Since its first description in 1988,Reference Zwicky, Daniel, Mugge and Lichtlen1 intraoperative transoesophageal echocardiography has become the standard of care in many surgical centres during congenital or acquired heart disease surgery. The primary role of transoesophageal echocardiography in a perioperative setting is anatomical evaluation of the cardiac defect with exclusion of additional defects, and assessment of the adequacy of repair post-bypass to detect residual lesions to be addressed in the operating room. Intraoperative transoesophageal echocardiography adds pre-operative information over the transthoracic study if anatomical clarification is required, influences surgical decision-making, and guides surgical strategies across a range of cardiac procedures.Reference Ayres, Miller-Hance and Fyfe2–Reference Gurbuz, Hecht and Arslan4 Epicardial echocardiography is used as an adjunct to visualise the post-operative surgical repair, especially in cases where transoesophageal views are limited.Reference Dragulescu, Golding and Van Arsdell5
An application of transoesophageal echocardiography that is less widely described is its utility in evaluating coronary artery patency and flow following cardiac surgery. Of the congenital cardiac lesions significant enough to require surgery, a number of important conditions involve intraoperative manipulation or re-implantation of the coronary arteries, which adds another layer of complexity to congenital heart disease surgery. These include transposition of the great arteries, anomalous coronary artery origins and/or course, supravalvular aortic stenosis, and coronary artery fistulae. Connective tissue disorders, such as Marfan Syndrome or Loeys–Dietz syndrome, or primary aortic valve abnormalities may lead to significant aortic root dilation and require valve-sparing aortic root replacement with concomitant coronary artery re-anastomosis.Reference Fraser, Liu and Zhou6 Acquired heart disease such as Kawasaki disease can lead to coronary artery aneurysm formation, stenosis, and/or occlusion, and coronary artery bypass grafting may be necessary to maintain distal coronary perfusion.Reference Ochi7
Current guidelines that outline the clinical indications and optimal imaging views for transoesophageal echocardiographyReference Ayres, Miller-Hance and Fyfe2 do not explain intraoperative coronary artery anatomy imaging. The objective of this review is to outline the utility of coronary artery assessment using transoesophageal and epicardial echocardiography during congenital or acquired heart disease surgeries, describe how it is performed, and to introduce a practical and systematic guideline for intraoperative coronary assessment. Whilst these echocardiographic imaging techniques are equally applicable in adults, the review focuses on intraoperative imaging of coronary arteries in paediatric congenital and acquired heart disease surgeries.
Instrumentation
Equipment
The transoesophageal echocardiography probe depends on the size of the patient and the ability for safe insertion. Safe practices in patient selection and transoesophageal echocardiography probe insertion are discussed elsewhere.Reference Ayres, Miller-Hance and Fyfe2 The availability of micro multi-probes that have a centre frequency of 6–7.5 MHz has allowed intraoperative assessment in neonates as small as 2.5 kg without major complicationsReference Scohy, Gommers and Jan ten Harkel8,Reference Pavithran, Natarajan, Vishwambaran, Arke and Sivakumar9 In our experience, imaging of the proximal coronaries is possible using the micro multi-probe in most cases, albeit the course beyond the proximal origins is less easily followed. Epicardial echocardiography is often an adjunct in these patients. At our institution, both transoesophageal and epicardial echocardiography are performed using Philips (IE33; Philips Ultrasound, Bothell, Washington, United States of America) machines with compatible probes.
The transoesophageal echocardiography probe best used in infants and small children is the paediatric mini multi-probe that has a frequency of 5–7 MHz and provides excellent resolution for cardiac anatomy. The adult multi-plane transoesophageal probe also operates with a frequency of 3–7 MHz and is generally reserved for older children, with again optimal coronary imaging capabilities. The three-dimensional matrix-array transducer operates at a frequency of 5–7 MHz and offers excellent colour Doppler image quality for detailed colour flow analysis, as well as real-time three-dimensional assessments and full-volume data sets for functional information.Reference Lang, Badano and Tsang10
The transducers used for epicardial echocardiography are analogous to those used for standard transthoracic imaging. It employs high frequency of 12 MHz sector array transducers with the smallest footprint for neonatal and small children or the 8 MHz phased array probes for older children. We find that the 12 MHz sector array transducer is ideal for coronary assessment regardless of age. A sterile sleeve is used to keep sterility within the operating field.
