Introduction
Myzus persicae (Sulzer) (Hemiptera: Aphididae) is a cosmopolitan pest of many cultivated plants. Its management relies on the use of synthetic insecticides, the intensive use of which has resulted in the development of resistant populations. The complex life cycle of the pest, the large populations it forms, the extended range of plant hosts (Blackman et al., Reference Blackman, Malarky, Margaritopoulos and Tsitsipis2007; Blackman and Eastop, Reference Blackman, Eastop, van Emden and Harrington2017), the variety of agroecosystems and their climatic variation, the geographical and temporal distribution of the hosts and the aphid populations compose important attributes in the development of resistance to insecticides used and the design of strategies for its management. Considerable data have been published on the nature and the evolution of resistance (Bass et al., Reference Bass, Puinean, Zimmer, Denholm, Field, Foster, Gutbrod, Nauen, Slater and Williamson2014). Greece presents an interesting case study of resistance because it concerns many of the above matters. In this review, we present data of studies extending over a period of more than 20 years on many vital aspects of the resistance issue.
M. persicae, known as the green peach-potato aphid (M. persicae s.s) or the tobacco aphid (M. persicae nicotianae Blackman), has a host range of over 400 plant species, belonging to 40 families, many of which are economically important. The aphid becomes, therefore, one of the most important insect pests worldwide, damaging plants by direct feeding, spoilage from honeydew, and virus infection by transmitting more than 100 viruses (Blackman and Eastop, Reference Blackman, Eastop, van Emden and Harrington2017).
In Greece, M. persicae exhibits holocyclic (cyclical parthenogenesis) as well as anholocyclic life cycles. Prunus persica (L.) (Rosaceae) is the primary host, on which the overwintering eggs (outcome of a single sexual generation) are laid in Autumn. In Spring, nymphs hatch into fundatrices (stem-mothers). Parthenogenetic (all female) generations follow on peach and later on many secondary herbaceous host-plants, cultivated and weeds, until the sexual forms appear in the Autumn to repeat the cycle. Anholocycly is exhibited with continuous parthenogenetic generations on herbaceous hosts during the whole year (obligate parthenogenesis) and occasionally with the appearance of few sexual forms (functional parthenogenesis) in the Autumn that contribute to the sexual phase of the life cycle (Blackman et al., Reference Blackman, Malarky, Margaritopoulos and Tsitsipis2007). The subspecies M. persicae nicotianae, specialized on tobacco Nicotiana tabacum L. (Solanaceae), is present in Greece (Margaritopoulos et al., Reference Margaritopoulos, Tsitsipis, Zintzaras and Blackman2000, Reference Margaritopoulos, Malarky, Tsitsipis and Blackman2007a).
M. persicae s.l. is controlled almost exclusively by synthetic insecticides. Due to continuous selection pressure, resistance has developed worldwide to almost all groups of insecticides used either via a metabolic route or through mutations on their target-proteins (Devonshire et al., Reference Devonshire, Field, Foster, Moores, Williamson and Blackman1998; Bass et al., Reference Bass, Puinean, Zimmer, Denholm, Field, Foster, Gutbrod, Nauen, Slater and Williamson2014). Seven different resistance mechanisms have been described (Bass et al., Reference Bass, Puinean, Zimmer, Denholm, Field, Foster, Gutbrod, Nauen, Slater and Williamson2014), and resistance has developed on 75 compounds, the aphid being among the 12 most resistant insect species recorded (Sparks and Nauen, Reference Sparks and Nauen2015). In the last two decades, the chemical control of M. persicae depended highly on neonicotinoids, due to resistance to other insecticide groups. This group has been proved effective, although its excessive and extensive use has led to many cases of resistance development (Bass et al., Reference Bass, Puinean, Zimmer, Denholm, Field, Foster, Gutbrod, Nauen, Slater and Williamson2014, Reference Bass, Denholm, Williamson and Nauen2015; Voudouris et al., Reference Voudouris, Williamson, Skouras, Kati, Sahinoglou and Margaritopoulos2017). The development of resistance has caused serious problems in crop protection. Measures and strategies have been proposed to face it within the framework of Integrated Resistance Management (IRM) (IRAC, http://www.irac-online.org). A basic principle is the rotation of insecticides with different modes of action (MoA groups categorized by IRAC) and the ‘window’ approach. The treatment timing (window) is based on the minimum duration of a generation, and the susceptible developmental stage of the insect-pest. Within the same window, insecticides with the same MoA may be used if necessary (ΜοΑ1). In each subsequent window, if needed, insecticides with a different MoA are selected (ΜοΑ2, ΜοΑ3, ΜοΑ4). In case of absence of products with different MoA, rotation follows. The challenge becomes bigger with the shortening of the list of available insecticides, because of safety restrictions and environmental reasons, and regulations, as well as the increased cost and time required to develop new active ingredients/formulations (Sparks and Nauen, Reference Sparks and Nauen2015). Recent examples are the decision of EU to ban the use of imidacloprid, thiamethoxam and clothianidin in open field applications, because of adverse effects on honeybees and other hymenopterous pollinators, allowed only in undercover crops, and the use of pymetrozine, also for environmental reasons.
We have been studying the bio-ecology of M. persicae as well as its ability to flourish in diverse agroecosystems treated with insecticides. A regional variation in the mode of reproduction has been observed, associated with the presence or absence of peach. The aphid populations on herbaceous crops in northern Greece (Macedonia prefecture), where the majority of peach orchards are found, invest in sexual reproduction, whereas in other regions anholocyclic (asexual) genotypes prevail. However, both holocyclic (sexual) and anholocyclic genotypes co-exist in the same region (Blackman et al., Reference Blackman, Malarky, Margaritopoulos and Tsitsipis2007). Microsatellite genotyping showed that the distribution of common genotypes (those found at more than one time and place, which are less likely to be products of sexual reproduction) matched that of clones characterized as anholocyclic in biological assays (Blackman et al., Reference Blackman, Malarky, Margaritopoulos and Tsitsipis2007). The two life cycle strategies should not be considered completely independent, since some gene-flow occurs between holocyclic and functional parthenogen (anholocyclic) genotypes (Vorburger et al., Reference Vorburger, Lancaster and Sunnucks2003; Margaritopoulos et al., Reference Margaritopoulos, Malarky, Tsitsipis and Blackman2007a; Kati et al., Reference Kati, Mandrioli, Skouras, Malloch, Voudouris, Venturelli, Manicardi, Tsitsipis, Fenton and Margaritopoulos2014).
