INTRODUCTION
Lake Bonneville was a large pluvial lake that covered much of the eastern Great Basin (Fig. 1) during the late Pleistocene (Gilbert, Reference Gilbert1890; Oviatt, Reference Oviatt2015). After reaching the elevation of ~1,550 m prior to ~18 cal ka BP lake, failure of a natural barrier led to the Bonneville flood, allowing the lake to overflow into Idaho, until at ~14.6 cal ka BP the warming climate of the Bølling-Allerød interstadial caused it to enter its regressive phase (Godsey et al., Reference Godsey, Currey and Chan2005; Oviatt, Reference Oviatt2015). As lake levels fell, Lake Bonneville separated into two bodies of water: the increasingly saline Great Salt Lake and a freshwater lake in the Sevier basin known as Lake Gunnison (Fig. 2) (Oviatt, Reference Oviatt1988, Reference Oviatt2015; Godsey et al., Reference Godsey, Oviatt, Miller and Chan2011).

Figure 1. (color online) Map of the eastern Great Basin in Utah that shows the geographic and geologic features referenced in the text, including the Lake Bonneville shoreline, the Old River Bed inland delta, an approximate shoreline for Lake Gunnison, and present-day lake systems.
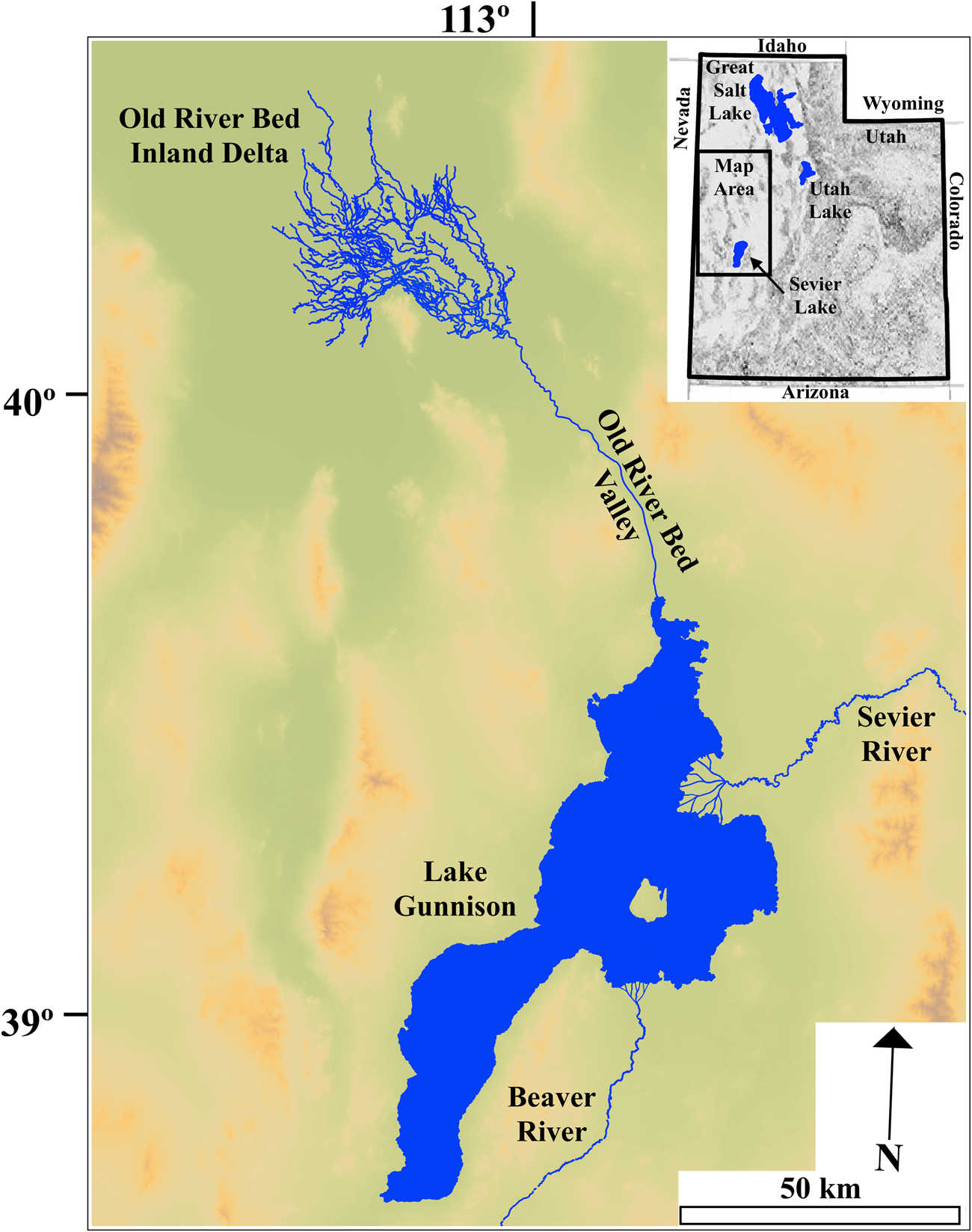
Figure 2. (color online) Map of the Lake Gunnison hydrologic system and the Old River Bed inland delta during the Pleistocene–Holocene transition based in part on Oviatt (Reference Oviatt1988) and Madsen et al. (Reference Madsen, Oviatt, Young, Page, Madsen, Schmitt and Page2015). The Beaver and Sevier River deltas and portions of the Lake Gunnison shoreline are approximations as they are not yet fully mapped.
Water levels at the Great Salt Lake quickly fell to near-modern elevations, rising only briefly during the Gilbert wet episode associated with the Younger Dryas (Oviatt et al., Reference Oviatt, Miller, McGeehin, Zachary and Mahan2005; Oviatt, Reference Oviatt2014). In contrast, Lake Gunnison remained fresh, with significant water flux from the Beaver and Sevier Rivers, and maintained an elevation of ~1,390 m, overflowing at its northern end into the Old River Bed (ORB) valley (Currey, Reference Currey1982; Oviatt, Reference Oviatt1988). This allowed a river to flow northwestward into the Great Salt Lake Desert and onto present-day Dugway Proving Ground (DPG) (Oviatt et al., Reference Oviatt, Madsen and Schmitt2003), where it spread out (see Fig. 2) creating a 2600 km2 system of channels and wetlands known as the ORB inland delta (Fig. 3) (Madsen, Reference Madsen, Schroder and Oviatt2016; Bradbury, Reference Bradbury2019).