Normal coronary artery anatomy
An overall understanding of normal coronary artery origins and course and usual segmental supply is essential before abnormalities of structure and function can be identified. The left coronary artery arises centrally from the left coronary sinus and continues as the left main coronary artery before bifurcating into the left anterior descending and circumflex arteries. The normal left coronary artery system is shown in Figure 1a.

Figure 1. Three-dimensional reconstruction from CT cardiac angiography showing (a) normal left coronary artery origin and proximal course with bifurcation to the left anterior descending (LAD) and left circumflex (LCx) coronary arteries, and (b) normal right coronary artery (RCA) origin and proximal course. Ao = aorta; LA = left atrium; LV = left ventricle; PA = pulmonary artery; RA = right atrium; RV = right ventricle.
The right coronary artery arises centrally from the right coronary sinus in an anterior and slightly inferior position to the left coronary ostium. The right coronary artery courses along the right anterior atrioventricular groove and continues posteriorly and inferiorly towards the diaphragmatic surface. The normal right coronary artery is depicted in Figure 1b.
Coronary artery two-dimensional assessment – A practical guide
Wherever possible, coronary arteries should be assessed in at least two imaging planes. Similar to transthoracic echocardiography, the gain and compression settings should be adjusted to enhance the blood-tissue interphase, the sector width reduced and focus directed to the area of interest. The individual coronary arteries will require separate plane adjustments to optimise their imaging quality and to ensure the coronary arteries in question are kept in focus and in the centre of the image. If the study switches from transoesophageal to epicardial imaging mid-way through an examination, it is an important reminder that imaging changes from visualising the most posterior structures at the top of the image display during transoesophageal echocardiography to viewing the most anterior structures with epicardial echocardiography.
Transoesophageal echocardiography
The coronary artery origins and their proximal course can be imaged by transoesophageal echocardiography in most cases. Typically, the transoesophageal examination of the coronary arteries starts with the mid-oesophageal four-chamber view at a 0° angle. Slight withdrawal of the probe with anteflexion from this position will display the left ventricular outflow tract and aortic valve anteriorly, and small adjustments with flexion and rotation in this plane can reveal the origin and proximal courses of both left and right coronary arteries. The usual left coronary artery origin and proximal course at 0° are shown in Supplementary movies S1A (two-dimensional imaging) and S1B (colour flow imaging).
The transducer is then rotated to 30–45° in the mid-oesophageal position and with minor tip anteflexion with or without probe withdrawal; the aortic valve is displayed in a short-axis plane. Similar to transthoracic echocardiography, this short-axis view is very useful in visualising the coronary artery origins and their proximal course. In this view, the left coronary ostium arises from the left coronary sinus, which is located posteriorly at approximately the 2 o’clock position. The left main coronary artery from ostium to bifurcation should be identified in this plane. Beyond the bifurcation, the proximal left anterior descending artery takes an anterior and leftward course and can be seen coursing towards the 5–6 o’clock position. The circumflex artery courses posteriorly, and usually only the very proximal aspect is visualised in this short-axis view. The right coronary artery origin arises anteriorly from the right coronary sinus in approximately the 7 o’clock position, and its proximal course may be followed towards the right atrioventricular groove. The usual right coronary artery origin and proximal course at 35° are shown in Supplementary movies S2A (two-dimensional imaging) and S2B (colour flow imaging).
Keeping the probe in a mid-oesophageal level, further angulation to 60–75° will display the right ventricular inflow–outflow view. In this view, minor adjustments with rotation and anteflexion will show the right coronary artery coursing anteriorly in the right atrioventricular groove. The aortic valve long-axis view, akin to the left ventricular three-chamber view, is seen when the transducer is rotated to 115–130° in a mid-oesophageal plane. In this view, both the right and left coronary arteries may be visualised; hence, it is important for the operator to take note if they are rotating the probe to the left or the right of the patient. If angling towards the right outflow tract, the right coronary artery will be visualised. Leftward rotation and anteflexion towards the left ventricular outflow can reveal both the left anterior descending artery and circumflex arteries, and the circumflex artery may be seen coursing posterior to the mitral valve in the left atrioventricular groove. If the coronary artery orientation is unclear in the long-axis plane, it is helpful to visualise the coronary artery in question in a short-axis plane and to rotate the probe keeping the same coronary in view. Figure 2 illustrates the typical positions and proximal course of the coronary arteries at different angles with transoesophageal echocardiography.
