Introduction
Oryzaephilus mercator and Oryzaephilus surinamensis (Coleoptera; Silvanidae) species attack already infested or malformed grains, preferring cereal products and oleaginous seeds. The beetle O. surinamensis is known worldwide as the greatest pest of stored products. However, according to Athié and Paula (Reference Athié and Paula2002), O. mercator is not considered a major plague of stored grains in Brazil.
Esterases are involved in numerous important biological processes in insects, some of them are already well known. Various works have demonstrated that certain esterases play an important role in juvenile hormone (JH) levels control (Yamamoto et al., Reference Yamamoto, Chadarevian and Pellegrini1988 for Drosophila). Some are present in the male reproductive system of many insect species, being important to the reproductive health of males (Mikhailov & Torrado, Reference Mikhailov and Torrado2000). Others seem to work in digestive processes (Healy et al., Reference Healy, Dumancic and Oakeshott1991 for Drosophila) and are also involved in insecticide resistance, as in O. surinamensis (Lee & Lees, Reference Lee and Lees2001; Rossiter et al., Reference Rossiter, Gunning and Rose2001a, Reference Rossiter, Conyers, MacNicoll and Roseb) and in Aedes aegypti (Bisset Lazcano et al., Reference Bisset Lazcano, Rodríguez, San Martín, Romero and Montoya2009).
Esterase isoenzymatic systems are constituted of four classes of enzymes: carboxylesterases (EC 3.1.1.1); cholinesterases, including acetylcholinesterases (EC 3.1.1.7) and pseudocholinesterases (EC 3.1.1.8); acetylesterases (EC 3.1.1.6); and arylesterases (EC 3.1.1.2). Each class has been defined for its specificity to the substrate, its sensibility to different types of inhibitors and for its amino acid residues in the active site (Oakeshott et al., Reference Oakeshot, van Papenrecht, Boyce, Healy and Russell1993).
The role played by esterases in insecticide resistance mechanisms have been broadly studied in insects, showing its fundamental importance. Two classes of esterases are involved in this process, cholinesterases and carboxylesterases. Among cholinesterases, acetylcholinesterase regulates the levels of acetylcholine in nerve synapses.
Studies have demonstrated insects may acquire insensitivity to insecticides due to point mutations in genes coding for acetylcholinesterases; the amino acid replacement in the enzyme active site was found in populations of Drosophila melanogaster (Menozzi et al., Reference Menozzi, Shi, Lougarre, Tang and Fournier2004). In Rhyzopertha dominica (Coleoptera: Bostrichidae), the acetylcholinesterase from resistant populations was less sensitive to inhibition by malaoxon than that from the susceptible, suggesting that qualitative and quantitative changes in this enzyme should contribute to resistance organophosphates (Guedes et al., Reference Guedes, Kambhampati, Dover and Zhu1997).
Resistance to insecticides containing an ester bond may occur by metabolic detoxification through the hydrolysis of carboxylic esters, or it may be due to insecticide sequestration (Wheelock et al., Reference Wheelock, Shan and Ottea2005). These mechanisms lead to a considerable increase of this enzymatic activity in resistant organisms, such as the MCE (Malathion-specific carboxylesterase) in resistant strains of Anisopteromalus calandrae (Baker et al., Reference Baker, Fabrick and Zhu1998) and Tribolium castaneum insects (Haubruge et al., Reference Haubruge, Amichot, Cuany, Berge and Arnaud2002). In contrast, Lucilia cuprina shows a decrease in carboxilesterase activity in organophosphate resistant strains. This has been explained by a mutant ali-esterase hypothesis. This hypothesis proposes that a structural mutation of a carboxylesterase results in a reduced ability to hydrolyse carboxilesterase substrates, such as methyl butyrate and naphthyl acetate esters, but an acquired ability to hydrolyse organophosphate substrates (Hughes & Raftos, Reference Hughes and Raftos1985).
