INTRODUCTION
Oases are precious and unique anthropogenic landscapes in semi-arid and arid environments. Key socioeconomic nodes, places of residency and circulation, they are also intensively cultivated and irrigated spaces (Lacoste, Reference Lacoste2014). While many oases are still exploited and managed in the world, they are considered today to be in critical condition. Less than 50 years ago, oases were the main elements of southeast Arabia's landscapes (United Arab Emirates [UAE] and Sultanate of Oman; Fig. 1), in the Al Hajar Mountains and along their piedmonts. Recent and fast development, drastic changes in population size, climate change, and consequent decreasing resources have led to the massive development of deep groundwater pumping (Mershen, Reference Mershen1998; Diener et al., Reference Diener, Gangler and Fein2003; Korn et al., Reference Korn, Haeser, Shreiber, Gangler, Nagieb, Siebert and Buerkert2004), at the expense of traditional agricultural practices and water management. In recent years, the UAE have implemented an active conservation program to preserve and revitalize some of these oases (al-Ismaily and Probert, Reference Al-Ismaily and Probert1998). To guarantee their successful preservation, however, it is essential to understand the interacting processes involved in their changes, not only the ones prevailing today, but also the long-term socio-environmental dynamics responsible for their formation, evolution, and current state (e.g., Garcier and Bravard, Reference Garcier, Bravard, Lavie and Marshall2014; Charbonnier et al., Reference Charbonnier, Purdue, Calastrenc, Régagnon, Sagory, Benoist, al-Tikriti and Yule2017b). This study aims to better comprehend the coevolution of climate change, farming strategies (water and soil management), settlement patterns, and socioeconomic activities.

Figure 1. Geographical and hydrological background. (A) Location of Masafi in the United Arab Emirates. (B) The Masafi palm grove in the Emirate of Fujairah and the center of the Al Hajar Mountains. (C) Corona Image from 1965 and zoom on the palm grove and the eastern alluvial fans flowing in its direction. The arrows and dashed lines indicate the orientation of the flow and the spatial extent of the fans (yellow, white, and orange from the oldest fan to the most recent one). (D) Google Earth Image (2017) with the urbanization of Masafi and the incised streams in blue partly flowing towards the palm grove and Wadi Sidji. (For interpretation of the references to color in this figure legend, the reader is referred to the web version of this article.)
In southeast Arabia, the Holocene climate history has been mainly inferred from paleolacustrine records, eolian sediments, speleothems, and occasionally fluvial deposits. These archives have revealed that Arabia was a very different landscape during the early and mid- Holocene period (~10.6 to ~6.3 ka; Fleitmann and Matter, Reference Fleitmann and Matter2009). A progressive increase in precipitation favored by the northward migration of the Intertropical Convergence Zone (ITCZ) and the Indian Ocean monsoon system (e.g., Burns et al., Reference Burns, Fleitmann, Matter, Neff and Mangini2001; Fleitmann et al., Reference Fleitmann, Burns, Neff, Mangini and Matter2003a, Reference Fleitmann, Burns, Mangini, Mudelsee, Kramers and Villa2007), or to the African monsoon expanding over Arabia (Jennings et al., Reference Jennings, Singarayer, Stone, Krebs-Kanzow, Khon, Nisancioglu, Pfeiffer, Zhang, Parker, Parton, Groucutt, White, Drake and Petraglia2015), provided favorable conditions for vegetation growth (e.g., Parker et al., Reference Parker, Eckersley, Smith, Goudie, Stokes and Ward2004, Reference Parker, Goudie, Stokes, White, Hodson, Manning and Kennet2006; Preston et al., Reference Preston, Parker, Walkington, Leng and Hodson2012, Reference Preston, Thomas, Goudie, Atkinson, Leng, Hodson and Walkington2015; Hilbert et al., Reference Hilbert, Parton, White, Clark-Balzan, Crassard, Groucutt and Jennings2014) and lake development (Parker et al., Reference Parker, Eckersley, Smith, Goudie, Stokes and Ward2004). Climatic conditions change abruptly around 6.2 ka. A decline in summer rainfall, due to the southward retreat of the monsoon rain belt coupled with a reduction in winter rainfall, resulted in the widespread desiccation of lakes (Parker et al., Reference Parker, Goudie, Stokes, White, Hodson, Manning and Kennet2006), the cessation of speleothem growth (Fleitmann et al., Reference Fleitmann, Burns, Neff, Mangini and Matter2003a, Reference Fleitmann, Burns, Mudelsee, Neff, Kramers, Mangin,i and Matter2003b), increased eolian activity (Atkinson et al., Reference Atkinson, Goudie, Thomas and Bailey2011), and peaks in aridity (between 5.4–5.1 ka; Preston, Reference Preston2011). Beyond that date, there is a paucity of environmental records. Climatic data is mainly provided by cave speleothems highlighting humid periods between 4–2.7 ka (Fleitmann et al., Reference Fleitmann, Burns, Mangini, Mudelsee, Kramers and Villa2007), 2.2–1.9 ka, and 450–350 yr (Fleitmann et al., Reference Fleitmann, Burns, Neff, Mangini and Matter2003a). Regrettably, this data is often disconnected from terrestrial landscapes, human occupations, and land use. Fluvial archives, which could provide this missing link, are patchy and weakly dated (Berger et al., Reference Berger, Bravard, Purdue, Benoist, Mouton and Braemer2012). Indeed, they are rarely discovered in southeast Arabia, due to the presence of the Al Hajar mountain chain and its Pleistocene alluvial fans. One object of research that has been completely neglected and that could provide new and relevant information on climate change and land use are oases that appear around the fourth millennium BC. The structure and sediments composing them are archives of Holocene natural and artificial landscapes, which can provide direct data on hydroclimatic conditions prior to human interventions and indirect data via resource—soil and water—management and subsistence strategies (Charbonnier et al., Reference Charbonnier, Purdue and Benoist2017a).
Archaeological studies in southeast Arabia have highlighted the existence of many cycles of sedentism and land abandonment throughout the Holocene. Data is scattered for the early to mid-Holocene (Drechsler, Reference Drechsler2009) but a decline in occupation is well-attested inland around ~ 5900 cal yr BP (Parker and Goudie, Reference Parker and Goudie2008). This “Dark Millennium” witnesses increasing occupation along coastal areas (e.g., Méry et al., Reference Méry, Charpentier, Auxiette and Pelle2009) and probably in refugia in the Al Hajar Mountains, where local resources could have been available (Presten and Parker, Reference Preston and Parker2013). After ~4500 cal yr BP, on the onset of southeast Arabia's current climatic regime, phases of land conquest (4500–3600, 3050–2600, and 550–50 cal yr BP) resulted in the emergence and development of oases (Cleuziou, Reference Cleuziou and Guilaine2005) alternating with phases of population movements (3600–3250 and 2600–2300 cal yr BP) and periods for which we have very little data (2300–550 cal yr BP; Table 1). Between ca. 4500 to 2300 cal yr BP, the socioeconomic system was structured around the exploitation, transformation, and export of copper ingots from the Al Hajar mountain chain (Cleuziou, Reference Cleuziou1982, Reference Cleuziou, Burnouf, Bravard and Chouquer1997, Reference Cleuziou, Braemer, Cleuziou and Coudart1999, Reference Cleuziou2009), while later and current oases (550–50 cal yr BP) invested more in developing cash crops. The paleobotanical study of early oases has pointed out the cultivation of cereals (Tengberg, Reference Tengberg, Neumann, Butler and Kahlheber2003) and date palms (e.g., Cleuziou and Costantini, Reference Cleuziou and Costantini1980; Weisgerber, Reference Weisgerber1981; Willcox, Reference Willcox and Frifelt1995; Tengberg, Reference Tengberg1998), which was also the case for more recent palm groves. Arabian farmers used and still use wells, cisterns, and channels to manage water but they also massively invested, probably from ~3050 cal yr BP (al-Tikriti, Reference Al-Tikriti2002), in underground galleries to distribute groundwater, a technology referred to as falaj in the UAE. Farmers would have coped with the Holocene aridification and decreasing levels of the aquifers by deepening their galleries and gardens. Based on the similarities between past and current water management and vegetation cover, and the disappearance of ancient gardens as a result of their digging progressively deeper, the study of oases has been hampered by the denial of their diachronic and dynamic dimensions (Power and Sheehan, Reference Power and Sheehan2011). Only very recent interdisciplinary studies in Arabia have refined our vision of the history of oases. Studies in the oasis of Tayma (Wellbrock et al., Reference Wellbrock, Grottker, Gebel, al-Tikriti and Yule2017), Al Ain (al-Tikriti, Reference Al-Tikriti2002; Power and Sheehan, Reference Power and Sheehan2011), Al-Madam (Córdoba, Reference Córdoba2013), Bat (Desruelles et al., Reference Desruelles, Fouache, Wassel, Cammas and Wattez2016), and Masafi (Charbonnier et al., Reference Charbonnier, Purdue and Benoist2017a, Reference Charbonnier, Purdue, Calastrenc, Régagnon, Sagory, Benoist, al-Tikriti and Yule2017b), the latter integrated into a larger project on multiple oases (Project ANR – Agence National de la Recherche OASIWAT, Director L. Purdue) have revealed that oases can be studied, and that they are dynamic, resilient, and mobile landscapes. Numerous questions remain, however, as we know very little about oasis agrosystems (soil and water management) and climate change.
Table 1. Major chrono-cultural phases in Southeast Arabia (Magee, Reference Magee1995, Reference Magee1997) correlated with occupation at Masafi.

