INTRODUCTION
Wind deposited silts are widespread in the mid-Atlantic region of the United States but receive considerably less attention than loess deposits elsewhere in the United States and worldwide. Loess distribution maps for North America generally show broad deposits in the Midwest stretching down the Mississippi valley, in the Pacific Northwest, and Alaska (Pye, Reference Pye1987; Bettis et al., Reference Bettis, Muhs, Roberts and Wintle2003; Muhs, Reference Muhs2007; Roberts et al., Reference Roberts, Muhs and Bettis2007). Although the mid-Atlantic loess deposits are less extensive and thinner than elsewhere, they are no less important in evaluating landscape evolution and past climatic changes. Mid-Atlantic loess is particularly important in understanding the early cultural history of the region and North America. In this article, we examined the distribution of loess in the northern mid-Atlantic (including Pennsylvania, New Jersey, Delaware, Maryland, and Virginia) by reviewing previous work done and summarizing our ongoing loess research on the Delmarva Peninsula and the region.
SETTING AND PREVIOUS LOESS RESEARCH IN THE MID-ATLANTIC
During the last glacial maximum (LGM) the Laurentide Ice Sheet reached into Pennsylvania, angling from southern New York to the east across northeastern Pennsylvania and northern New Jersey to Long Island, New York. In the west, glacial ice covered the northwestern corner of Pennsylvania from New York to Ohio (Berg et al., Reference Berg, Edmunds, Geyer, Glover, Hoskins, MacLachlan, Root, Sevon and Socolow1980; Sevon and Braun, Reference Sevon and Braun1997). The Late Wisconsinan glacial front reached its maximum extent approximately 26 ka BP and retreated from its terminal position beginning around 22.5 ka BP (Braun, Reference Braun2006). Major rivers draining the glaciated region of Pennsylvania, New Jersey, and New York included the Allegheny River flowing south across western Pennsylvania to join the Ohio River and eventually the Mississippi River; the Susquehanna River flowing south across central Pennsylvania and emptying into the Atlantic Ocean in Maryland; and the Delaware River flowing south at the border between Pennsylvania and New Jersey and into the Atlantic Ocean between New Jersey and Delaware. On the Atlantic coast, the LGM sea level ranged from 120 m (Fairbanks, Reference Fairbanks1989; McHugh et al., Reference McHugh, Hartin, Mountain and Gould2010) to 85 m below present (Dillon and Oldale, Reference Dillon and Oldale1978), and the continental shelf was exposed more than 75 km beyond the present shoreline. For the purposes of this article, the northern mid-Atlantic includes portions of the Appalachian Plateaus, Ridge and Valley, Blue Ridge, and Coastal Plain physiographic provinces.
In the mid-Atlantic, soils formed in silty sediments, and loess was recognized early in the twentieth century. Early Soil Survey reports from the Delmarva Peninsula in Maryland noted the similarity of silty parent sediments to loessial soils elsewhere; however, the confounding proximity of the Chesapeake Bay and low elevation led workers to the conclusion that the silt deposit was “laid down under the sea” (Perkins and Hershberger, Reference Perkins and Hershberger1929: 18). By 1964, in at least some Maryland county Soil Survey reports, the silts were described as a “mantle of silts or loess, [which] probably was blown from glaciated areas to the north” (Matthews, Reference Matthews1963: 62). In Pennsylvania, Peltier’s (Reference Peltier1949) investigation of Susquehanna River terraces identified loess on Pleistocene terraces along the north and west branches and main stem of the Susquehanna attributing the parent silts to glacial outwash plains along the river. Peltier also noted loess along the Juniata River, which did not drain a glaciated region. Thorp et al. (Reference Thorp, Smith, Baldwin, Bowser, Flint, Gould, Moss, Reed, Smith and Trowbridge1952) in mapping Pleistocene eolian deposits depicted loess deposits along the length of the Allegheny River in western Pennsylvania, along the Susquehanna River, and the lower Delaware River but none on the Delmarva Peninsula or in southern Maryland along the Chesapeake Bay.
The first study focused on loess in the region was in New Jersey. Tedrow and MacClintock (Reference Tedrow and MacClintock1953) identified a loess deposit east of the Delaware River as thick as 3 m, but generally between 0.6 m and 0.9 m, overlying varying bedrock. The carbonate-free silts were absent on rolling back slopes, and particle size decreased with distance from the river. Tedrow and MacClintock (Reference Tedrow and MacClintock1953) hypothesized that there had likely been a continuous loess mantle that had been eroded from all but flat areas post deposition. They concluded that the parent silts came from broad outwash plains along the Delaware River draining glaciated northern Pennsylvania and New Jersey and southern New York and that the loess was deposited during the Pleistocene (Tedrow and MacClintock, Reference Tedrow and MacClintock1953).