Figure 3. (color online) Map illustrating channel systems of the Old River Bed inland delta using the color scheme described by Madsen et al. (Reference Madsen, Oviatt, Young, Page, Madsen, Schmitt and Page2015). Sample locations and average 87Sr/86Sr ratios of mollusks from each channel system are also shown.
As climate in the Great Basin became increasingly arid during the Pleistocene–Holocene transition (Madsen et al., Reference Madsen, Rhode, Grayson, Broughton, Livingston, Hunt, Quade, Schmitt and Shaver2001; Rhode, Reference Rhode, Schroder and Oviatt2016; Thompson et al., Reference Thompson, Oviatt, Honke, McGeehin, Schroder and Oviatt2016), the extensive wetlands and abundant water at the ORB inland delta attracted large game animals and human foragers (Madsen, Reference Madsen, Graff and Schmitt2007, Reference Madsen, Schroder and Oviatt2016). These Pleistocene–Holocene foragers were likely descendants of the first immigrants to enter North America and employed a subsistence strategy focused on the hunting of large game and collection of wetland resources (Elston et al., Reference Elston, Zeanah and Codding2014; Madsen, Reference Madsen, Schroder and Oviatt2016), both of which were likely abundant at the ORB inland delta (Madsen, Reference Madsen, Graff and Schmitt2007). These foragers likely inhabited the DPG region on a semi-permanent basis (Arkush and Pitblado, Reference Arkush and Pitblado2000), leaving behind an extensive archaeological record of their behavior and use of the channel systems and landscape of the ORB inland delta (Madsen et al., Reference Madsen, Oviatt, Young, Page, Madsen, Schmitt and Page2015; Madsen, Reference Madsen, Schroder and Oviatt2016). Much of this record seems to be associated with individual channel systems of the ORB inland delta, whose ages suggest that humans occupied the area from ~13.2 cal ka BP until shortly after ~9.3 cal ka BP (Madsen et al., Reference Madsen, Oviatt, Young, Page, Madsen, Schmitt and Page2015).
Water flow at the ORB inland delta appears to have varied through time, and channels are described as having gravel or sand morphologies, respectively indicating high-energy or low-energy stream flow (Oviatt et al., Reference Oviatt, Madsen and Schmitt2003), with some channels perhaps intermediate between the two (Madsen et al., Reference Madsen, Oviatt, Young, Page, Madsen, Schmitt and Page2015). In general, high-energy gravel channels have ages older than ~11.5 cal ka BP, whereas low-energy sand channels have ages younger than ~11.5 cal ka BP, illustrating a reduction in water supply to the area around this time (Oviatt et al., Reference Oviatt, Madsen and Schmitt2003). This is supported by a coeval lack of lacustrine sediments and appearance of wetlands in the Sevier basin indicating that Lake Gunnison had regressed to a point where overflow into the ORB valley had ceased (Oviatt, Reference Oviatt1988; Oviatt et al., Reference Oviatt, Madsen and Schmitt2003). Because of the persistence of wetlands in the ORB area from ~11.5 cal ka BP until ~9.3 cal ka BP, the change in channels from high- to low-energy morphologies infers a change in the dominant water source from Lake Gunnison overflow to diffuse ground water discharge (Oviatt et al., Reference Oviatt, Madsen and Schmitt2003).
Although evidence shows that the eastern Great Basin was dominated by arid climatic conditions during the Pleistocene–Holocene transition (Rhode, Reference Rhode, Schroder and Oviatt2016; Thompson et al., Reference Thompson, Oviatt, Honke, McGeehin, Schroder and Oviatt2016), several localities within the region, including the ORB inland delta of central Utah, show the persistence of wetland ecosystems during this time as well (Madsen et al., Reference Madsen, Oviatt, Young, Page, Madsen, Schmitt and Page2015; Oviatt et al., Reference Oviatt, Madsen, Miller, Thompson and McGeehin2015; Schmitt and Lupo, Reference Schmitt and Lupo2018). One potential explanation for this discrepancy is that lowland wetlands in the Bonneville basin persisted due to the slow discharge of piedmont aquifers with elevated water tables due to infiltration of Lake Bonneville water earlier in the late Pleistocene (Oviatt et al., Reference Oviatt, Madsen, Miller, Thompson and McGeehin2015; Schmitt and Lupo, Reference Schmitt and Lupo2018). The persistence of mesic-adapted mammal communities at many suspected locations of spring discharge provides support for this hypothesis (Schmitt and Lupo, Reference Schmitt and Lupo2018), although the extent of such discharge has not yet been quantified.
For the ORB inland delta, however, discharge of old Lake Bonneville water from piedmont aquifers was probably not the provenance of ground water reaching the area. Although wetlands and the human occupation of the ORB inland delta are coincident with putative discharge of old Lake Bonneville ground water elsewhere in the eastern Great Basin (Oviatt et al., Reference Oviatt, Madsen, Miller, Thompson and McGeehin2015; Schmitt and Lupo, Reference Schmitt and Lupo2018), ground water reaching the ORB inland delta appears to have come primarily from the Sevier basin (Madsen et al., Reference Madsen, Oviatt, Young, Page, Madsen, Schmitt and Page2015). The radiogenic 87-strontium and stable 86-strontium isotopic ratio (87Sr/86Sr) data for mollusk shells from ORB inland delta channels postdating the regression of Lake Gunnison (Rhode and Louderback, Reference Rhode, Louderback, Madsen, Schmitt and Page2015) have values similar to those of Lake Gunnison mollusks (Hart et al., Reference Hart, Quade, Madsen, Kaufman and Oviatt2004), suggesting that ground water reaching the area originated in the Sevier basin. However, 87Sr/86Sr ratios of mollusks from only six channel systems at the ORB inland delta have been measured thus far, leaving the majority of channels at the ORB inland delta unsampled and their 87Sr/86Sr ratios unknown (Madsen et al., Reference Madsen, Oviatt, Young, Page, Madsen, Schmitt and Page2015). Understanding where water in the region ultimately came from may provide an explanation for the persistence of wetland ecology in the ORB area and concomitant archaeology indicating human occupation of the area throughout the Pleistocene–Holocene transition despite the general drying trend observed in the eastern Great Basin during this time (Madsen et al., Reference Madsen, Rhode, Grayson, Broughton, Livingston, Hunt, Quade, Schmitt and Shaver2001; Rhode, Reference Rhode, Schroder and Oviatt2016; Thompson et al, Reference Thompson, Oviatt, Honke, McGeehin, Schroder and Oviatt2016). To this end, the goal of this study was to test the provenance of water reaching the ORB inland delta during the Pleistocene–Holocene transition and to identify whether that provenance changed through time.