In this paper, we aim to better comprehend and discuss oasis formation, management, and climate change, as well as their dynamic interactions during the Holocene, a period poorly documented in Arabia and for which sedimentary archives are scarce. Based on the systematic study of one oasis, the oasis of Masafi (Hajar Mountains, Emirate of Fujairah, French Archaeological Mission in the UAE), we are able to answer three major questions: What was the initial natural environment during the Holocene and when did the oasis system of production appear? How did this system evolve in the framework of long-term and abrupt climate changes? How does this system compare to other landscapes in southeastern Arabia? We propose here a diachronic reconstruction of the oasis landscape using the methods of agrarian geoarchaeology, hydraulic archaeology, spatial analysis, geophysics, chronology, and geomorphology. The combination of these methods in a well-dated archaeological environment and on thick sedimentary sequences have allowed us to provide new and unique data on water and agricultural strategies, provide new sedimentary archives of Holocene terrestrial dynamics, discuss the impact of Holocene climate change on Arabian communities, and rethink the environmental and landscape history of southeastern Arabian oases.
STUDY AREA
Climatic, geological, and hydrological background
The oasis of Masafi is located north of the Al Hajar Mountains (Fig. 1A). The mountain chain, which covers Northern Oman and the United Arab Emirates (UAE), is nearly 700 km long, 40 to 130 km wide, and 3000 m high in some areas. These mountains contain two scarce and precious resources: copper and water. Composed of superimposed sheets of Cretaceous limestone and ophiolites (gabbros, peridotite, serpentinite, and copper veins) as a result of subduction events during the Cretaceous and renewed uplift during the Oligocene, these geological formations are also fractured, enabling the recharge of most aquifers and the formation of springs. To the east and the west of the mountains, lying on top of the bedrock, old and recent Quaternary alluvial deposits (Tourenq et al., Reference Tourenq, Brook, Knuteson, Shuriqi, Sawaf and Perry2011) also contain the largest reserve of fresh groundwater aquifer (Rizk and El Etr, Reference Rizk and El Etr1997; Brook et al., Reference Brook, Al Houquani, Darawsha, Al Alawneh, Achary and Mohamed2006). This supply in water has been crucial through time in the bimodal arid to hyper-arid climate of the Al Hajar Mountains (Böer, Reference Böer1997). Temperatures in Masafi reach an annual average value of 28.4°C with average summer temperatures of 35.3°C (June to September) and 23°C in winter (December to March; http://worldweatheronline.com, 2009–2017 average). Annual average precipitation do not exceed 161 mm/year, with precipitation in summer (June to August) reaching an average of 0.6 mm and 30.7 mmm in winter (December to March; http://worldweatheronline.com, 2009–2017 average). The low amount of rainfall in winter (rainfall from the east coast of Africa, frontal storms from the Mediterranean, and southward advance of the westerlies) and during the summer months (Indian Monsoon convective storms and northward shift of the ITCZ), would have required the development of specific irrigation techniques and soil management.
The oasis of Masafi is located in a small depression at the border between the Emirate of Fujairah to the south (our area of study) and the Emirate of Ras al Khaimah to the north (UTM coordinates: 40 R, 415730 E, 2798920 N; Fig. 1B). This terraced palm grove covers nearly 50 ha in the upstream basin of Wadi Sidji (Fig. 1B and C). This intermittent and entrenched stream flows west-southwest through the Al Hajar Mountains and into the dunes of the Rub’ al-Khali desert, 30 km downstream. While the oasis overlooks the wadi to the west, it is surrounded by high-sloped bedrock and Quaternary alluvial fans (Fig. 1C), composed of basic igneous bedrock part of the Ophiolite complex, mainly harzburgite and serpentinite with calcite veins. Lying on top of the bedrock are Quaternary deposits, comprised of boulders, cobbles, gravel, and sand, increasingly cemented in their lower part.
The study of satellite images (Fig. 1C and D) has highlighted the fact that Masafi is directly located in the trajectory of gullies located at the terminal end of alluvial fans originating 1 to 1.5 km to the east. These fans are composed of coarse gravel, stones, and blocks of harzburgite, as well as some blocks of limestone and finer material composed of light yellowish-brown silty sands, which correspond to weathered deposits and old pockets of eolian sands. Channeling these gullies could have provided both soil and water to agricultural fields but sheetflow erosion or uncontrolled water management could have led to soil erosion as well as the deposition of coarse sediments in cultivated areas of the palm grove.
Masafi has been the main producer of mineral water in the Gulf since 1976. Today, water is drilled into deep aquifers to irrigate the dominant date palms. Indeed, apart from a few mango and jujube trees, carrots, and onions, traces of agriculture are scarce in the palm grove.
Archaeological background
Archaeological excavations have been conducted in Masafi since 2007 (directed by A. Benoist 2007–2016 and J. Charbonnier since 2017) as part of the French Archaeological Mission in the United Arab Emirates (directed by S. Méry). These excavations have allowed for the discovery of five sites surrounding the palm grove and occupied since the Bronze Age (Masafi 1–5; Fig. 2A, Table 1; Benoist et al., Reference Benoist, Bernard, Charbonnier, Goy, Hamel and Sagory2012; Benoist, Reference Benoist2013). Masafi 4 and Masafi 5, located on small hills, are respectively dated from 3950–3550 cal yr BP (Middle Bronze Age) to 3050–2550 cal yr BP (Iron Age II), and 3550–3050 cal yr BP (Late Bronze / early Iron Age I). The three other sites, Masafi 1–3, were occupied between 3050–2550 cal yr BP (Iron Age II). Masafi 2 is a large fortified village erected on the rocky hill of Jebel Halyan. Masafi 1 is a large building with a pillared room, surrounded by a probable market 60 m to the south. Masafi 3 is a small shaped sanctuary where incense burners and copper snake figurines were discovered. In our current state of knowledge, the area of Masafi seems to have been abandoned around 2550–2550 cal yr BP (Iron Age III) and up until ~550 cal yr BP (Late Islamic period; Benoist et al., Reference Benoist2013). After that date, a mud brick fort and mosque were built south of the palm grove, while small stone structures and masonry houses were in use at the site of Masafi 2. The discovery of numerous Chinese and Japanese sherds suggests that Masafi was well integrated in an international trade network (Sasaki and Sasaki, Reference Sasaki and Sasaki2006). During the first half of the twentieth century AD, the father of the current sheikh of Fujairah built his residence east of the oasis, above the ruins of Masafi 3 (Ziolkowski and al-Sharqi, Reference Ziolkowski and al-Sharqi2005), underlining the persistent importance of the oasis through time.

