fig. 2) are also associated with the succession observed at Bond Nunatak and Mount Vélain. Lagoon Island rocks are typically massive, fine-grained–aphanitic andesite lavas, which are typically feldspar porphyritic. Breccias and autoclastic breccias are associated with the lavas, along with thinner volcaniclastic units.
Further outcrops of the Bond Nunatak Formation are observed at Sighing Peak and outcrops to the east of McCallum Pass (Fig. 2). At Sighing Peak, a total thickness of at least 600 m is observed. The basal part of the succession, which crops out at sea level, is a coarse volcanic breccia; the clasts are angular and include glassy shards and spatter material. The basal breccia is ~ 200 m in thickness and is overlain by a more massive andesite unit, characterized by feldspar porphyritic lavas with multiple flows identified. In part, the andesite flows preserve columnar jointing (Fig. 3c).
The geographical extent of the Bond Nunatak Formation mapped with a degree of uncertainty is shown in Figure 2.
2.e. Reptile Ridge Formation
The geology of Reptile Ridge is distinct to that seen elsewhere on central and western Adelaide Island. The central and western parts of Reptile Ridge (Fig. 2) are characterized by poorly bedded rhyolite–rhyodacite crystal tuffs, lithic tuffs, ignimbrites and rare lava flows. In many places the silicic tuffs have been subject to intense hydrothermal alteration, which has led to extensive haematite mineralization.
At least 250 m thickness of crystal tuffs and lithic tuffs is exposed on Reptile Ridge. The volcanic rocks are interpreted as subaerial and include columnar jointed ignimbrites and crystal tuffs. The ignimbrite and crystal tuff units are typically phenocryst poor, but with a mineral assemblage characterized by embayed quartz, K-feldspar, plagioclase, biotite, magnetite, apatite, titanite, rutile and zircon.
Similar facies are exposed on Webb Island and Killingbeck Island (Fig. 2). On Webb Island, a succession of almost 300 m is exposed of flow-banded rhyolite lavas, crystal tuffs, ignimbrites and near vent breccias. At Killingbeck Island, ~ 70 m thickness of lithic-rich crystal tuffs and agglomerate crop out, which are characterized by extensive Fe-mineralization. The tuffs are lithic-rich (> 20%) and are very friable. At the base of the exposed succession are bedded volcaniclastic sediments, which are also observed on Reptile Ridge.
2.f. Adelaide Island Intrusive Suite
A large part of the exposed geology on Adelaide Island consists of plutonic rocks of the Adelaide Island Intrusive Suite. The geology of the Wright Peninsula, Mount Bouvier and the area around Mount Gaudry is dominated by granodiorites, tonalities and gabbroic rocks. Many of the plutons are heterogeneous and are characterized by concentrations of well-rounded xenoliths, which are typically more mafic than the host (Fig. 3d). Dioritic/andesitic, feldspar-phyric xenoliths are common, and can account for 30–40% of the rock. Where hybrid plutons are observed, tonalite and/or quartz monzonite intrude the gabbro/dioritic phase. At several localities the plutonic rocks are observed to intrude the sedimentary and volcanic successions, and it has been assumed that the intrusive suite post-dates the volcaniclastic/volcanic successions (Dewar, Reference Dewar1970).
Granodiorite and hybrid gabbro/granodiorite plutons are the most abundant on Adelaide Island. The granodiorite is leucocratic and is dominated by plagioclase (~ 50–60%), which often weathers orange/brown, quartz typically accounts for ~ 10% of the rock and K-feldspar ~ 5%. Mafic minerals are common (25%), with green/brown amphibole abundant, along with minor amounts of biotite and epidote.
The entire volcaniclastic/volcanic stratigraphy and the plutonic rocks of Adelaide Island are cut by dolerite and intermediate–felsic composition dykes. The dykes are typically < 1 m thick, dip steeply (> 75° to the southeast) and strike in the range 210–230° (Fig. 4), with rare dykes host to ultramafic xenoliths (Dewar, Reference Dewar1970).

Figure 4. Radial plot of dyke strike direction from Adelaide Island.
3. Geochronology and lithostratigraphy
3.a. Geochronology: previous work
Dewar (unpub. Ph.D. thesis, Univ. Birmingham, 1965) made the first attempts to construct a lithostratigraphy for Adelaide Island. He defined two major lithological units on the Island: a succession of stratified volcanic and sedimentary rocks and an intrusive suite of granitoids and gabbros. He subdivided the volcano-sedimentary succession into three separate mappable units. Dewar (unpub. Ph.D. thesis, Univ. Birmingham, 1965) assigned a probable Late Jurassic age to his Mount Bouvier summit succession based on the molluscan fauna from Buchia Buttress, which showed similarities to other southern hemisphere Mesozoic faunas. This age was later verified by Thomson (Reference Thomson1972). The successions further south at Milestone Bluff, Fletcher Bluff and Window Buttress also contain a molluscan fauna that was given a probable Late Jurassic age (Thomson & Griffiths, Reference Thomson, Griffiths, Moyes, Willan and Thomson1994). However, further south at Cape Alexandra, leaf fossils from hornfelsed mudstones and siltstones were considered to be Cretaceous or even Tertiary in age (Jefferson Reference Jefferson1980; Griffiths & Oglethorpe, Reference Griffiths and Oglethorpe1998).
