Compared with north American shale oil, most shale oil systems in China are developed in continental lacustrine systems, especially in saline lacustrine sedimentary systems. It is generally believed that the saline lake basin is ‘restricted to life’ due to its high salinity and harsh environment. However, some studies have shown that saline lakes can also develop complex biological systems (Hammer Reference Hammer1986). Lake biological species change with the change of salinity. With the increase of salinity, the number of biological species decreases, but the biomass does not necessarily decrease. As a result, high productivity could be a common characteristic of saline lakes. Therefore, the formation of high-quality saline lake source rocks provides the basis for many large oil and gas fields in the world (Amils et al. Reference Amils, Ellis-Evans and Hinghofer-Szalkay2007; Cangemi et al. Reference Cangemi, Censi, Reimer, D'Alessandro, Hause-Reitner, Madonia, Oliveri and Pecoraino2016).
Jianghan Basin is an important saline lake oil-bearing basin in eastern China. A lot of shale oil has been produced from the inter-salt shale of Qianjiang Formation, which is composed of more than 160 alternate layers of salt and shale. Generally, each rhythm is a ‘salt-shale-salt’-type self-sourced and self-reservoired shale oil system. However, not all inter-salt shale layers are organic-rich shales, and only those organic-rich shale strata are likely to be favourable conditions for shale oil exploration. The study on the formation model of organic-rich shale in a saline lake environment is helpful to the exploration of continental saline lake shale oil.
Currently, research concerning the organic geochemistry and formation model of the inter-salt organic-rich shales is quite sparse. Further detailed studies of these deposits is of great significance for the accurate estimation of shale oil resources in Jianghan Basin and for integration into more widely applicable models for the genesis of lacustrine shale oil to accurately estimate shale oil resources in Jianghan Basin and integrate them into a more widely applicable lacustrine shale oil genetic model. In this study, we present total organic carbon (TOC), mineral composition, major and trace element and biomarker characteristics of the inter-salt shale. The primary goal of this paper is to provide geochemical data on the inter-salt shale, and to propose a hypothesis for its formation in order to achieve a better understanding of the shale oil system.
1. Geological background
Jianghan Basin is a saline lake basin in eastern China. As the tectonic subsidence and depositional centre of Jianghan Basin, Qianjiang Depression is the most oil-bearing depression in Jianghan Basin (Fig. 1). The main source rocks and reservoirs are in Qianjiang Formation, which is a most significant oil-producing reservoir in Jianghan Basin (Zhang et al. Reference Zhang, Wang, Yang and Qi2005).
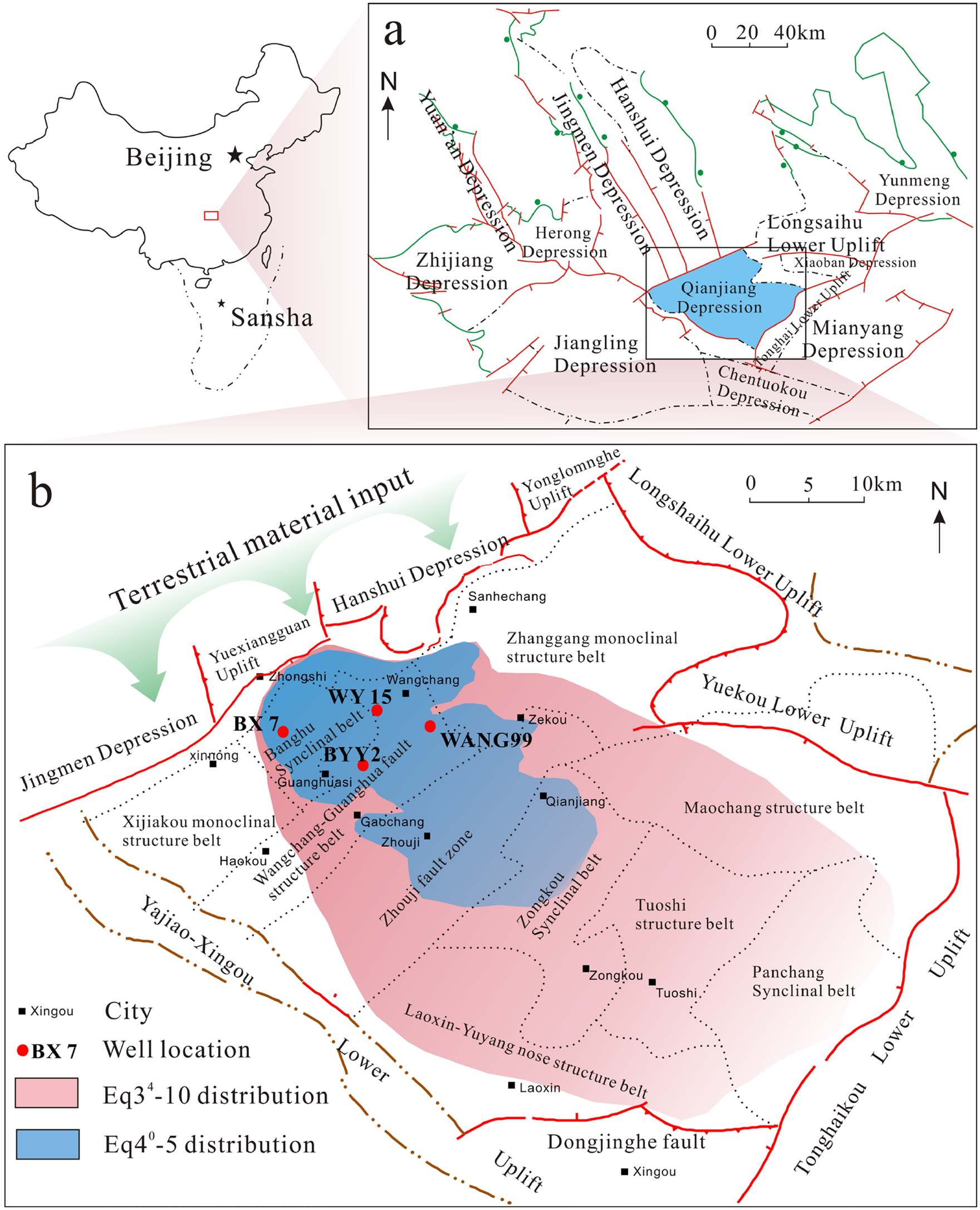
Figure 1 Tectonic map of the Qianjiang Depression with sampling locations. (a) Geological structure of Jianghan Basin; (b) Geological structure of Qianjiang depression.
The Qianjiang Depression is filled with about 6000 m of Cenozoic sediments, and the Eocene is the main subsiding stage with the salt-rich formation deposited in the Qianjiang Formation (Eq). The Qianjiang Formation consists of more than 160 cyclothems of ‘salt-shale/sandstone-salt’, and is divided into four members from the top to bottom: Eq1, Eq2, Eq3 and Eq4 (Fig. 2). Generally, within each rhythm, salt formation on the bottom and inter-salt shale consist of a mixture of fine-grained siliciclastic, carbonate, sulphate and salt (Fang Reference Fang2006), and on the top, make a ‘salt-shale-salt’-type self-sourced and self-reservoired shale oil system (Fig. 2).