Figure 2. (color online) The Masafi palm grove. (A) Digital elevation model of the palm grove and location of the main archaeological sites. (B) Location of the five main terraces and the eight test pits studied. The dashed line corresponds to the geophysical transect. (C) Results of the electrical resistivity tomography (ERT) geophyical profile.
MATERIAL AND METHODS
Mapping the spatial and vertical extension of the palm grove and its watershed
To understand the spatial extension of the palm grove, its structure, and main components, the current oasis was placed within its microregional to regional archaeological and geomorphological context. Data were extracted from the Shuttle Radar Topography Mission (digital elevation model [DEM] with a 90-m resolution) and from topographic maps at a scale of 1/25000 published in 1976 and kindly provided to us by the Fujairah Municipality. In the perspective of a regressive approach, the oasis structures (terrace boundaries and slope changes), hydraulic constructions (canals, wells, and cisterns), and houses were mapped. This survey was conducted using mainly a differential GPS (DGPS) in more open areas and a total station in densely vegetated areas. In parallel, and in order to cover the largest area possible, kite views were taken using a camera fixed on a kite. These vertical views, replaced in a geo-referenced frame located using the DGPS and/or total station, were used to build a DEM (Fig. 2A and B) by photogrammetry and orthographic pictures using Agisoft Photo Scan Pro. These data (DGPS, total station, photogrammetry) were combined using the GIS software (Arcgis 10.2), allowing us to obtain a model covering a 10 × 10 m dot grid (Fig. 1B and 2A), as well as providing information on the excavations. The surface covered by this detailed mapping comes to 29 ha with a resolution ranging from 10 cm under the palm trees to 5 cm in open spaces.
In order to understand the paleotopography of the palm grove, we conducted in 2017 a geophysical survey involving two-dimensional electrical resistivity tomography (ERT). ERT was carried out with a Wenner-Schlumberger array and the device used a multi-electrode system Abem Terrameter LS with 64 electrodes. An electrode spacing of 2 m allowed us to investigate 382 m, following an east-west transect, at a depth of nearly 12 m. Data inversion and interpretation were carried out using the Res2dinv software (Loke and Barker, Reference Loke and Barker1996), which uses a least-square inversion technique to get a subsurface model from apparent resistivities measured in the field. Less than five iterations allowed us to get a RMS error below 5%, which is considered as satisfying (Fig. 2C).
Agrarian geoarchaeology and hydraulic archaeology
In order to understand the formation and evolution of the palm grove, we decided to pursue a systematic study of the agricultural terraces to identify irrigated fields and connected hydraulic structures. The approach and methods adopted are based on the ones of geoarchaeology, archaeology, agronomy, chronology, and taphonomy of hydraulic and agricultural systems (Fig. 3; Berger and Jung, Reference Berger, Jung and Chouquer1996; Purdue and Berger, Reference Purdue and Berger2015). Eight test pits (TP) were dug between 2011 and 2016 (east-west transect) in the framework of the excavation of Masafi 1 (TP A) and directly in the palm grove to understand the past and current organization of the terraces (TP B–H; Fig. 2B). Each stratum was defined based on its texture, color, structure, stratigraphic boundaries, as well as the presence of macroremains (e.g., charcoal and shells), artifacts (sherds), and pedological features (e.g., secondary carbonates, iron oxides, and bioturbation). The precise description of the strata allowed us to create a typology of the deposits encountered and classify sterile layers, agricultural soils, eolian deposits, and major phases of erosion, as well as hydraulic structures such as canals and wells. Due to the high number of strata identified, this typology is key to chronostratigraphical correlations throughout the palm grove.
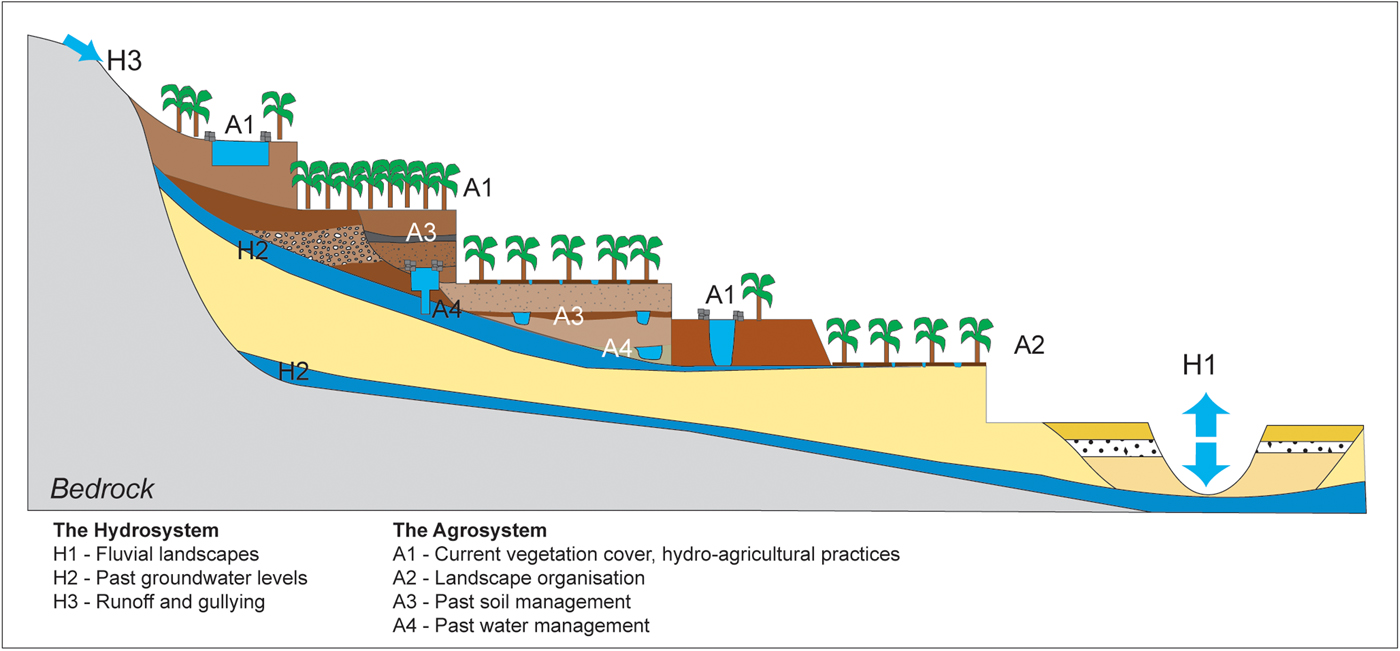
Figure 3. (color online) Principle and objectives of the geoarchaeological study of oases to reconstruct the long-term dynamics of the hydrosystem and the agrosystem.
Radiocarbon, optically stimulated luminescence (OSL), and ceramic dating
Agricultural environments and terraces are very difficult to date, even more so when they are constantly reworked and modified (e.g., Acabado, Reference Acabado2009; Kinnaird et al., Reference Kinnaird, Bolòs, Turner and Turner2017). Dating the Masafi terraces was obtained by four means. First, accelerator mass spectrometry dating was conducted when in situ burning events were visible or layers of charcoal were identified in agricultural deposits or hydraulic structures. In this situation, sampling was made directly in the profile. More often, however, charcoal and large macroremains were obtained after sieving 10 L of bulk sediments and sorting under binoculars. Samples were identified when possible prior to their dating. Fifteen radiocarbon dates were processed at the Poznan Radiocarbon Laboratory (Table 2). The protocol consists in a chemical pre-treatment (Brock et al., Reference Brock, Higham, Ditchfield and Bronk Ramsey2010), followed by the combustion and graphitisation of the sample (Czernik and Goslar, Reference Czernik and Goslar2001). The 14C content was measured by a “Compact Carbon AMS” spectrometer (Goslar et al., Reference Goslar2004) and the 14C age calculated (Stuiver and Polach, Reference Stuiver and Polach1977) and calibrated using INTCAL 13-calibration curve (Reimer et al., Reference Reimer, Bard, Bayliss, Warren Beck, Blackwell, Bronk Ramsey and Brown2013) on the OxCal ver. 4.2 software (Bronk Ramsey and Lee, Reference Bronk Ramsey and Lee2013).
Table 2. Radiocarbon dates obtained in the test pits studied. All the dates were calibrated using INTCAL 13-calibration curve (Reimer et al., Reference Reimer, Bard, Bayliss, Warren Beck, Blackwell, Bronk Ramsey and Brown2013) and Oxcal ver 4.2 software (Bronk and Lee, Reference Bronk Ramsey and Lee2013).

The second method used at Masafi is OSL dating, which provides dates in briefly used structures (Berger, Reference Berger2003; Huckleberry and Rittenour, Reference Huckleberry and Rittenour2013). Two dates were processed at the Oxford Dating Luminescence Laboratory. The protocol is based on the luminescence measurement of sand-sized quartz, extracted and measured in a Lexsyg Smart luminescence reader (Richter et al., Reference Richter, Richter and Dornich2015) using a single aliquot regenerative post-infrared (post-IR) blue OSL measurement protocol (Wintle and Murray, Reference Wintle and Murray2006). One further sample was dated at the University of Freiburg using the same device. The fine-grained (silty) sample, however, did not deliver enough quartz grains and a post-IR infrared stimulated luminescence protocol was applied, using a stimulation temperature of the second measurement at 225°C. Dose-rate calculations were obtained using DRAC (Durcan et al., Reference Durcan, King and Duller2015) and are based on the concentration of radioactive elements derived from elemental analysis (Oxford, ICP-MS/AES; Freiburg, high-resolution gamma spectrometry). The paleodose determination and rate calculations are based on Aitken (Reference Aitken1985) and integrate attenuation factors (Mejdahl, Reference Mejdahl1979), dose rate conversion factors (Guerin et al., Reference Guerin, Mercier and Adamiec2011), and an absorption coefficient for the water content (Zimmerman, Reference Zimmerman1971). The contribution of cosmic radiation to the total dose rate was based on data by Prescott and Hutton (Reference Prescott and Hutton1994), and rely on latitude, altitude, and burial depth (Tables 3 and 4).
Table 3. Optically stimulated luminescence (OSL) dates conducted on quartz grains at the Luminescence Dating Laboratory of the University of Oxford (J.-L. Swenniger). The year of processing is 2016.

Table 4. Optically stimulated luminescence (OSL) date conducted on feldspar grains at the University of Freiburg (F. Preusser). The year of processing is 2016.

The third method used is ceramic dating. Sherds were systematically sampled during the excavation of the TP (Table 5) and identified by A. Benoist and M.-P. Pellegrino. Ceramics were found in primary or secondary position. Most of the sherds encountered, rounded and eroded, and probably in secondary position, were dated between 3250–2600 cal yr BP (Iron Age I and II) or 1218–500 cal yr BP (Islamic period; Magee, Reference Magee1996; Benoist, Reference Benoist2000; Kennet, Reference Kennet2004), and might have originated from Masafi 1 and 3. Indeed, these sites to the east were subject to erosion and gullying (Charbonnier et al., Reference Charbonnier, Purdue and Benoist2017a) and some of these gullies seem to have found their way to the gardens (Fig. 1C). Some sherds could also have been brought with displaced soils or with fertilizers (waste is often spread in the fields). The sherds therefore mainly provide us with a terminus post quem. What is significant here is that only Iron Age sherds were encountered in the Iron Age cultivation layers, strengthening the results of absolute dating.
Table 5. Ceramics extracted from the test pits during their excavation and chronology. *, sherds that can be associated with certitude with a specific stratigraphic unit; Terr, Terrace; TP, Test Pit; SU, Stratigraphic unit; Undat., Undated; dep., deposition; P, primary; S, secondary.