A limited attempt to date some of the sequences and plutonism on Adelaide Island using Rb–Sr dating was made by several workers. Thomson & Pankhurst (Reference Thomson, Pankhurst, Oliver, James and Jago1983) dated a rhyolite from Webb Island, which yielded a Rb–Sr whole-rock age of 67 ± 17 Ma. Several plutons have also been dated, yielding Early Tertiary ages: granodiorite–granite plutons from the Wright Peninsula and Anchorage Island gave ages of 60 ± 3 Ma and 62 ± 2 Ma, respectively (Pankhurst Reference Pankhurst1982). A gabbro and aplite from the Faure Islands (25 km south of Adelaide Island) gave an age of 48 ± 4 Ma (Moyes & Pankhurst, Reference Moyes, Pankhurst, Moyes, Willan and Thomson1994).
The only other attempt to construct a coherent stratigraphy for Adelaide Island was by Griffiths & Oglethorpe (Reference Griffiths and Oglethorpe1998) who used updated field mapping in combination with new geochronology data. They obtained 40Ar–39Ar spectra, fission-track dating (zircon, apatite) and K–Ar (hornblende) from volcanic rocks, plutonic clasts from conglomerate, as well as dykes from multiple sites across Adelaide Island. The overall picture was somewhat confused with many samples not fulfilling statistically valid criteria, exhibiting Ar loss, excess Ar and possible resetting by later stage plutonism, and other ages not consistent with the molluscan fauna. Their new stratigraphy included six mappable units.
3.b. Geochronology: this study
The U–Pb (zircon) dating was carried out using a Cameca 1270 (samples J6.347.1, J6.335.1) and a Cameca 1280 (samples J8.403.1, J8.20.1s) ion-microprobe at the NORDSIM facility, Swedish Museum of Natural History (Stockholm), following the method of Whitehouse & Kamber (Reference Whitehouse and Kamber2005). As part of this study, four samples have been dated from separate units across Adelaide Island and all yielded robust, stratigraphically consistent ages (Table 1). The combination of new geological mapping, a revised stratigraphy and the new U–Pb (zircon) ages allow some of the 40Ar–39Ar ages of Griffiths & Oglethorpe (Reference Griffiths and Oglethorpe1998) to be placed in a better context. A coherent litho- and chronostratigraphy also permits improved correlations with the well-dated fossiliferous fore-arc sequences documented from Alexander Island (e.g. Butterworth et al. Reference Butterworth, Crame, Howlett and Macdonald1988).
Table 1. U–Pb ion-microprobe zircon data

1 Analysis identification. Asterisks show spots included in age calculations; i indicates inherited grain.
2 Percentage of common 206Pb estimated from the measured 204Pb. Data is not corrected for common Pb.
Calculations were made using Isoplot 3.1 (Ludwig, Reference Ludwig2003) and used the decay constants of Steiger & Jäger (Reference Steiger and Jäger1977).
Age errors (in text, figure and table) are quoted as 2σ except where stated.
3.c. Buchia Buttress Formation
A feldspar porphyritic silicic crystal tuff (J6.347.1) from the succession at Buchia Buttress was selected for U–Pb analysis. The sample is ~ 550 m above the exposed base of the Buchia Buttress Formation and is stratigraphically just above the coarse sandstones where molluscan fossils are most abundant.
Clear, well-faceted, prismatic zircons from the rhyodacitic tuff (J6.347.1) display oscillatory growth zoning under cathodoluminescence (Fig. 5a). Thirteen analyses of zircon yielded 206Pb–238U ages with a weighted average of 150.0 ± 2.5 Ma. Excluding two analyses (spot 5: inherited grain, spot 8: high concentration of common Pb), the remaining analyses yield a concordia age of 149.5 ± 1.6 Ma (Fig. 6a), and this age is interpreted to date the eruption of the rhyodacitic crystal tuff. An age of ~ 150 Ma is in excellent agreement with the molluscan fauna from Buchia Buttress (Thomson, Reference Thomson1972), which was assigned a probable Kimmeridgian–Tithonian age and probably closer to Early Tithonian (~ 150 Ma).

Figure 5. Cathodoluminescence images of analysed zircons. (a) Silicic crystal tuff (J6.347.1) from the Buchia Buttress Formation; (b) rhyolitic ignimbrite (J8.403.1) from the Milestone Bluff Formation; (c) rhyolitic ignimbrite (J8.20.1s) from the Reptile Ridge Formation; (d) tonalite (J6.335.1) from Mount Bouvier. Scale bars are 100 μm in length.