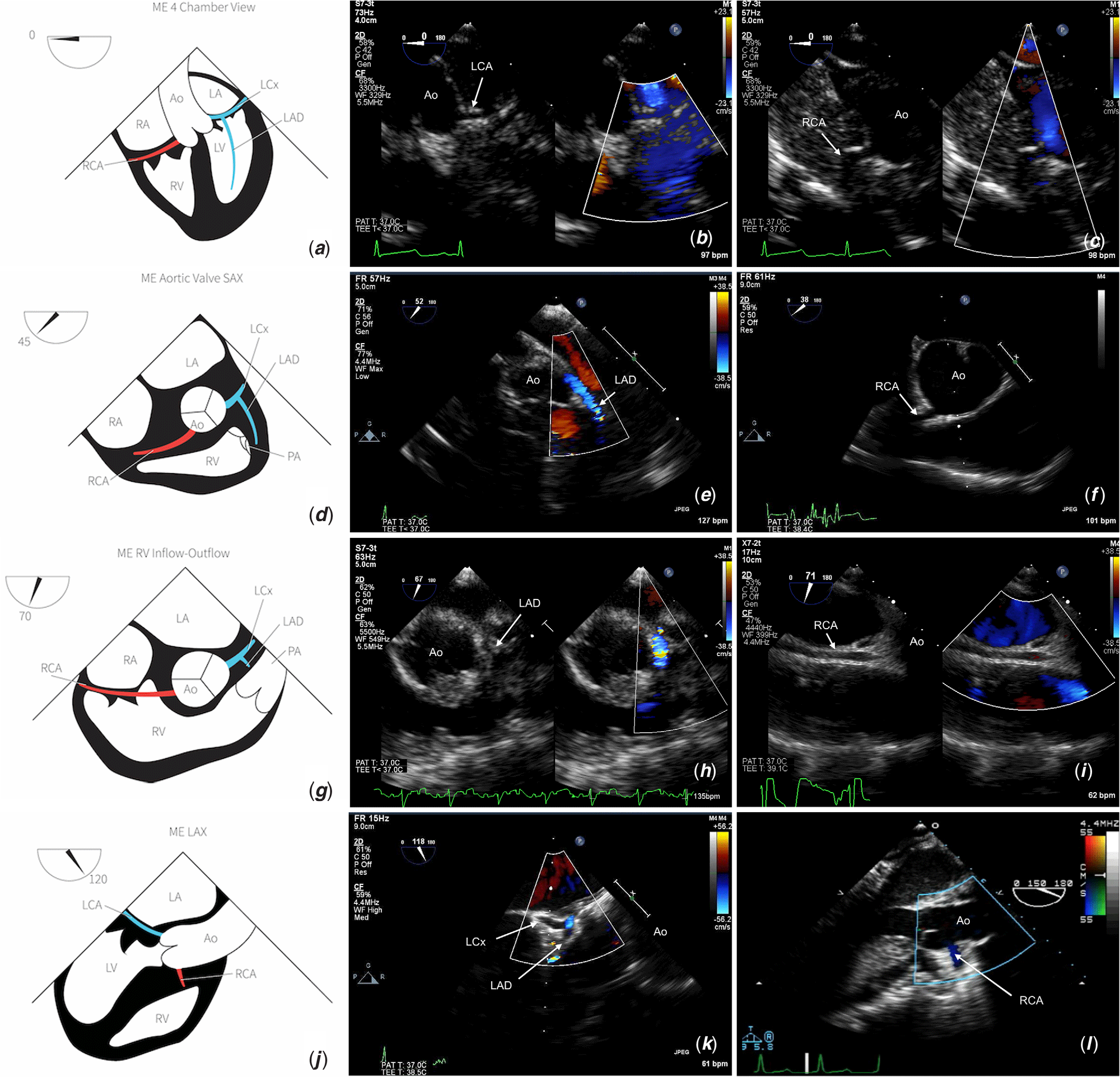
Figure 2. Illustration and typical coronary artery anatomy viewed at (a–c) 0° , (d–f) 45°, (g–i) 70°, and (j–l) 120° by transoesophageal echocardiography. Ao = aorta; LAD = left anterior descending; LCA = left coronary artery; LCx = left circumflex coronary artery; RCA = right coronary artery.