Last, chronostratigraphical control based on the definition of sedimentary facies was used as relative dating on terraces for which similar paleosoils or phases of agricultural use were encountered.
RESULTS
The spatial organization and (paleo)topography of the palm grove
Field surveys, the DEM, and the geophysical transect provide extremely relevant information on the current and past organization of the palm grove, which is difficult to perceive and map under the dense vegetation cover (Fig. 2A–C). Five main terraces, sloping to the west, were distinguished. Three main terraces (T1–3), lying on the eastern side of the oasis, present a homogeneous surface: T1, 250 m wide, lies at an elevation slightly above 440 m; terrace T2, 70 m wide, around 437 m; and terrace T3, 160 m long, at 435 m. The uppermost one, T1, lies 23 m above the bottom of Wadi Sidji, located about 600 m to the west. It is currently urbanized, while T2 is cultivated and irrigated for palm trees and T3 is partly abandoned. The topography is more irregular on T4 and T5, which contain abandoned parts of the palm grove and the recent constructions of small terrace walls and agricultural plots. The slope gently decreases from 433 m at the base of T3 to 429 m on T4, 30 m wide, and to 425 m on T5. The bottom of Wadi Sidji, overlaid by T5, lies 417 m above sea level. The eight TP studied are representative of all the terraces, with TP A and TP B on T1, TP C–E on T2, TP F on T3, TP G on T4, and TP H on T5.
The ERT profile allows us to characterize more precisely the composition and thickness of these terraces (Fig. 2C). Terraces 1 and 2 present average to high resistivity values, with even higher values in localized areas (>64.2 ohm·m), which could reveal the existence of embedded agricultural terraces with different lithologies or the presence of ancient gullies filled with gravels. Terrace 3 and 4, on the contrary, are composed of much finer material, visible directly on the surface due to its erosion. The terraces are indeed composed of very fine cemented silts, which remind one of eolian sediments. Of particular interest is the paleotopography revealed by this profile. West of the palm grove, the metamorphic bedrock was identified close to the surface, while the very eastern part of the oasis is composed of fractured harzburgite. In the middle, ca. 5 m below T1 and 2, we can identify less resistant material filling a 10-m-deep, 50-m-wide depression, in contact with these two substrata, and outcropping to the west. This depression must be a fault depression, which could explain why Masafi has benefited from good water supply for millennia, but also why the oasis has acted as a sediment trap that provides a unique paleoenvironmental record.
Identification and classification of the agricultural and hydraulic deposits
Due to the depth of the TP, the thickness of the deposits, and the high number of stratigraphic units identified, we defined six pedo-sedimentary facies (Table 6) that were encountered both in agricultural deposits and in the fills of canals, wells, and/or cisterns. Facies 1 and 2 correspond to Pleistocene and Holocene deposits. While Facies 1 is composed of well-sorted white to beige silts more-or-less pedologically developed (Facies 1 a and b), Facies 2 is composed of weakly sorted clayey silts, rich in blocks, stones (Facies 2a), and local gravel of harzburgite (Facies 2b). Facies 3 is composed of gravelly sediments. Facies 4 is comprised of five subfacies (a–e) characteristic of agricultural deposits, which differ in color (reddish-brown to greyish-beige) and inclusions (charcoal, ashes, and shells). Facies 5 is typical of backfill or slope-wash deposits (stones, blocks, and sand), while Facies 6, composed of well-sorted white silts, is interpreted as eolian or abandonment sediments. The presentation of the lithostratigraphy of the TP will directly refer to these facies.
Table 6. Pedo-sedimentary typology of the natural/cultivated deposits encountered in the test pits of the Masafi palm grove and first interpretation

Lithostratigraphy
The stratigraphical results obtained from the eight TP are presented per terrace following an east-west transect (Fig. 2B). As many strata were identified in each TP, they were grouped in Facies (presented in Table 6) and are presented by phase.
T1, TP A
The archaeological excavation of Masafi 1 has allowed for the digging of a large north-south trench and the description of about 1.4 m of stratigraphy (referred to as the North Trench in Charbonnier et al., Reference Charbonnier, Purdue, Calastrenc, Régagnon, Sagory, Benoist, al-Tikriti and Yule2017a; Fig. 4A). We have identified 3 different phases. Phase 1 is composed of archaeological occupation soils. Above, Phase 2 is comprised of small earthen canals (10–20 cm wide), part of a runoff water-channeling system (Charbonnier et al., Reference Charbonnier, Purdue and Benoist2017a) connected to cultivated deposits. The latter are composed of light-brown silts (Facies 4e) dated from 2847–2752 cal yr BP. These deposits are eroded and buried under 80 cm of alluvial fan gravel (Facies 3).

Figure 4. (color online) Terrace 1. (A) View from above of the site of Masafi 1 and lithostratigraphy of Test Pit A, Iron Age Period. (B) View from the west of Test Pit B and view from above of the tree plantation hole (Ziziphus sp.) identified in the pit (stratum 6) with a presentation of the lithostratigraphy (Iron Age to the Islamic Period). Facies are described in detail in Table 6.
T1, TP B
The stratigraphy exposed measures 2.3 m, which allowed us to distinguish four major phases of deposition (Fig. 4B). Phase 1 is composed of cemented light-beige to white silts, with a little gabbro gravel (Facies 1b, strata 1 to 3). An OSL date indicates deposition between 10.19–9.17 ka. These silts are buried under cultivated deposits (Phase 2) (Facies 4b and c; strata 5, 6, 12 and 15) On top of Stratum 6, we discovered a well-preserved tree planting hole (diameter = 1.5 m, depth = 90 cm) in which burnt fragments of Ziziphus sp. (Herveux, L, personal communication, 2016) were dated from ~3211–2989 cal yr BP. During Phase 3, these deposits are eroded by gravel from the reactivation of eastern alluvial fans (Facies 3, Strata 16–30), and forming sheetflow deposits as well as sinuous shallow gullies. Phase 4 is composed of backfill deposits and a wall (Strata 30–33, Facies 5).
T2, TP C
Located less than 50 m west of TP B (Fig. 2B), TP C was opened in the middle of T2. A depth of 4.5 m was reached before identifying the substratum (Facies 2a), indicating a substantial drop in the topography. Five different phases have been identified (Fig. 5). Phase 1 is composed of stones and blocks of gabbro in a cemented green matrix (Facies 2a) buried under 80 cm of dark-orange silty clays (Stratum 1, Facies 2b). The latter correspond to the less-resistant material identified in the ERT profile (Fig. 2C). Phase 2 is indicative of the first management of the palm grove, as two hydraulic structures have been identified. The first one is a small canal filled with greyish-brown clayey silts (Stratum 2b, Facies 4d) (Fig. 5). The texture, dark color, and weak organization of the deposits in the canal suggest its use as a drainage structure. Just above, dark-brown to reddish fine silts (Strata 7 and 8, Facies 4a), indicative of in situ burning, were dated from 2745–2490 cal yr BP. A well (Strata 9–11, Facies 5) was dug directly into them. During Phase 3, these agricultural deposits are covered by harzburgite gravel and coarse sands, rich in weathered sherds, alternating with fine hard carbonated silts of about 1 m (Strata 12–20, Facies 6 and 3). An OSL date obtained in Stratum 16 indicates deposition between 2.23–1.95 ka. Phase 4 is indicative of a probable return to agriculture, with the occurrence of brown silts (Strata 23, 25, and 26, Facies 4b), dated from 1525–1371 cal yr BP in Stratum 25. The last phase encountered is composed of 1.25 m of beige bioturbated silts and sands, which correspond to the more recent palm tree environment (Strata 27-31, Facies 4e) (Phase 5).
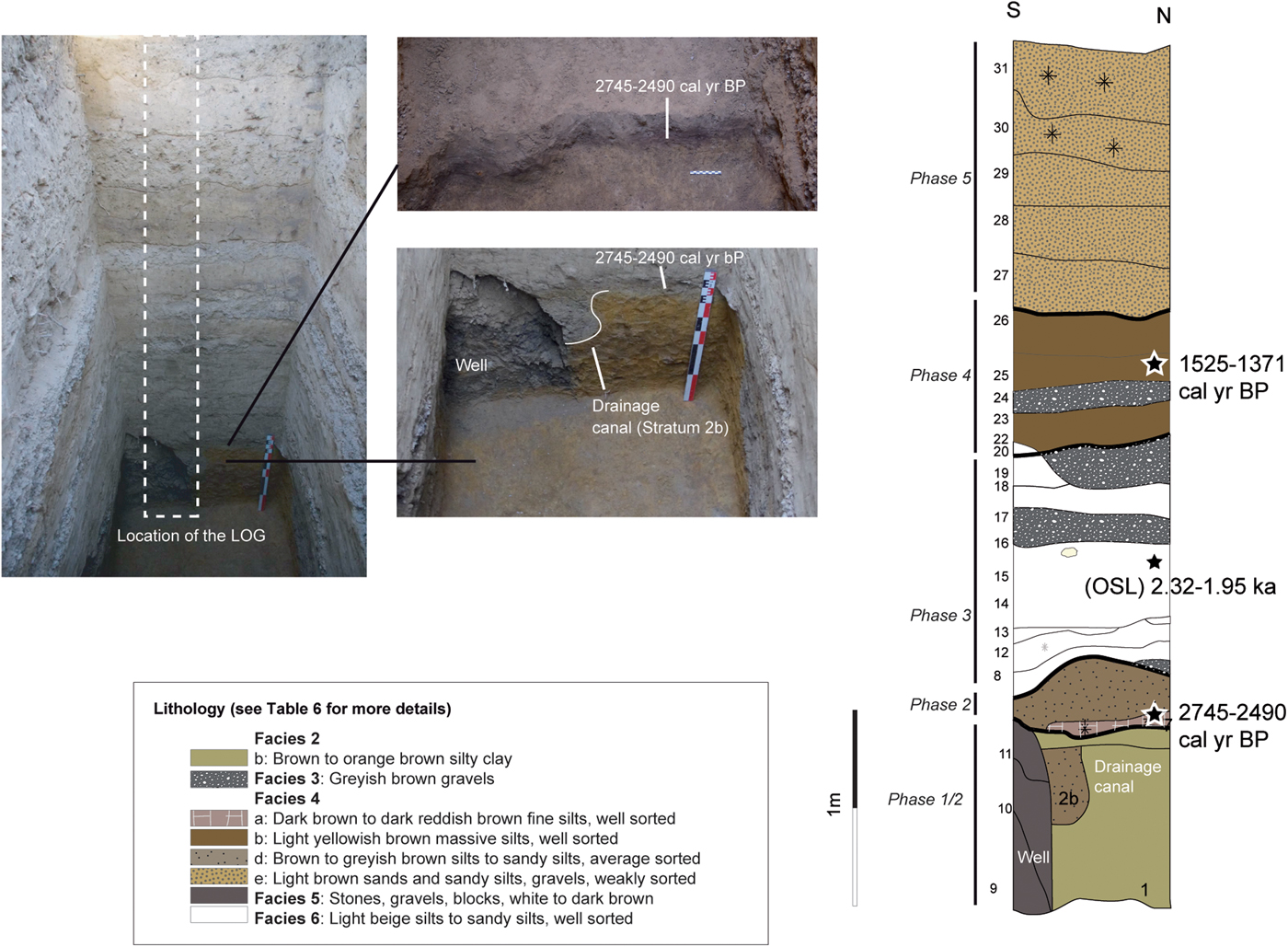
Figure 5. (color online) Terrace 2. View from the east of Test Pit C with a zoom on an in situ burnt agricultural layer (Stratum 7) (Top image), and on an Iron Age drainage canal dug in the perched groundwater table and recut by a well (Bottom image); presentation of the lithostratigraphy (Iron Age Period until today). Facies are described in detail in Table 6.
T2, TP D
Test pit D was opened also on T2, less than 50 m north of TP C (Fig. 2B). We reached the bedrock at a depth of 4.8 m (Fig. 6). Seven distinct phases can be proposed. Phase 1 is composed of stones and blocks of gabbro cemented in an orange matrix (Stratum 39, Facies 2a), buried under nearly 0.9 m of brown structured silty clayey deposits, presenting traces of in situ vegetation development (Strata 38–36, Facies 2b). Stratigraphic correlations with a nearby TP (not presented here) provided a date of 18,210–17,620 cal yr BP for the lower part of these deposits. This paleosoil also corresponds to the less-resistant material discovered thanks to the ERT profile (Fig. 2C). Phase 2 is composed of rubified brown silts rich in charcoal (Strata 37, 1, and 2, Facies 4a and c), dated from 2721–2381 cal yr BP. Above, Phase 3 deposits are comprised of fine, hard, carbonated silts alternating with gravel from sheetflow or shallow gullies in which sherds dated from the first millennium BC (Table 4) were discovered (Strata 5–10, Facies 3 and 6). A probable return to agricultural practices characterizes Phase 4 (Strata 11–13), with the deposition of greyish-beige sandy silts containing ashes and soil aggregates (Facies 4c and d) dated from 1858–1874 cal yr BP (Stratum 12). Above (Phase 5), traces of in situ burning, ashes, and soil aggregates still indicate agricultural activities, but during later periods (663–551 cal yr BP). These deposits are buried under gravel and light-beige massive silts (Strata 18-23, Facies 3 and 6) (Phase 6). The last dynamic (Phase 7) is composed of 1.25 m of beige bioturbated silts and sands, which correspond to the more recent palm tree environment (Strata 27–31, Facies 4e).