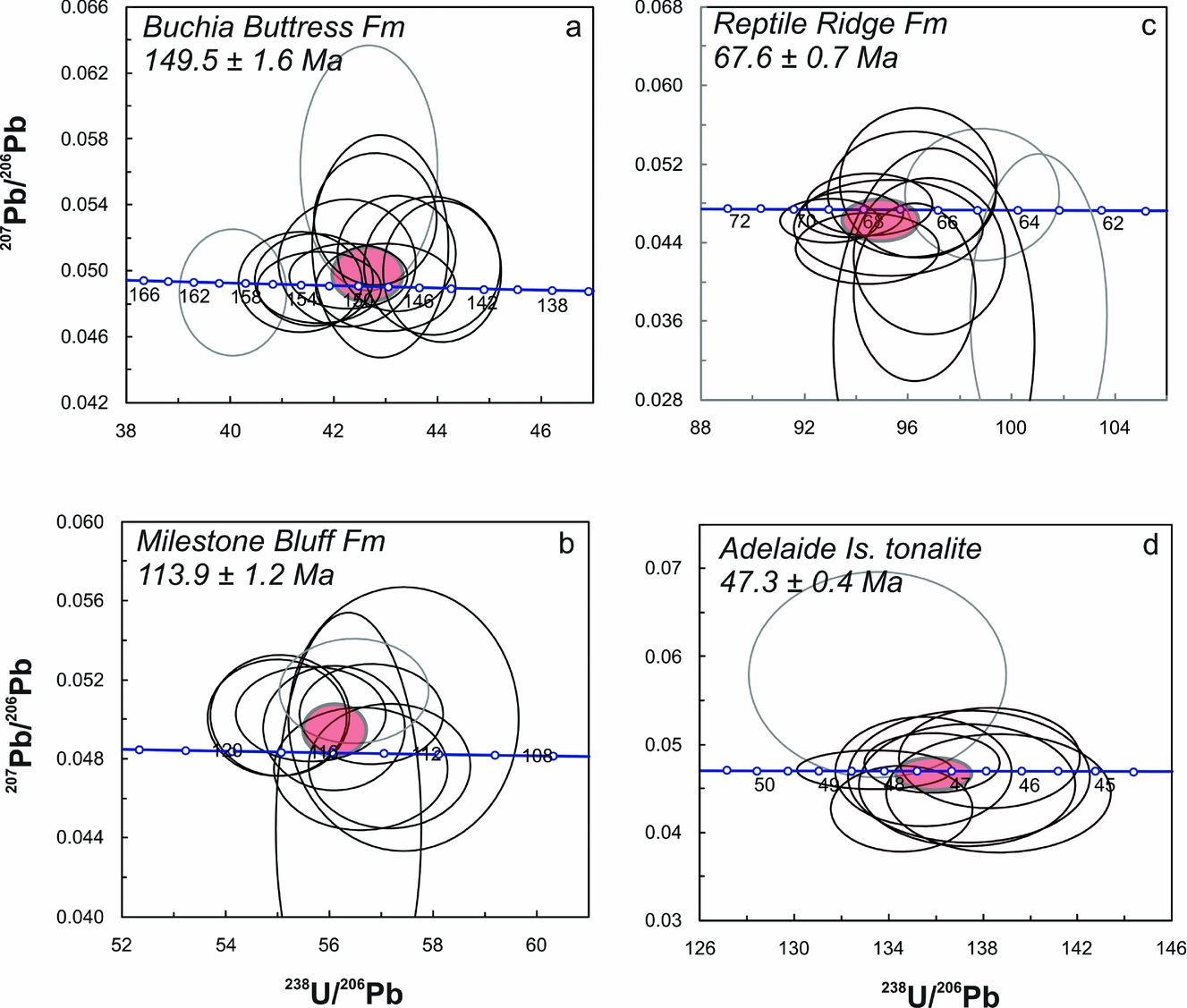
Figure 6. U–Pb concordia diagrams. (a) Silicic crystal tuff (J6.347.1) from the Buchia Buttress Formation; (b) rhyolitic ignimbrite (J8.403.1) from the Milestone Bluff Formation; (c) rhyolitic ignimbrite (J8.20.1s) from the Reptile Ridge Formation; (d) tonalite (J6.335.1) from Mount Bouvier.
Griffiths & Oglethorpe (Reference Griffiths and Oglethorpe1998) dated a basaltic lava stratigraphically above (~ 250 m) the crystal tuff dated here. The age spectrum showed evidence of Ar loss or alteration, but based on their highest temperature step, they used an age of 76 Ma as the minimum age of eruption (although this unit may form part of the later Bond Nunatak Formation: see later explanation).
The Buchia Buttress Formation is considered to be the lowermost succession exposed on Adelaide Island, and although the tuff sample comes from ~ 550 m above the lowest exposed units, an age of ~ 150 Ma is considered valid for the formation. The nature of the deposits in the lower part of sequence suggests rapid deposition in a transitional shallow marine apron fan environment.