The genetic studies we performed showed also that long-distance migration is not so common in M. persicae in Greece, or that it is of low success. Nevertheless, there is evidence of movement of anholocyclic clones ('superclones’) in other European countries and in Chile (Margaritopoulos et al., Reference Margaritopoulos, Kasprowicz, Malloch and Fenton2009), and also of the existence of an introduction route of M. persicae nicotianae from Europe to the New World (Zepeda-Paulo et al., Reference Zepeda-Paulo, Simon, Ramírez, Fuentes-Contreras, Margaritopoulos, Wilson, Sorenson, Briones, Azevedo, Ohashi, Lacroix, Glais and Figueroa2010). These traits are to be taken into consideration in the design of wide area management strategies, as they provide information on between-crop aphid movement and the possible gene flow of insecticide resistance genes among crops (Margaritopoulos et al., Reference Margaritopoulos, Blackman, Tsitsipis and Sannino2003, Reference Margaritopoulos, Malarky, Tsitsipis and Blackman2007a; Blackman et al., Reference Blackman, Malarky, Margaritopoulos and Tsitsipis2007).
The populations of both M. persicae persicae and M. persicae nicotianae have a broadly heterogeneous genetic structure in mainland Greece, and this genetic diversity is the fuel powering evolution. The ability of sexual reproduction leads to the creation of novel genotypes, which may exhibit important competitive traits such as the combination of multiple insecticide resistance mechanisms (Margaritopoulos et al., Reference Margaritopoulos, Skouras, Nikolaidou, Manolikaki, Maritsa, Tsamandani, Kanavaki, Bacandritsos, Zarpas and Tsitsipis2007b; Voudouris et al., Reference Voudouris, Kati, Sadikoglou, Williamson, Skouras, Dimotsiou, Georgiou, Fenton, Skavdis and Margaritopoulos2016). The insect's adaptability in Greece seems to be impressive (e.g., adaptation of sexual M. persicae nicotianae populations to tobacco, ability to invest in different reproductive strategies) (Blackman et al., Reference Blackman, Malarky, Margaritopoulos and Tsitsipis2007; Margaritopoulos et al., Reference Margaritopoulos, Malarky, Tsitsipis and Blackman2007a) combined with rapid evolution events (e.g., changes in the frequencies of the carboxylesterase genes and new chromosomal rearrangements) (Kati et al., Reference Kati, Mandrioli, Skouras, Malloch, Voudouris, Venturelli, Manicardi, Tsitsipis, Fenton and Margaritopoulos2014). Parallel to the aphid's bio-ecology, various studies have been conducted on the resistance of M. persicae populations to various insecticides with different MoA, the underlying resistance mechanisms, and the evolution of this phenomenon over the years (Margaritopoulos et al., Reference Margaritopoulos, Skouras, Nikolaidou, Manolikaki, Maritsa, Tsamandani, Kanavaki, Bacandritsos, Zarpas and Tsitsipis2007b, Reference Margaritopoulos, Tsamandani, Kanavaki, Katis and Tsitsipis2010; Voudouris et al., Reference Voudouris, Kati, Sadikoglou, Williamson, Skouras, Dimotsiou, Georgiou, Fenton, Skavdis and Margaritopoulos2016, Reference Voudouris, Williamson, Skouras, Kati, Sahinoglou and Margaritopoulos2017).
Considering the insect's economic importance and the accumulation of multiyear data, we make, herewith, a review serving both academic and practical purposes. We present new and historical data of over 20-year research, on the effectiveness of insecticides earlier or currently used to control M. persicae in Greece, and on the incidence of resistance mechanisms in the aphid's populations. After the recent ban of certain neonicotinoids and pymetrozine, we present also new bioassay data on flonicamid and review published data on spirotetramat, flupyradifurone and sulfoxaflor, candidate insecticides suitable in Integrated Pest Management (IPM) and IRM programs.
The framework of studies
During our extended studies, thousands of wingless parthenogenetic aphid samples, deriving from various hosts and different geographical areas, have been tested by bioassays and/or biochemical and molecular diagnostics for resistance to insecticides belonging to many chemical groups.
Sampling
The aphids were collected from peach, tobacco, pepper Capsicum annuum L. (Solanaceae) and weeds in the period 1998–2019. The surveys were conducted in major agricultural regions of northern (Macedonia, Thrace), central (Thessaly), western (Epirus) and southern (Peloponnese) Greece, and the island of Crete. A concise plan of the studies is shown in table 1. Peach orchards were surveyed in April and May (before aphid migration), and herbaceous crops from June to August. Samples from weeds were usually collected during winter.
Table 1. Aphid samples of M. persicae collected from various hosts and regions of Greece, examined for insecticide resistance development with bioassays, biochemical and DNA diagnostics during the period 1998–2019.

a Tob, tobacco; other, pepper and weeds.
b Dose-response bioassays with imidacloprid (Imid), acetamiprid (Acet), deltamethrin (Delt), pirimicarb (Pir), pymetrozine (Pym), spirotetramat (Spir) and flonicamid (Flon); single-dose bioassays with imidacloprid.
c Metabolic resistance mechanisms: carboxylestearse overproduction (est) and over-expression of the CYP6CY3 gene (CYP6Y3); resistance mutations: MACE (S431F), kdr (L1024F), skdr (M981 T), R81T (nAChR).
d Bioassays with field samples or clones and diagnostics with individuals from clones or field samples.
One problem in the aphid population studies is ‘clonal amplification’, i.e., the proliferation of parthenogenetic genotypes. Inadequate sampling design or luck of information on the distinct genotypes of the material under investigation could bias the results. We addressed this problem by collecting aphid samples from peach, where each aphid clone from different trees presumably originates from different sexually produced eggs, and from secondary crops in northern Greece where holocyclic populations predominate (Blackman et al., Reference Blackman, Malarky, Margaritopoulos and Tsitsipis2007). In some cases, the aphid material was genotyped with microsatellite DNA markers (Kati et al., Reference Kati, Mandrioli, Skouras, Malloch, Voudouris, Venturelli, Manicardi, Tsitsipis, Fenton and Margaritopoulos2014; Voudouris et al., Reference Voudouris, Kati, Sadikoglou, Williamson, Skouras, Dimotsiou, Georgiou, Fenton, Skavdis and Margaritopoulos2016). During the long term survey a high number of different M. persicae genotypes were examined.