In Pennsylvania, loess research has focused on the central portion of the state along the Susquehanna River and the southeast along the Delaware River with deposit thickness generally less than 1.5 m (Ciolkosz et al., Reference Ciolkosz, Cronce and Sevon1986). Millette and Higbee (Reference Millette and Higbee1958) studied loess along the Susquehanna, sampling for comparison with fine alluvium and loess in Quebec, Canada. From southcentral Pennsylvania east of the Susquehanna River, two charcoal samples from the contact of loess with underlying materials returned anomalously young dates (600±60 14C yr BP and 2350±70 14C yr BP), which were interpreted as the likely result of sample contamination (Ciolkosz, Reference Ciolkosz2000). Carey et al. (Reference Carey, Cunningham and Williams1976) sampled a transect though loess in southeastern Pennsylvania west of the Delaware River to examine the physical properties of the sediments and deposit. In their study area, they found loess at varying elevations, overlying different bedrock, residuum, and alluvium, with more than 95% of the deposit on landscapes with less than 8% slope. The loess deposit thinned from south to north, and the percent of very fine sand decreased from east to west away from the Delaware River. They concluded that the loess likely formed a continuous mantle at one time but had since been eroded from sloping landforms and that the sources of sediments for wind transportation were the Delaware River and Coastal Plain (Carey et al., Reference Carey, Cunningham and Williams1976). Little or no loess-specific work has been done in western Pennsylvania along the Allegheny River or in the state’s northern tier along the LGM glacial front.
Comparatively little loess research has been undertaken in Delaware and Virginia. Simonson (Reference Simonson1982) made the case for silty mantles as loess in Delaware, Maryland, and Virginia based on the landscape positions and elevations occupied by the deposits, the contrast between the silts and the underlying materials, and the proximity to loess in Pennsylvania and New Jersey. On the Piedmont of northern Virginia, Feldman et al. (Reference Feldman, Zelanzy, Pavich and Millard2000) identified a loess cap burying an upland paleosol that produced a thermoluminesence date of 13,800±1000 yr BP and noted the possibility of the Susquehanna River or Potomac River as the sediment source.
The most research into loess or loess deposits in the region has taken place in Maryland. In addition to Simonson’s (Reference Simonson1982) work noted previously, Rabenhorst et al. (Reference Rabenhorst, Foss and Fanning1982) identified a loess cap over serpentine residuum on the Maryland Piedmont, and Wright (Reference Wright1972) found loess over well-developed paleosols on the Coastal Plain west of the Chesapeake Bay. East of the Chesapeake Bay on the northern Delmarva Peninsula, Foss et al. (Reference Foss, Fanning, Miller and Wagner1978) sampled four transects across the loess deposit. They found that deposit thickness of the carbonate-free loess ranged from greater than 2 m to less than 50 cm, the deposit generally thinned with increasing distance from the Chesapeake Bay, and mean particle size generally decreased with distance from the bay. The surface horizon of a paleosol buried by the loess produced an uncalibrated bulk soil radiocarbon date of 10,520±240 yr BP. They concluded that the source of sediments was the ancestral channel of the Susquehanna River.