3.d. Milestone Bluff Formation
Approximately 80 m above the exposed base of the Milestone Bluff Formation, a rare silicic crystal-lithic tuff and minor ignimbrite unit overlie a sequence of cobble/boulder conglomerates and coarse sandstones. The entire succession of crystal-lithic tuffs and ignimbrites are ~ 30 m in thickness and dip shallowly (< 8°) to the northeast. The volcanic rocks are overlain by further cobble/boulder conglomerates. The sample selected for analysis (J8.403.1) was from the ignimbrite unit, which is characterized by typically flattened, porphyritic pumice, with aspect ratios of ~ 5:1 (Fig. 3a).
The ignimbrite is feldspar porphyritic and generally finer grained than the crystal-lithic tuffs with which it is interbedded. The volcanic rocks are mostly subaerial, but some of the more cherty, laminated crystal tuffs may have been emplaced into water.
Zircons from the rhyodacitic ignimbrite are clear, prismatic and well faceted. Under cathodoluminescence, zircons have patchy and faint oscillatory growth zoning (Fig. 5b), but do not show any evidence for inheritance. Ten analyses on nine grains yield a weighted average of 113.8 ± 1.1 Ma for the 206Pb–238U ages and a concordia age of 113.9 ± 1.2 Ma (Fig. 6b), which is taken as the preferred age, close to the Aptian–Albian boundary (Fig. 7).

Figure 7. Comparative stratigraphy from Adelaide Island and the Fossil Bluff Group of Alexander Island (from Storey et al. Reference Storey, Brown, Carter, Doubleday, Hurford, Macdonald and Nell1996).
Griffiths & Oglethorpe (Reference Griffiths and Oglethorpe1998) also attempted to date a tuff from Milestone Bluff, but the sample suffered Ar loss and gave a poorly defined plateau age of 27.8 ± 0.6 Ma. The Milestone Bluff Formation sequence is also characterized by an abundant belemnite population and rare bivalves, which were considered to be Late Jurassic in age (Thomson & Griffiths, Reference Thomson, Griffiths, Moyes, Willan and Thomson1994), but a well-constrained age of ~ 114 Ma is stratigraphically consistent and indicates a separate phase of sedimentation and volcanism to that exposed in central and eastern parts (Buchia Buttress Formation) and suggests the Late Jurassic age is unreliable.
3.e. Reptile Ridge Formation
A moderately welded, rhyolitic ignimbrite (J8.20.1s) from the northern section of Reptile Ridge was sampled at a stratigraphic height of ~ 120 m. The sample is pale grey, fine grained, feldspar porphyritic and is characterized by partially flattened pumice clasts, which are also feldspar porphyritic. The rock is unaltered, but is adjacent to some areas of intense hydrothermal alteration with characteristic mineralization.
The rhyolitic ignimbrite (J8.20.1s) yielded clear, well-faceted, prismatic zircons, with aspect ratios of up to 4:1. They also contain quartz inclusions. Under cathodoluminescence the zircons have typically patchy zoning, but faint oscillatory zoning is evident in most grains (Fig. 5c). Twelve analyses on ten grains have 206Pb–238U ages with a weighted average of 66.8 ± 1.0 Ma. Excluding two analyses, which are in interpreted to have suffered a small degree of Pb loss (spots 1, 3), a concordia age of 67.6 ± 0.7 Ma (Fig. 6c) is obtained, and this is the preferred age for Reptile Ridge Formation volcanism.
An age close to the Cretaceous–Tertiary (K–T) boundary for the Reptile Ridge Formation volcanic rocks of the Wright Peninsula and nearby islands (Fig. 2) is in agreement with the Rb–Sr (whole-rock) age (Thomson & Pankhurst, Reference Thomson, Pankhurst, Oliver, James and Jago1983) of 67 ± 17 Ma from Webb Island, albeit with a large degree of uncertainty.
Griffiths & Oglethorpe (Reference Griffiths and Oglethorpe1998) also dated two rhyolitic tuffs from the Reptile Ridge Formation. A sample from Reptile Ridge yielded a poor 40Ar–39Ar plateau age of 56.2 ± 0.5 Ma with a spectrum indicating significant Ar loss. A better defined plateau from Piñero Island gave an age of 57.7 ± 0.5 Ma. A granodiorite intruding the Reptile Ridge Formation gave a Rb–Sr (whole-rock) age of 60 ± 3 Ma (Pankhurst, Reference Pankhurst1982) and a zircon fission-track age of 60.0 ± 3.7 Ma for an adjacent granodiorite pluton (Griffiths & Oglethorpe, Reference Griffiths and Oglethorpe1998), both indicating an age of > 60 Ma for the Reptile Ridge Formation rhyolites.