Figure 6. (color online) Terrace 2. View from the east of Test Pit D and zoom on the central cultivated deposits (Phase 4 / 5) with a presentation of the lithostratigraphy (Iron Age Period until today). Facies are described in detail in Table 6.
T2, TP E
Test pit E is located 50 m west of TP C (Fig. 2B) and corresponds to the natural exposure of T2. A total height of 3.3 m of stratigraphy allowed for the identification of four different phases (Fig. 7). Phase 1 is composed of very fine, greenish cemented silts, mottled green/orange in their upper part (Strata 1–4, Facies 2b), on top of which we identified a unique polyhedric to granular brown soil (Stratum 4). These deposits, similar to those in TP C and D, correspond to the less resistant sediments identified in the ERT profile (Fig. 2C). Multiple dates were processed in this soil: microcharcoal were dated from ~920–670 cal yr BP, while an OSL date processed on feldspar grains (no quartz available) indicates probable deposition between 9.23–7.41 ka (Age pIR-225) to 7.07–6.33 ka (Age IR-50). We suspect the recent 14C date to result from contamination along the cross section due to regular burning, and we favor the Neolithic date. Above (Phase 2), we encountered a succession of six dark-brown soils (Strata 4–14, Facies 4b, 4d, and 4e) with two small earthen irrigation canals. Stratum 10 provided a date of 3637–3463 cal yr BP. While this date could be coherent, we suspect that, due to the texture and weak sorting of the deposits, their location close to the surface, chronostratigraphic comparison with other close-by TP, and the total absence of Bronze Age sediments in the palm grove, that these deposits could result from the voluntary reworking and displacement of ancient agricultural sediments to build this terrace. Moreover, OSL dates currently studied at Durham University suggest that the deposition of these sediments might have occurred around the turn of the era (Late Pre-Islamic period; Kennedy, L.S., personal communication, 2016).

Figure 7. (color online) Terrace 2. View from the west of Test Pit E and lithostratigraphy (Neolithic Period until today). Facies are described in detail in Table 6.
Above, Phase 3 corresponds to the digging of a well filled with fine light-grey silts (Strata 19–28, Facies 1b) and backfill deposits on top (Strata 32–49, Facies 5). Phase 4 corresponds to beige bioturbated silts and sands (Strata 50–52, Facies 4e) identified in all the upper parts of the palm grove (Test Pits C and D).
T3, TP F
Test pit F is located 136 m west of TP E, on the western edge of T3 (Fig. 2B). The stratigraphy exposed measures 1.94 m and comprises three superimposed hydraulic structures (Fig. 8). Three phases were identified. Phase 1 is composed of fine greenish to orange cemented silts (Stratum 1, Facies 2a) with traces of soil development on its surface (cf. ERT profile; Fig. 2C). Phase 2 is comprised of irrigation Canals 1– 3. Canal 1 is filled with laminated gravel of harzburgite and serpentinite (Strata 2–7, Facies 2a). Its base was dated from 504–317 cal yr BP. Canal 2 is filled with dark-brown silts (Strata 9 and 10, Facies 4e) and was dated from 540–485 cal yr BP. Canal 3 is much deeper than the previous ones and is filled with fine beige laminated silts and sands. Its base was dated from 471–306 cal yr BP (Stratum 11a) and central part from 473–307 cal yr BP (Stratum 15). Phase 3 is composed of anthropogenic and backfill deposits (Strata 19–25, Facies 5), while the upper part of the TP is comprised of about 60 cm of light-brown gravelly silts (Strata 20, 27, and 28, Facies 4e).

Figure 8. (color online) Terrace 3. View from the west of Test Pit F and lithostratigraphy (Islamic Period). Facies are described in detail in Table 6.
T4, TP G
This very shallow TP, opened less than 50 m west of TP F, on the edge of T4 (Fig. 2B), revealed the presence of fine cemented silts directly at the surface (Strata 1-4, Facies 2 and b; Fig. 9) (Phase 1). A pit was dug in these deposits and was filled with homogeneous blocky dark-brown clay, indicating water stagnation, in which a Late Islamic sherd (550–50 cal yr BP) was found (Phase 2).

Figure 9. (color online) Terrace 4. View from the west of Test Pit G and lithostratigraphy (Late Islamic Period). Facies are described in detail in Table 6.
T5, TP H
Terrace 5 is located at the western end of the palm grove (Fig. 10). Two phases were identified. Phase 1 is composed of laminated gravel and cobbles in a white cemented carbonated matrix. Due to their location close to Wadi Sidji, these deposits correspond to Pleistocene fluvial deposits. Above, gravels in a silty matrix were encountered with no visible and datable material (Facies 4e, Phase 2).

Figure 10. (color online) Terrace 5. (A) View from the west of Wadi Sidji, terrace 5 and the junction between cultivated deposits, (B) Zoom on the ancient cemented fluvial deposits and weakly sorted gravel of Test Pit H (Pleistocene to Late Islamic Period).
INTERPRETATION
The geoarchaeological, geophysical, and spatial study of the oasis of Masafi was used to reconstruct a nearly 20-ka-long landscape record. Chronostratigraphical correlations are presented in Figure 11. Final phases were defined and designated with Roman numerals (Phase I to VII). Diachronic paleogeographical sketches of the oasis are presented in Figure 12.

Figure 11. (color online) Chronostratigraphical correlation between the test pits studied and proposed phasing. Final phases are mentioned in Roman numerals (I to VIII).