Figure 2 The lithological characteristics of the inter-salt shales from Qianjiang Formation.
Currently, with a high TOC content and shale oil content, the tenth cyclothem of the Eq34 inter-salt shale (Eq34-10) is the most important target layer for shale oil exploration in the Qianjiang Depression. The fifth cyclothem of the Eq40 inter-salt shale (Eq40-5) shows some similar petrological characteristics with Eq34-10, but lower TOC content and oil content.
2. Samples and experiments
2.1. Samples
Sixty-five core samples were collected from Eq34-10 rhythmic shale of well Wang 99, and 57 core samples were collected from Eq40-5 rhythmic shale of well WY15 (Fig. 1b). The mineral composition, main and trace elements and TOC of all samples were analysed. Some additional core samples of inter-salt shale from other wells were collected for biomarker studies.
2.2. Mineral composition
A small part of each samples, which had been grounded to less than 200 mesh powder, were used to determine the mineralogical composition by X-ray diffraction.
2.3. TOC
The TOC contents were determined by infrared spectroscopy using a CS 230, after removing the carbonates by treatment with hydrochloric acid at 60 ± 5 °C.
2.4. Major and trace element
Major and trace elemental analyses were carried out using inductively coupled plasma-optical emission spectrometry and inductively coupled plasma-mass spectrometry, respectively. Please refer to Wei et al. (Reference Wei, Wang, Wang, Zhang, He and Ma2020) and Wang et al. (Reference Wang, Wang, Wei, He and Ma2020) for the detailed experimental process.
2.5. Gas chromatography–mass spectrometry (GC–MS)
Twenty-five core samples from Eq34-10 shale and 17 core samples from Eq40-5 shale were collected, and were grounded into 100 mesh powder. Then, the powdered samples were extracted with chloroform for 72 h using a Soxhlet apparatus. Silica gel column chromatography was used to separate the extracts. The saturates were obtained by eluting with n-hexane and then analysed by 6890N GC–5975N MS. The GC was equipped with a HP-5 capillary column (30 m × 0.32 mm i.d., 0.25 μm film thickness). The GC oven temperature was raised from 50 °C to 300 °C at 4 °C min−1 and then held at 300 °C for 30 min. The carrier gas was helium. MS conditions were electron ionisation at 70 eV with an ion source temperature at 250 °C.
2.6. Statistical analysis
In this paper, IBM SPSS Statistics 22 was hired to perform the R-mode cluster analysis. The Pearson correlation coefficient method of R-mode hierarchical cluster analysis was chosen to study the relationship between trace elements.
3. Results
3.1. Mineral composition
The mineral composition of inter-salt shale is very complex, shows great heterogeneity and is always interbedded or filled in fracture with gypsum, anhydrous glauberite, glauberite and halite (Li et al. Reference Li, Chen, Cao, Ma, Liu, Li, Jiang and Wu2018a). Hence, several samples with high sulphate mineral content were excluded when calculating the average mineral composition and major oxides. The main mineral composition of the Eq34-10 rhythmic shale includes clay (31.02 %), quartz (12.08 %), feldspar (3.47 %), calcite (11.56 %), dolomite (32.00 %), glauberite (4.87 %) and pyrite (3.17 %). The main mineral composition of the Eq40-5 rhythmic shale includes clay (14.54 %), quartz (7.97 %), feldspar (18.02 %), calcite (16.09 %), dolomite (33.13 %), glauberite (2.03 %), anhydrite (3.26 %), gypsum (0.72 %) and pyrite (3.47 %). The values in parentheses are the averages of mineral content of the rest samples, and the mineral compositions of each sample are provided in online Appendix.
3.2. TOC
Table 1 lists the TOC of the Eq34-10 rhythmic shales and Eq40-5 rhythmic shales. TOC of the Eq34-10 rhythmic shale ranges from 0.25 % to 2.94 %, with an average of 1.51 %, and for Eq40-5 rhythmic shale, TOC ranges from 0.20 % to 1.57 %, with an average of 0.72 %. Generally, Eq34-10 rhythmic shale has a higher TOC than Eq40-5 rhythmic shale. In addition, TOC of the lower shale is generally less than the upper shale for both Eq34-10 rhythmic shale (Fig. 3) and Eq40-5 rhythmic shale (Fig. 4). Abnormally low TOC in several samples is closely related with the sulphate mineral content due to the dilution effect of sulphate mineral increase on TOC.