In summary, an age of ~ 67 Ma for the Reptile Ridge Formation is considered a reliable date that is geologically consistent. It indicates an entirely separate phase of volcanism on eastern Adelaide Island to the volcanism that punctuates the turbiditic sequences to the west of the Wright Peninsula.
3.f. Bond Nunatak/Mount Liotard formations
The volcanic rocks of the Bond Nunatak Formation and the Mount Liotard Formation are all basaltic andesite–andesite in composition and are not suitable for U–Pb (zircon) dating. But given the improved stratigraphy and chronology from elsewhere on Adelaide Island, it is possible to use existing geochronology with renewed confidence.
Griffiths & Oglethorpe (Reference Griffiths and Oglethorpe1998) dated a basaltic andesite from southern Mount Liotard, which is part of the Mount Liotard Formation. The Ar spectrum was stepped and did not produce a plateau age, although a minimum age of ~ 63 Ma was interpreted from the maximum step. Another andesite from western Jenny Island (Fig. 2) also forms part of the Mount Liotard Formation and yielded a good plateau age of 49.7 ± 0.5 Ma.
Griffiths & Oglethorpe (Reference Griffiths and Oglethorpe1998) also attempted to date a granitoid clast from one of the conglomerate units at Bond Nunatak, which forms part of the Bond Nunatak Formation. An amphibole separate from the granitoid generated a poor plateau age of 45–50 Ma. There is an indication of significant Ar loss, which was attributed to potential resetting from the nearby Mount Bouvier tonalite intrusion. The highest temperature step gave an age of 59 Ma, which was considered the minimum (plutonic) age prior to incorporation in the conglomerate.
The successions that comprise the Bond Nunatak and the Mount Liotard formations are interpreted to be closely related. Basaltic andesite lava flow and breccias dominate both formations, with rare coarse sandstones and conglomerates at Bond Nunatak and Carvajal (Fig. 2).
Many of the 40Ar–39Ar ages determined by Griffiths & Oglethorpe (Reference Griffiths and Oglethorpe1998) are not considered reliable and have probably been reset by the emplacement of Tertiary-age plutonism. However, a basaltic andesite lava from high up in the Buchia Buttress Formation is more likely to represent part of the Bond Nunatak Formation, overlying the Buchia Buttress Formation. Griffiths & Oglethorpe (Reference Griffiths and Oglethorpe1998) dated this lava sequence and the age spectrum showed evidence of Ar loss or alteration, but based on their highest temperature step, they used an age of 76 Ma as the minimum age of eruption.
A probable age estimate for the Bond Nunatak and Mount Liotard formations is akin to that of the Reptile Ridge Formation, i.e. ~ 67 Ma, but trending towards an older age (~ 75 Ma). Unfortunately, no relationship is observed between them, but all three formations are intruded by Tertiary-age granitoid and gabbro plutonic rocks.
3.g. Intrusive rocks of Adelaide Island
Large parts of Adelaide Island, particularly the central and eastern parts of the Island, are dominated by granitoid and gabbroic plutons (Fig. 2). Previous attempts at dating the age of plutonism have been more successful than the attempts to date the age of volcanism and sedimentation.
Griffiths & Oglethorpe (Reference Griffiths and Oglethorpe1998) used fission-track dating (apatite and zircon) on three granodiorite samples from the Wright Peninsula area (Fig. 2), yielding ages of 44.2 ± 3.8 Ma, 52.0 ± 2.9 Ma and 52.4 ± 3.5 Ma. Two dolerite dykes from the Sheldon Glacier area (Fig. 2) were also dated (K–Ar: hornblende) by Griffiths & Oglethorpe (Reference Griffiths and Oglethorpe1998) and gave average ages of 58 ± 4 Ma and 48 ± 6 Ma.
As part of this current study, a tonalite (J6.335.1) from near Mount Bouvier (Fig. 2) was dated using U–Pb (zircon). The sample was characterized by prismatic zircons with oscillatory zoning, typical of magmatic rocks. The zircons tend to have a non-luminescent outer portion (Fig. 5d). Nine analyses yielded a weighted average 206Pb–238U age of 47.0 ± 0.7 Ma, indistinguishable from the preferred concordia age of 47.3 ± 0.4 Ma (Fig. 6d), which was calculated by omitting one analysis (spot 5) that contained common Pb.
An Eocene age for plutonism on Adelaide Island is consistent with age ranges from previous work (44–58 Ma) and suggests that plutonism post-dates the main phases of sedimentation and volcanism. At several localities, the acidic–intermediate plutonic rocks are seen in association with the gabbroic rocks of Adelaide Island and in all cases, the tonalite–granodiorite rocks are the final intrusive phase.
4. Correlations along the fore-arc margin
4.a. The Fossil Bluff Group of Alexander Island
The Mesozoic fore-arc of the Antarctic Peninsula is well exposed on Alexander Island (Fig. 1) where the stratigraphical relationship between the fore-arc basin and the accretionary complex can be observed (Doubleday, Macdonald & Nell, Reference Doubleday, Macdonald and Nell1993). The fore-arc basin sedimentary rocks on Alexander Island are assigned to the Fossil Bluff Group (Taylor, Thomson & Willey, Reference Taylor, Thomson and Willey1979).