This goal was achieved by collecting mollusk shells from ORB inland delta channel systems previously radiocarbon dated by Madsen et al. (Reference Madsen, Oviatt, Young, Page, Madsen, Schmitt and Page2015) that reflect distinct periods before and after the regression of Lake Gunnison and measuring their 87Sr/86Sr ratios. Strontium readily exchanges with calcium in biological systems (Lenihan, Reference Lenihan1966), calcium-rich biogenic tissues such as shell reflect the isotopic composition of sources of strontium available to an organism when it was alive (Capo et al., Reference Capo, Stewart and Chadwick1998; Kohn and Cerling, Reference Kohn and Cerling2002). As the 87Sr/86Sr ratios of freshwater mollusks are dependent on the ratio of strontium to calcium (Sr/Ca) of a water source (Buchardt and Fritz, Reference Buchardt and Fritz1978), the 87Sr/86Sr ratios of ORB inland delta mollusks can be used as proxies for the chemical properties of the water in which they once lived. Results are compared to the 87Sr/86Sr ratios of potential ground water sources during the Pleistocene–Holocene transition, including the 87Sr/86Sr ratios of mollusks from Lake Gunnison in the Sevier basin, carbonate proxies for the 87Sr/86Sr ratio of Lake Bonneville, and modern ground waters in the DPG area from local aquifers, allowing for the testing of three hypotheses:
1) The 87Sr/86Sr ratios of ORB inland delta mollusks from channels dating between ~13.2 cal ka BP and ~11.5 cal ka BP will match 87Sr/86Sr ratios of Lake Gunnison mollusks (Hart et al., Reference Hart, Quade, Madsen, Kaufman and Oviatt2004) and/or the modeled 87Sr/86Sr ratios for Lake Gunnison, signifying lake overflow was the provenance of water to the ORB inland delta during this time as suggested by Oviatt et al. (Reference Oviatt, Madsen and Schmitt2003), Madsen et al. (Reference Madsen, Oviatt, Young, Page, Madsen, Schmitt and Page2015), and Rhode and Louderback (Reference Rhode, Louderback, Madsen, Schmitt and Page2015).
2) The 87Sr/86Sr ratios of ORB inland delta mollusks from younger channels dating between ~11.5 cal ka BP and ~9.3 cal ka BP will match one of two sources: the 87Sr/86Sr ratios of Lake Bonneville carbonates (Hart et al., Reference Hart, Quade, Madsen, Kaufman and Oviatt2004; Pedone and Oviatt, Reference Pedone and Oviatt2013), indicating a source related to slow discharge of stored lake water from piedmont aquifers, or the 87Sr/86Sr ratios of mollusks from Lake Gunnison (Hart et al., Reference Hart, Quade, Madsen, Kaufman and Oviatt2004), indicating a ground water provenance in the Sevier basin.
3) Water at the ORB inland delta was sourced from a local regional aquifer in the DPG area, and ORB inland delta mollusks will have 87Sr/86Sr ratios similar to modern ground waters in the region, which are higher than those of Lake Bonneville carbonates (Lerback et al., Reference Lerback, Hynek, Bowen, Bradbury, Solomon and Fernandez2019).
METHODS
Field methods
Species of mollusk genera including Anodonta, Helisoma, Physa, Pyrgulopsis, and Stagnicola are found in organic-rich “black mat” deposits associated with the distributary channels of the ORB inland delta. These deposits, and mollusk shells within them, were located by surveying channels that had been previously dated by Madsen et al. (Reference Madsen, Oviatt, Young, Page, Madsen, Schmitt and Page2015). In many instances, shells were found eroding out of channel margins, and samples with intact pearly lusters were obtained a few centimeters beneath the surface. Geographic coordinates of collected mollusks were noted using the NAD83 datum (Table 1).
Table 1. 87Sr/86Sr ratios of Old River Bed inland delta mollusks,

aFor mollusk samples collected, only the genus is reported.
bNAD83 datum used.
c2σ radiocarbon dates of Old River Bed inland delta channels converted to cal ka BP using the CalPal2007_HULU calibration curve (Danzeglocke et al., Reference Danzeglocke, Jöris and Weninger2012) and as reported in Madsen (Reference Madsen, Oviatt, Young, Page, Madsen, Schmitt and Page2015).
dThe Rust channel is not directly dated but cuts through the Seafoam channel and is believed to be younger than ~9.3 cal ka in age (Madsen et al., Reference Madsen, Oviatt, Young, Page, Madsen, Schmitt and Page2015).
Water samples were collected from sources that may have been part of the Lake Gunnison and/or ORB inland delta hydrologic systems. These included the Beaver and Sevier Rivers that emptied into Lake Gunnison, the shallow water-bearing zone and springs of the Dugway playa and playa-margin, deep ground water aquifers in the Sevier basin and ORB valley, ground water or surface water from the Simpson Mountains and Government Creek, and a spring in the Sevier basin (Fig. 4).

Figure 4. (color online) Map showing present-day hydrologic systems discussed in the text, water sample locations, and 87Sr/86Sr ratios and strontium concentration data for each water sample.
Each water sample was collected in a 15-mL polypropylene vial that had been previously acid-washed using 10% hydrochloric acid (HCl) and triple rinsed with deionized Milli-Q water, similar to methods outlined by Brennan et al. (Reference Brennan, Fernandez, Mackey, Cerling, Bataille, Bowen and Wooller2014). After collection, sample vials were labeled, and geographic coordinates were noted in the NAD83 datum (see Fig. 4). Sample vials were then sealed in individual Ziploc® bags to reduce contamination risk. Parameters such as conductivity, pH, and temperature were not recorded for this study.