Diagnostic tests
Field collected samples, which were brought in the lab, or aphid clones reared in the lab, were tested with dose-response (FAO dip-test, leaf-dip test) or diagnostic dose (topical application) bioassays. For the biochemical (enzyme activity measurement) and DNA (Taqman, Polymerase chain reaction restriction fragment length polymorphism (PCR-RFLP), bidirectional polymerase chain reaction amplification of specific alleles (bi-PASA PCR)) diagnostics, individual aphids of the above two categories were used. Detailed information on methods used and results have been published in peer-reviewed papers (Cox et al., Reference Cox, Denholm, Devonshire, Simon, Dedryver, Rispe and Hullé2004; Anstead et al., Reference Anstead, Mallet and Denholm2007; Margaritopoulos et al., Reference Margaritopoulos, Skouras, Nikolaidou, Manolikaki, Maritsa, Tsamandani, Kanavaki, Bacandritsos, Zarpas and Tsitsipis2007b, Reference Margaritopoulos, Tsamandani, Kanavaki, Katis and Tsitsipis2010; Kati et al., Reference Kati, Mandrioli, Skouras, Malloch, Voudouris, Venturelli, Manicardi, Tsitsipis, Fenton and Margaritopoulos2014; Voudouris et al., Reference Voudouris, Kati, Sadikoglou, Williamson, Skouras, Dimotsiou, Georgiou, Fenton, Skavdis and Margaritopoulos2016, Reference Voudouris, Williamson, Skouras, Kati, Sahinoglou and Margaritopoulos2017).
Statistical treatment
In the unpublished bioassay data presented here, the LC50 values and the 95% fiducial limits were calculated by probit analysis using PoloPlus 2.0 (LeOra Software, Berkeley, CA, USA). Differences in LC50 values between field samples/clones and the most susceptible field sample/clone were examined using their lethal dose ratio, i.e., a difference was statistically significant when the calculated 95% confidence intervals of this ratio did not include 1 (ratio test; Robertson et al., Reference Robertson, Russell, Preisler and Savin2007)
The statistical tests used (analysis of new data or reanalysis of published ones) were performed using R (R Core Team, 2017). Frequencies were compared by the χ2 test (with Yates' correction) or Fisher's exact test when the analysis showed that χ2 approximation may be incorrect. When the test returned a significant value, pairwise comparisons were performed by the Bonferroni correction. Mean LC50 values were compared between regions and crops by the non-parametric Kruskal–Wallis H test, once the data deviated from normality (Shapiro–Wilk normality test). Pairwise post-hoc comparisons were made by the Tukey and Kramer (Nemenyi) test after Kruskal–Wallis H test.
Neonicotinoids
Imidacloprid
Resistance development
The first study on Greek populations was conducted during 1998–2000 (Cox et al., Reference Cox, Denholm, Devonshire, Simon, Dedryver, Rispe and Hullé2004). Aphid clones collected from various hosts and localities (peach: northern and central Greece, tobacco: northern, central and southern Greece, other crops: northern, central and southern Greece) were tested with a diagnostic dose of imidacloprid (topical application method). In 1998, all tested clones (Ν = 345) were susceptible to imidacloprid while in 1999–2000 (merged data) the non-susceptible ones reached 37.1% (N = 490). Non-susceptible clones were considered the ones with <10% mortality. The emergence of resistance was verified in later studies (Voudouris et al., Reference Voudouris, Kati, Sadikoglou, Williamson, Skouras, Dimotsiou, Georgiou, Fenton, Skavdis and Margaritopoulos2016), with the same bioassay method, in clones from peach (northern, central and southern Greece) and tobacco (northern Greece), where non-susceptible clones climbed to 44.4 (Ν = 151) and 51.2% (Ν = 41) in 2007 and 2012 respectively. However, differences were not significant (χ2, P > 0.106). The non-susceptible clones were characterized by a statistical criterion (significant difference in the mortality between the test and the reference clones), which is more conservative than the criterion used by Cox et al. (Reference Cox, Denholm, Devonshire, Simon, Dedryver, Rispe and Hullé2004). The frequencies for 2007 and 2012 were even higher (56.9 and 56.1%, respectively) when Cox et al. (Reference Cox, Denholm, Devonshire, Simon, Dedryver, Rispe and Hullé2004) criterion was used. In the latter case, differences were statistically significant (37.1 vs. 56.9%: χ2 = 17.815, df = 1, P < 0.001; 37.1 vs. 56.1%: χ2 = 4.932, df = 1, P = 0.026).
In addition, increased imidacloprid resistance was diagnosed with dose-response bioassays, where LC50 values and resistance factors (RF) were calculated. The field samples/clones examined were collected from peach (Ν = 84) and tobacco (Ν = 126) from northern, central and southern Greece (Margaritopoulos et al., Reference Margaritopoulos, Skouras, Nikolaidou, Manolikaki, Maritsa, Tsamandani, Kanavaki, Bacandritsos, Zarpas and Tsitsipis2007b: surveys in 2004–2006; Voudouris et al., Reference Voudouris, Williamson, Skouras, Kati, Sahinoglou and Margaritopoulos2017: surveys in 2007 and 2015). In 2004–2007 field samples were tested (bioassays with FAO dip-test) while in 2015 lab reared clones (leaf-dip bioassays). A strict criterion was used, i.e., RF>15, to distinguish the resistant samples. Increased resistance occurred with time. Especially in tobacco, the increase was statistically significant (χ2 = 29.186, df = 2, P < 0.001) with frequencies of resistant samples/clones being 78.7 and 86.7% in 2007 and 2015 respectively, in comparison to 34.7% in 2004–2005. In peach, the respective frequencies were 13.3 and 6.7% for 2007 and 2015 compared to 2.6% in 2004–2006 (χ2 = 2.287, df = 2, P = 0.319) (Voudouris et al., Reference Voudouris, Williamson, Skouras, Kati, Sahinoglou and Margaritopoulos2017). The frequency distribution of RF values for each sampling year and crop are shown in fig. 1.