Figure 3 The mineral content and TOC of Eq34-10 rhythmic inter-salt shale from well Wang99.

Figure 4 The mineral content and TOC of Eq40-5 rhythmic inter-salt shale from well WY15.
Table 1 Correlation coefficients of the relationships between various element concentrations of Eq34-10 rhythmic shale. Abbreviations: Lithuim (Li), Beryllium (Be), Vanadium (V), Chromium (Cr), Cobalt (Co), Nickel (Ni), Germanium (Ge), Yttrium (Y), Niobium (Nb), Cesium (Cs), Tin (Sn), Hafnium (Hf), Tantalum (Ta), Tungsten (W), Lead (Pb), Bismuth (Bi), Thallium(TI), Thorium (Th), Uranium (U), Rubidium (Rb).

3.3. Major elements
The results of major oxides and trace elements concentrations of the shale samples and several widely used geochemical ratios are presented in online Appendix. The major oxides calcium oxide (CaO) and aluminium oxide (Al2O3) are the dominant constituents, with an average content of 16.52 % and 9.60 % for Eq34-10 rhythmic inter-salt shales, and 15.20 % and 9.39 % for Eq40-5 rhythmic inter-salt shales, respectively. Other major oxides such as potassium oxide (K2O), sodium oxide (Na2O), magnesium oxide (MgO), iron oxide (Fe2O3), phosphorus oxide (P2O5) and manganese oxide (MnO) are also present in low concentrations (online Appendix). The data show a large standard deviation of major oxides. This indicates that the terrigenous saline lacustrine shale is highly heterogeneous and complex in mineral composition and diagenesis.
3.4. Trace elements
The factors that affect the abundance of trace elements in sediments are numerous and complex (Bach & Irber Reference Bach and Irber1998). The trace element contents of each sample are provided in online Appendix. R-mode hierarchical cluster analysis can distinguish these complex factors and clarify the similarities and differences in different elements of Eq34-10 rhythmic shales and Eq40-5 rhythmic shales. Figure 5 shows the results of cluster analysis, and the correlation coefficients between each other are shown in Tables 1 and 2. Basically, the trace elements can be divided four major groups (Fig. 5).
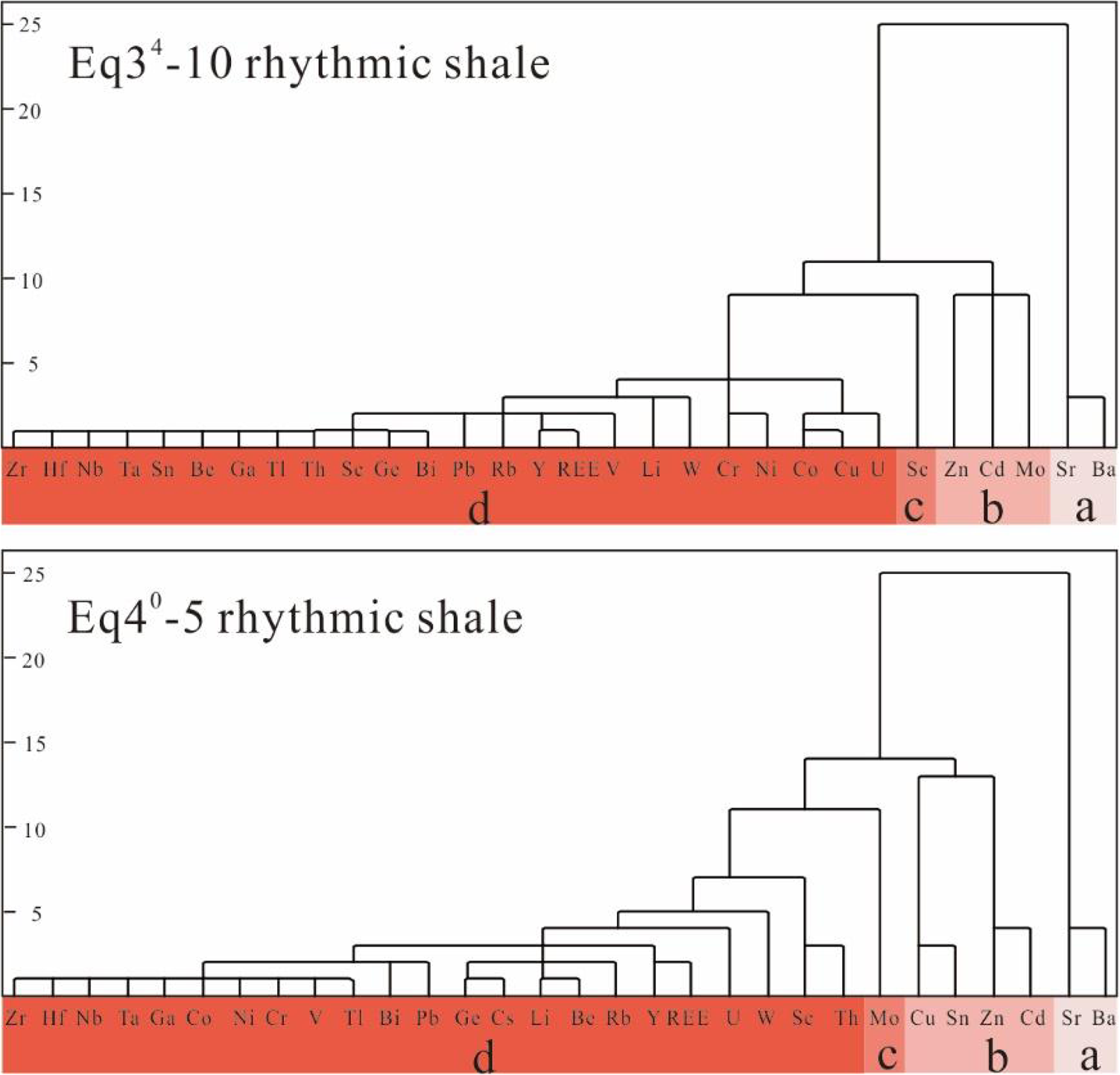
Figure 5 R-model hierarchical cluster analysis of trace elements.
Table 2 Correlation coefficients of the relationships between various element concentrations of Eq40-5 rhythmic shale.