Rossiter et al. (Reference Rossiter, Gunning and Rose2001a) verified two highly resistant strains of O. surinamensis to chlorpyrifos-methyl, showing differences in the resistance to fenitrothion, in which esterases are strongly involved in the primary resistance mechanism to chlorpyrifos-methyl and possibly in the resistance to fenitrothion. In this same study, experiments using polyacrylamide gel electrophoresis showed quantitative differences in esterase activity levels, verified by the presence of strongly coloured bands on the gels of these two highly resistance strains, and also, qualitative differences in esterases among themselves, observed by their relative mobility. These were later classified as β-esterases, and qualitative differences among them were proposed as an explanation for sensitivity and resistance variation to fenitrothion among lineages (Rossiter et al., Reference Rossiter, Conyers, MacNicoll and Rose2001b).
Another study, involving the resistance to insecticides in O. surinamensis strains, showed that among the tested enzymatic systems, esterases were the highest activity isoenzymes, being responsible for the greater resistance to organophosphates. The acetylcholinesterase involved in this resistance has been purified and characterized (Conyers et al., Reference Conyers, MacNicoll and Price1998). Lee et al. (Reference Lee, Lees and Campbell2000) observed that a hydrolytic activity of esterases were positively related with an increase of the O. surinamensis strains resistance to fenitrothion organophosphates, and the zymograms showed differences in band patterns between resistant and susceptible strains. Functional characterization and genetic analysis of this isoenzymatic system in O. surinamensis and O. mercator have been presented in this study. It has also shown a possible relation between sensitivity variability of these enzymes against organophosphates and tolerance variation to these compounds in different lineages.
Material and methods
Maintenance of O. mercator and O. surinamensis strains in laboratory
In order to perform this study, adults of four strains of O. mercator (OmLA, OmLB, OmLC and OmLC-M) and two strains of O. surinamensis (OsLA and OsLB) have been used. They were obtained in dwellings (OmLA), pet shops (OmLB, OmLC) and grain stores (OsLA, OsLB) within the county of Maringá, Paraná. OmLC-M strain was derived from the OmLC strain, which was exposed to malathion at a concentration of 0.05 μg g−1 for eight months.
Insects were kept in containers, in a temperature of 30°C±2 and controlled humidity of 60±10% RH, with a normal diet composed of animal rations and previously crushed sunflower seeds.
Determining LC50
Lethal concentration, which kills 50% of the insects (LC50), was calculated for the strains for each insecticide: the organophosphates malathion and chlorpyrifos-methyl. Tests were performed in culture containers enclosing the tested insecticide as well as 50 g of normal diet. Concentration was estimated in micrograms of insecticide per gram of culture medium (μg g−1).
Technical grade samples of the insecticide Malathion 500-CE were provided by Dipil Chemistry Industry (Rodovia Guilherme Jensen, Massaranduba, SC, Brazil) and LORSBAN 480 BR (chlorpyrifos-methyl) was obtained by Dow Chemical (Dow Sudeste Industrial Ltd, São José dos Pinhais Pinhais, PR, Brazil). Different organophosphates concentrations were tested. For malathion, concentrations between 0.05 μg g−1 and 1.1 μg g−1 were used; and, for chlorpyrifos-methyl, concentrations used were between 0.01 μg g−1 and 0.48 μg g−1. Specific concentrations were prepared using water as prescribed for each product.
For each bioassay, 25 adult insects, unsexed and with no definite age, were put in glass containers which were sealed with voile tissue and elastic bands to avoid escape. Insects remained exposed to treatments for 24 h, when mortality was assessed. Survivors were frozen and later esterases were examined by gel electrophoresis. Bioassays were replicated 2–4 times.
LC50 was estimated using JSPEAR software, through the Sperman-Kaber method modified by Hamilton et al. (Reference Hamilton, Russo and Thurston1977). The resistance factor (RF) was obtained through the rate between the most resistant population LC50 and the most susceptible population LC50 of each species (Ceruti & Lázzari, Reference Ceruti and Lázzari2003).