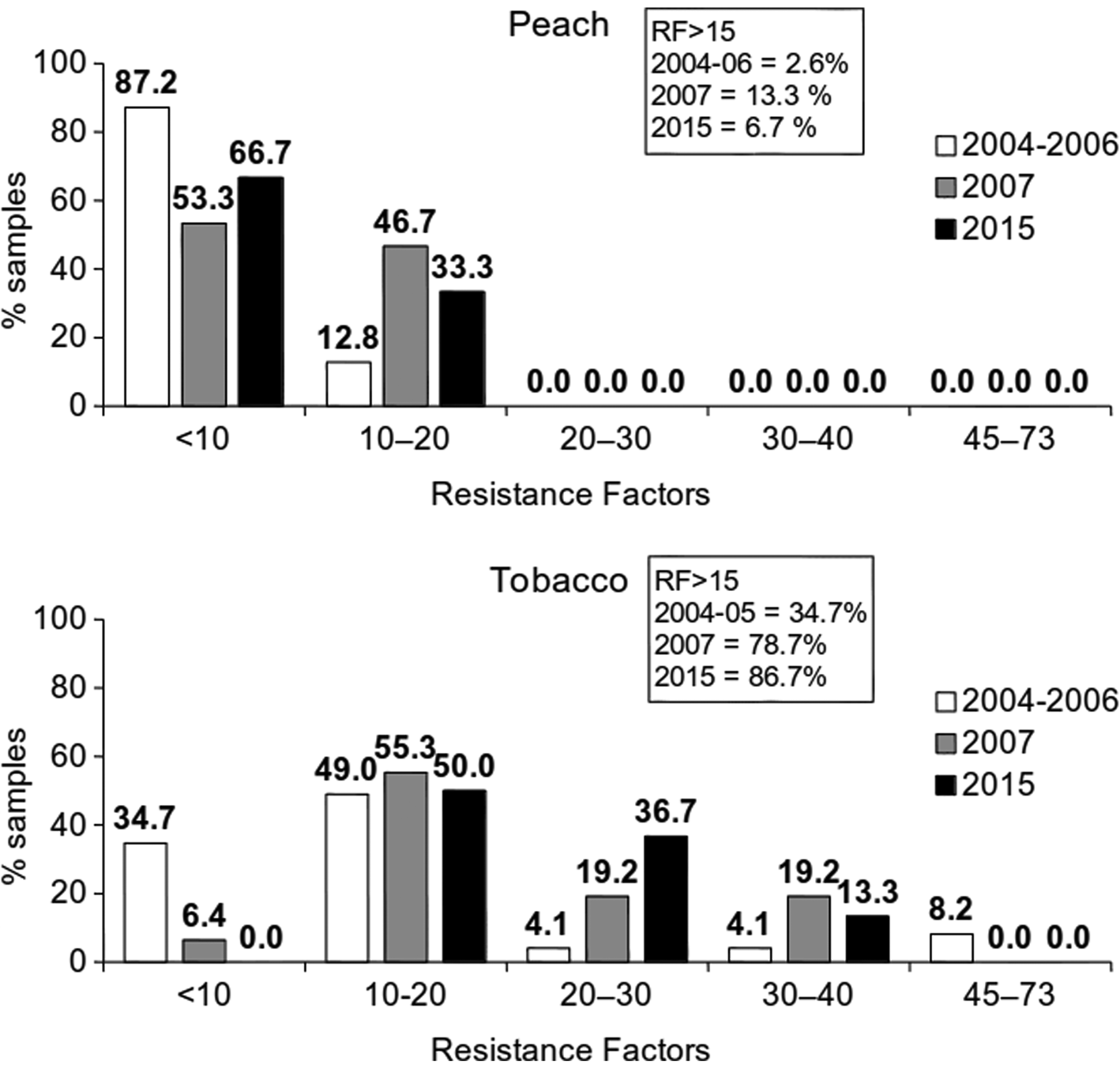
Figure 1. Frequency distribution of resistance factors (RF) from dose-response bioassays of imidacloprid in field samples/clones of Myzus persicae, collected from peach and tobacco in 2004–2006, 2007 and 2015. In the box, frequencies of samples/clones with RF>15 (N = 210).
Differences among crops and localities
The distribution of RF values showed resistance differences between samples from peach and tobacco. This tendency was also evident in the mean LC50 values among localities and crops. Generally, in most comparisons the mean LC50 values of peach populations were significantly lower (P < 0.05) than those from tobacco (Margaritopoulos et al., Reference Margaritopoulos, Skouras, Nikolaidou, Manolikaki, Maritsa, Tsamandani, Kanavaki, Bacandritsos, Zarpas and Tsitsipis2007b: Kruskal–Wallis H test, Dunn's test as post-hoc tests; Voudouris et al., Reference Voudouris, Williamson, Skouras, Kati, Sahinoglou and Margaritopoulos2017: Kruskal–Wallis H test, Nemenyi test for post-hoc tests). In 2004–2006 (FAO dip-test) the mean LC50 values in peach and tobacco populations were 0.9–1.6 ppm and 3.8–5.7 ppm, respectively (Margaritopoulos et al., Reference Margaritopoulos, Skouras, Nikolaidou, Manolikaki, Maritsa, Tsamandani, Kanavaki, Bacandritsos, Zarpas and Tsitsipis2007b). The corresponding values in 2007 (FAO dip-test), were 1.4–3.7 and 4.9–7.5 ppm. In 2015, with leaf-dip tests, the mean LC50 values of peach populations were 24.5–39.5 ppm and those of tobacco 69.7–85.9 ppm (Voudouris et al., Reference Voudouris, Williamson, Skouras, Kati, Sahinoglou and Margaritopoulos2017). The differences between the two crops were observed even in localities in northern Greece where peach orchards are adjacent to tobacco crops.