The entire Fossil Bluff Group has a thickness of ~ 7 km of predominantly clastic sedimentary rocks. The lowermost part of the succession (Middle Jurassic; Macdonald et al. Reference Macdonald, Leat, Doubleday and Kelly1999) is interpreted as trench-slope apron deposits (Doubleday, Macdonald & Nell, Reference Doubleday, Macdonald and Nell1993), whilst the main part of the Group is interpreted as the infill of a fore-arc basin (Butterworth et al. Reference Butterworth, Crame, Howlett and Macdonald1988). The sedimentary rocks are mostly marine volcaniclastic deposits with fluvial intervals. Seven formations are recognized within the Group (Selene Nunatak, Atoll Nunataks, Ablation Point, Himalia Ridge, Spartan Glacier, Pluto Glacier and Neptune Glacier formations; Fig. 7), with deposition spanning from Middle Jurassic (~ 170 Ma) to Albian times (~ 100 Ma) (Doubleday, Macdonald & Nell, Reference Doubleday, Macdonald and Nell1993; Kelly & Moncrieff, Reference Kelly and Moncrieff1992; Moncrieff & Kelly, Reference Moncrieff and Kelly1993; Nichols & Cantrill, Reference Nichols and Cantrill2002).
Contemporaneous volcanic rocks are rare in the Fossil Bluff Group, although minor mafic volcanic rocks and silicic tuffs have been identified in the far north and south of the basin (Doubleday & Storey, Reference Doubleday and Storey1998). McCarron (Reference McCarron1994) documented Late Cretaceous to Early Tertiary volcanic rocks (mostly andesites) in the fore-arc that post-date the main phase of basin-wide sedimentation.
The stratigraphy of the Fossil Bluff Group records two major stages in the development of the fore-arc basin. The two oldest formations (Selene Nunatak and Atoll Nunataks formations; Fig. 7) are exposed on the west side of the basin and record the transition from trench-slope to fore-arc sedimentation (Doubleday et al. Reference Doubleday, Leat, Alabaster, Nell and Tranter1994). The sediments of these formations were derived from the LeMay Group accretionary complex rather than the main volcanic arc to the east. The later formations are part of the second stage of fore-arc basin sedimentation and represent a large-scale, shallowing-upward succession from Kimmeridgian age to Albian (Butterworth & Macdonald, Reference Butterworth, Macdonald, Thomson, Crame and Thomson1991). The Ablation Point Formation (late Kimmeridgian) crops out in the northeast part of the basin and is characterized by sandy turbidites, interpreted as the collapse of a submarine fan complex.
The Himalia Ridge Formation is found throughout the outcrop extent of the Fossil Bluff Group and is interpreted to be a basin-wide feature, with a thickness of 2.2 km, characterized by steep scarp slopes (Butterworth, Reference Butterworth and Macdonald1991). The Himalia Ridge Formation is interpreted as Tithonian in age (Fig. 7), based on bivalve, ammonite and belemnite fauna, located in the lower 1 km of the succession (Butterworth et al. Reference Butterworth, Crame, Howlett and Macdonald1988). The upper 1 km of the formation largely lacks fossils, with the exception of the upper 50 m, which are regarded as Early Cretaceous in age (Berriasian; Thomson, Reference Thomson1979). The formation is dominated by boulder/cobble conglomerate turbidites and medium-grained sandstones. The conglomerates typically form beds of 1–4 m thickness and can be structureless or graded. The conglomerate clasts are all arc-derived volcanic and plutonic rocks of acidic composition (Butterworth, Reference Butterworth and Macdonald1991). The Himalia Ridge Formation is completely arc-derived and was synchronous with a period of increased arc volcanism (Butterworth, Reference Butterworth and Macdonald1991), with the outcrops at the far north of the basin characterized by the occurrence of rare basaltic sills, pillow lavas and rhyolitic sills/lavas, which were considered to be Oxfordian–Kimmeridgian (~ 155 Ma) in age (Macdonald et al. Reference Macdonald, Leat, Doubleday and Kelly1999).
The Spartan Glacier Formation is approximately 1 km in thickness and represents a basin shallowing succession (Butterworth & Macdonald, Reference Butterworth, Macdonald, Thomson, Crame and Thomson1991) and is characterized by shelf mudstones and thin sandstone units. It contains tectonically induced slide deposits (Storey et al. Reference Storey, Brown, Carter, Doubleday, Hurford, Macdonald and Nell1996). The overlying Pluto Glacier Formation is very similar, but has thicker sandstone beds. The formation has a total thickness of approximately 1400 m and it represents deposition in an inner- to mid-shelf environment.