More recently, Wah (Reference Wah2003) characterized the physical, chemical, and mineralogical properties of loess with 39 sampling sites on the Delmarva Peninsula. Lowery et al. (Reference Lowery, O’Neal, Wah, Wagner and Stanford2010) and Wah et al. (Reference Wah, Wagner and Lowery2014) continued to refine the landscape evolution, the timing of loess deposition, paleoclimate, and the relationship between loess, landscape, and archaeological materials on the Delmarva Peninsula. General locations of these studies are shown in Figure 1.
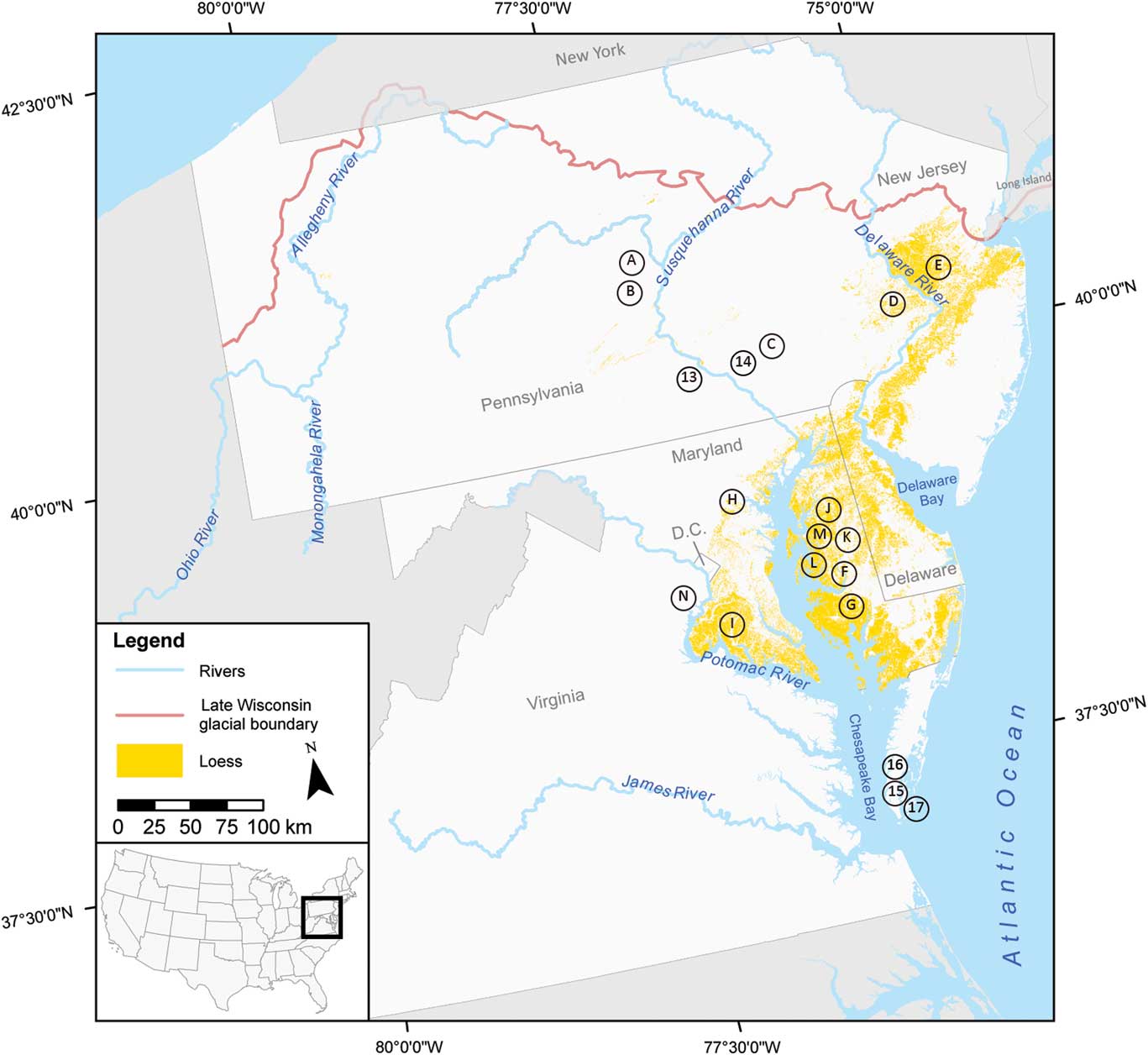
Figure 1 (color online) Distribution of loess in the mid-Atlantic region as mapped using the Natural Resources Conservation Service (NRCS) Gridded Soil Survey Geographic (gSSURGO) Database. Letters and numbers represent the general locations of previous studies and locations of sites discussed in this article. (A) Peltier (Reference Peltier1949). (B) Millette and Higbee (Reference Millette and Higbee1958). (C) Ciolkosz (Reference Ciolkosz2000). (D) Carey et al. (Reference Carey, Cunningham and Williams1976). (E) Tedrow and MacClintock (Reference Tedrow and MacClintock1953). (F) Perkins and Hershberger (Reference Perkins and Hershberger1929). (G) Matthews (Reference Matthews1963). (H) Rabenhorst et al. (Reference Rabenhorst, Foss and Fanning1982). (I) Wright (Reference Wright1972). (J) Foss et al. (Reference Foss, Fanning, Miller and Wagner1978). (K) Wah (Reference Wah2003). (L) Lowery et al. (Reference Lowery, O’Neal, Wah, Wagner and Stanford2010). (M) Wah et al. (Reference Wah, Wagner and Lowery2014). (N) Feldman et al. (Reference Feldman, Zelanzy, Pavich and Millard2000). (13) Goldsboro. (14) Lytle Farm. (15) Savage Neck (Rick et al., Reference Rick, Barber, Lowery, Wah and Madden2015). (16) Savage Neck (Lowery, Reference Lowery2016). (17) Mockhorn Island (Lowery and Stanford, Reference Lowery and Stanford2013).
MAPPING LOESS IN THE MID-ATLANTIC
The loess distribution map for the mid-Atlantic (Fig. 1) was created using the Natural Resources Conservation Service (NRCS) Gridded Soil Survey Geographic (gSSURGO) raster data for Pennsylvania, New Jersey, Maryland, Delaware, Virginia, and Washington, D.C. (U.S. Department of Agriculture, NRCS, Soil Survey Staff, 2016a, 2016b, 2016c, 2016d, 2016e, 2016f). Soil map units with “Parent Material Kind” of “Loess,” “Noncalcareous loess,” and “Eolian deposits” with “Parent Material Textural Modifier” of “Loamy” or finer were selected. The resulting map shows the broadest loess deposits in Maryland east of the Potomac River and the Chesapeake Bay and in New Jersey east of the Delaware River. Very little loess is mapped along Pennsylvania’s northern tier and the Susquehanna and Allegheny Rivers, and none is mapped in Washington, D.C., or Virginia.