Identifying the esterases
Polyacrylamide gel electrophoresis (PAGE) technique (Davis, Reference Davis1964) was employed in this work. PAGE was performed in a vertical gel in a discontinuous buffer system, using 11% (acrylamide/bis-acrylamide solution) in resolution gels and 4% in stacking gels.
Insect samples were homogenised individually at 0°C in 25 μl of Tris-HCl 1.5 M pH 8.8 buffer for O. mercator and in 30 μl for O. surinamensis, containing glycerol at 10%. Some 260 O. mercator and 160 O. surinamensis were analysed. Twelve specimens of each species and of each development stage, last larval stage, pupae and adults were used for esterase pattern identification during insect development. Electrophoresis was performed for five hours, at a constant voltage of 200 V. The running buffer was Tris-glycine 0.1 M pH 8.3. After electrophoresis, in order to lower the pH, gels were pre-incubated in 50 ml of 0.1 M pH 6.2 phosphate buffer. After 30 min, the buffer was taken out and the staining solution was added. Staining solution was prepared with 50 ml of sodium phosphate solution 0.1 M pH 6.2, n-propanol (5 ml), Fast Blue RR Salt (Acros Organics, NJ, USA) (0.06 g) and, α-naphthyl acetate (0.03 g) and β-naphthyl acetate (0.02 g) as substrates, previously solubilised in acetone (1 ml). After about an hour of incubation, in the dark at room temperature, esterases were visualised in gels as black or red bands, indicating the presence of α e β-esterases, respectively.
Specificity to the substrate
In order to cluster bands with similar affinity to α and β-naphthyl acetate substrates and to contribute with genetic analysis, gels were separately incubated with: (i) 0.05 g of α-naphthyl acetate; (ii) 0.05 g of β-naphthyl acetate; (iii) 0.03 g of α-naphthyl acetate and 0.02 g of β-naphthyl acetate.
Treatment with inhibitors for the biochemical characterization of the esterases
Technical grade inhibitors malathion 500-CE and carbaryl were provided by Dipil Chemistry Industry and Lamy Chemical Ltda (Rua Geraldo Burck, SP, Brazil), respectively. Technical grade inhibitors iodoacetamide, eserine sulphate, phenylmethylsulphonyl fluoride and p-chloromercuribenzoate were obtained from Sigma Chemical Co. (Aldrich Chemie, Steinheim, Germany). These esterase inhibitors were the key factor for functional characterization and genetic analysis (Healy et al., Reference Healy, Dumancic and Oakeshott1991).
Tests for esterases biochemical characterization involved the use of the inhibitors eserine sulphate at 0.1 mM; malathion at 0.3 mM; phenylmethylsulphonyl fluoride (PMSF) at 10 mM for O. mercator and 7.8 mM for O. surinamensis; iodoacetamide (IAC) at 10 mM; p-chloromercuribenzoate (pCMB) at 1.5 mM and carbaryl at 0.0745 mM. In these tests, gels were pre-incubated for one hour in 50 ml of phosphate buffers 0.1 M pH 6.2 containing one of the inhibitors and then treated with staining solution also containing the inhibitor. PMSF was previously dissolved in 1 ml of methanol and added to the incubation buffer. Control gels were prepared simultaneously to treated gels with the same samples.
Results
Study of the esterase patterns in Oryzaephilus mercator
The analysis of esterase patterns in about 260 individuals of OmLA, OmLB and OmLC strains has indicated the presence of 15 enzymes of monomeric structure (table 1). These were identified and clustered in seven groups based on the affinity to the substrate, sensitivity to the inhibitors and migration in electrophoresis gels. The enzymes were considered products of the expression of seven loci, all of which were considered polymorphic, since they presented more than one band (fig. 1).
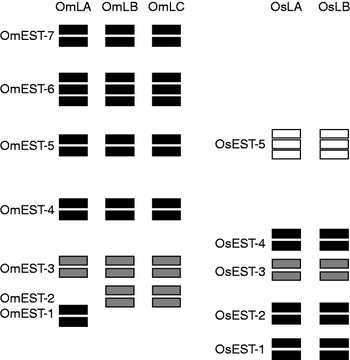
Fig. 1. Comparative zimogram of the esterase pattern in adults of Oryzaephilus mercator and Oryzaephilus surinamensis (, α-Esterase;
, β-Esterase;
, α,β-Esterase).