Figure 12. (color online) Sketch of the paleo-geographical and hydro-agricultural evolution of the oasis of Masafi from the late Pleistocene to the late Holocene.
Phase I: the “natural” existence of the oasis (18–6.3 cal ka BP; late Pleistocene to mid-Holocene period)
At the center of the palm grove (T2), 5 m below the surface, the base of the TP is composed of nearly 1 m of orange/greenish clayey silts (Phase Ia, Facies 2b). As revealed by the ERT profile, these deposits correspond to the upper part of the fill of a fault depression (Fig. 2C). Their fine texture and homogeneous sorting indicate eolian deposition, while their color is due to an ancient perched aquifer. They can be followed to the west, where they naturally and progressively outcrop. Two distinct preserved paleosoils have been encountered within them. The oldest one (TP D), is composed of well-sorted brown prismatic silty clay with root prints, indicating vegetation development and humid conditions during the late Pleistocene (18210–17620 cal yr BP). This date, however, was obtained on bulk organic matter. We processed a 14C date on charcoal, which provided modern results and was therefore rejected (Table 2). The second paleosoil identified is located at the base of TP E. Composed of dark-brown blocky silty clay with well-defined iron features, this soil was dated between 9.23–7.41 and 7.07–6.33 ka (OSL). Upstream and to the west on T1, the base of TP B is composed of fine, white, and homogeneous silts containing small gravel of serpentinite that have been dated from the Early Holocene (11.15–9.23 ka, OSL). Their fine homogeneous texture and sorting suggest eolian deposits but the local gravel inclusion indicate their secondary removal and their initial existence as sand pockets upstream of the watershed, east of the palm grove.
Late Pleistocene to mid-Holocene deposits are directly overlaid by late Holocene deposits. There is a sedimentary gap between ~6.3 ka and ~3210 cal yr BP (Bronze Age, Phase Ib). To the eastern edge of the palm grove, at a higher elevation on T1, erosion processes could have removed soils or sedimentation rates could have decreased during that period. In the fault depression, we suspect that sedimentary archives are no longer available in situ due to the voluntary displacement of sediments during Phase II, which served as perfect agricultural soils.
Phase II: agricultural expansion and artificializing of the palm grove, wells, and runoff-water harvesting (~3210–2380 cal yr BP; Iron Age period)
The first attested and in situ traces of agricultural development in the oasis reveal the existence of three different hydro-agricultural strategies. First (Phase IIa), tree plantation holes with the exploitation of Ziziphus were identified east of the palm grove on T1 (TP A) and dated from ~3211–2989 cal yr BP (Iron Age I/early Iron Age II). Water was supplied on this high terrace by a well-developed runoff-water channeling system definitely in use between 2847–2752 cal yr BP (Iron Age II). Slightly after that period (Phase II B), around 2720–2380 cal yr BP (Iron Age II), we observe on T2 a major phase of agricultural restructuration and management. Traces of this activity are located about 5 m below the archaeological site of Masafi 1, and erode parts of the Neolithic paleosol of Phase I. This suggests the voluntary digging of the oasis to reach the perched aquifer and exploit it. In TP B, a small drainage canal suggest the voluntary evacuation of excess water. This strategy has been noticed in many modern contexts in the framework of the creation of palm groves (Ruf, T., personal communication, 2015). Drainage waters could have been used downstream for the production of fodder crops (Daoud, Reference Daoud2011). The burnt sediments on top of this drainage structure, identified in TP C and D, are indicators of voluntary swidden agriculture to prepare the palm grove for massive agriculture. Finally, a well was dug directly into these sediments (TP B). As it is not very deep, it also suggests the existence of a nearby aquifer during the Iron Age.
Phase III: abandonment of the oasis and drainage activation (~2380–1860 cal yr BP; Iron Age III and late Pre-Islamic period)
This phase is well attested in Masafi. On T1, the agricultural deposits of Masafi 1 (TP A), as well as the urban and public areas were abandoned and buried under gravel in gullies and sheetflow. On T2, small gullies less than 1 m wide have been encountered in TP D, suggesting a concentrated flow in some areas that eroded the Late Pleistocene paleosoil. In TP C, very well-sorted silty to sandy silts, light-beige to white, occasionally laminated, resemble eolian or temporary abandonment deposits. No traces of gullying have been encountered west of T2, suggesting their removal at a later period.
Phase IV–VI: partial reoccupation and agriculture (~1860–550 cal yr BP; late Pre-Islamic, Sasanian, and Middle Islamic periods)
These three phases are scattered in the oasis. Although there is no archaeological evidence of human occupation during these periods, our findings indicate the contrary. Late Pre-Islamic period deposits dated from after 1858–1874 cal yr BP and prior to 1525–1371 cal yr BP have been identified mainly on T2 (TP C and D, phase IV; Fig. 11 and 12). Light-beige laminated silts indicate the absence of agriculture in the western part of T2 (TP C), while deposits in TP D contain traces of ashes, charcoal, and soil aggregates resulting from bioturbation, soil ploughing, or fertilization. Based on stratigraphic correlation and similar soil signatures, the area around TP E was probably also used at that time. Traces of hydraulic activity have been attested, with the digging of a very large well, filled at its base with light-beige to greyish sediments and slopewash deposits. We suspect that the sediments used during this period correspond to the reworking of soils located west of the palm grove.
The palm grove shows evidence of being in use between 1525–1371 cal yr BP (Sasanian period, TP C and probable TP E based on chronostratigraphic correlations, phase V; Fig. 11). The succession of deposits belonging to Facies 4b suggest soil development and possible agriculture, but their occurrence could also result from more humid conditions associated with vegetation development. The soil composing this terrace could find its origin in the remobilization of ancient soils located initially to the west of the palm grove.
Similarly, the succession of three soils (rich in organic matter and with traces of in situ burning) in TP D indicate a return to agriculture at ~663–551 cal yr BP (Middle Islamic period, phase VI; Fig. 11). Interestingly however, these deposits stand in a lower elevation than the older ones, indicating a phase of voluntary digging in the palm grove, which confirms its probable use during that period. No traces of hydraulic management were noticed however, nor archaeological traces of occupation.
Phase VII: major agricultural expansion, drainage activation, runoff-water harvesting, and falaj irrigation (~ >550 cal yr BP; Late Islamic period)
The Late Islamic period witnesses a major agricultural expansion in Masafi (Phase VIIa). On T3 and 4, agricultural soils directly overly early Holocene deposits. On T3 (TP F), three superimposed irrigation structures dated from ca. ~540–306 cal yr BP suggest the irrigation of the western part of the palm grove. The fills of the irrigation structures, mainly their texture and grading, suggest: the use of runoff harvesting techniques and available water to do so; intense erosion, as indicated by the rapid fill of the hydraulic structures; and the will to irrigate and cultivate all the available areas of the palm grove. Slightly to the west, the pit identified in TP G and also dated from the Late Islamic period suggests the existence of small humid areas or ponds. The discovery of a falaj gallery south of the palm grove, in which a well-preserved sixteenth century vessel was encountered, reveals the exploitation of underground water supplying large areas of the palm grove.
Above these deposits, we encountered 1 m of light-brown silts throughout the oasis (Phase VIIb, TP C–K). Rich in gravel and weakly sorted, they indicate the restructuration of the palm grove. One remaining question is the origin of the sediments, as both the mixed silts and gravel content indicate eolian and local sediments. Two hypotheses can be put forward: (1) the natural erosion of small pockets of sand from the eastern watershed of the palm grove; and (2) the removal and remix of Bronze Age to Islamic soils from the western part of the palm grove, totally deprived of soil to create this artificial landscape. Currently, though, we have noticed that the palm grove is supplied with marine sand.
DISCUSSION
Three questions were raised in the introduction: what was the initial natural environment during the Holocene and when did the oasis system of production appear? How did this system evolve in the framework of climate change? How does this system compare to other landscapes in southeastern Arabia? To answer these questions, unravel the forcing drivers of changes (climatic versus social and regional versus local), and measure the extent to which oases can be used as new environmental records for the Holocene, we relate our results to regional paleoenvironmental records (Fig. 13).