As a function of the way soils were mapped or interpreted in the field and/or the way in which soil parent material information was entered into the gSSURGO Database by NRCS soil scientists, the loess distribution map overestimates the loess deposits in some areas and underestimates in others. The Maryland southern Delmarva Peninsula and the spine of the Delmarva Peninsula along the Maryland-Delaware border are two areas in which the loess deposit is overestimated. In contrast, loess in northern Pennsylvania and along the Susquehanna River is underrepresented. Unadilla series soils, noted as “formed in water or wind-deposited material that is high in coarse silt and very fine sand” in the NRCS Official Soil Series Description (U.S. Department of Agriculture, NRCS, Soil Survey Staff, 2017), are mapped for 3985 ha on Pennsylvania’s northern tier, along the Allegheny River in western Pennsylvania, the Susquehanna River in central Pennsylvania, and the Delaware River in eastern Pennsylvania and New Jersey but do not appear on the map generated by selecting loess or eolian parent materials in the gSSURGO Database. Strikingly, loess does not appear on the map in Washington, D.C., where loess is certainly present, and the loess deposit mapped on the southern Delmarva in Maryland ends abruptly at the Virginia state line. Querying the gSSURGO Database by series name using soil series that are known to be loessial ends with similar results—overrepresentation of loess deposits in some areas and underrepresentation in others. For Maryland, the search using “Parent Material Kind” resulted in a map with loess distribution more closely resembling what we observe in the field.
LOESS ON THE DELMARVA PENINSULA
The Delmarva Peninsula is bounded to the west by the Chesapeake Bay with the Delaware Bay to the northeast and the Atlantic Ocean to the east. The Coastal Plain landscapes are generally flat with slightly higher elevations and more rolling topography on the northern Delmarva Peninsula. Two thin loess deposits named by Lowery et al. (Reference Lowery, O’Neal, Wah, Wagner and Stanford2010) have been identified on the Delmarva Peninsula. The older loess, Miles Point Loess, is spatially limited, covering an area of approximately 540 km2, whereas the younger deposit, Paw Paw Loess, spreads over approximately 5000 km2 (Fig. 2). The Miles Point Loess is buried by the Paw Paw Loess, and in no location has the Miles Point Loess been observed at the modern surface.

Figure 2 (color online) Loess distribution on the Delmarva Peninsula, southern Maryland, and Washington, D.C., showing the approximate extent of the Miles Point Loess east of the Chesapeake Bay. Numbers give the general locations of sites discussed in this article. (1) Cators Cove. (2) Oyster Cove. (3) Blackwalnut Point. (4) Paw Paw Cove. (5) Miles Point. (6) Parsons Island. (7) Wye Island. (8) Chesapeake Farm. (9) Barnstable Farm. (10) Smithsonian Environmental Research Center (SERC). (11) Washington, D.C. (12) Patuxent. (13) Jefferson Island.
The older Miles Point Loess is present from Dorchester County in the south to Queen Anne’s County in the north on the western Delmarva Peninsula and has been tentatively identified as far north as Cecil County. In sampled sites, the deposit ranged from 63 to 118 cm thick. Particle size is dominated by fine particles with >56% silts (2–50 µm), little or no sand coarser than fine (0.25 mm), and no coarse fragments (>2 mm) (Wah, Reference Wah2003; Wah et al., Reference Wah, Wagner and Lowery2014). Mineralogically, the fine silt fraction is primarily quartz with trace amounts of mica and feldspar. Clay (<2 µm) mineralogy is vermiculite, mica, hydroxyl-interlayered vermiculite, and kaolinite with quartz and feldspar (Wah, Reference Wah2003).
The younger Paw Paw Loess is present across the peninsula in Maryland and Delaware. On the flatter landscapes of the southern Delmarva Peninsula, it forms a more continuous mantle, whereas to the north it is absent from sloping elements of the dissected terrain. Paw Paw Loess likely blanketed all of the western Delmarva Peninsula but was eroded when European colonization of the area resulted in land clearing and intensive agriculture. Along the Chesapeake, the Paw Paw Loess overlies the Miles Point Loess. The deposit is generally less than 2 m thick and exhibits broad thinning trends from the west to east and north to south. Exceptions to these trends occur south and east of confluences of larger rivers and of meander bends in the larger rivers.
The physical and mineralogical properties of the Paw Paw Loess are similar to those of the older, buried Miles Point Loess. Particle size is silt dominated with varying amounts of very fine and fine sands. As with the older loess, the mineralogy of the silts is quartz with lesser amounts of mica and feldspar, and the clay mineralogy is made up of vermiculite, mica, chlorite, hydroxyl-interlayered vermiculite, and kaolinite (Wah, Reference Wah2003).