Epicardial echocardiography
The coronary artery views obtained with epicardial imaging are dependent on the position and angulation of the probe on the heart. It is essential for the operator to keep the transducer marker to the left of the patient in order to maintain a correct left–right orientation. The concepts used to optimise image quality as previously mentioned are the same as for standard transthoracic or transoesophageal imaging. For coronary artery assessment, the simplest starting point is to orientate the probe marker on the transducer to approximately 2 o’clock to display the aortic valve in a short-axis plane. The epicardial probe is usually positioned at the level of the aortic root at the same plane as the coronary arteries, with angulation and small adjustments to reposition the probe in order to attain the desired image akin to a conventional aortic valve short-axis view. It is also usually possible to image the coronary arteries with the probe positioned over the proximal ascending aorta and angulated inferiorly, or at the left ventricular outflow tract and angulated superiorly. Gentle placement and manipulation of the epicardial probe on the surface of the heart are often sufficient to attain adequate images. It is important to avoid unnecessary pressure over the coronary arteries that may lead to coronary artery compression or falsely represent coronary stenoses when there is none. Ensuring there is sufficient ultrasound gel within the sterile probe cover as well as irrigation fluid within the chest cavity allows for optimal probe contact and image quality.
When a short-axis image is achieved, the left coronary artery arises from the posterior leftward aortic sinus and right coronary artery will be seen arising from the right coronary sinus in an anterior position. Similar to transoesophageal echocardiography, it is possible to follow the proximal course of the left main, left anterior descending, circumflex, and right coronary arteries by rotating the transducer clockwise to 3 o’clock and using minor angulation adjustments. From this position, rotating the probe counterclockwise by 90° will display the left ventricular outflow tract and aortic valve in a long-axis plane (akin to the parasternal long-axis image by transthoracic echocardiography) and minor tilting of the probe leftward or rightward may display both left and right ostia and proximal course. It is also possible to visualise the bifurcation into left anterior descending and circumflex arteries in this plane.
Coronary artery colour and spectral Doppler assessment
The assessment of coronary arteries is not complete without further interrogation using colour and spectral Doppler techniques. Optimal colour flow imaging involves reducing the colour sector width and box size to the area of interest and lowering the colour scale to 20–40 cm per second so that colour flow in the coronary artery can be identified. A normal coronary artery should display laminar antegrade systolic and diastolic colour flow at low velocities. Aliasing of flow at standard velocities or needing a higher colour scale to demonstrate laminar flow may be suggestive of coronary artery narrowing.
Where possible, the sample volume for spectral Doppler is positioned over the proximal coronary artery being interrogated. The left main and right coronary arteries are usually sampled just beyond their origins, and left anterior descending and circumflex arteries are sampled just after the bifurcation. In the setting of suspected coronary artery stenoses, the spectral Doppler sample volume should be positioned and interrogated at the point of maximal colour flow aliasing. Adjusting the sample volume width to a smaller sample volume may aid to improve the clarity of the Doppler signal.
The normal spectral Doppler flow pattern in both left and right coronary arteries comprises of a late systolic peak followed by diastolic flow. The pattern is biphasic and should return to baseline between systole and diastole with no flow reversal. The pattern and velocity of flow are highly dependent on getting adequate parallel alignment of the Doppler beam to the coronary artery. If the beam is not completely parallel to the flow, as is often the case with the circumflex artery, the pattern will be monophasic, but should still be seen in both systole and diastole. Use of angle correction may be an option, but it is far better to try different probe angles and orientations to optimise the Doppler angle. Similar challenges with coronary artery alignment exist when using epicardial echocardiography, although there may be more flexibility with probe angulation to obtain an optimal Doppler angle. Examples of normal colour and spectral Doppler assessment of the coronary arteries are shown in Figure 3.

Figure 3. Transoesophageal echocardiography showing examples of normal colour and spectral Doppler assessment of the proximal (a and b) left and (c and d) right coronary arteries. The sample volume for spectral Doppler is placed in the proximal coronary artery being interrogated. Ao = aorta; LAD = left anterior descending; RCA = right coronary artery.