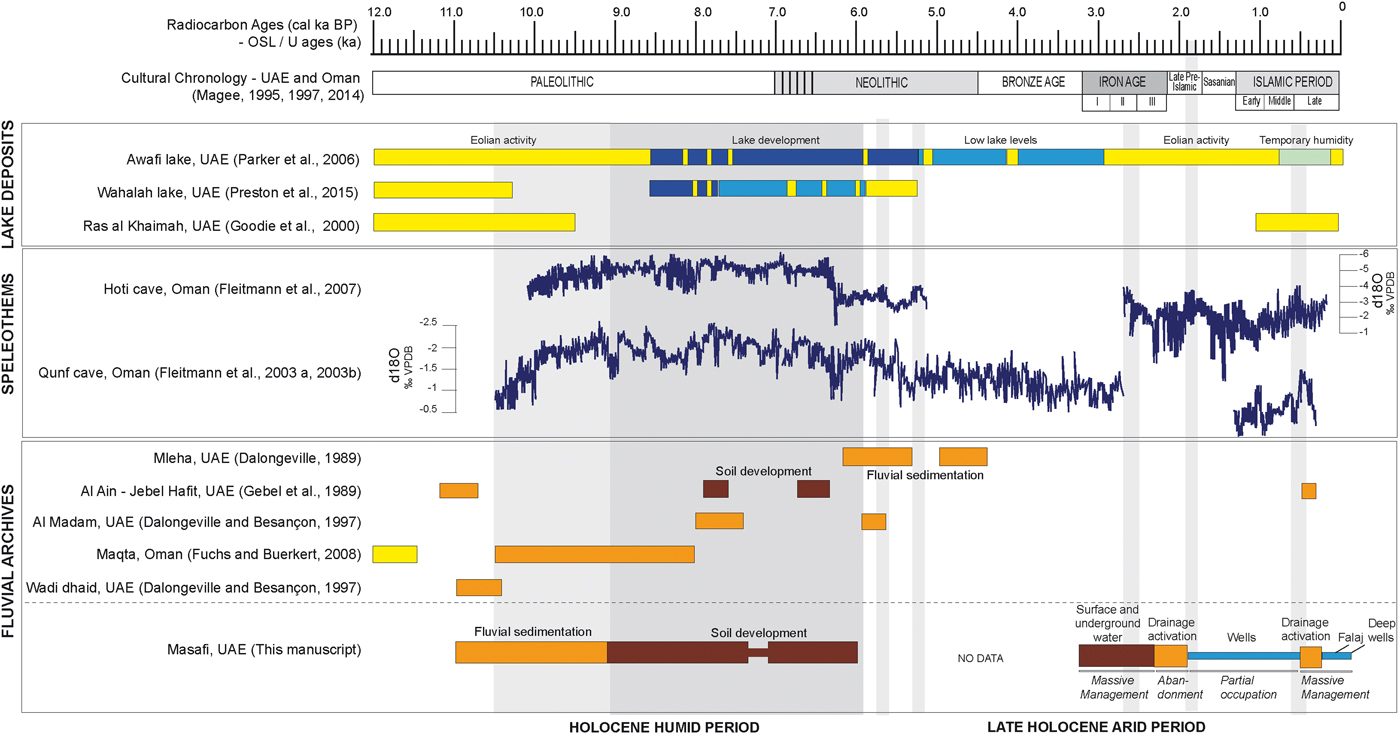
Figure 13. (color online) Comparison of the results obtained in Masafi with regional lacustrine, terrestrial, speleothem, and eolian records in the United Arab Emirates and northern Oman.
A new terrestrial record for the late Pleistocene and Holocene Pluvial period in the Al Hajar Mountains
The existence of a fault depression in the center of the oasis of Masafi provides a new and rare record of environmental conditions during the late Pleistocene and early–middle Holocene period. Research in both Oman and the UAE for the late glacial period indicate arid conditions, reduced precipitation, cessation of speleothem growths (Fleitmann et al., Reference Fleitmann, Burns, Neff, Mangini and Matter2003a, Reference Fleitmann, Burns, Mudelsee, Neff, Kramers, Mangin,i and Matter2003b), and lack of lacustrine sediments (Burns et al., Reference Burns, Fleitmann, Matter, Neff and Mangini2001; Parker et al., Reference Parker, Goudie, Stokes, White, Hodson, Manning and Kennet2006; Fuchs and Buerkert, Reference Fuchs and Buerkert2008). Recent research (Preston et al., Reference Preston, Thomas, Goudie, Atkinson, Leng, Hodson and Walkington2015) suggests that dune accumulation could also reflect reduced wind speed and the progressive transition to more humid periods. Soil development and vegetation growth in Masafi between 18210–17620 cal yr BP do not support these results. New chronological data should be obtained before suggesting that Masafi could have been an isolated case in the Al Hajar mountains.
The early to mid-Holocene period (10.5 to 6.2 ka) is recorded in many lacustrine, fluvial, and speleothem records throughout Arabia. The onset of the Holocene humid period is estimated at ~ 11–10 ka due to monsoonal incursions (Fleitmann et al., Reference Fleitmann, Burns, Mangini, Mudelsee, Kramers and Villa2007; Cheng et al., Reference Cheng, Fleitmann, Edwards, Wang, Cruz, Auler, Mangini, Wang, Kong and Burns2009; Fleitmann and Matter, Reference Fleitmann and Matter2009; Farrant et al., Reference Farrant, Duller, Parker, Roberts, Parton, Knox and Bide2015), increased winter rains from Mediterranean cyclones (Schulz and Witney, Reference Schulz and Whitney1986; al-Farraj, Reference Al-Farraj1995; Enzel et al., Reference Enzel, Kushnir and Quade2015), or the expansion of the African Monsoon over Arabia (Jennings et al., Reference Jennings, Singarayer, Stone, Krebs-Kanzow, Khon, Nisancioglu, Pfeiffer, Zhang, Parker, Parton, Groucutt, White, Drake and Petraglia2015). In southeast Arabia, the oldest fluvial archives have been dated from ~11.1–10.5 cal ka BP on the piedmont of the Al Hajar mountains in the UAE (Gebel et al., Reference Gebel, Hans, Liebau and Raehle1989; Dalongeville and Besançon, Reference Dalongeville, Besançon and Boucharlat1997) and in northern Oman (Fuchs and Buerkert, Reference Fuchs and Buerkert2008). The formation of gravel terraces 20 km west of the oasis of Masafi (Dalongeville and Besançon, Reference Dalongeville, Besançon and Boucharlat1997) is coherent with the phase of drainage activation and soil erosion we identified and dated from 11.1–9.2 ka in Masafi. This confirms an early onset of wetter conditions in the UAE. This occurs in parallel with dune emplacement in the northern UAE between 12.5 and 10 cal ka BP (e.g., Goudie et al., Reference Goudie, Colls, Stokes, Parker, White and Al-Farraj2001; Parker et al., Reference Parker, Goudie, Stokes, White, Hodson, Manning and Kennet2006; Preston and Parker, Reference Preston and Parker2013) and a regional upwelling record of the Arabian sea (e.g., Sirocko et al., Reference Sirocko, Sarnthein, Erlenkeuser, Lange, Maurice and Duplessy1993). Coastal erosion due to higher sea levels formed transgressive dunes that carried carbonate sand inland (Hadley et al., Reference Hadley, Brouwers, Bown, Alsharhan, Glennie, Whittle and Kendall1998 in Parker and Goudie, Reference Parker and Goudie2008). In Masafi, the accumulation of fine eolian carbonated silts on the slopes of the Al Hajar Mountains is in accordance with these processes.
A maximum level of humidity is reached between ~9 and 7 cal ka BP (Berger et al., Reference Berger, Bravard, Purdue, Benoist, Mouton and Braemer2012). In southeast Arabia (UAE, Oman), evidence of the Holocene humid phase is suggested by the development of lacustrine conditions (Parker et al., Reference Parker, Goudie, Stokes, White, Hodson, Manning and Kennet2006; Preston et al., Reference Preston, Thomas, Goudie, Atkinson, Leng, Hodson and Walkington2015), increasing detritism between ~8 and 7.6 cal ka BP (Dalongeville and Besançon, Reference Dalongeville, Besançon and Boucharlat1997) and soil development between ~6 and 5.6 cal ka BP (Gebel et al., Reference Gebel, Hans, Liebau and Raehle1989). Results in Masafi provide a new record of these conditions with the identification of a well-developed paleosoil with probable marshy conditions between 9.2–7.4 and 7–6.3 ka. While we have no evidence of occupation in Masafi, coastal archaeological sites have been discovered in the UAE and Oman. We suspect the oasis to have been a place of circulation at that time.
The onset of regional aridity gradually appeared in northern southeast Arabia around 7.7 cal ka BP (Parker et al., Reference Jennings, Singarayer, Stone, Krebs-Kanzow, Khon, Nisancioglu, Pfeiffer, Zhang, Parker, Parton, Groucutt, White, Drake and Petraglia2015) up until 5.9 cal ka BP (Neff et al., Reference Neff, Burns, Mangini, Mudelsee, Fleitmann and Matter2001; Uerpmann, Reference Uerpmann, Potts, Naboodah and Hellyer2003; Parker et al., Reference Parker, Eckersley, Smith, Goudie, Stokes and Ward2004, Reference Parker, Goudie, Stokes, White, Hodson, Manning and Kennet2006). Increased eolian activity (Goudie et al., Reference Goudie, Colls, Stokes, Parker, White and Al-Farraj2000; Atkinson et al., 2001; Parker and Goodie, Reference Parker and Goudie2008) and cessation of speleothem growth in northern Oman between ~6.3–5.2 ka (Fleitmann et al., Reference Fleitmann, Burns, Neff, Mangini and Matter2003a, Reference Fleitmann, Burns, Mudelsee, Neff, Kramers, Mangin,i and Matter2003b) have been widely attributed to the southward retreat of the monsoon rain belt (Neff et al., Reference Neff, Burns, Mangini, Mudelsee, Fleitmann and Matter2001) with a decline in summer rainfall and the shift to dominant northern moisture (Fleitmann and Matter, Reference Fleitmann and Matter2009). Interestingly, these events occurred simultaneous to the progressive decline of human presence in Arabia for nearly a millennium beginning around 5800 cal yr BP. During this “Dark Millennium” (Uerpmann, Reference Uerpmann, Potts, Naboodah and Hellyer2003), populations migrated to the southern coasts of Oman (Biagi, Reference Biagi1994). In southeast Arabia (UAE), fluvial archives suggest drainage activity on the piedmont of the Al Hajar mountains until ~4.2 cal ka BP. Lacustrine deposits (Parker et al., Reference Parker, Goudie, Stokes, White, Hodson, Manning and Kennet2006) do indicate decreasing lake level around ~5.2 cal ka BP, but they persist at lower levels until ~3.0 cal ka BP. In Masafi, we have a sedimentary gap during the mid-Holocene shift to aridity and early portion of the late Holocene, between ~6.3–3.2 cal ka BP (Neolithic and Bronze Age). Archaeological evidence of permanent occupation starting at ~ 4–3.6 cal ka BP (Table 1, Masafi 4), however, indicates the presence of farmers and local resource availability. We suspect erosion or the voluntary displacement of sediments during later periods to have removed sedimentary archives dated from that period. Further studies in Masafi might allow us to fill this gap but other oases should be also be studied for comparison.
An environmental and human record for the late Holocene arid period