4.b. Correlations between Alexander Island and Adelaide Island
Any correlations between successions on Alexander Island and Adelaide Island are complicated by the significant distance (150 km) between the two areas, the absence of a basement accretionary complex on Adelaide Island and the paucity of good chronostratigraphic ages for many of the successions. Much of the lithostratigraphy, particularly on Alexander Island and previously on Adelaide Island, is based on molluscan fauna and plant fossil ages, which are in turn based on southern hemisphere correlations. Some molluscan fauna ages have proved robust, but others can be tens of millions of years in disagreement with reliable radiogenic dates. The improved chrono- and lithostratigraphy of Adelaide Island has at least permitted tentative correlations to be made with the successions of Alexander Island.
The Buchia Buttress Formation of central Adelaide Island has been reliably dated at 149.5 ± 1.6 Ma (interbedded crystal tuff), coincident with a Tithonian age suggested by studies of molluscan fauna from the same succession (Thomson, Reference Thomson1972). The boulder/cobble conglomerate and coarse juvenile volcaniclastic sandstones of the Buchia Buttress Formation are interpreted to form a direct correlation or extension of the Himalia Ridge Formation of Alexander Island (Fig. 7), which is characterized by coarse-grained conglomeratic rocks and sandstones that form prominent ridge scarps. The rare silicic rocks of the Buchia Buttress Formation are also reported from the Himalia Ridge Formation, along with basaltic pillow lavas, identified from northern Alexander Island (Macdonald et al. Reference Macdonald, Leat, Doubleday and Kelly1999) and tentatively dated at ~ 155 Ma. The Buchia Buttress Formation forms the lowermost succession on Adelaide Island, whilst the Himalia Ridge Formation also forms the lowest unit in the main fore-arc succession (Butterworth et al. Reference Butterworth, Crame, Howlett and Macdonald1988). Both units are the result of increased volcanic activity in the main arc setting on the Antarctic Peninsula. The primary source of the volcaniclastic and conglomerate material was from the east and southeast of Adelaide and Alexander islands.
A possible correlation between the Milestone Bluff Formation (113.9 ± 1.2 Ma) and the Aptian–Albian Pluto Glacier Formation is also made. The Pluto Glacier Formation forms some of the youngest rocks of the Fossil Bluff Group and at Succession Cliffs they form the uppermost sequence. Both formations are characterized by alternating units of thickly bedded sandstone and siltstone with occasional pebble/cobble conglomerate channels. Rare green crystal tuffs are reported from the upper part of the Succession Cliffs of Alexander Island (Butterworth et al. Reference Butterworth, Crame, Howlett and Macdonald1988), and silicic crystal tuffs and ignimbrites also form occasional horizons in the Milestone Bluff Formation. Ammonites, bivalves and belemnites are present in both formations and have been dated as Aptian–Albian in age at Succession Cliffs (Crame & Howlett, Reference Crame and Howlett1988) and previously thought to be Late Jurassic on Adelaide Island (Thomson & Griffiths, Reference Thomson, Griffiths, Moyes, Willan and Thomson1994), an age which is now known to be incorrect given the crystal tuff age reported here.
The three volcanic formations (Mount Liotard, Bond Nunatak, Reptile Ridge) on Adelaide Island have an age close to the K–T boundary (Reptile Ridge Formation: 67.6 ± 0.7 Ma), which is in good agreement with the fore-arc magmatism described from Alexander Island (McCarron, Reference McCarron1997). The dominantly volcanic sequences from Alexander Island post-date the main episode of fore-arc sedimentation, with the volcanic rocks erupted in the interval 46–80 Ma, with a prominent peak at ~ 65 Ma (McCarron & Millar, Reference McCarron and Millar1997). Rhyolitic ignimbrites of the Colbert Formation (2.5 km thick) are dated at 64.9 ± 1.3 Ma, 64.6 ± 1.0 Ma and 63.3 ± 1.4 Ma and are potentially correlated with the Reptile Ridge Formation rhyolites of Adelaide Island.
The basaltic andesite-dominated Mount Liotard Formation is also correlated with the andesites of the Monteverdi Formation of Alexander Island (Fig. 7), which has been dated at 79.7 ± 2.5 Ma (K–Ar: McCarron & Millar, Reference McCarron and Millar1997). The andesite lavas, volcaniclastic rocks and conglomerates of the Bond Nunatak Formation are in turn correlated with the Finlandia Formation of north central Alexander Island (Fig. 7). Ages from the Finlandia Formation are younger (~ 45–50 Ma; McCarron & Millar, Reference McCarron and Millar1997) than the Bond Nunatak Formation (~ 75 Ma), but the Finlandia Formation ages may reflect resetting by nearby Tertiary plutonism, akin to that observed on Adelaide Island. No geochemical data exist for the andesites from the Bond Nunatak or Mount Liotard formations to confirm if they are high-Mg in character.