The first group (a) includes two typical large-ion lithophile elements strontium (Sr) and barium (Ba), which is representative of the chemical deposition of saline lakes. The second group (b) and third group (c) includes molybdenum (Mo), zinc (Zn), cadmium (Cd) and scandium (Sc) for Eq34-10 rhythmic shales, Mo, Zn, Cd, tin (Sn) and copper (Cu) for Eq40-5 rhythmic shales. These elements are mainly affected by palaeoclimate, sedimentation processes and biogenic substance flux. The fourth group (d) includes a majority of high field-strength elements. These elements are less affected by geochemical action and mainly related with terrigenous detrital. Obviously, the first, second and third types of elements, such as Ba, Sr, Cd, Zn, Sn, Cu, Mo and Sc, are greatly influenced by the sedimentary processes of saline lakes. These elements are effective media for studying the palaeoenvironmental conditions of saline lakes.
4. Discussion
4.1. Origin of organic matter
The distribution pattern of n-alkanes in the inter-salt shale from the Eq34-10 cyclothem shows a similar characteristic. The carbon numbers of n-alkane mainly range from C 13 to C 35, and show bimodal distribution patterns with maximum abundance at C 18 and C 24 (or C 22) (Table 3; Fig. 6). The C 21−/C 21+ ratios are in the range of 0.94–1.46, showing a weak predominance of the medium-long-chain n-alkanes. Previous studies have shown that the chain length of n-alkanes in algae usually ranges from 11 to 25 in carbon number (Meyers & Ishiwatari Reference Meyers and Ishiwatari1993), and the chain length of n-alkanes in underwater aquatic plants and floating plants reaches its maximum value at C 21, C 23 or C 25 (Ficken et al. Reference Ficken, Li, Swain and Eglinton2000). The distribution characteristics of n-alkanes indicate that the source rock has a mixed source of algae and submerged aquatic plants and/or floating plants. In addition, the distribution characteristics of regular steranes have also been widely applied to indicate the source of organic matter. The relative abundance of C 27, C 28 and C 29 5α20R steranes are in the range of 42.45–49.99 %, 16.01–22.31 % and 31.74–41.14 %, respectively (Table 3). This suggests a mixed organic matter source of algal, plankton, bacterial and land plants, with the former three being dominant (Fig. 7).