Sample preparation and analysis
Mollusk shells were broken into large fragments and cleaned by placing them in vials of Milli-Q deionized water (Element, Millipore, Burlington, Massachusetts, USA). Ultrasound was used to remove visible particulate matter. The water was decanted and fragments again rinsed, with the process repeated as necessary until visible particulate matter was completely removed. Shell fragments were then leached in a solution of 0.1% nitric acid (HNO3) for 10 minutes to remove surface contaminants. No dark deposits were observed after treatment. After leaching, the fragments were rinsed with Milli-Q deionized water and dried in a dust-free environment. Approximately 100 mg of shell fragments were digested in 10 mL of 10% HNO3. The mass of shell fragments and the mass of digest were used to calculate the solution factor needed to determine the strontium concentration in shell material. All operations were performed under laminar flow, and the quality of acids used was trace-metal grade. Polypropylene centrifuge tubes were acid-washed.
Aliquots of each water sample were filtered using a polypropylene syringe with an attached 0.45-μm polyethersulfone cartridge filter (Brennan et al., Reference Brennan, Fernandez, Mackey, Cerling, Bataille, Bowen and Wooller2014), both of which were acid-washed using 10% HCl and triple rinsed with Milli-Q deionized water prior to use. Aliquots of each filtered sample were then analyzed for strontium concentration and 87Sr/86Sr values.
Strontium concentration determination
The concentrations of strontium and calcium in shell and water samples were measured using an Agilent 7500ce quadrupole inductively coupled plasma mass spectrometer (ICP-MS) with a double-pass spray chamber with a perfluoralkoxy nebulizer (0.1 mL/min), a quartz torch, and platinum cones. Sample dilutions were performed using calibrated pipettors (Eppendorf, Hauppauge, New York, USA) with a target concentration in the range 20–200 ppb Sr and 2–20 ppm Ca. An external calibration curve was prepared using single-element strontium and calcium standard solutions (Inorganic Ventures, Christiansburg, Virginia, USA). Indium at a concentration of 10 ppb was added to diluted samples, calibration curve, and machine blanks and used as an internal standard. Machine blanks were included in the sequence at a rate of two blanks every six samples, and the standard deviation of the signal obtained for the machine blanks was used to determine the detection limit. Certified reference material 1643e (CRM 1643e, Trace Elements in Water, National Institute of Standards and Technology, Gaithersburg, Massachusetts, USA) was run in the same sequence with samples (once every 6 samples). The average strontium and calcium concentrations determined during runs for CRM 1643e were within 5% of the certified values.
87Sr/86Sr determination
An aliquot of the shell digest or water sample containing 200 ng of strontium was apportioned for 87Sr/86Sr analysis using a multi-collector ICP-MS (MC-ICP-MS) (Neptune, Thermo Scientific, Bremen, Germany). The strontium contained in the aliquot was purified using automated ion chromatography with PrepFAST MC (ESI, Omaha, Nebraska, USA), equipped with a 1-mL column containing SrCa resin (Sr-Spec Resin®, Eichrom, Lisle, Illinois) (Mackey and Fernandez, Reference Mackey and Fernandez2011). Purified samples were introduced into the MC-ICP-MS using a self-aspiration perfluoralkoxy nebulizer (0.05 mL/min), double-pass quartz spray chamber, sapphire injector, and nickel cones. Standard Rb-Sr cup configuration (82Kr-L4, 83Kr-L3, 84Kr-L2, 85Rb-L1, 86Sr-C, 87Sr-H1, 88Sr-H2) was used. The 87Sr/86Sr ratios were corrected for mass bias using an exponential law, normalizing to 86Sr/88Sr = 0.1194 (Steiger and Jager, Reference Steiger and Jager1977). Isobaric interferences on 87Sr/86Sr ratios, such as from 87Rb and 86Kr, were corrected by simultaneous monitoring of 85Rb and 83Kr and using the corresponding invariant ratios 87Rb/85Rb = 0.385706 and 86Kr/83Kr = 1.502522 (Steiger and Jager, Reference Steiger and Jager1977). Certified reference material CRM 987 (SrCO3, National Institute of Standards and Technology) was used to check the quality of the data. First, a solution of CRM 987 in 6M HNO3 was purified for strontium in the same way as sample aliquots, and this purified standard was run as a sample during each run. Second, a solution of CRM 987 in 5% HNO3 was run as a standard together with samples (1 standard every 3 samples) during each MC-ICP-MS sequence. Machine blanks were run before and after samples or standards, and the intensities from the previous blank in the sequence were used for blank correction of each isotope identified. CRM 987 certified 87Sr/86Sr value is 0.71034 ± 0.00026. The long-term precision average and SD (1σ) measured in our lab is 0.710285 ± 0.000015.
River discharge estimates
Discharge estimates for the Beaver and Sevier Rivers are those of Hart et al. (Reference Hart, Quade, Madsen, Kaufman and Oviatt2004) and are expressed as the fraction of total discharge each river contributed to Lake Gunnison rather than an absolute discharge amount. They are based on the Sevier River having five times the discharge of the Beaver River (Hart et al., Reference Hart, Quade, Madsen, Kaufman and Oviatt2004).
RESULTS
Old River Bed inland delta mollusks
The lowest 87Sr/86Sr ratio measured was that of a large Stagnicola specimen from the Black D channel (0.70975) (see Fig. 3). The Black D channel of the Black channel system was active from ~12.9 cal ka BP until ~12.2 cal ka BP, making it among the oldest at the inland delta (Madsen et al., Reference Madsen, Oviatt, Young, Page, Madsen, Schmitt and Page2015). Two other Stagnicola shells from the Black D channel have moderately higher 87Sr/86Sr ratios (0.71007, 0.71009), and averaging the three yields an 87Sr/86Sr ratio of 0.70997.