Such differences in resistance (see below the resistance mechanism frequencies) among populations from peach and tobacco in Greece, have been attributed to factors related to the aphid's biological cycle and the selection pressure from insecticides (Blackman et al., Reference Blackman, Malarky, Margaritopoulos and Tsitsipis2007; Margaritopoulos et al., Reference Margaritopoulos, Skouras, Nikolaidou, Manolikaki, Maritsa, Tsamandani, Kanavaki, Bacandritsos, Zarpas and Tsitsipis2007b; Voudouris et al., Reference Voudouris, Kati, Sadikoglou, Williamson, Skouras, Dimotsiou, Georgiou, Fenton, Skavdis and Margaritopoulos2016, Reference Voudouris, Williamson, Skouras, Kati, Sahinoglou and Margaritopoulos2017). Aphid populations in peach consist of a large number of different genotypes, as a result of sexual reproduction, and often show different resistance profiles. The aphids migrate from peach to tobacco or to other herbaceous crops end of May–early June. During the crop season, populations are under intense selection pressure. In Greece, usually 1–2 neonicotinoid applications were performed in peach orchards and one in setting water in tobacco followed by foliar applications. Under such circumstances resistant genotypes are selected, proliferating through parthenogenetic reproduction and resulting in homogenized resistant populations. In addition, anholocyclic genotypes (clones >1 year old), overwintered on weeds, move to tobacco. These genotypes might have also become resistant being under continuous selection through neonicotinoid applications for many years/crop seasons.
The published data (Margaritopoulos et al., Reference Margaritopoulos, Skouras, Nikolaidou, Manolikaki, Maritsa, Tsamandani, Kanavaki, Bacandritsos, Zarpas and Tsitsipis2007b; Voudouris et al., Reference Voudouris, Kati, Sadikoglou, Williamson, Skouras, Dimotsiou, Georgiou, Fenton, Skavdis and Margaritopoulos2016, Reference Voudouris, Williamson, Skouras, Kati, Sahinoglou and Margaritopoulos2017) has also shown differences in resistance among populations from tobacco or peach from different localities, with higher mean LC50 values in northern Greece. This reflects differences in the frequency and intensity of chemical control applications among regions. However, other factors related to bio-ecology and the genetic structure of aphid populations as well as to geographical barriers among the regions, cannot be excluded.
Acetamiprid
Due to recent ban of all outdoor uses of imidacloprid by EU authorities, we monitored the effectiveness of acetamiprid in Greece. A total of 24 M. persicae clones were examined in leaf-dip bioassays with mortality recorded 72 hr post-treatment (for method details see Voudouris et al., Reference Voudouris, Williamson, Skouras, Kati, Sahinoglou and Margaritopoulos2017), which were collected from peach and tobacco from various regions of Greece in 2018–2019. The clones were compared with our standard susceptible clone (4106A). In addition, the reference clones 5191A (Puinean et al., Reference Puinean, Foster, Oliphant, Denholm, Field, Millar, Williamson and Bass2010), FRC (Bass et al., Reference Bass, Puinean, Andrews, Cutler, Daniels, Elias, Paul, Crossthwaite, Denholm, Field, Foster, Lind, Williamson and Slater2011) and 5444B (provided by Rothamstead Research), resistant to neonicotinoids, were examined. The resistance profiles for 4106A, 5191A, 5444B and FRC are: SS, SR, SS and non-tested for the S431F (MACE) mutation; SS, SS, RR and non-tested for both L1014F (kdr) and M918 T (super-kdr) mutations; SS, SS, RR and RR for the R81T mutation. In addition, the clones 5191A, 5444B and FRC share the same metabolic resistance mechanisms, i.e., overexpression of P450 CYP6CY3 gene and carboxylesterase overproduction. The lowest LC50 value was observed in the susceptible 4106A clone (1.752 ppm). LC50 values of the field collected clones ranged from 1.936 to 224.678 ppm and RF values from 1.1 to 128.2. The corresponding values for the reference clones 5191A, 5444B and FRC were 13.678, 97.989 and 931.018 ppm (LC50), and 7.8, 55.9 and 547.1 (RF). In 18 of the field clones the LC50 value was significantly higher than that of the susceptible one (ratio test, P < 0.05). It is interesting, that four and 12 field collected clones showed LC50 values higher than the 5444B and 5191A reference resistant clones, respectively (table 2, Supplementary_table_S1). The 33.3% of the clones showed RF>15 and 16.7% high values ranging from 101.0 to 128.2. The data suggest resistance development in acetamiprid of aphid populations in Greece, taken into account that in a survey a decade ago (2006–2007) 14.5% of 55 field samples, examined with the FAO dip-test, showed RF>15 with RF values ranging from 3.7 to 22.1 (Skouras, Reference Skouras2009).
Table 2. Summary statistics for LC50 in ppm and RF values (in italics) of field-collected (2017–2019) clones of Myzus persicae from Greece that was examined in bioassays with acetamiprid, pymetrozine and flonicamid. The LC50 and RF values of four reference clones are given for comparison purposes.
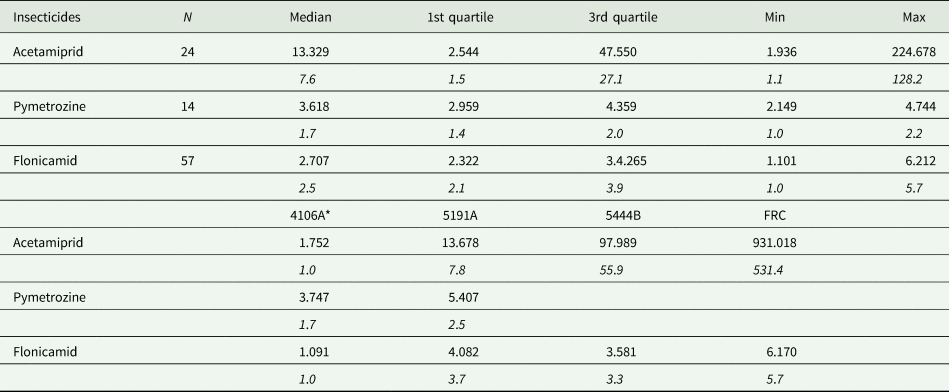
N, number of field clones examined. *4106A, standard susceptible clone; 5191A, 5444B and FRC, reference clones resistant to neonicotinoids and other chemical classes of insecticides (see text for details). For pymetrozine a field-collected clone, with lower LC50 than 4106A, was used for RF calculations.