Figure 6 Representative chromatograms of biomarkers identified in the inter-salt shale.
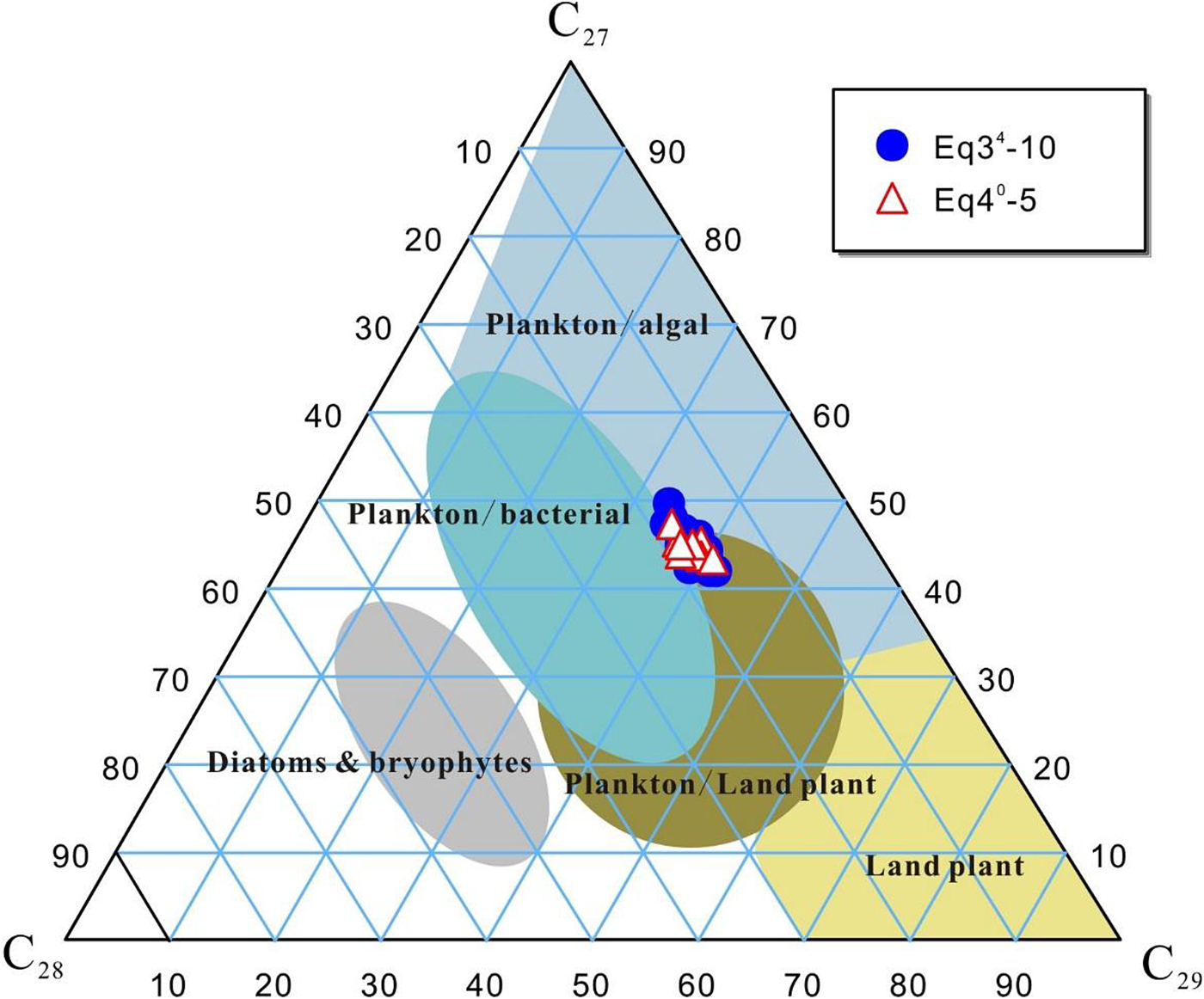
Figure 7 Ternary diagram of regular steranes (C 27–C 29 5α20R) showing the relationship between steranes compositions and source organic matter input.
Table 3 Biomarker characteristics of inter-salt shale from Qianjiang Formation.

Therefore, the organic matter in the inter-salt shale was predominantly derived from endogenous lower aquatic organisms, algae and bacteria. In other words, the conditions that affect the development of lake organisms and the preservation of organic matter are the key factors that control the development of organic-rich shale.
4.2. Palaeoclimate
The ratios of Rb/Sr and Sr/Cu were extensively hired to infer the palaeoclimate. Generally, a hot arid climate leads to low Rb/Sr and high Sr/Cu ratios (>5), while a humid climate leads to high Rb/Sr and low Sr/Cu (1.3~5) (Lerman Reference Lerman1978). Rb/Sr ratios of Eq34-10 rhythmic shales and Eq40-5 rhythmic shales range from 0.002 to 0.368, with an average of 0.107, and from 0.002 to 0.523, with an average of 0.131 (Table 4), respectively – far below the value of post-Archean Australian shale (PAAS) (~0.8) (Taylor & McLennan Reference Taylor and McLennan1985). The Sr/Cu ratios of inter-salt shale samples vary greatly, ranging from 8.03 to 3340.34 with an average of 228.41 for Eq34-10 rhythmic shales, and from 3.94 to 1723.33 with an average of 136.53 for Eq40-5 rhythmic shales, respectively (Table 4). These values suggest a hot arid palaeoclimate.
Table 4 Geochemical index characteristics of inter-salt shale from Qianjiang Formation.

The chemical properties of rare earth elements (REEs) are stable, and the REEs in sediments are mainly inherited from terrestrial clastic materials. Therefore, REEs are usually regarded as terrigenous clastic proxies (Chang et al. Reference Chang, Chu, Feng, Huang and Zhang2009). The Sr/Cu ratio is negatively correlated with REE (Fig. 8a), and the Rb/Sr ratio is positively correlated with REE (Fig. 8b). This indicates that the palaeoclimate controlled the relative amount of terrestrial source material input in the lakes, and there was more terrestrial clastic material input in a warm and humid climate, and less terrestrial clastic material input in a hot arid climate. In addition, REE is negatively correlated with Sr/Ba, the salinity index of ancient lakes (Fig. 8c). These results indicate that palaeoclimate, terrestrial material input and palaeolake salinity are coupled with each other. During the hot arid climate, the amount of terrestrial detrital material input decreases and the salinity of lake water is relatively low.