The Green and Red channels (see Fig. 3) were active from ~12.2 cal ka BP until ~11.2 cal ka BP, an age range that overlaps the regression of Lake Gunnison at ~11.5 cal ka BP (Oviatt, Reference Oviatt1988; Madsen et al., Reference Madsen, Oviatt, Young, Page, Madsen, Schmitt and Page2015). The 87Sr/86Sr ratios for mollusks from the Green (Helisoma, 0.71046; Stagnicola, 0.71011) and Red (Planorbis, 0.71016; Physa, 0.71017) channels are similar to each other, likely due to the channels being contemporaneously active (Madsen et al., Reference Madsen, Oviatt, Young, Page, Madsen, Schmitt and Page2015). The average 87Sr/86Sr ratio of these two channels is 0.71022.
The Blue channel (see Fig. 3) is particularly important, as it is the first channel that clearly postdates the regression of Lake Gunnison. Active between ~11.2 cal ka BP and ~10.6 cal ka BP (Madsen et al., Reference Madsen, Oviatt, Young, Page, Madsen, Schmitt and Page2015), measured 87Sr/86Sr ratios (Stagnicola, 0.70977; Unknown, 0.70995, Stagnicola, 0.70982) for Blue channel mollusks are lower than other channel systems (see Table 1). The average 87Sr/86Sr ratio for the channel is 0.70985.
The Light Blue channel is well developed in the eastern inland delta (see Fig. 3) and was active from ~11.2 cal ka BP until ~9.8 cal ka BP (Madsen et al., Reference Madsen, Oviatt, Young, Page, Madsen, Schmitt and Page2015). It is of particular interest to archaeologists, as numerous artifacts are found along its banks (Madsen et al., Reference Madsen, Oviatt, Young, Page, Madsen, Schmitt and Page2015). The 87Sr/86Sr ratios (Helisoma, 0.71033; Stagnicola, 0.71012; Unknown, 0.71032; Anodonta, 0.71022; Stagnicola, 0.71010; Helisoma, 0.71023; Physa, 0.71009) for mollusks from this channel are similar and have an average of value of 0.71020.
The Buff and Brown channels are respectively dated to ~10.3 cal ka BP and ~10.2 cal ka BP (Madsen et al., Reference Madsen, Oviatt, Young, Page, Madsen, Schmitt and Page2015). The 87Sr/86Sr ratios of shells from the Buff (Physa, 0.71017; Unknown, 0.71005) and Brown (Stagnicola, 0.71001; Helisoma, 0.71001) channels are similar to each other, suggesting that water in each was of a similar provenance. The two systems have an average 87Sr/86Sr ratio of 0.71006.
The youngest dated channel system surveyed at the ORB inland delta is the Seafoam channel (see Fig. 3), which was active between ~10.2 and ~9.3 cal ka BP (Madsen et al., Reference Madsen, Oviatt, Young, Page, Madsen, Schmitt and Page2015). The 87Sr/86Sr ratio (Anodonta, 0.71021) of a mollusk from the channel is similar to the ratios of shells from the Light Blue, Red, and Green channel systems (see Table 1). The Rust channel (see Fig. 3) is not formally age dated but cuts through the Seafoam channel and is therefore likely younger than ~9.3 cal ka BP (Madsen et al., Reference Madsen, Oviatt, Young, Page, Madsen, Schmitt and Page2015). A shell fragment from the Rust channel was found to have an 87Sr/86Sr ratio (Unknown, 0.71106) that is distinctly higher than the range of values measured for shells from other channels (0.70975–0.71046).
Water samples
The 87Sr/86Sr ratios for the Beaver River increase downstream, with water from Beaver Canyon having a lower ratio (0.70672) than waters at the Kiersek Nursery (0.70724) (see Fig. 4). A 2001 measurement from the river by Hart et al. (Reference Hart, Quade, Madsen, Kaufman and Oviatt2004) is similar (0.70782), suggesting that the 87Sr/86Sr ratio of the Beaver River is relatively consistent through time.
The Sevier River was sampled at three locations along its length: headwaters near Hatch, Utah; downstream at the Delta Shooting Range; and at Gunnison Reservoir (see Fig. 4). The Hatch sample is downstream of Mammoth Creek, a major tributary to the Sevier River draining the Markagunt Plateau. This 87Sr/86Sr ratio (0.70993) was higher than samples from further downstream at the Delta Shooting Range (0.70931) and Gunnison Reservoir (0.70913). Values are moderately higher than those measured by Hart et al. (Reference Hart, Quade, Madsen, Kaufman and Oviatt2004) (0.70857–0.70912), perhaps due to changes in human land use of the region that have accelerated the extraction of strontium from rocks, similar to observations of rivers near Lake Biwa, Japan (Nakano et al., Reference Nakano, Tayasu, Wada, Igeta, Hyodo and Miura2005).
Aquifer ground water was sampled from three private wells: one in the Sevier basin and two in the ORB valley. In all cases, water was from depths greater than 17 m, and wells were purged prior to sampling. The Henrie Family well in the Sevier basin (see Fig. 4) has an 87Sr/86Sr ratio (0.70916) similar to the Sevier River, especially the Gunnison Reservoir (0.70913). In contrast, the 87Sr/86Sr ratios of aquifer ground waters from the Keg Mountain Ranch (0.71089) and River Bed Ranch (0.71164) in the ORB valley have higher ratios (Table 2).
Table 2. Water 87Sr/86Sr ratios.

aNAD83 datum used.
The shallow water-bearing zone of the Dugway playa is approximately 1 m below the surface and is in unconfined or locally confined interbedded sand and clay deposits with low transmissivity rates (Fitzmayer et al., Reference Fitzmayer, Larsen, Braxton, Staes and Spangler2004). Waters from this zone have high 87Sr/86Sr ratios (0.71171, 0.71197, 0.71214) similar to those of the TS3-1 (0.71231) and Staley (0.71249) spring systems on the Dugway playa (see Fig. 4).
The highest ground water 87Sr/86Sr ratios are from springs located along the margin of the Dugway playa. This includes Fish Springs (0.71327, 0.71324) and Redden Springs (0.71308) (see Fig. 4), whose higher 87Sr/86Sr ratios suggest discharge from an aquifer in which water is interacting with deeper, more radiogenic materials, differentiating them from the ground waters of the Dugway playa (Lerback et al., Reference Lerback, Hynek, Bowen, Bradbury, Solomon and Fernandez2019).