DATING THE LOESS DEPOSITS
A number of 14C and optically stimulated luminescence (OSL) dates have been obtained for the loess deposits on the Delmarva Peninsula. 14C dates from the surface horizon of the Tilghman Paleosol range from 21,046 to 22,018 cal yr BP (17,820±170, AA-3807; dates calibrated using OxCal v. 4.3.2, IntCal13 atmospheric curve; Bronk Ramsey, Reference Bronk Ramsey2009; Reimer et al., Reference Reimer, Bard, Bayliss, Beck, Blackwell, Bronk Ramsey and Grootes2013) at Paw Paw Cove to 30,888–31,481 cal yr BP (27,240±230 14C yr BP, Beta-239558) at Miles Point (Lowery et al., Reference Lowery, O’Neal, Wah, Wagner and Stanford2010). OSL dates for the surface horizon of the Tilghman Paleosol at Miles Point are 27,940±1635 yr BP (UIC2020BL) and 29,485±1720 yr BP (UIC2019BL) with another date of 40,570±2670 yr BP (UIC2011BL) at the base of the Tilghman Paleosol and loess deposit above the contact with underlying sandy sediments (Lowery et al., Reference Lowery, O’Neal, Wah, Wagner and Stanford2010). Charcoal from the surface horizon of a sandy paleosol buried by the Paw Paw Loess at Wye Island returned a date of 20,113–21,067 cal yr BP (17,070±180 14C yr BP, Beta-165424) (Wah, Reference Wah2003). These OSL and 14C dates place the deposition of the Miles Point Loess during the Late Wisconsinan, Marine Oxygen Isotope Stage (MIS) 3 (approximately 60 ka BP to 27 ka BP) (Lowery et al., Reference Lowery, O’Neal, Wah, Wagner and Stanford2010). Dates from nine sites on the western Delmarva Peninsula and one site west of the Chesapeake Bay are included in Table 1. Radiocarbon dates for the eolian Parsonsburg Sand on the Delmarva Peninsula reported by Denny et al. (Reference Denny, Owens, Sirkin and Rubin1979) and calibrated by Markewich et al. (Reference Markewich, Litwin, Wysocki and Pavich2015) provide further evidence of eolian transportation and deposition of sediments during MIS 3 and MIS 2 (approximately 27 ka BP to 11 ka BP).
Table 1 14C and optically stimulated luminescence (OSL) dates for sites on the Delmarva Peninsula and west of the Chesapeake Bay.

a 95.4% probability, calibrated using OxCal v. 4.3.2, IntCal13 atmospheric curve (Bronk Ramsey, Reference Bronk Ramsey2009; Reimer et al., Reference Reimer, Bard, Bayliss, Beck, Blackwell, Bronk Ramsey and Grootes2013).
Archaeological materials help refine the timing of loess deposition. On the western Delmarva Peninsula, nondiagnostic pre-Clovis assemblages have been recovered within the Tilghman Paleosol at three sites including Miles Point, (Lowery et al., Reference Lowery, O’Neal, Wah, Wagner and Stanford2010), Parsons Island, (Lothrop et al., Reference Lothrop, Lowery, Spiess and Ellis2016), and Oyster Cove (Stanford and Bradley, Reference Stanford and Bradley2012). The true age of these assemblages has yet to be determined. In the same region, diagnostic Clovis artifacts from approximately 13 ka BP have been recovered from the top of the Tilghman Paleosol formed in the Miles Point Loess and buried by the Paw Paw Loess at multiple sites including Paw Paw Cove (Lowery et al., Reference Lowery, O’Neal, Wah, Wagner and Stanford2010), Jefferson Island (Stanford and Bradley, Reference Stanford and Bradley2012), and Savage Neck, Virginia (Lowery, Reference Lowery2016). Sixteen kilometers southeast of Savage Neck at Mockhorn Island, Virginia, Lowery and Stanford (Reference Lowery and Stanford2013) excavated Clovis artifacts from the surface of a paleosol buried by eolian sands. Early Archaic materials from approximately 11.5 ka BP are found in or just below the plowed surface of the modern soil formed in the Paw Paw Loess (Lowery et al., Reference Lowery, O’Neal, Wah, Wagner and Stanford2010). The presence of Clovis and that of Early Archaic materials constrain the deposition of the Paw Paw Loess to a relatively narrow time frame beginning shortly after 13 ka BP and coinciding with the Younger Dryas stade (Lowery et al., Reference Lowery, O’Neal, Wah, Wagner and Stanford2010). Figure 3 shows the loess deposits, dates, and cultural materials for five sites on the western Delmarva Peninsula.

Figure 3 (color online) Stratigraphic profiles for five sites on the western Delmarva Peninsula with soil horizons and depths, 14C and optically stimulated luminescence (OSL) sampling locations and ages, and cultural materials recovered from the soil profile. Oyster Cove (2), Blackwalnut Point (3), Paw Paw Cove (4), and Miles Point (5) all have the modern soil formed in the Paw Paw Loess overlying the Tilghman Paleosol formed in the Miles Point Loess with an unconformity at the contact of the two loess deposits. Wye Island (7) has the Paw Paw Loess overlying a sandy paleosol.