Table 1. Esterase characterization in Oryzaephilus mercator and Oryzaephilus surinamensis.
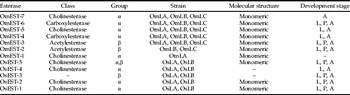
OmEST, esterase in Oryzaephilus mercator; OsEST, esterase in Oryzaephilus surinamensis;
α, α-esterase; β, β-esterase; –, undefined; L, larvae; P, pulp; A, adult.
The analysis of the esterase pattern during insect development has indicated that OmEST-7 is present exclusively in adults, OmEST-4 and OmEST-5 were only detected in larvae and adults while OmEST-2, OmEST-3 and OmEST-6 are present in every stage of development (table 1). OmEST-1 was present only in the OmLA strain, and OmEST-2 was absent in this strain. The other esterases were present in the three analysed strains, OmLA, OmLB and OmLC (fig. 2).
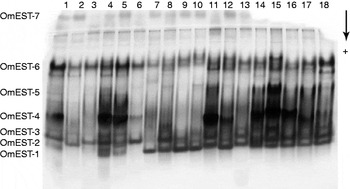
Fig. 2. Esterases in adults of three Oryzaephilus mercator strains. Samples 1–6, OmLA; 7–12, OmLB; and 13–18, OmLC.
Study of the esterase patterns in O. surinamensis
Approximately 160 specimens from the two O. surinamensis strains (OsLA and OsLB) were analysed regarding their esterase pattern. The 11 enzymes observed in this species were considered as products of five loci (fig. 1) which were found in larvae and adult stages of development. However, at the pupa stage, OsEST-4 was absent and these five loci, OsEST-1, OsEST-2 and OsEST-5 were classified as monomeric (table 1).
Esterase characterization in O. mercator and O. surinamensis
Esterases were characterized regarding their specificity to the substrates α-naphthyl acetate and β-naphthyl acetate. OmEST-1, OmEST-4, OmEST-5, OmEST-6 and OmEST-7 are enzymes which preferably hydrolysed α-naphthyl acetate; OmEST-1 hydrolysed exclusively α-naphthyl acetate substrates, which were considered α-esterases; and OmEST-2 and OmEST-3 hydrolysed exclusively β-naphthyl substrates, being considered β-esterases (fig. 1). Substrate affinity was the same for the three strains of O. mercator.
In two strains of O. surinamensis, the esterases OsEST-1, OsEST-2 and OsEST-4 were characterized as α-esterases (preferably hydrolysing α-naphtyl acetate) and OsEST-3 as β-esterases (preferably hydrolysing β-naphtyl acetate). OsEST-5 presented affinity to both substrates, being characterized as an α/β-esterase (fig. 1).
Malathion, eserine sulphate, carbaryl, p-chloromercuribenzoate (pCMB), phenylmethylsulphonyl fluoride (PMSF) and iodoacetamide (IAC) were tested in order to characterize the inhibition pattern and to verify a possible homology among enzymes of the two species. Except for PMSF and IAC, which indicated the presence of serine and cysteine residues, respectively, the inhibitors enabled esterase classification as carboxylesterases, acetylesterases, cholinesterases and arylesterases (Oakeshot et al., Reference Oakeshot, van Papenrecht, Boyce, Healy and Russell1993). OmEST-1, OmEST-5 and OmEST-7 esterases, which only differed in the inhibition by malathion, were identified as cholinesterases. OmEST-4 and OmEST-6 were classified as carboxylesterases, due to their inhibition by malathion and not by eserine. OmEST-2 and OmEST-3 were characterized as acetylesterases, since they were not inhibited by any inhibitor. The iodoacetamide inhibitor has not altered esterase activity, whilst the PMSF has inhibited every esterase, except OmEST-1. This inhibition pattern was observed in the three O. mercator strains, OmLA, OmLB and OmLC (table 2).