One interesting finding is the difference in the RF value between 5444B and FRC clones, which are homozygous for the R81T mutation, possessing also the CYP6CY3 overexpression metabolic mechanism. Bass et al. (Reference Bass, Puinean, Andrews, Cutler, Daniels, Elias, Paul, Crossthwaite, Denholm, Field, Foster, Lind, Williamson and Slater2011) reported that CYP6CY3 was overexpressed in the FRC clone 28-fold, while we found a 6.6-fold overexpression in 5444B clone by the same quantitative PCR method. This difference might explain the different resistance levels observed in the two clones, although further investigation is needed. The 5191A clone, with a 22-fold CYP6CY3 overexpression and without the R81T mutation, showed a much lower RF value from both clones, as reported with other neonicotinoids (Puinean et al., Reference Puinean, Foster, Oliphant, Denholm, Field, Millar, Williamson and Bass2010; Bass et al., Reference Bass, Puinean, Andrews, Cutler, Daniels, Elias, Paul, Crossthwaite, Denholm, Field, Foster, Lind, Williamson and Slater2011).
Neonicotinoids resistance mechanisms
Cytochrome P450 monooxygenases
This resistance mechanism relates to over-expression of the CYP6CY3 gene leading to the production of higher quantities of the enzyme (a cytochrome P450 monooxygenase) metabolizing the insecticide molecules entering the insect. This over-expression is due to gene amplification (up to 100 copies of the CYP6CY3 gene in some aphid genotypes) and the expansion of a dinucleotide microsatellite in the promoter region (Puinean et al., Reference Puinean, Foster, Oliphant, Denholm, Field, Millar, Williamson and Bass2010; Bass et al., Reference Bass, Puinean, Zimmer, Denholm, Field, Foster, Gutbrod, Nauen, Slater and Williamson2014). The mechanism has been detected in Greek M. persicae populations. Of the total, 58.6% of peach and tobacco clones collected in 2012–2013 showed over-expression of the CYP6CY3 gene (9–36 times) (Voudouris et al., Reference Voudouris, Kati, Sadikoglou, Williamson, Skouras, Dimotsiou, Georgiou, Fenton, Skavdis and Margaritopoulos2016), which is within the range previously reported (10–75 times) (Puinean et al., Reference Puinean, Foster, Oliphant, Denholm, Field, Millar, Williamson and Bass2010; Bass et al., Reference Bass, Puinean, Andrews, Cutler, Daniels, Elias, Paul, Crossthwaite, Denholm, Field, Foster, Lind, Williamson and Slater2011, Reference Bass, Puinean, Zimmer, Denholm, Field, Foster, Gutbrod, Nauen, Slater and Williamson2014).
This resistance mechanism seems to confer moderate levels of resistance to neonicotinoids. A resistant clone was 27.5–56.5 times more resistant to various neonicotinoids, in topical bioassays, compared to the susceptible reference clone (Philippou et al., Reference Philippou, Field and Moores2010; Puinean et al., Reference Puinean, Foster, Oliphant, Denholm, Field, Millar, Williamson and Bass2010; Bass et al., Reference Bass, Puinean, Andrews, Cutler, Daniels, Elias, Paul, Crossthwaite, Denholm, Field, Foster, Lind, Williamson and Slater2011). It is considered, that such resistance levels are not sufficient to compromise the effectiveness of neonicotinoids in the field, when they are applied at the recommended doses (Bass et al., Reference Bass, Puinean, Zimmer, Denholm, Field, Foster, Gutbrod, Nauen, Slater and Williamson2014). However, populations with high frequencies of such resistant individuals, arising from the continuous selection pressure imposed by the neonicotinoids, might be difficult to control.
R81T mutation
A M. persicae clone (FRC) collected from peach in southern France in 2009 was found very resistant to neonicotinoids. The study led to the detection of a new resistance mechanism related to the existence of a novel point mutation (R81T) in the postsynaptic nicotinic acetylcholine receptor (nAChR loop D of the β1 subunit) (Bass et al., Reference Bass, Puinean, Andrews, Cutler, Daniels, Elias, Paul, Crossthwaite, Denholm, Field, Foster, Lind, Williamson and Slater2011). This resistance mechanism spread fairly fast in European and north African countries such as southern France (2009–2010; Bass et al., Reference Bass, Puinean, Andrews, Cutler, Daniels, Elias, Paul, Crossthwaite, Denholm, Field, Foster, Lind, Williamson and Slater2011; Slater et al., Reference Slater, Paul, Andrews, Garbay and Camblin2012), Spain (2010; Slater et al., Reference Slater, Paul, Andrews, Garbay and Camblin2012), Italy (2012; Panini et al., Reference Panini, Dradi, Marani, Butturini and Mazzoni2014), Greece (2015–2016; Voudouris et al., Reference Voudouris, Williamson, Skouras, Kati, Sahinoglou and Margaritopoulos2017), Tunisia (2014–2016; Charaabi et al., Reference Charaabi, Boukhris-Bouhachem, Makni and Denholm2018), Morocco and Belgium (http://www.irac-online.org). The mutation results in reduced sensitivity of nAChR through the loss of electrostatic interactions of the electronegative insecticide with the positively charged arginine residue, which has been substituted by the neutral threonine at the D loop, conferring a ‘vertebrate-like’ quality to the β1 subunit (Bass et al., Reference Bass, Puinean, Zimmer, Denholm, Field, Foster, Gutbrod, Nauen, Slater and Williamson2014). Because of the mode of action of neonicotinoids on nAChR, cross resistance has been anticipated to all neonicotinoids. Bass et al. (Reference Bass, Puinean, Andrews, Cutler, Daniels, Elias, Paul, Crossthwaite, Denholm, Field, Foster, Lind, Williamson and Slater2011) reported RF values 1679 and 225 for imidacloprid and thiamethoxam respectively, in bioassays with the leaf spraying method. The level of resistance was greatly attenuated by the synergistic action of the overexpression of the CYP6CY3 gene. In another study, with the FRC clone, with a different bioassay method (plant-dipping into insecticide solution), the reported RF values were 2350 for imidacloprid, 270 for thiamethoxam, >2500 for thiacloprid, and 82 for acetamiprid (Slater et al., Reference Slater, Paul, Andrews, Garbay and Camblin2012). Furthermore, Cutler et al. (Reference Cutler, Slater, Edmunds, Maienfisch, Hall, Earley, Pitterna, Pal, Paul, Goodchild, Blacker, Hagmann and Crossthwaite2013) in leaf spraying bioassays with the FRC clone, reported RF values 3013, 182 and 54 for clothianidin, dinotefuran and nitenpyram, respectively. Beyond M. persicae, R81T target site resistance was found in Aphis gossypii (Glover) (Hemiptera: Aphididae) from Japan (2012; Hirata et al., Reference Hirata, Kiyota, Matsuura, Toda, Yamamoto and Iwasa2015) and China (2014; Chen et al., Reference Chen, Li, Chen, Ma, Liang, Liu, Song and Gao2017), conferring moderate to high resistance to various neonicotinoids tested (RF: 24–394 in a Japanese and 1215–1776 in a Chinese resistant clone). Moderate or high levels of cross resistance has also been observed to sulfoxaflor (sulfoximine), classified in MoA group 4 (chemical group 4C; neonicotinoids: chemical group 4A), in the FRC M. persicae clone (RF = 43; Cutler et al., Reference Cutler, Slater, Edmunds, Maienfisch, Hall, Earley, Pitterna, Pal, Paul, Goodchild, Blacker, Hagmann and Crossthwaite2013), in a M. persicae clone from Spain homogygous for the R81T mutation (RF = 70; Bass et al., Reference Bass, Denholm, Williamson and Nauen2015) and in a resistant clone of A. gossypii from China (RF = 260; Chen et al., Reference Chen, Li, Chen, Ma, Liang, Liu, Song and Gao2017).