SOIL FORMATION IN THE LOESS
Soils formed in the two loess deposits are relatively strongly developed across the Delmarva Peninsula with argillic horizons recorded at all observations and fragipans at many. The Miles Point Loess was subject to pedogenesis throughout its deposition beginning approximately 40 ka BP and continuing until sometime after 20 ka BP and possibly as late as 13 ka BP. The Tilghman Paleosol formed in the Miles Point Loess has extremely coarse prismatic structure in the argillic horizons, and fragipans are described in all observations. The overlying Paw Paw Loess has been undergoing soil formation from approximately 13 ka BP to the present. The modern soil formed in the Paw Paw Loess, like the Tilghman Paleosol, is relatively well developed with argillic horizons with very coarse and coarse prismatic structure. Fragipans are generally present in the modern soil in areas in which it overlies the Tilghman Paleosol. Elsewhere on the Delmarva Peninsula, the modern soil formed in the Paw Paw Loess is less likely to have developed a fragipan. The relatively thin Paw Paw Loess deposit has allowed pedogenic processes to extend through the modern soil and into the Tilghman Paleosol post burial. Continued soil formation in the Tilghman Paleosol and “welding” of the modern soil and Tilghman Paleosol have resulted in a complex pedogenic history in which morphological and physical properties of the modern soil influence or overprint those of the Tilghman Paleosol. The surface horizon of the buried Tilghman Soil is strongly expressed in poorly and somewhat poorly drained settings but becomes less distinctive in well-drained environments where the organic carbon is more readily oxidized. On the western Delmarva Peninsula, the surface of the Tilghman Soil forms a prominent stratigraphic marker.
LANDSCAPE AND ENVIRONMENT
The sites on the western Delmarva Peninsula with Miles Point Loess buried by Paw Paw Loess are remnants of upland landscapes that have been and continue to be lost through sea-level rise and bank erosion. At the time of the Miles Point Loess deposition, sea level was approximately 30 m lower than present, and during the Younger Dryas and Paw Paw Loess deposition it was between 55 m (Oldale et al., Reference Oldale, Colman and Jones1991) and 36 m (Horton et al., Reference Horton, Peltier, Culver, Drummond, Engelhart, Kemp and Mallinson2009) lower. In addition to being much higher in elevation above sea level, these sites were horizontally removed from the water. Rather than being exposures on the Chesapeake Bay as they are now, Cators Cove was 7 km and Barnstable Farm was 11 km away from the Susquehanna River paleochannel.
Soils formed in loess record periods of landscape stability and episodes of erosion on the Delmarva Peninsula. The Tilghman Paleosol formed in the Miles Point Loess is indicative of an extended term of regional landscape stability and pedogenesis at the end of MIS 3 and through the LGM. In contrast to the LGM stability, the period leading up to and possibly including the Younger Dryas stade saw broad erosion of soils on the Delmarva Peninsula. In addition to the Tilghman Paleosol, the Paw Paw Loess overlies paleosols formed in sandy sediments with intact or partially intact surface horizons and truncated paleosols with surface horizons entirely eroded. Sandy paleosols likely developed during the Late Wisconsinan and LGM and are equivalent to the Tilghman Paleosol. The Tilghman Paleosol itself was affected by erosion with Clovis-age materials resting on a surface with consistently older 14C and OSL dates. The youngest date for the surface horizon of the Tilghman Paleosol is approximately 8 ka older than diagnostic Clovis projectile points resting at the unconformity. Broad landscape erosion prior to or coeval with the deposition of Paw Paw Loess shortly after 13 ka BP is also seen in the Chesapeake Bay. Deep cores show sediment filling of the Susquehanna River channel within the Chesapeake Bay with basal dates of 12,750 cal yr BP and 12,340 cal yr BP (Colman et al., Reference Colman, Bratton and Baucom2000). Colman et al. (Reference Colman, Bratton and Baucom2000) recorded 219 cm of sediment accumulated in the margin of the former Susquehanna River channel between 12,750 cal yr BP and 10,000 cal yr BP (core MD99-2204) and 890 cm of sediment between 12,340 cal yr BP and 10,130 cal yr BP center of the channel (core MD99-2207).
Loess distribution, deposit characteristics, and particle-size distribution within the loess suggest multiple sources for the parent silts. Certainly, the floodplains of the Susquehanna River acted as the main source of sediments with winds from the north and west redepositing silts across the uplands of the western Delmarva Peninsula. Some or all of the sediments on the Susquehanna floodplains may have been glacially derived and transported from southern New York and northern Pennsylvania for the older Miles Point Loess; however, by the time of Paw Paw Loess deposition, the Laurentide Ice Sheet had retreated clear of the Susquehanna River drainage basin (Ridge, Reference Ridge2003). Unusually thick deposits with increased sand contents in the Paw Paw Loess south and east of meander bends and confluences of rivers on the Delmarva Peninsula suggest local additions to sediment sources from materials eroded from the upland landscape and deposited on floodplains of the larger tributaries and along the Susquehanna. These eroded and redeposited sediments may be at least in part the older Miles Point Loess missing from the modern landscape. Loess on the western shore of the Chesapeake indicates that at some times winds transporting sediments were out of the east.
In addition to providing broad insight into landscape stability and erosion, loess on the Delmarva Peninsula yields specific information about past vegetation and, by extension, climate in the mid-Atlantic during the Late Pleistocene. Macroorganics, phytoliths, and pollen from the well-dated buried surface horizon of the Tilghman Paleosol have been recovered from Miles Point and Parsons Island. Paleobotanical records from these sites show a shift from mixed conifer-hardwood forest with yellow birch (Betula alleghaniensis), red spruce (Picea rubens), and balsam fir (Abies balsamea) (Lowery et al., Reference Lowery, O’Neal, Wah, Wagner and Stanford2010) with sedge (Carex) and C4 grasses (Puseman et al., Reference Puseman, Yost and Nurse2014) “to an open canopy forest dominated by cedar (Cedrus) and alder (Alnus), and with a lesser component of poplar (Populus), birch (Betula), and elm (Ulmus),” with decreases in sedges and C4 grasses and an increase in C3 grasses at the end of the LGM (Puseman et al., Reference Puseman, Yost and Nurse2014, p. 11).