Figure 8 Relationship between trace element palaeoclimate (Sr/Cu, Rb/Sr and Ga/Rb) and palaeosalinity proxies (Sr/Ba) and REE.
Generally, gallium (Ga) is mainly related to the kaolinite, reflecting a warm and humid climate, while Rb is related to illite, indicating weak chemical weathering and related to a dry and cold climate (Roy & Roser Reference Roy and Roser2013). Therefore, with the cooling and drying of the climate, the Ga/Rb ratio in sediments tends to decline (Roy & Roser Reference Roy and Roser2013). However, without a correlation diagram, there is no obvious correlation between the Ga/Rb ratios and REE (Fig. 8d) or other palaeoclimate proxies. Cluster analysis shows that Ga belongs to the fourth group of elements, which are less affected by geochemical action and mainly related with terrigenous detrital. Therefore, the Ga/Rb ratio is not suitable for evaluating the palaeoclimate of terrigenous saline lacustrine.
Many studies have shown that the δ18O value of authigenic carbonate in the lake is mainly determined by the isotopic composition of the water (Chen, Reference Chen2007). Last (1990) believed that the dolomite formed in the restricted saline lake environment was mainly affected by strong evaporation, and this kind of dolomite has a higher δ18O value. Chen (Reference Chen2007) further emphasised that evaporation is the most important factor to change the carbon isotopic composition of lake water. As shown in Figure 9, the δ18O value of carbonate has a significant positive correlation with the content of dolomite, indicating that the dolomite was formed in the closed lake system under the condition of intense evaporation of water. The increase of dolomite content indicates the enhancement of evaporation and increased salinity of water, which is also closely related to a hot arid climate.

Figure 9 The relationship between dolomite content and the δ18O value of carbonate.
4.3. Source weathering
The Chemical Index of Alteration (CIA) can effectively indicate the degree of feldspar weathering clay minerals in samples, and has been widely used to estimate the source areas weathering processes. CIA is calculated based on the molar proportions of the oxides:

where CaO* represents only the silicate-related CaO content, not carbonate and phosphate. McLennan (Reference McLennan1993) assumed logical Ca/Na ratios of 1 in silicate material. Considering that the CaO content is much higher than Na2O, CaO* is assumed to be equivalent to Na2O in the present study. Except for the abnormal data, CIA values range from 39.05 to 58.49 and from 39.18 to 53.98, with an average of 51.27 and 46.03 for two sets of shale, respectively (Table 4). This indicates a low degree of palaeoweathering conditions in the source rocks during the deposition of Eq34-10 and Eq40-5 rhythmic shales (Fig. 10). In addition, it seems that there were relatively higher palaeoweathering conditions during the deposition of Eq34-10 rhythmic shales than for Eq40-5 rhythmic shales.

Figure 10 Ternary plots of Al2O3-(CaO + Na2O)-K2O (A-CN-K) showing the relationship between mineral composition and degree of chemical weathering.
4.4. Palaeosalinity
Gammacerane is the most common biomarker in salinised sedimentary environments, and its content is often positively correlated with salinity. C 30+ hopanes in common with gammacerane may also be controlled by the salinity of the environment (Duan et al. Reference Duan, Zheng, Wang, Wu, Wang and Zhang2006; Wang et al. Reference Wang, Zheng, Chen, Xie and Sun2017). High relative abundances of gammacerane and C 30+ hopane have been detected in the shale samples, and the gammacerane/αβ-C30 hopane ratio ranges from 1.38 to 3.68. The relative abundance of C 30+ hopane is 41.69–60.15 % (Table 3). This indicates that the shale deposited under a high-salinity, intense reductive environment.
In addition, the C 35/C 34 hopane ratio is usually positively correlated with the salinity of sedimentary water. Figure 11 shows a relative high value of C 35/C 34 hopane ratio on the bottom of two sets of inter-salt shale, and a relative low value of C 35/C 34 hopane ratio on the top of two sets of inter-salt shale, which is exactly the opposite of the distribution characteristics of TOC. This indicates that the salinity of the palaeolake corresponding to the two sets of shale tends to decrease, and high salinity corresponds to low TOC, and low salinity to high TOC. In addition, the C 35/C 34 on the top of Eq34-10 rhythmic shales is higher than that of Eq40-5 rhythmic shales (Fig. 11). It seems that the salinity during the deposition of Eq34-10 rhythmic shales is relatively lower than for Eq34-10 rhythmic shales. This may reveal the fact that the palaeoproductivity of lakes in a high-salinity water environment is low, and the reduction of water salinity is conducive to the survival of lake organisms, thus increasing the productivity.