Following the publication of the novel R81T mutation target site resistance mechanism of M. persicae to neonicotinoids, the high degree of resistance it confers, and the fast spread to other European countries, we studied the existence of the mutation in Greece, after having done considerable work in neonicotinoid resistance in this species (Voudouris et al., Reference Voudouris, Kati, Sadikoglou, Williamson, Skouras, Dimotsiou, Georgiou, Fenton, Skavdis and Margaritopoulos2016, Reference Voudouris, Williamson, Skouras, Kati, Sahinoglou and Margaritopoulos2017). In total, 1208 aphids were tested with PCR-RFLP or Taqman assay that were collected from peach (549 aphids), tobacco (528 aphids), pepper (106 aphids), and weeds (25 aphids) during 2012–2016. The aphids were from various localities of northern (peach, tobacco, weeds), western (pepper), central (peach), and southern (peach, pepper) Greece, including the Crete island in the south.
The mutation was not found in any of the 2012–2014 samples. However, in 2015, it was detected in peach samples from Meliki, northern Greece. In all, 12.5% of the aphids in these samples were R81T heterozygotes. In 2016, the mutation was found again in peach samples in more localities. In particular, 15.0, 36.7, 5.0 and 20.0% from Argos (southern Greece), Tirnavos (central Greece), Velvedos and Meliki (both in northern Greece) were R81T heterozygotes. In the total sample from peach, 4.3 and 21.3% in 2015 and 2016 were heterozygotes. This increase was significant (χ2 = 13.89, df = 1, P < 0.001). In 2016, there have been observed control failures with neonicotinoids in peach orchards in northern Greece, which may be associated with the presence of high R81T frequencies.
Synopsis – mechanisms
Our studies show that the main neonicotinoid resistance mechanism, especially for tobacco populations showing higher resistance than those from peach (fig. 1), is the increased detoxification from the monooxygenase cytochrome P450. The second known mechanism (R81T mutation), found recently in 2015, follows an increasing trend, requiring continuous monitoring.
As the R81T was detected only in peach samples, it is possible that the resistant allele intruded into Greece through sexual genotypes from European countries, and dispersed through sexual reproduction in the peach populations. The increase in 2016 can be attributed to: (a) selection of resistant genotypes through intensive application of neonicotinoids, (b) dispersal of the resistant allele from the invading individuals to many genotypes in different regions through sexual reproduction on peach and (c) new invasions from European countries. The fact that the mutation has not been detected in tobacco, even though the aphid migrates from peach to tobacco in northern Greece (Blackman et al., Reference Blackman, Malarky, Margaritopoulos and Tsitsipis2007; Margaritopoulos et al., Reference Margaritopoulos, Malarky, Tsitsipis and Blackman2007a), cannot be explained from our data. Possibly, genotypes with the R81T mutation are negatively selected on tobacco or have not reached the crop in sufficient numbers to be sampled.
It is not clear why R81T reached Greece only recently and has been found only in samples from peach at low/moderate frequencies (0.0–12.5% and 5.0–36.7% of aphids in samples from peach were heterozygotes in 2015 and 2016 respectively). This could be attributed to low gene-flow between Greek and European or northern African populations. Homozygote individuals were 6–100% in southern France, 15–100% in northern Spain, 10–100% in Italy and 0–23% in Tunisia and in most sampling locations high frequencies of the resistant allele were found (Slater et al., Reference Slater, Paul, Andrews, Garbay and Camblin2012; Puinean et al., Reference Puinean, Elias, Slater, Warren, Field, Williamson and Bass2013; Panini et al., Reference Panini, Dradi, Marani, Butturini and Mazzoni2014; Charaabi et al., Reference Charaabi, Boukhris-Bouhachem, Makni and Denholm2018) It is worth noticing that in laboratory bioassays homozygous R81T clones from peach in France were more resistant than heterozygous ones (approximately 9–103-fold for imidacloprid and 4–30 for thiacloprid), and that the resistant allele is semi-recessive (Mottet et al., Reference Mottet, Fontaine, Caddoux, Brazier, Mahéo, Simon, Micoud and Roy2016), explaining partially the low resistance in Greek populations from peach found to be heterozygous (see additional factors discussed in ‘Differences among crops and localities’ section). Consequently, continuous monitoring of the persicae/nicotianae populations in Greece, for the R81T presence, is required to follow its rate of dispersal in different localities and its frequencies. Furthermore, additional surveys are needed to validate the present situation regarding the CYP6CY3 mechanism as this will clarify the importance of the two resistance mechanisms known to act synergistically.