Intraoperative coronary artery assessment
Before starting intraoperative imaging, the operator should have prior knowledge of patient’s native coronary artery anatomy, the surgical procedure performed, and whether any technical difficulties were encountered. Clear and collaborative communication between both the surgeon and operator allows for targeted imaging, identification of any post-surgical issues, and directed re-intervention, if necessary.
Two-dimensional imaging should show widely patent coronary ostia and proximal course without kinking, angulation, discrete narrowing, or significant calibre changes to suggest intrinsic stenosis or extrinsic compression from neighbouring structures. Aliasing or a mosaic flow pattern at a higher colour Doppler scale represents higher velocity flow and may indicate coronary artery stenosis. Simultaneous comparison of two-dimensional and colour Doppler imaging of the vessel can be helpful to detect the precise location of the stenosis. High peak (>100–120 cm/s or >4 mmHg) and mean Doppler velocities (>70 cm/s or >2 mmHg) or loss of phasic flow that fails to return to baseline may raise suspicion of coronary artery stenosis (unpublished data, 319 cases). Normal flow velocities for the left anterior descending coronary artery are in the range of 35–86 cm/s and RCA 29–81 cm/s (unpublished data, 106 cases). Identifying an abnormal flow profile intraoperatively after weaning from cardiopulmonary bypass allows the surgeon to inspect the surgical repair and to intervene on the coronary artery immediately if required. The assessment of global ventricular function and regional wall motion abnormalities may further suggest coronary insufficiency to the affected coronary territory. Figures 4–6 show examples of left anterior descending (Fig 4, Supplementary movie S3), left circumflex (Fig 5, Supplementary movie S4), and right coronary artery (Fig 6, Supplementary movie S5) stenoses by colour and spectral Doppler following arterial switch operations, together with improved Doppler tracings post-second cardiopulmonary bypass run and surgical revision of the affected coronary artery. Figure 7 depicts an example of proximal left anterior descending coronary artery narrowing following repair of supravalvular aortic stenosis. Further runs of cardiopulmonary bypass were required to repair the coronary artery, with an excellent final result after the third bypass run.
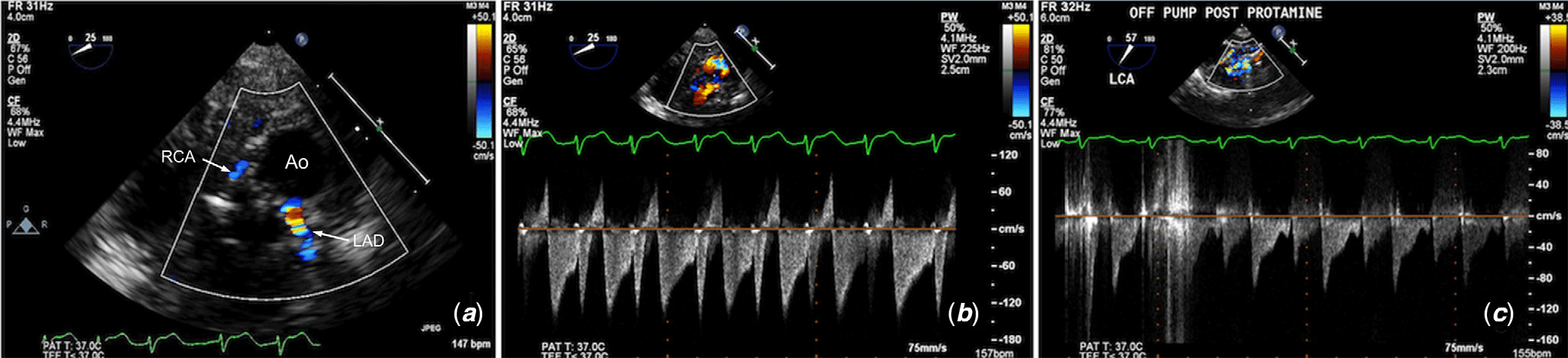
Figure 4. Transoesophageal echocardiography (TOE) imaging of the left anterior descending (LAD) coronary artery post-arterial switch operation shows (a) colour flow acceleration beyond the origin with (b) increased peak velocities and prominent flow reversal in systole. (c) TOE post-second cardiopulmonary bypass run post-revision of the LAD shows lower peak velocities and no flow reversal. Ao = aorta; LAD = left anterior descending; RCA = right coronary artery. (Corresponds with Supplementary movie S3)

Figure 5. Epicardial echocardiography imaging of the circumflex coronary artery post-arterial switch operation shows (a) colour flow acceleration over a long segment proximally, with (b) increased peak velocities and an abnormal Doppler pattern. (c) After revision following a second bypass run, there is an interval decrease in the peak velocity. Ao = aorta; LAD = left anterior descending; RCA = right coronary artery. (Corresponds with Supplementary movie S4)

Figure 6. Epicardial echocardiographic imaging of the right coronary artery post-arterial switch operation shows (a) turbulent flow and narrowing beyond the ostium with (b) increased Doppler flow velocities. (c) Post-revision there is phasic flow with an interval decrease in peak velocity. Ao = aorta; LCA = left coronary artery; RCA = right coronary artery. (Corresponds with Supplementary movie S5)

Figure 7. TOE imaging of the left anterior descending (LAD) coronary artery post-repair of supravalvular aortic stenosis shows (a) proximal kinking and diffuse narrowing of LAD with colour flow acceleration, and (b) increased peak and mean velocities. (c) TOE post-revision of the LAD after the third cardiopulmonary bypass run shows a widely patent calibre vessel with (d) normal peak velocities and Doppler pattern.