Figure 11 Vertical distribution characteristics of TOC and C 35/C 34 hopane.
Sr and Ba have similar chemical properties and are separated by different geochemical behaviours under different sedimentary environments. As the salinity of water increases, Ba will precipitate first in the form of carbonate and sulphate. As the salinity of water continues to increase, Sr will also precipitate. Therefore, Sr abundance and Sr/Ba values are positively correlated with palaeosalinity, and can be used as sensitive indicators for palaeosalinity discrimination during sediment deposition (Ma & Wen Reference Ma and Wen1991). It is generally believed that a Sr/Ba value of less than 1 means freshwater deposition, while a Sr/Ba value greater than 1 means saline environment (Xu et al. Reference Xu, Liu, Guo, Li, Huang, Zhao, Pan and Zhang2018). Both sets of shale have similarly high contents of Sr and Ba (Appendix 1), and the Sr/Ba values are generally above 1, with an average of 4.08 and 4.22 for Eq34-10 and Eq40-5, respectively (Table 4). These values indicate a high salinity lake during two sets of shale deposition. In addition, the Sr/Ba ratio is negatively correlated with REE (Fig. 8c), which represents the input of terrigenous clastic in the present study. Therefore, the salinity of the palaeolake decreases with the increase of the input of terrigenous clastic materials.
4.5. Depositional conditions and redox proxies
Generally, the pristane/phytane (Pr/Ph) ratio below 1 represents typical hypoxia conditions, usually hypersaline or carbonate environments, and values above 1 reflect oxidation conditions (Haven et al. Reference Haven, Leeuw, Rullkötter and Damsté1987). In this study, the Pr/Ph ratio was in the range of 0.15–0.27, indicating that the inter-salt shale deposited under a strong reducing environment (Table 3). The cross-plot of Pr/n-C 17 versus Ph/n-C 18 has been widely used to indicate source rock types and depositional environments. As shown in Figure 12, the inter-salt shale deposited in a hyper-saline lacustrine and reducing environment.

Figure 12 Cross plots of Pr/n-C17 versus Ph/n-C18 ratios of the inter-salt shale.
As the best diagnostic element, Mo is an indicator of anoxic conditions for sediment deposition in the condition of sulphate-reducing. Mo contents of Eq34-10 rhythmic shales are in the range of 0.85–17.48, with an average of 5.53 – higher than the value of Eq40-5 rhythmic shales, which range from 1.87 to 7.88, with an average of 3.71. Mo is positively correlated with TOC, as is shown for the inter-salt shale in Figure 13. In addition, the same plot also shows an intense to extreme restriction for the inter-salt shale by comparing sediments from several modern anoxic marine sedimentary environments (Fig. 13).

Figure 13 Mo content versus TOC for the inter-salt shale from Qianjiang Formation.
Because the concentrations of single elements varies with different lithologies, trace element ratios, such as V/(V + Ni), V/Cr, Ni/Co and U/Th (Yan et al. Reference Yan, Chen, Wang, Wang and Wang2009), are widely used as redox indices. Both sets of inter-salt shales show a similar redox condition. Most samples plot in the anoxic regions of V/(V + Ni), while in the oxic regions of V/Cr, Ni/Co and U/Th (Fig. 14). Apparently, the higher oxygen conditions indicated by V/Cr, Ni/Co and U/Th are in conflict with the V/(V + Ni) ratios and the organic geochemical data, such as low Pr/Ph ratio and high gammacerane content index. Therefore, the empirical indexes V/Cr, Ni/Co and U/Th have proved futile in evaluating the redox conditions of salt-lake facies shale, and we need to be more cautious when using trace element ratios as redox proxies for terrigenous saline lacustrine.