Table 2. Esterase classification in adult insects exposed to different inhibitor classes.
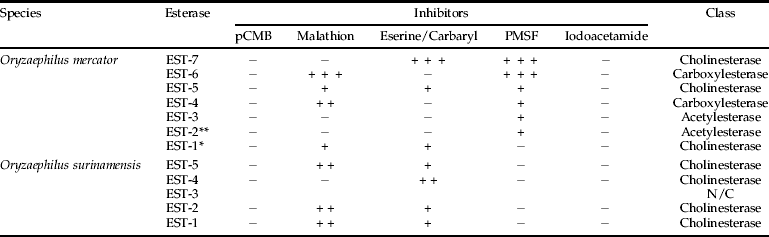
N/C, not classified; +, ++, +++, inhibition degrees; –, not inhibited;
*, esterase present in OmLA strain;
**, esterase absent in OmLA strain.
Oryzaephilus surinamensis enzymes were not inhibited by p-chloromercuribenzoate (pCMB), phenylmethylsulphonyl (PMSF) and iodoacetamide (IAC) inhibitors; esterase isozymes were inhibited by eserine and malathion, except OsEST-4, which was not inhibited by malathion. Therefore, all O. surinamensis esterases were classified as cholinesterases (table 2). The same inhibition pattern has been observed in both lineages of O. surinamensis.
A comparative analysis of the esterases of both species, considering migration in electrophoresis gel (figs 2 and 3), the pattern of inhibition and affinity to substrates (table 1) showed homology of OmEST-1 and OsEST-2 (cholinesterases, α-esterases and monomeric structures) and between OmEST-3 and OsEST-3 (β-esterases), migrating to the same portion on the electrophoresis gel.
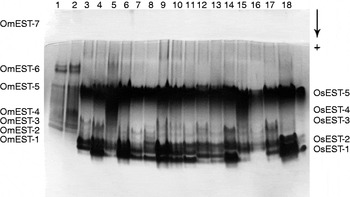
Fig. 3. Comparison between the esterases of adults of Oryzaephilus mercator and Oryzaephilus surinamensis strains. Samples 1 and 2, OmLB of O. Mercator; 3–10, OsLB; and 11–18, OsLA of O. surinamensis.
Study of the toxicity of organophosphates malathion and chlorpyrifos-methyl LC50 analysis in O. mercator and O. surinamensis
Bioassays using malathion in O. mercator strains have shown that among field strains, which were collected and kept in the laboratory without any sort of treatment, OmLA (LC50=0.1295 μg g−1) was the most resistant and OmLB (LC50=0.0617 μg g−1) the least resistant. The OmLC-M derived strain (LC50=0.2687 μg g−1) presented a resistance factor (RF) of 2.78 in relation to its original strain, OmLC. Similarly, bioassay results regarding chlorpyrifos-methyl has shown that OmLA (LC50=0.1269 μg g−1) was the most resistant and OmLB (LC50=0.0342 μg g−1) the least resistant. The RF of OmLA was 3.71, considering that OmLB was the most susceptible strain (table 3).
Table 3. LC50 values (μg/g) and the resistance factor for adults of Oryzaephilus mercator and Oryzaephilus surinamensis.

*, lethal concentration 50% (LC50) and confidence interval (CI);
n, number of analyzed insects;
RF, resistance factor;
**, non-exposed insects;
μg g−1, micrograms of the insecticide per gram of culture medium.
Bioassays concerning malathion and chlorpyrifos-methyl with O. surinamensis has shown that OsLB strain regarding both insecticides presented a higher LC50 than OsLA (table 3) but presented a more evident difference in the resistance to malathion, in which the LC50 of OsLB was 0.9484 μg g−1 and the LC50 of OsLA was 0.5877 μg g−1, with an RF of 1.61.