At our institution, transoesophageal echocardiography is usually the first line intraoperative imaging modality for post-bypass coronary artery interrogation, with supplemental epicardial imaging if further anatomical clarification is required. Focused two-dimensional, colour flow, and spectral Doppler imaging of the coronary arteries are performed immediately post-bypass in cases where surgical manipulation of the coronary arteries has occurred, or in cases who have unexpected significant ventricular dysfunction or electrographic signs of ischaemia post-bypass.
Specific conditions requiring coronary artery surgical manipulation
Intraoperative coronary artery assessment is indicated in cases that require surgical manipulation of the coronary arteries. In the paediatric population, surgeries include transposition of the great arteries post-arterial switch operation,Reference Wong, Golding and Hess11 repair of anomalous coronary artery origins or primary coronary artery stenosis,Reference Yamagishi, Yasu, Ohara, Kuro and Miyatake12 relief of supravalvular aortic stenosis,Reference Arnaiz, Koolbergen, Adsuar and Hazekamp13 and post-aortic valve and/or root replacement.Reference Koh, Ferdinand, Jin, Gibson and Pepper14 The value of intraoperative coronary artery imaging may be extrapolated to the assessment of post-Ross or Ross–Konno operations for left ventricular outflow tract obstruction. In adult heart disease, these indications extend to transcatheter aortic valve implantation proceduresReference Delgado, Ng and Shanks15 and coronary artery bypass grafting.Reference Savage, Lytle and Aronson16,Reference Guarracino, Cariello and Tritapepe17
Whilst it seems intuitive that the coronary arteries should be interrogated routinely intraoperatively post-surgical repair using echocardiography, there are only a small number of studies addressing the topic. In transposition of the great arteries, potential surgical complications following arterial switch operation include coronary artery origin stenosis due to anatomical distortion or kinking, torsion, or overstretching of the vessel.Reference Sung, Chang and Lee18 The technical difficulty of the surgery is further increased with abnormal coronary artery patterns such as a single or intramural coronary artery, which is an independent risk factor for early post-operative morbidity and mortality.Reference Kirklin, Blackstone, Tchervenkov and Castaneda19,Reference Khairy, Clair and Fernandes20 A higher peak systolic velocity and higher peak velocity time integral in the left main coronary artery Doppler profile correlated with increased reoperation rates following the arterial switch operation.Reference Wong, Golding and Hess11
In patients who have undergone coronary artery transfer for homograft aortic valve and root replacement, Koh et al found that intraoperative transoesophageal echocardiography detected important ostial and proximal coronary artery stenosis.Reference Koh, Ferdinand, Jin, Gibson and Pepper14 Coronary artery transfer from the native root to the graft may risk kinking, overstretching, or malpositioning of the coronary buttons during this process. The distal homograft suture line is frequently in close proximity to the coronary ostia and misplacement of sutures may interfere or interrupt coronary flow.Reference Koh, Ferdinand, Jin, Gibson and Pepper14
In Kawasaki disease following coronary artery bypass grafting, transoesophageal echocardiography has a limited ability to visualise the patency of mammary grafts despite reliable interrogation of proximal segments.Reference Tardif, Vannan, Taylor, Schwartz and Pandian21 Epicardial echocardiography has the advantage of direct transducer placement over the revascularised coronary artery, allowing for assessment of graft anastomosis patency and assess distal coronary flow.Reference Xing, Khabbaz and Karia22 Arruda et al reported that epicardial intraoperative power Doppler imaging was feasible in nearly all of their patients and accurately detected graft obstruction.Reference Arruda, Dearani, Click, Ishikura and Seward23