Evidence of climate conditions during the mid–late Holocene is very scarce, but most records show regional drying beyond ~4.2 ka. Indirect data are provided by an increasing number of archaeological sites and climate data can be obtained from speleothems in Oman and marine cores from the Indian Ocean. Masafi provides a new terrestrial record of the hydro-sedimentary dynamics that have prevailed for the last three millennia in southeast Arabia, from ~3.2 cal ka BP to today. Between ~3.2–2.3 cal ka BP, archaeological data record a real increase in settlement patterns in various ecological zones (Benoist, Reference Benoist2000; Magee, Reference Magee2014). The establishment of a socioeconomic system structured around agricultural production and the export of copper ingots from the Al Hajar Mountains to the Near East and Indus Valley (Benoist, Reference Benoist2000), could explain a renewed occupation. Two major technological innovations could have at least partly contributed to this demographic increase: the domestication of the camel around 1200 BC and the introduction (or development) of underground water supply as a response to decreasing resources (al-Tikriti, Reference Al-Tikriti2002; Córdoba and Del Cerro, Reference Córdoba and Del Cerro2005; al-Tikriti, Reference Al-Tikriti and Avanzini2010; Córdoba, Reference Córdoba2013). Results obtained in the oasis of Masafi and presented in this manuscript and in recently published papers (Charbonnier et al., Reference Charbonnier, Purdue and Benoist2017a, Reference Charbonnier, Purdue, Calastrenc, Régagnon, Sagory, Benoist, al-Tikriti and Yule2017b) suggest runoff-water channeling and a high, exploitable, and drained aquifer between ~3–2.5 cal ka BP (Iron Age). While we suspect farmers to have lowered their gardens to exploit this groundwater, maybe as a result of reduced surface water around ~2.7 cal ka BP, this suggests available water and precipitation in the Al Hajar mountains despite regional aridification. Indirect evidence of similar conditions at Al Ain is provided by al-Tikriti (Reference Al-Tikriti2017), who also suggests a higher ground-water level during that period. After ~2.6 cal ka BP and until 2.3 cal ka BP (Iron Age I), a majority of sites, Masafi included, are abandoned (Benoist, Reference Benoist2000), possibly as a result of regional decreasing resources (Del Cerro and Córdoba, 2005; Mouton, Reference Mouton and Avanzini2010; Córdoba, Reference Córdoba2013) and/or conflicts between groups, as suggested by traces of major fire events or weapons (Benoist, Reference Benoist2000, Reference Benoist2013). In Masafi, we witness a period of concentrated and sheetflow deposition between ~2.3–1.8 cal ka BP, probably following the abandonment of the hydraulic system and indicating drainage activation and soil erosion. These wetter conditions reveal that water depletion is unlikely to explain the abandonment of Masafi, which is in contradiction with previous studies that stressed the role of ground-water drawdown and water depletion in the desertion of Iron Age settlements (Boucharlat, Reference Boucharlat, Potts, Al Naboodah and Hellyer2003; Córdoba and Del Cerro, Reference Córdoba and Del Cerro2005; Córdoba, Reference Córdoba2013). This understated period of humidity framed between ~ 3.2–1.8 cal ka BP, with a phase of increased detritism between ~2.3–1.8 cal ka BP, is partly recorded in speleothems between ~ 2.7–2.4 ka (Fleitmann et al., Reference Fleitmann, Burns, Neff, Mangini and Matter2003a, Reference Fleitmann, Burns, Mudelsee, Neff, Kramers, Mangin,i and Matter2003b) and at ~1.8–1.7 ka in marine pollen records (Gupta et al., Reference Gupta, Anderson and Overpeck2003). This short and poorly recorded period of humidity would be related to a short-term intensification of winter rainfall in our area of study, while a moderate instability of the Southwest monsoon could explain the more meridional signatures (Chauhan et al., Reference Chauhan, Singh, Hananto, Carton, Klingelhoefer, Dessa, Permana, White and Graindorge2009).
Following this period, between ~1.8–0.5 cal ka BP, data on oasis economy, resource availability, and climate change are even more scattered in southeast Arabian. This period of time coincides with a record of precipitation minimum in the northeastern Arabian sea (von Rad et al., Reference von Rad, Schaff, Michels, Schulz, Berger and Sirocko1999 in Parker and Goudie, Reference Parker and Goudie2008), a cessation of speleothem growth (Fleitmann et al., Reference Fleitmann, Burns, Mudelsee, Neff, Kramers, Mangin,i and Matter2003b) and major dune sand accumulation at ~1 ka in the UAE (Goudie et al., Reference Goudie, Colls, Stokes, Parker, White and Al-Farraj2000; Stokes et al., Reference Stokes, Bray, Goudie, Wood, Alsharhan and Wood2003). Between ~1.8–1.6 cal ka BP (late Pre-Islamic period) and ~1.6–1.2 cal ka BP (Sasanian period), only a couple of sites are known to have been occupied north of the Al Hajar Mountains (e.g. Mouton, Reference Mouton and Avanzini2010; Kennet, Reference Kennet, Potts and Hellyer2013). The economic foundation seems to have been agriculture (dates, cereals, and fruit trees) and long-distance trade through the caravan road. Similarly, we only suspect the presence of villages, field systems, and falaj between 1.2–0.5 cal ka BP (Early to Middle Islamic period), despite a regional boom in Indian trade related to agriculture and mining (Potts, Reference Potts and Potts2012). In Masafi, the lack of settlements for these three periods but evidence of hydro-agricultural management does suggest human occupation. The scale of this activity is unknown, however. Masafi probably benefited from more resources than other sites and could have played the role of a refugium during that period.
Regional archives are more abundant after 550 cal yr BP. Slightly more humid conditions are recorded between 550–450 yr in speleothems (Fleitmann et al., Reference Fleitmann, Burns, Neff, Mangini and Matter2003a, Reference Fleitmann, Burns, Mudelsee, Neff, Kramers, Mangin,i and Matter2003b) and in δ18O measurements and pollen records from marine cores in the Indian Ocean between ~ 650–450 yr (e.g., Gupta et al., Reference Gupta, Anderson and Overpeck2003; Chauhan et al., Reference Chauhan, Singh, Hananto, Carton, Klingelhoefer, Dessa, Permana, White and Graindorge2009; Miller et al., Reference Miller, Leroy, Collins and Lahijani2016). Other records, however, indicate that areas located close to the ITCZ northern limit became drier as a result of cooling waters (e.g., Fleitmann et al., Reference Fleitmann, Burns, Neff, Mudelsee, Mangini and Matter2004). Terrestrial records in the UAE do suggest wetter conditions and drainage activation (Gebel et al., Reference Gebel, Hans, Liebau and Raehle1989). We also have records of alluvial fan activation at ~500 cal yr BP in Masafi, based on irrigation-canal fills composed of surface deposits. While this indicates the exploitation of available runoff water and precipitation, the progressive and first development of falaj irrigation around the sixteenth to seventeenth century AD could point to a progressively decreasing ground-water level. This major technological investment is indicative of perennial occupation. This is in accordance with a regional agricultural expansion (export of dates to India; Mershen, Reference Mershen2001; Power and Sheehan, Reference Power and Sheehan2012), which occurred after the Portuguese conquest and the establishment of an Omani Empire. This could also explain the progressively decreasing resources we witness in Masafi and the investment in electric pumping during the mid-twentieth century.
CONCLUSION
This study of the Masafi palm grove in the UAE is one of the first that simultaneously provides information on sedimentary dynamics, erosion processes, resource management, and environmental change in the Al Hajar Mountains from the late Pleistocene to the late Holocene. The results allow us to identify and describe the natural environment of the oasis during the Holocene and the appearance of the oasis system of production around 3250 cal yr BP. In parallel with climate change, this system evolved with cycles of intensive agriculture (investment in cash crops) versus seasonal or opportunistic cropping. The sedimentary record suggests that the oasis was a humid area during the late Pleistocene–early Holocene periods. Eolian sedimentation and increased erosion during the middle Holocene supplied soils for future agriculture. During suspected arid periods, Masafi provided evidence of available surface water and perched aquifers (~3210–2380 cal yr BP), while increased erosion and reactivation of alluvial fans was recorded between ~2380–1870 cal yr BP and after ~550 cal yr BP. Our results indicate decreasing water availability through time, but not necessarily drier conditions during the Holocene, as has always been thought to be the case in the area. Moreover, the occurrence of surface water and its exploitation up to the twentieth century in the palm grove do suggest a regular water supply despite global climatic change. Compared to other landscapes in Arabia, the oasis of Masafi seems to have developed independently from regional climatic trends, taking advantage of local conditions, such as orogenic precipitation, in periods of regional adverse climatic conditions. While oases are resilient witnesses of socio-environmental changes and key recorders of human adaptations and practices, more studies throughout Arabia and the Al Hajar Mountains will allow us to determine if Masafi is a unique case and has acted as a refugium for Arabian herders and farmers from the Neolithic onwards.
ACKNOWLEDGMENTS
The authors would like to acknowledge the Fujairah Tourism and Antiquities Authority, and especially Ahmed al-Shamsi, Saeed al-Semahi, and Salah Ali Hassan for their support, which enables us to carry out our study of the Masafi oasis. We would also like to thank the French Ministry of Foreign Affairs and International Development (MAEDI) for its financial support as well as the French Agence National de la Recherche (ANR), under grant ANR-16-CE27-0008 (Project OASIWAT). We deeply thank Frank Preusser (University of Freiburg, Institute of Earth and Environmental Science) and Jean-Luc Schwenniger (Luminescence Dating Laboratory, University of Oxford) for their collaboration and advice on the OSL dating. Last, we thank Dr. T. Faith and two anonymous reviewers for their comments and help to improve this paper.