A comparison between the two species shows that O. surinamensis strains present a high rate of LC50 in relation to the most resistant O. mercator strains. The most resistant field O. mercator strain, OmLA, presented a LC50 of 0.1295 μg g−1 for malathion and 0.1269 μg g−1 for chlorpyrifos-methyl, while in the most resistant O. surinamensis strain, OsLB presented a LC50 of 0.9484 μg g−1 for malathion and 0.3888 μg g−1 for chlorpyrifos-methyl.
Analysis of the O. mercator esterases treated with malathion and chlorpyrifos-methyl
Esterase analysis in insects exposed to malathion (table 4) has indicated sensibility variations among strains. OmEST-1, present exclusively in the OmLA strain, was fully inhibited in exposed insects to 0.075 μg g−1 and 0.1 μg g−1 when the bioassays were performed using malathion. However, OmEST-2, absent exclusively in OmLA, was partially inhibited with 0.1 μg g−1 and 0.2 μg g−1 in OmLC-M. OmEST-3, present in all strains, was fully inhibited with malathion concentrations of 0.075 μg g−1 and 0.1 μg g−1 in OmLA and partially inhibited with 0.1 μg g−1 and 0.2 μg g−1 concentrations in OmLC-M (fig. 4). Meanwhile, in the most sensitive strains to malathion, OmEST-3 was not inhibited. This higher sensitivity of OmEST-3 in OmLA can also be observed in insects exposed to chlorpyrifos-methyl (table 5). OmEST-4, present in all strains, was inhibited only in OmLA, in insects exposed to 0.072 μg g−1 and 0.12 μg g−1 of chlorpyrifos-methyl.
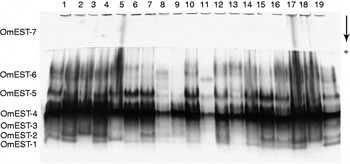
Fig. 4. Esterase behaviour in adults of Oryzaephilus mercator in OmLC-M strain, exposed to malathion. Sample 1–4, control (insects unexposed to malathion); 5–9, insects exposed to 0.1 μg g−1 (micrograms of the insecticide per gram of culture medium); 10–14, insects exposed to 0.2 μg g−1; and 15–19, insects exposed to 0.3 μg g−1.
Table 4. Esterase behaviour in Oryzaephilus mercator exposed to malathion.

+,++,+++, inhibition degrees; –, not inhibited;
*, esterase present in OmLA strain;
**, esterase absent in OmLA strain.
Table 5. Esterase behaviour in Oryzaephilus mercator exposed to chlorpyrifos-methyl.

+,++,+++, inhibition degrees; –, not inhibited;
*, esterase present in OmLA strain;
**, esterase absent in OmLA strain.
Analysis of the O. surinamensis esterases treated with malathion and chlorpyrifos-methyl
In the bioassays using malathion at 0.3 μg g−1 and 0.4 μg g−1, a partial inhibition of OsEST-1, OsEST-2 and OsEST-3 enzymes exclusively in the OsLB strain was observed. Under concentration of 0.8 μg g−1, a partial inhibition occurred for the same esterases in OsLA (table 6).
Table 6. Esterase behaviour in Oryzaephilus surinamensis exposed to malathion.

+,++,+++, inhibition degrees; –, not inhibited.
Esterase patterns in insects exposed to chlorpyrifos-methyl has not presented any significant variation when compared to esterase patterns of unexposed insects.
Discussion
Oryzaephilus surinamensis and O. mercator are very similar morphologically, being quite hard to differentiate. In the present study, these species were initially identified by head morphology. Esterase patterns using polyacrylamide gel electrophoresis (PAGE) has been used for the characterization of Drosophila species (Lapenta et al., Reference Lapenta, Bicudo, Ceron and Cordeiro1998; Bernardo & Bicudo, Reference Bernardo and Bicudo2009). In the case of O. mercator and O. surinamensis, esterase patterns permitted a clear species differentiation. In O. mercator and O. surinamensis, there were identified 15 and 11 esterases as products of seven and five loci, respectively. Just OmEST-1 and OsEST-2, and OmEST-3 and OsEST-3 seem to be homologous between both species.