Loess in the mid-Atlantic plays a critical role in understanding the early human history of North America. Multiple archaeological sites with cultural materials predating Clovis (13 ka BP) have been identified on the Delmarva (Lowery et al., Reference Lowery, O’Neal, Wah, Wagner and Stanford2010; Stanford and Bradley, Reference Stanford and Bradley2012; Lothrop et al., Reference Lothrop, Lowery, Spiess and Ellis2016). Pre-Clovis artifacts are within or below the surface horizon of the Tilghman Paleosol formed in buried Miles Point Loess. Clovis artifacts lie on or above the surface of the Tilghman Paleosol (Lowery et al., Reference Lowery, O’Neal, Wah, Wagner and Stanford2010). Erosion and truncation of the landscape prior to and/or during Paw Paw Loess deposition resulted in an unconformity at the contact of the Miles Point Loess and Paw Paw Loess. Clovis-age materials on the approximately 8 ka older surface of the Tilghman Paleosol are lag left behind when soils were eroded (Lowery et al., Reference Lowery, O’Neal, Wah, Wagner and Stanford2010). Early Archaic materials (11.5–11 ka BP) recovered from below and within the plowed surface horizon of the modern soil mark the end of Paw Paw Loess deposition (Lowery et al., Reference Lowery, O’Neal, Wah, Wagner and Stanford2010).
The landscapes and soils on the western Delmarva Peninsula are particularly favorable for early archaeological sites. The Paw Paw Loess caps and preserves the Late Pleistocene landscape on which pre-Clovis people lived and provides vertical separation between pre-Clovis and cultural materials from later time periods. The relative thinness of Paw Paw Loess benefits early archaeological sites by being thick enough to preserve the Late Pleistocene landscape, but not so thick that it prevents the identification of sites or excavation once a site has been identified. The clay mineralogy of the modern soil and the Tilghman Paleosol, particularly the absence of smectitic clays, is another aid to the preservation of pre-Clovis sites and their context. Archaeological sites with high expandable clay contents can be subject to mixing of cultural deposits and artifact movement down the soil profile. The landscape, vegetation, and climatic information preserved in the loess and the soil formed in the loess help reconstruct the environment that influenced the behavior of the people living there.
BEYOND THE DELMARVA PENINSULA
West of the Chesapeake Bay, expansive flat landscapes like those of the southern Delmarva are not nearly as common, but loess is almost always present wherever nearly level positions occur in proximity to potential sources. On the more uplifted and dissected Coastal Plain landscapes of southern Maryland southeast of Washington, D.C., loess is mainly restricted to narrow ridge crests. Presumably because of its susceptibility to erosion, flanking side slopes are entirely devoid of loess, and loess becomes quite sparse across the more rolling Piedmont topography farther west. As on the Delmarva Peninsula, loess may have at one time formed a continuous mantle west of the Chesapeake Bay that has since been largely eroded as a result of land clearing and agricultural practices. In Washington, D.C., loess covers virtually every nearly level, undisturbed landscape with deposit thickness ranging from 50 to 95 cm at elevations ranging from 6 m to 50 m above sea level. On the flat landscape at Patuxent, 97 cm of loess buries a paleosol with increased medium and fine sand contents. A silty paleosol buried by loess has been identified in limited areas along the Chesapeake Bay on the western shore; however, little work has been performed on the overlying loess or the paleosol. A bulk soil sample from the surface horizon of this buried paleosol at the Smithsonian Environmental Research Center (SERC) returned a date of 21,797–22,227 cal yr BP (18,140±60 14C yr BP, Beta-468060). Locations of these three sites are shown in Figure 2.
Deposit trends with distance from source area and particle size are similar to those on the Delmarva Peninsula. The floodplain of the ancestral Susquehanna River appears to have been a major source for western loess, but the Potomac River and other large tributaries likely provided additional significant contributions.
Soils formed in loess west of the Chesapeake demonstrate a level of pedogenesis similar to that of the modern soil formed in the Paw Paw Loess on the Delmarva Peninsula. Slightly weaker expression of argillic horizons in the loessial soils of southern Maryland and Washington, D.C., are likely the result of different loess compositions attributable to source areas and transportation distances. Whereas much of the loess on the Delmarva Peninsula was carried to distances of at least 10 km to more than 50 km from the floodplain of the ancestral Susquehanna River, more immediate proximities to the likely sources of the Potomac River floodplain and other large tributaries could account for somewhat coarser textures west of the Chesapeake Bay. As on the Delmarva Peninsula, paleosols underlying the loess were subjected to varying degrees of erosion and surface truncation prior to loess deposition; however, wholly intact buried soils are not uncommon. Unlike the somewhat poorly drained soils formed in loess on the Delmarva Peninsula, those west of the Chesapeake Bay tend to be moderately well to well drained. Preservation of organic matter in paleosol surfaces is therefore greatly diminished, and having lost most if not all of their original organic contents, the buried surfaces now exhibit lighter colorations more typical of eluvial (E) horizons.