Figure 14 Cross plots of trace-element ratios as palaeoredox proxies.
4.6. Palaeoproductivity
Phosphorus (P) is a significant nutrient element for primary productivity and is widely used as an index for palaeoproductivity (Murray & Leinen Reference Murray and Leinen1993). As shown in Figure 15, P is positively correlated with TOC for the inter-salt shales, indicating that the supply of P improves the palaeoproductivity and plays an important role in the organic matter accumulation during this period.
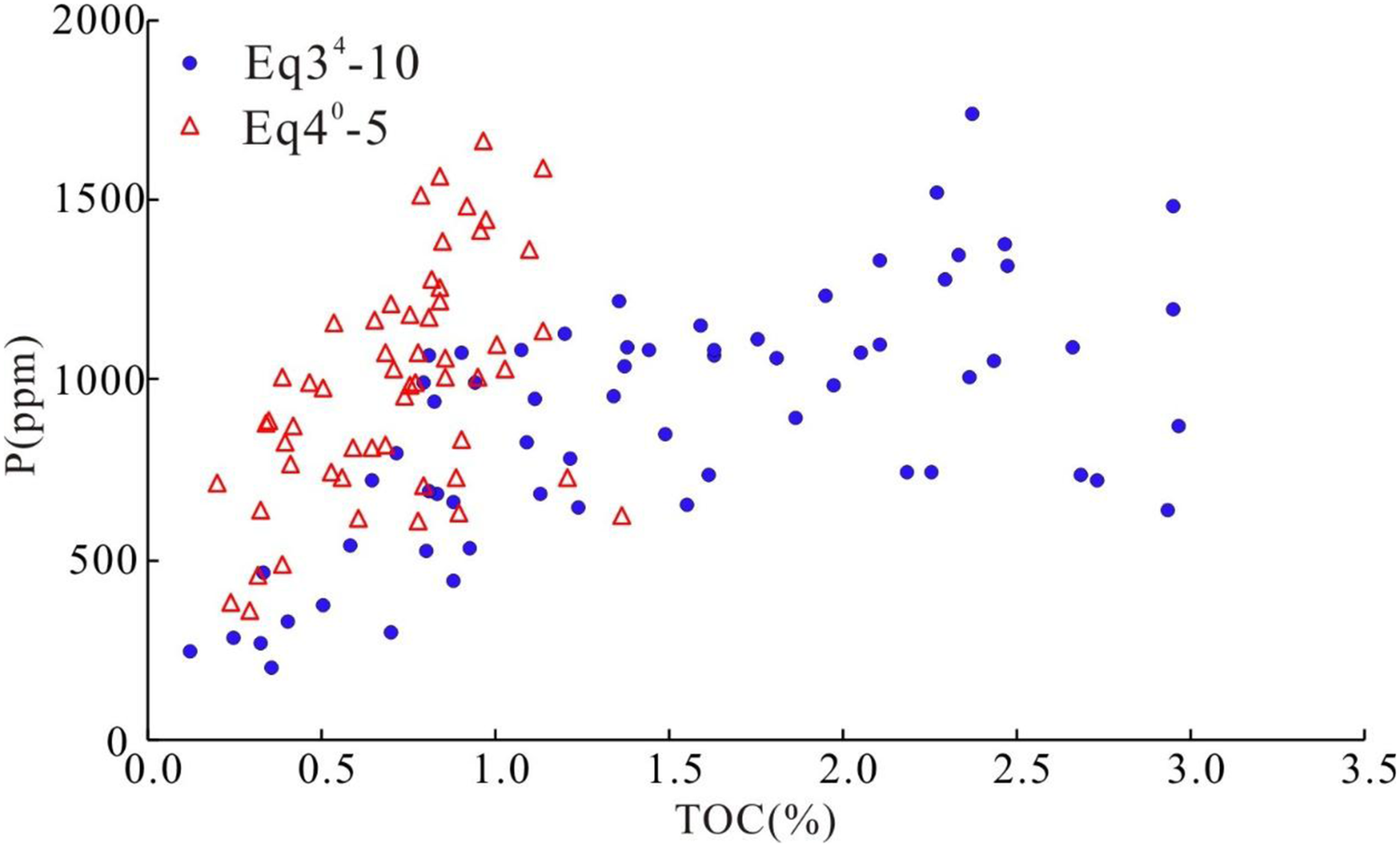
Figure 15 The relationship between P concentration and TOC of inter-salt shale.
4.7. Summary of formation model of inter-salt organic-rich shale
Modern and ancient saline lake basins are only distributed in arid–semi-arid areas. However, a continuous dry climate can make the saline lake gradually concentrated, gradually reducing the area or even causing it to disappear, and it cannot form large-scale source rocks. Under the overall dry climate, if the intermittent warm and humid climate occurs, the runoff of rivers increases and a large amount of fresh water is injected into the saline lake; thus, the saline lake area increases and the water desalinates, and organisms will appear in a short time and produce a large amount of organic matter. When the climate turns dry, intense evaporation reduces the area of the lake and increases its salinity. The brine makes it unsuitable for life, and a large number of organisms will die and become buried. The brief explosion and subsequent rapid death of this organism will produce a large amount of organic matter in a relatively short period, and the strongly reductive sedimentary environment will allow the organic matter to be preserved.
The Qianjiang Formation in the Qianjiang Depression deposited the inter-salt shale with extremely developed lamina against the background of an arid and semi-arid climate in the late Eocene–early Oligocene, and was controlled by a monsoon climate and subtropical high pressure. When the climate is relatively warm and humid, the rainfall is large, which increases the amount of fresh water deposited into the lake and reduces the salinity of the lake. At the same time, a large number of nutrient elements were also injected into the lake with the terrigenous clastic material, which contributed to the lake algae bloom. In addition, the hypoxic reducing environment can preserve the deposited organic matter and form organic-rich dolomitic mudstone. However, during the hot arid climate, high temperature, less rainfall and weakened weathering lead to reduced fresh water in the lake, increased lake salinity and a reduced supply of terrestrial nutrients, which is not conducive to the growth of lake organisms. Low lake productivity and rapid precipitation of carbonate minerals resulted in low organic carbon content in sediments, and formed argillaceous dolomite and even halite with low organic matter content.
5. Conclusions
The organic matter in the inter-salt shale was predominantly derived from endogenous lower aquatic organisms, algae and bacteria.
There was a hot arid palaeoclimate and a low degree of chemical weathering conditions during the deposition of the inter-salt shale.
The inter-salt shale deposited in an extreme restriction hyper-saline lacustrine and reducing environment, and the salinity of the palaeolake decreases with the increase of the input of terrigenous clastic materials.
Some trace elements and their ratios, which are mainly inherited from terrestrial clastic materials, such as Ga/Rb, V/Cr, Ni/Co and U/Th, are not suitable for evaluating the palaeoclimate and palaeoredox conditions of terrigenous lacustrine deposit.
The inter-salt shale deposited under an arid and semi-arid climate condition, with an intermittent warm and humid climate. A warm and humid climate is conducive to the formation of organic-rich shale, while a hot and dry climate is prone to argillaceous dolomite and even halite with low organic matter content.
6. Supplementary material
Supplementary material is available online at https://doi.org/10.1017/S1755691022000020
7. Acknowledgments
The authors acknowledge the financial support of the National Natural Science Foundation of China (grant numbers 41831176, 41572350 and 41903060), the National Key R&D Program of China (grant number 2017YFA0604803), the National Science and Technology Major Project of the Ministry of Science and Technology of China (number 2017ZX05049005) and the Open Fund of the Key Laboratory of Exploration Technologies for Oil and Gas Resources (Yangtze University), Ministry of Education (number K2021-20).