The esterase study of both Oryzaephilus species development indicated four developing stages specific esterases. OmEST-4, OmEST-5 and OsEST-4 were present exclusively in the larvae and adult stages and OmEST-7 occurs only in the adult stage. The other enzymes were present in all stages. Differential expression of esterase genes may reflect the diversity of functions performed by these enzymes, and it has been detected in many different insects.
The functional characterization of esterases, according to the criteria used by Healy et al. (Reference Healy, Dumancic and Oakeshott1991), has permitted the identification of two acetylesterases, two carboxylesterases and three cholinesterases in O. mercator, and four cholinesterases in O. surinamensis. In the present study, cholinesterases were characterized by eserine sulphate sensitivity, as broadly used in insect acetylcholinesterases identification. The high number of cholinesterases found in both beetle species may indicate that, in addition to cholinergic acetylcholinesterases that act in the central nervous system, non cholinergic esterases that act in digestive and/or detoxification processes may also occur, as already demonstrated in D. melanogaster (Healy et al., Reference Healy, Dumancic and Oakeshott1991; Campbell et al., Reference Campbell, Robin, Court, Dorrian, Russell and Oakeshott2003).
In contrast, carboxylesterases are the most frequent esterases for many insect species. This can be observed in D. melanogaster (Healy et al., Reference Healy, Dumancic and Oakeshott1991), where ten esterase enzymes were classified as carboxylesterases, six as cholinesterases and six as acetylesterases. In A. aegypti, 12 of 23 esterases were classified as carboxylesterases and only three were cholinesterases (Lima-Catelani et al., Reference Lima-Catelani, Ceron and Bicudo2004).
The amino acid sequence in the enzyme active site has been an additional criterion for esterase classification, especially in mammals. Carboxylesterases and cholinesterases present an octapeptide consensus sequence (Chatonnet & Lockridge, Reference Chatonnet and Lockridge1989), and these are considered members of a multigene family of the cholinesterases/carboxylesterases (Oakeshott et al., Reference Oakeshot, van Papenrecht, Boyce, Healy and Russell1993). Carboxylesterases, cholinesterases and possibly acetylesterases contain a serine residue in their active site. However, arylesterases, which are sensitive to sulfhydrilic reagents, presents a cysteine residue instead of serine (Oakeshott et al., Reference Oakeshot, van Papenrecht, Boyce, Healy and Russell1993).
The inhibitor PMSF is an indicator of serine residue in the active site (Sekar & Hageman, Reference Sekar and Hageman1979). In our study, OmEST-2, OmEST-3, OmEST-4, OmEST-5, OmEST-6 and OmEST-7 were fully or partially inhibited by this compound. It is possible that in the other cholinesterases/carboxylesterases found, this serine residue present in the active site may not be accessible to PMSF.
LC50 analysis is an important tool in pest insect resistance studies, being useful for resistance comparative analysis. LC50 obtained regarding malathion and chlorpyrifos-methyl for O. mercator strains, has shown differences in the sensibility to these compounds even though those strains have been collected in the same city. A cross resistance to both organophosphates has been observed; once that among field strains, the OmLA has shown greater resistance to malathion and also to chlorpyrifos-methyl. In O. surinamensis, OsLB has presented greater resistance when compared to OsLA for malathion; but, when exposed to chlorpyrifos-methyl, they have shown similar sensitivity. Likewise, Lee et al. (Reference Lee, Lees and Campbell2000) has verified the existence of cross resistance among chlorpyrifos-methyl, fenitrothion and malathion insecticides in three O. surinamensis strains. These authors showed that the same strain was the most resistant to the three insecticides, and the greatest sensitivity has also been verified in a single strain.
For O. mercator OmLC-M strain, previously exposed to malathion, a RF has been obtained of 2.8 in relation to its original strain, OmLC, and a RF of 4.35 in relation to the most susceptible strain. Despite the small differences in the resistance factors observed, OmLC-M may be considered the most resistant strain, highlighting the role of malathion as a selective agent.