Transoesophageal echocardiography has also been reported to be helpful with confirming the diagnosis of anomalous coronary artery origins from the opposite sinusReference Fernandes, Alam, Smith and Khaja24,Reference Kasprzak, Kratochwil and Peruga25 or from the pulmonary artery.Reference Chigurupati, Sukesan, Lovhale, Dharan and Koshy26 In the latter example, colour and pulse wave spectral Doppler were useful in assessing retrograde flow in the anomalous left coronary artery and the presence of septal collaterals.Reference Chigurupati, Sukesan, Lovhale, Dharan and Koshy26
Proposed intraoperative guideline for coronary artery evaluation
An algorithm for coronary artery imaging is proposed in Figure 8. We recommend performing transoesophageal echocardiography to assess the coronary arteries in surgeries that involve coronary artery manipulation or are adjacent to the main site of repair. In addition, postoperatively if there are electrocardiographic ST changes concerning for ischaemia, unexpectedly poor global or regional dysfunction or ventricular arrhythmias post-bypass, assessment of coronary artery flows should be performed. If coronary artery assessment by transoesophageal echocardiography is unclear and further clarification is desired, then epicardial imaging should be performed.

Figure 8. Proposed clinical algorithm for intraoperative transoesophageal or epicardial echocardiography. CA = coronary artery; Cx = circumflex, LAD = left anterior descending; LCA = left coronary artery; LMCA = left main coronary artery; LV = left ventricular; RCA = right coronary artery; TEE = transoesophageal echocardiography.
Limitations
There are several factors that may affect the quality or feasibility of coronary artery imaging. As with acquiring skills in transoesophageal echocardiography, operator comfort and experience with probe angling take time and practice to develop. Interference from cauterisation artefacts, arrhythmias, or intraoperative hypotension can affect two-dimensional imaging and lead to poor Doppler tracings that may be difficult to interpret. In patients where insertion of a transoesophageal probe is contraindicated,Reference Ayres, Miller-Hance and Fyfe2 then epicardial imaging should be performed. Epicardial echocardiography again relies on the comfort levels of the surgeon or operator, and it is helpful to have effective communication between the primary operator and cardiologist to obtain optimal imaging views. Practical issues such as probe manipulation in a small operating field, putting too much pressure on the aortic root and/or coronary artery, or time limitations in an unstable patient are important factors to consider.
Summary
Transoesophageal and epicardial echocardiography are indispensible modalities for the intraoperative assessment of coronary arteries and should be used routinely in any surgeries that require manipulation or risk distortion of the coronary arteries. Importantly, it allows for a detailed intraoperative examination following weaning from bypass to evaluate the adequacy of the surgical repair so that immediate issues are corrected and perioperative morbidity is minimised. Familiarity of the standard transoesophageal and epicardial views and probe manipulation to achieve the desired coronary artery imaging planes are essential. Repeated practice and utilisation of transoesophageal and epicardial echocardiography skills will enhance the operator’s comfort and confidence levels in performing these two techniques.
Supplementary Material
To view supplementary material for this article, please visit https://doi.org/10.1017/S1047951120000116
Acknowledgements
The authors would like to acknowledge Emily Taylor for supplying the original artwork with transoesophageal echocardiography (TOE) coronary illustrations, and David Crouchley for supplying the CT coronary artery images.
Financial Support
This research received no specific grant from any funding agency, commercial, or not-for-profit sectors.
Conflicts of Interest
None