The 87Sr/86Sr ratios of Government Creek (0.71044) and Simpson Springs (0.71108) differ from one another despite being in the same general region (see Fig. 4). This may be because Government Creek drains both the Simpson and Sheeprock Mountains (Fitzmayer et al., Reference Fitzmayer, Larsen, Braxton, Staes and Spangler2004) or because Simpson Springs represents ground water discharge at a singular location near the Simpson Mountains.
Baker Hot Springs was the only thermal spring sampled in the Sevier basin (see Fig. 4). Its 87Sr/86Sr ratio (0.71275) is higher than values for the Beaver and Sevier Rivers and is consistent with values measured for other springs in the Sevier basin with similarly high 87Sr/86Sr ratios (Oviatt, C. G. and Pedone, V., written communication, 2018). Therefore, based on its similarities to ground water elsewhere in the region, Baker Hot Springs was used as a proxy for ground water in the mass balance model for Lake Gunnison.
Mass balance model
Earlier attempts at modeling the 87Sr/86Sr ratio of Lake Gunnison using a mass balance model were hampered by the lack of ground water data in the Sevier basin (Hart et al., Reference Hart, Quade, Madsen, Kaufman and Oviatt2004). This led to a mismatch between predicted 87Sr/86Sr ratios for Lake Gunnison in the Sevier basin (0.70850) and observed ratios (0.70930–0.71049) of lake mollusks (Hart et al., Reference Hart, Quade, Madsen, Kaufman and Oviatt2004). The present study attempts to rectify this by using the 87Sr/86Sr ratio and strontium concentration data for a ground water sample from Baker Hot Springs in the Sevier basin (see Fig. 4). The mass balance equation for Lake Gunnison is

where f represents the fraction of strontium input from each source and the 87Sr/86Sr ratios are those for each component of the hydrologic system.
This model does not include strontium input from rainfall as it is considered negligible for large bodies of water (Graustein and Armstrong, Reference Graustein and Armstrong1983; Gosz and Moore, Reference Gosz and Moore1989; Hart et al., Reference Hart, Quade, Madsen, Kaufman and Oviatt2004), nor does it include strontium input from eolian dust due to a lack of available data for the region. The initial ground water discharge estimate (2.9 m3/s) is the estimated value for spring discharge elsewhere in the region (Waddell and Barton, Reference Waddell and Barton1980) and the same value used by Hart et al. (Reference Hart, Quade, Madsen, Kaufman and Oviatt2004) in their mass balance models.
Using data for Baker Hot Springs as a proxy for Sevier basin ground water, the 87Sr/86Sr ratios and strontium concentration of the Beaver and Sevier Rivers measured by Hart et al. (Reference Hart, Quade, Madsen, Kaufman and Oviatt2004) and this study, allows estimation of the 87Sr/86Sr ratio for Lake Gunnison water. Resulting values (Table 3) are in reasonable agreement with published values of Lake Gunnison mollusks (0.70930–0.71049). Sensitivity analysis of the data (Fig. 5) was completed for estimated ground water discharge rates ranging from 0.0 m3/s to 6.0 m3/s. Ratios of 87Sr/86Sr consistent with those of Lake Gunnison mollusks were calculated for ground water discharge values between 2.9 m3/s and 4.5 m3/s (see Fig. 5), showing the fraction of total discharge into Lake Gunnison by ground water was 0.04–0.14. This indicates that ground water was a significant component of the Sevier basin hydrologic system and may indicate that discharge of Lake Bonneville ground water from aquifers occurred in the Sevier basin, similar to that which is believed to have been occurring elsewhere in the eastern Great Basin at this time (Oviatt et al., Reference Oviatt, Madsen, Miller, Thompson and McGeehin2015).

Figure 5. (color online) Sensitivity analysis showing the fraction of total discharge values for ground water that yield 87Sr/86Sr ratios consistent with published values for Lake Gunnison mollusks (Hart et al., Reference Hart, Quade, Madsen, Kaufman and Oviatt2004). Results show ground water discharge was an important component of the Lake Gunnison hydrologic system in the Sevier basin.
Table 3. Mass balance model results.

a 87Sr/86Sr ratio and strontium concentration (Sr) data as reported by Hart and colleagues (Reference Hart, Quade, Madsen, Kaufman and Oviatt2004).
b 87Sr/86Sr ratio and strontium concentration data of water samples measured for this study (Table 2).
DISCUSSION
Hypothesis one: Lake Gunnison overflow from ~13.2–11.5 cal ka BP
The results of this study support the first hypothesis that the 87Sr/86Sr ratios of mollusks from the Black D, Green, and Red channels (0.70975–0.71046) (see Fig. 3) are within the expected value range of Lake Gunnison (0.70930–0.71049) (Fig. 6). Channel values are also within the range of estimated 87Sr/86Sr ratios calculated for Lake Gunnison using the updated mass balance model (see Table 3). These 87Sr/86Sr ratios, in conjunction with older channels that have geomorphological characteristics indicative of high-energy stream flow (Oviatt et al., Reference Oviatt, Madsen and Schmitt2003), favor the hypothesis that lake overflow was the likely source of water to the ORB inland delta until Lake Gunnison regressed at ~11.5 cal ka BP.

Figure 6. (color online) The range of 87Sr/86Sr ratios (vertical bars) for n mollusk samples from Old River Bed inland delta channels plotted against the range of cal ka BP ages (horizontal bars) for those channels. A diamond indicates that only one sample (n=1) was analyzed for a channel. The red vertical line marks the regression of Lake Gunnison at ~11.5 cal ka BP. The 87Sr/86Sr ratios of mollusks from the Old River Bed inland delta consistently fall within the range of published values for Lake Gunnison mollusks (0.70930–0.71049) (Hart et al., Reference Hart, Quade, Madsen, Kaufman and Oviatt2004), even after the regression of Lake Gunnison. They do not fall within the range of known values for Lake Bonneville carbonates (0.71129–0.71175) (Hart et al., Reference Hart, Quade, Madsen, Kaufman and Oviatt2004; Pedone and Oviatt, Reference Pedone and Oviatt2013). The anomalously high 87Sr/86Sr ratio of the Rust channel (0.71106) was the only channel whose value fell within the range of local aquifer ground waters (0.71044–0.71108) reported in this study (Table 2) and is similar to that measured for Simpson Springs. This may indicate that spring discharge from the Simpson Mountains into Government Creek was the dominant source of water to the Old River Bed inland delta after ~9.3 cal ka BP.