Farther away from the Maryland Delmarva Peninsula, loess has been identified and soils formed in the loess have been described and sampled, but comparatively little focused research has been undertaken. On the southern tip of the Delmarva Peninsula in Virginia, a loess deposit with high amounts of very fine sands was identified during archaeological excavations of Holocene oyster shell middens (Rick et al., Reference Rick, Barber, Lowery, Wah and Madden2015). Soils formed in the loess had clay loam argillic horizons and from a pedogenic perspective were comparable to the modern soil formed in the Paw Paw Loess to the north in Maryland. In Pennsylvania, loess was identified on a narrow Pleistocene terrace 5 m above a tributary east of the Susquehanna River at Lytle Farm. Deposit thickness ranged from 49 to 130 cm with the landscape truncated prior to deposition and no buried surface preserved in the underlying alluvium. On the basis of pedogenesis, this deposit appears to be equivalent to the Late Pleistocene Paw Paw Loess. Similar pedogenesis was observed in 154 cm of loess atop a glacial outwash terrace 11 m above the east side of the Susquehanna River at Goldsboro in Pennsylvania. The underlying soil there had also been largely truncated. Locations of these sites are shown in Figure 1.
CONCLUSIONS
Loess deposits in the mid-Atlantic are best preserved on the broad, flat landscapes of the Delmarva Peninsula where the older, less extensive Miles Point Loess deposited during MIS 3 is buried by the younger Paw Paw Loess deposited during the Younger Dryas stade. Physically and mineralogically, the two deposits are similar with both being made up of predominantly silt-sized particles with very fine and fine sands and little or no coarse or very coarse sands or coarse fragments. Mineralogy of the fine silt fraction of the noncalcareous loesses is quartz dominated, and the clay mineralogy is mica, vermiculite, hydroxyl-interlayered vermiculite, and kaolinite. Pedogenesis of soils formed in the loess deposits is relatively strongly expressed with argillic horizons in all soils described and fragipans in many. The relatively thin Paw Paw Loess has allowed pedogenic processes to continue to act on the underlying Tilghman Paleosol formed in the Miles Point Loess and has resulted in welding of the modern soil and Tilghman Paleosol. The surface horizon of the Tilghman Paleosol forms a distinct stratigraphic marker on the western Delmarva Peninsula.
The loess on the Delmarva Peninsula has proved to be critical in addressing Late Pleistocene landscape evolution and environments. The well-dated Tilghman Paleosol formed in the Miles Point Loess points to general landscape stability during the Late Wisconsinan glacial maximum, whereas truncated soils buried by the Paw Paw Loess are indicative of periods of erosion approaching the end of the Pleistocene. Erosion of the terrestrial landscape supports other research in the region showing sediment filling of the Susquehanna River channel at this time, approximately 12.5 ka BP. The intact paleosol formed in the Miles Point Loess and stability during the LGM and erosion coincident with the Younger Dryas taken together suggest that rapid changes in temperature affected vegetation and destabilized the regional landscape rather than prolonged cold temperatures.
Mid-Atlantic loess makes an important contribution to the understanding of early regional and national human occupations of North America. Pre-Clovis cultural materials have been recovered from the within the surface horizon of the buried Tilghman Paleosol dating to the LGM, and Clovis-age artifacts lie on top of the truncated surface and are buried by the Paw Paw Loess.
Elsewhere in the mid-Atlantic, loess has been identified in southern Maryland and Washington, D.C.; in Virginia, particularly on the Virginia Delmarva Peninsula; in New Jersey east of the Delaware River; and in Pennsylvania along the northern tier and major rivers – the Allegheny, Susquehanna, and Delaware – draining glaciated areas. Loesses in these areas have received less attention but, based on the expression of pedogenesis of soils formed in them, are thought to be the equivalent of the Paw Paw Loess from the Delmarva Peninsula in Maryland.
The extent of loess deposits in the mid-Atlantic is not entirely clear. Mapping created using the NRCS gSSURGO Database and selecting parent material overestimates the loess deposits in some areas and underestimates in others. The Maryland southern Delmarva and the spine of the Delmarva along the Maryland-Delaware border are two areas in which the loess has been overestimated. Very little loess is mapped along the Pennsylvania northern tier or along the Susquehanna River, and no loess is shown in Washington, D.C., or in Virginia.
ACKNOWLEDGMENTS
We thank Dr. Dennis Stanford and Dr. Torben Rick from the Department of Anthropology, National Museum of Natural History, Smithsonian Institution for continued support of soils and landscape research in the mid-Atlantic and particularly for assistance with dating deposits; Joshua Barth of Shippensburg University for geographic information system analysis and mapping; and Dr. Martin Rabenhorst, University of Maryland, for initiating this project.