Among the three enzymatic systems (cytochrome p450, glutathione S-transferases and esterase) studied by Conyers et al. (Reference Conyers, MacNicoll and Price1998), in O. surinamensis, esterases are mostly related to detoxification and insensitivity mechanisms to insecticides, due to its ability to hydrolyse ester bonds. Once the organophosphates, carbamates and pyrethroids have ester bonds, they can be detoxified by this bond's hydrolysis (Lee et al., Reference Lee, Lees and Campbell2000).
In our study, esterase analysis of insects exposed to insecticides has shown a differential enzyme response, even though there was a similar esterase pattern among strains of the same species. The most resistant O. mercator and O. surinamensis strains have presented more sensitive enzymes to organophosphates. For many insect species, the important role of esterases in the detoxification of insecticides has already been demonstrated, including for O. surinamensis (Lee et al., Reference Lee, Lees and Campbell2000; Rossiter et al., Reference Rossiter, Gunning and Rose2001a). It is possible that this differential sensitivity is responsible for at least part of the sensitivity difference to these compounds presented among strains.
In O. mercator, the most resistant field strain, OmLA, has also presented an additional esterase, the cholinesterase OmEST-1, which was sensitive when these insects were exposed to different concentrations of malathion and chlorpyrifos-methyl. Although cholinesterases are confined to the central nervous system, cholinesterases with non-cholinergic functions have already been described in non-neural tissue (Chatonnet & Lockridge, Reference Chatonnet and Lockridge1989). Therefore, it is possible that OmEST-1 is a non-cholinergic cholinesterase and that it plays a role in this greater resistance. Non-cholinergic cholinesterases have already been verified in other species, such as the EST-9 in D. melanogaster (Healy et al., Reference Healy, Dumancic and Oakeshott1991) and the EST-2 in Triatoma infestans (Tavares et al., Reference Tavares, Azeredo-Oliveira and Ceron1998). In addition to OmEST-1, OmLA presented a higher inhibition pattern of OmEST-3 and OmEST-4 exposed to malathion and chlorpyrifos-methyl in relation to the most sensitive strains. The inhibition pattern for OmEST-3 exposed to malation was also seen in the most resistant strain OmLC-M.
Oryzaephilus surinamensis presented greater sensitivity than O. mercator, for both malathion and chlorpyrifos-methyl. One possible explanation, to be studied, is the participation of OsEST-5, the cholinesterase which strongly stained the polyacrylamide gels, indicating its abundance in O. surinamensis. The presence of strongly stained bands in O. surinamensis have also been observed by Rossiter et al. (Reference Rossiter, Gunning and Rose2001a). These authors, using the PAGE system, have observed high esterase activity in O. surinamensis strains resistant to chlorpyrifos-methyl; this high activity has produced thick and intensely stained bands on the gels. According to these results, Rossiter et al. (Reference Rossiter, Gunning and Rose2001a) has suggested that the PAGE system may be used for the rapid detection of the resistance to chlorpyrifos-methyl, as this resistance is diagnosed by thick intensely stained bands when compared to the standard bands of insects with known resistances. Besides OsEST-5, which may be involved with greater resistance of O. surinamensis, OsEST-2 is homologous to OmEST-1, present only in the most resistant strain of O. mercator.
The difference in response of these two species to insecticides may reflect the evolution of differentiated defence mechanisms among them, probably due to a history of previously differentiated exposal to insecticides, varying in kind and quantity of applied insecticides.
Although O. mercator is not labelled as one of the major pest species in Brazil (Athié & Paula, Reference Athié and Paula2002), specimens were easily found infesting processed products in the country of Maringá, PR, Brazil; there was no difficulty collecting these insects for the present study. On the other hand, O. surinamensis is considered a major pest and responsible for considerable economical loss (Athié & Paula, Reference Athié and Paula2002).
Our results indicate that the variability in the ability to respond to different types of organophosphates, among the different studied lineages of this species, may be related to the variability of the differential sensibility of the esterases.