Hypothesis two: Ground water discharge from ~11.5–9.3 cal ka BP
The Blue, Buff, Brown, Light Blue, and Seafoam channels have ages younger than ~11.2 cal ka (Madsen et al., Reference Madsen, Oviatt, Young, Page, Madsen, Schmitt and Page2015) and 87Sr/86Sr ratios (0.70977–0.71033) similar to older channels related to Lake Gunnison overflow (0.70975–0.71046) (see Fig. 6). This places the 87Sr/86Sr ratios of younger channels along a continuum (see Fig. 6) with the 87Sr/86Sr ratios of Lake Gunnison mollusks (0.70930–0.71049) (Hart et al., Reference Hart, Quade, Madsen, Kaufman and Oviatt2004). However, the regression of Lake Gunnison at ~11.5 cal ka (Oviatt, Reference Oviatt1988) implies lake overflow alone cannot explain these observations. Therefore, the results support the hypothesis that younger channels at the ORB inland delta were fed by ground water originating in the Sevier basin (Madsen et al., Reference Madsen, Oviatt, Young, Page, Madsen, Schmitt and Page2015; Rhode and Louderback, Reference Rhode, Louderback, Madsen, Schmitt and Page2015). No channel systems were found to have 87Sr/86Sr ratios similar to those of Lake Bonneville carbonates (0.71129–0.71175) (Hart et al., Reference Hart, Quade, Madsen, Kaufman and Oviatt2004; Pedone and Oviatt, Reference Pedone and Oviatt2013), and the data do not support the hypothesis that ground water at the ORB inland delta was due to discharge from piedmont aquifers infiltrated by the pluvial lake (see Fig. 6).
Hypothesis three: Local aquifer discharge
The 87Sr/86Sr ratios of modern ground water at the Dugway playa and playa-margin springs (0.71171–0.71327) are mostly higher than those of Lake Bonneville carbonates (0.71129–0.71175) and far exceed the range of published values for mollusks from Lake Gunnison in the Sevier basin (0.70930–0.71049) or those measured at the ORB inland delta (0.70975–0.71046). Even deep aquifer ground water in the ORB valley (0.71089–0.71164) has values higher than those measured for ORB inland delta mollusks. However, ground waters discharged from Government Creek and Simpson Springs (see Fig. 4) have lower 87Sr/86Sr ratios of 0.71044 and 0.71108, respectively (see Table 2), similar to those measured for ORB inland delta mollusks.
The value of Simpson Springs is particularly intriguing, as it is nearly equivalent to the 87Sr/86Sr ratio (0.71106) of the Rust channel (see Fig. 3). The Rust channel has the only 87Sr/86Sr ratio departing from the Lake Gunnison continuum (see Fig. 6) and is the youngest channel system of the ORB inland delta with an age hypothesized to be younger than ~9.3 cal ka BP (Madsen et al., Reference Madsen, Oviatt, Young, Page, Madsen, Schmitt and Page2015). The location of the Rust channel in the eastern inland delta does, however, provide a potential explanation for its anomalously high 87Sr/86Sr ratio.
Simpson Springs is located at the northwest margin of the Simpson Mountains, which along with the Sheeprock Mountains compose the Government Creek drainage (see Fig. 4) (Fitzmayer et al., Reference Fitzmayer, Larsen, Braxton, Staes and Spangler2004). Channels of the easternmost ORB inland delta are down gradient from Government Creek and probably received water from this source (Oviatt et al., Reference Oviatt, Madsen and Schmitt2003). Although Simpson Springs most likely did not supply water to the ORB inland delta directly, its 87Sr/86Sr ratio may be reflective of spring discharge elsewhere in the Simpson Mountains. If so, this may indicate that increased spring discharge in the Simpson Mountains during the early Holocene reached Government Creek and flowed into the easternmost ORB inland delta and Rust channel (see Fig. 3). This could be tested by sampling spring systems in the Simpson and Sheeprock Mountains and measuring their 87Sr/86Sr ratios and certainly merits further investigation.
Thus, the 87Sr/86Sr ratio of the Rust channel may represent stream flow related to the modern hydrologic system of ground water flow from the Simpson Mountains. It is important to note that this was the only mollusk shell analyzed that supports the hypothesis that local aquifer discharge was the provenance of ground water at the ORB inland delta; therefore, in the case of the Rust channel, the 87Sr/86Sr ratios of mollusks from the ORB inland delta provide circumstantial evidence in support of the third hypothesis.
Implications for ground water flow from the Sevier basin
Mollusks from younger channels of the ORB inland delta that were active after Lake Gunnison regressed at ~11.5 cal ka BP are within the expected range of 87Sr/86Sr ratios of mollusks from Lake Gunnison in the Sevier basin (0.70930–0.71049) (see Fig. 6) (Hart et al., Reference Hart, Quade, Madsen, Kaufman and Oviatt2004). This leads to the conclusion that water reaching the ORB inland delta came from the Sevier basin both prior to and after the regression of Lake Gunnison. To explain these observations, a variation of the Oviatt et al. (Reference Oviatt, Madsen, Miller, Thompson and McGeehin2015) ground water discharge hypothesis is proposed.
In this scenario, despite Lake Gunnison regressing below its overflow threshold at ~11.5 cal ka BP, water flow continued into the Sevier basin via the Beaver and Sevier Rivers. This created a shallow water table, as evidenced by the appearance of wetlands in the Sevier basin at this time (Oviatt, Reference Oviatt1988; Madsen et al., Reference Madsen, Oviatt, Young, Page, Madsen, Schmitt and Page2015), in turn recharging a near-surface phreatic aquifer system. It is speculated that this was perhaps similar to seepage from Lake Chad recharging a phreatic aquifer in the African Sahel (Isiorho et al., Reference Isiorho, Matisoff and When1996) and allowed ground water to flow from the Sevier basin to the ORB inland delta along a subsurface flowpath, perhaps a paleochannel through the ORB valley. Paleochannels and other preferential subsurface flowpaths have high hydraulic conductivities and short residence times, allowing them to transport water with chemical properties similar to that of surface waters (Sophocleous, Reference Sophocleous2002) and may explain why the range of 87Sr/86Sr ratios (0.70977–0.71033) of younger ORB inland delta channels is within the range of known 87Sr/86Sr ratios for Lake Gunnison (0.70930–0.71049) as reported by Hart et al. (Reference Hart, Quade, Madsen, Kaufman and Oviatt2004).