The pulse of plutonism on Adelaide Island has been dated by several sources (Pankhurst, Reference Pankhurst1982; Griffiths & Oglethorpe, Reference Griffiths and Oglethorpe1998; this study) in the interval 44–60 Ma. The plutonic rocks (adamellite, granite, diorite: Rouen Intrusive Complex; Fig. 7) of Alexander Island have also been dated by several sources (Pankhurst, Reference Pankhurst1982; Storey et al. Reference Storey, Brown, Carter, Doubleday, Hurford, Macdonald and Nell1996; McCarron & Millar, Reference McCarron and Millar1997) in the interval 46–75 Ma, suggesting a similar history to that seen on Adelaide Island and a similar compositional range from calc-alkaline granitoids to more mafic compositions. The plutonism on Alexander Island is also associated with late-stage mafic dykes, which cross cut the entire succession.
5. Tectonic and volcanic implications
Potential correlations between successions on Adelaide Island and Alexander Island pose interesting issues concerning the position of terrane boundaries on the Antarctic Peninsula as well as the source of the volcaniclastic sediments and also whether the Western Domain (Fig. 1) was exotic (Vaughan & Storey, Reference Vaughan and Storey2000).
The volcaniclastic successions on Adelaide Island have been dated in the interval 150–120 Ma and this work has allowed tentative correlations to the Fossil Bluff Group of Alexander Island (Fig. 7). Possible correlations have also been made between the andesite–rhyolite sequences of Adelaide Island, which have a total thickness close to 2 km, and the north–south linear belt of fore-arc volcanic rocks on Alexander Island (Alexander Island Volcanic Group; McCarron, Reference McCarron1997). However, such correlations are based on lithological similarities and age relationships, but given the distance between units, the correlations should be treated with caution.
The Late Jurassic–Cretaceous volcano-sedimentary sequences and the Late Cretaceous–Tertiary andesite–rhyolite lava successions of Alexander Island and Adelaide Island bracket the Palmer Land tectonic and intrusive event (~ 107 Ma; Vaughan, Pankhurst & Fanning, Reference Vaughan, Pankhurst and Fanning2002; Leat et al. Reference Leat, Riley, Wareham, Millar, Kelley and Storey2002), which was associated with terrane accretion (Vaughan & Storey, Reference Vaughan and Storey2000). The source for the volcaniclastic sediments and conglomerates of the upper parts of the Fossil Bluff Group and the Buchia Buttress and Milestone Bluff formations has to be the main volcanic arc, suggesting coeval volcanic activity from Late Jurassic through to the Albian times. This is consistent with ages determined by Leat et al. (Reference Leat, Flowerdew, Riley, Whitehouse, Scarrow and Millar2009) who documented significant volcanic activity in the NW Palmer Land area (Fig. 1) from ~ 153 Ma to ~ 107 Ma. The NW Palmer Land area is the preferred source region for the fore-arc volcanic and sedimentary rocks and is consistent with the palaeo-flow direction of conglomerate clasts on Adelaide Island (Griffiths & Oglethorpe, Reference Griffiths and Oglethorpe1998). A single granitic conglomerate clast from the Bond Nunatak Formation has yielded an 40Ar–39Ar whole-rock age of 138.1 ± 1.9 Ma (Griffiths & Oglethorpe, Reference Griffiths and Oglethorpe1998), consistent with plutonic ages from NW Palmer Land (Leat et al. Reference Leat, Flowerdew, Riley, Whitehouse, Scarrow and Millar2009). Also, no plutonic rocks of Mesozoic age have been identified either on Adelaide Island or the adjacent coast of Graham Land (Thomson & Pankhurst, 1993; Griffiths & Oglethorpe, Reference Griffiths and Oglethorpe1998; this study).
The Late Cretaceous–Early Tertiary andesite–rhyolite fore-arc volcanic rocks from Alexander Island are distinct from the main Mesozoic arc volcanic rocks of the Antarctic Peninsula, in that they were generated in the fore-arc as a result of ridge subduction (McCarron, Reference McCarron1997). The > 2 km sequence of andesite lavas on Adelaide Island is similar in many respects to the Alexander Island succession and is distinct from the volcanic sequences identified from western Graham Land and Palmer Land.
The pre- and post-Palmer Land event correlations, combined with the source region of the volcano-sedimentary successions, suggest that Alexander Island and Adelaide Island may have formed part of the same fore-arc terrane in Mesozoic reconstructions of the Antarctic Peninsula and also that an exotic origin for Alexander Island and/or Adelaide Island is unlikely given the requirement to be proximal to a significant sediment/volcanic source region, suggested here as western Palmer Land.
Acknowledgements
This study is part of the British Antarctic Survey Polar Science for Planet Earth programme, funded by the Natural Environmental Research Council. Tom Marshall, James Wake and the air operations staff at Rothera Base are thanked for their field support. Kerstin Lindén and Lev Ilyinsky are thanked for their assistance at the NORDSIM facility. This is NORDSIM contribution number 295. This paper benefited from the considered and helpful reviews of David Macdonald, John Smellie and Simon Kelly.