Implications for Old River Bed archaeology
Water availability is likely what mattered most to the foragers of the ORB inland delta, and determining the source of this water allows for a better understanding of why people remained in the area despite increasing regional aridity and the regression of Lake Gunnison. Long ground water flowpaths, such as the phreatic aquifer we hypothesize to have transported ground water from the Sevier basin along the ORB valley, are generally more consistent water sources than discharge from short flowpaths (Khan and Khan, Reference Khan, Khan, Senapathi, Prasanna and Chung2019). This consistent ground water flow from the Sevier basin would have sustained the wetlands of the ORB inland delta and maintained resource availability for human use throughout the Pleistocene–Holocene transition. This may explain the long occupation of the ORB inland delta by human foragers and the extensive recycling of artifacts, which implies that people remained in the area for long periods of time and likely only moved short distances within the inland delta as resources became depleted around their habitation sites (Oviatt et al., Reference Oviatt, Madsen and Schmitt2003; Schmitt et al., Reference Schmitt, Madsen, Oviatt, Quist, Graf and Schmitt2007).
Ground water flow from the Sevier basin during the Pleistocene–Holocene transition may also provide an explanation for the observed archaeological record in the ORB valley. Evidence for human activity in the ORB valley is rare, except at its southern and northern ends, and has been hypothesized to be due to either the burial of archaeological sites beneath Holocene sediment or the unsuitability of the valley for Pleistocene–Holocene foragers (Trammell and DeGraffenried, Reference Trammell and DeGraffenried2012). Based on the geochemical evidence of ground water flow from the Sevier basin, it is reasonable to hypothesize that the lack of archaeological sites in the mid-ORB valley, especially after the regression of Lake Gunnison after ~11.5 cal ka BP, may have been due to a lack of consistent surface water within the valley and associated wetland resources utilized by the Pleistocene–Holocene foragers of this region.
CONCLUSIONS
The 87Sr/86Sr ratios of mollusks from older channels of the ORB inland delta have values (0.70975–0.71046) similar to those modeled (see Fig. 5) and measured (0.70930–0.71049) for Lake Gunnison (Hart et al., Reference Hart, Quade, Madsen, Kaufman and Oviatt2004). This is consistent with the hypothesis that Lake Gunnison overflow brought water to the region when these channels were active between ~13.2 cal ka BP and ~11.2 cal ka BP (Madsen et al., Reference Madsen, Oviatt, Young, Page, Madsen, Schmitt and Page2015; Rhode and Louderback, Reference Rhode, Louderback, Madsen, Schmitt and Page2015).
The 87Sr/86Sr ratios of mollusks from younger channels at the ORB inland delta have values (0.70977–0.71033) along the same continuum as those of older channels (see Fig. 6) and also suggest a water source in the Sevier basin. Mollusks do not have 87Sr/86Sr ratios similar to those measured for Lake Bonneville carbonates (0.71129–0.71175) (Hart et al., Reference Hart, Quade, Madsen, Kaufman and Oviatt2004; Pedone and Oviatt, Reference Pedone and Oviatt2013) and do not support the hypothesis that wetlands at the ORB inland delta were sustained by the slow discharge of stored Lake Bonneville ground water from piedmont aquifers (see Fig. 6). Furthermore, with the exception of the Rust channel, which is believed to be younger than ~9.3 cal ka BP, 87Sr/86Sr ratios of modern ground waters (see Table 2) did not match measured 87Sr/86Sr ratios of ORB inland delta mollusks and appear to rule out a local aquifer as a primary source of ground water discharge during the Pleistocene–Holocene transition. Based on these results, we propose for consideration a variation of the Oviatt et al. (Reference Oviatt, Madsen, Miller, Thompson and McGeehin2015) ground water discharge hypothesis; in our hypothesis, ground water was transported from the Sevier basin to the ORB inland delta via a phreatic aquifer, potentially recharged by a shallow water table in the Sevier basin during the Pleistocene–Holocene transition.
Continued ground water flow from the Sevier basin to the ORB inland delta after the regression of Lake Gunnison at ~11.5 cal ka BP (Oviatt, Reference Oviatt1988) permitted the continued availability of resources for human foragers despite the increasingly xeric climate of the region at this time (Madsen et al., Reference Madsen, Rhode, Grayson, Broughton, Livingston, Hunt, Quade, Schmitt and Shaver2001; Rhode, Reference Rhode, Schroder and Oviatt2016; Thompson et al., Reference Thompson, Oviatt, Honke, McGeehin, Schroder and Oviatt2016). The Pleistocene–Holocene transition was a period of significant technological innovation and behavioral adaptation by the foragers in the Great Basin (Jones and Beck, Reference Jones, Beck, Bousman and Vierra2012). Consistent ground water discharge that sustained wetlands at the ORB inland delta allowed for the continued human occupation of the area, and the inland delta may have been an oasis in the region that provided time for human foragers to develop and implement adaptive responses to their changing environment.
ACKNOWLEDGMENTS
This research was completed in partial fulfillment of the requirements for a Doctor of Philosophy in Geology at the University of Utah. Funding for the 87Sr/86Sr ratio analyses of mollusks and water was provided by the IMWU-PWEP (Environmental Programs) Cultural Resources Office of Dugway Proving Ground as part of their ongoing mission to facilitate successful implementation of the National Historic Preservation Act. The authors did not receive any financial compensation for this work.
We thank those individuals who helped provide the framework for the ideas presented in this study: Thure Cerling, Rachel Quist, Charles Oviatt, Nathan Anderson, Nathan Nelson, David Tillson, and Cole Sterling. Special thanks to Bryce Sansing who assisted with sample collection in Beaver Canyon and to Theo Kaplan, Chuck Cosgrove, David Henrie, and Sam Kiersek, all of whom allowed sampling of water on their respective properties.