Belgium was the most northern country the R81T mutation was found and Tunisia the most southern. Surveys in Australia and UK failed to detect this mutation (de Little et al., Reference de Little, Edwards, van Rooyen, Weeks and Umina2017). Given the recent distribution of R81T and that it is incompletely dominant in expression, Charaabi et al. (Reference Charaabi, Boukhris-Bouhachem, Makni and Denholm2018) suggested that the mutation is less likely to be selected where M. persicae populations are predominantly anholocyclic. However, some gene-flow occurs between holocyclic and anholocyclic populations through the few sexual morphs produced by the functional parthenogenetic genotypes. This has implications in the creation of novel-resistant genotypes in anholocyclic populations, which has been proved in regions where the two life cycle strategies co-exist (Blackman et al., Reference Blackman, Spence, Field and Devonshire1999; Kati et al., Reference Kati, Mandrioli, Skouras, Malloch, Voudouris, Venturelli, Manicardi, Tsitsipis, Fenton and Margaritopoulos2014). Thus, we believe it is a matter of time the R81T mutation is detected in regions where the aphid invests mostly in anholocycly.
Pyrethroids
Bioassays
Resistance levels
The available data for the Greek M. persicae populations derives from field samples, collected during 2005–2007, via FAO dip-test bioassays with deltamethrin. The samples were collected from tobacco (Ν = 44, northern and central Greece) and peach (Ν = 43, northern and central Greece) (Margaritopoulos et al., Reference Margaritopoulos, Skouras, Nikolaidou, Manolikaki, Maritsa, Tsamandani, Kanavaki, Bacandritsos, Zarpas and Tsitsipis2007b; Skouras, Reference Skouras2009). Of the total, 85.0% of the samples had high RF values (>26) and only 5.8% low RF values (<10) with a high percentage of the samples (56.3%) showing very high RF values (100 or 109–1266). Reanalysis of the data showed, that the majority of the samples (90.8%) from all 3 years were found to be resistant (RF >15). In addition, an increase in resistance was observed in 2006 and 2007 (92.1 and 100%, respectively) compared to 2005 (78.3%) (Fisher's Exact test, P = 0.023, pairwise comparisons among years significant only for 2005 vs. 2007: P = 0.018). The increase of resistance over the years is also revealed by the increase of the mean LC50 values in all samples collected per year (Supplementary_table_S2; Kruskal–Wallis H test, χ2 = 58.323, df = 2, P < 0.001, post-hoc test: Nemenyi test, P < 0.05 in all pairwise comparisons). The very high resistance observed in many samples suggested possible control failures. More recent data from bioassays on Greek populations is not available. The frequencies of the resistance mechanisms have been observed for over 19 years (see below) and they have remained high.
Differences between crops
Significant differences have been observed (P < 0.05; Kruskal–Wallis H test, post-hoc test: Nemenyi test) between crops, and higher values were recorded in samples from peach than from tobacco, especially in the last year of the survey (2007) (Supplementary_table_S2). It is characteristic that in northern Greece, in 2007, the mean LC50 value was almost three times higher in peach than in tobacco, even though the aphid migrates from peach to tobacco and vice versa (Blackman et al., Reference Blackman, Malarky, Margaritopoulos and Tsitsipis2007). This phenomenon has been thoroughly analyzed earlier (Margaritopoulos et al., Reference Margaritopoulos, Skouras, Nikolaidou, Manolikaki, Maritsa, Tsamandani, Kanavaki, Bacandritsos, Zarpas and Tsitsipis2007b; Voudouris et al., Reference Voudouris, Williamson, Skouras, Kati, Sahinoglou and Margaritopoulos2017), and it has been attributed to the more frequent use of pyrethroids, in peach orchards for other pests, compared to tobacco or other crops. Therefore, the selection pressure for pyrethroid resistant aphids is much higher in peach. The control programs are different on tobacco (use of different active ingredients) resulting in the selection of genotypes with different resistant mechanisms. Consequently, the pyrethroid-resistant aphids, migrating from peach to tobacco not possessing appropriate resistance mechanisms, are eliminated.
Pyrethroid resistance mechanisms
There are two known resistance mechanisms in M. persicae making the pyrethroid target site non-sensitive (voltage-gated sodium channel). The kdr (L1014F mutation) and super-kdr (M918 T mutation) are distributed worldwide (Anstead et al., Reference Anstead, Mallet and Denholm2007; Bass et al., Reference Bass, Puinean, Zimmer, Denholm, Field, Foster, Gutbrod, Nauen, Slater and Williamson2014). The kdr mutation was first described in 1997. M. persicae clones, homozygous for the kdr-resistant allele, exhibited increased resistance by 35 times to deltamethrin, while the carboxylesterace over-production (metabolic resistance mechanism), in some clones, enhanced it by an additional 15 times (Devonshire et al., Reference Devonshire, Field, Foster, Moores, Williamson and Blackman1998; Martinez-Torres et al., Reference Martinez-Torres, Foster, Field, Devonshire and Williamson1999). Later, the super-kdr (M918 T) mutation was found in Μ. persicae, which significantly increases the resistance provided by the kdr mechanism (Eleftherianos et al., Reference Eleftherianos, Foster, Williamson and Denholm2008). A 100-fold reduction of the sodium channel sensitivity, in functional expression experiments of the Drosophila para gene with M918 T and L1014F, was recorded (Vais et al., Reference Vais, Williamson, Goodson, Devonshire, Warmke, Usherwood and Cohen2000). In bioassays with several type I and II pyrethoids, M. persicae clones heterozygous to the L1014F mutation, showed 23–73 times resistance, while the resistance was much higher in the presence of the M918 T mutation. A clone heterozygous in both mutations showed 49–455 times resistance, depending on the pyrethroid tested (Eleftherianos et al., Reference Eleftherianos, Foster, Williamson and Denholm2008). It is important to note that Μ918Τ has only been found together with L1014F within the same individuals. The two mutations show worldwide distribution (Anstead et al., Reference Anstead, Mallet and Denholm2007; Bass et al., Reference Bass, Puinean, Zimmer, Denholm, Field, Foster, Gutbrod, Nauen, Slater and Williamson2014). Recently, another form (M918L) of the super-kdr mutation has been found in populations from Western Europe. This mutation has two variants, one caused by an a/c and the other by an a/t nucleotide substitution. The variants of the M918L mutation were not found to be linked with the L1014F mutation. To distinguish the two Μ918L variants, we use the terms M918L-WE (variant reported by Fontaine et al., Reference Fontaine, Caddoux, Brazier, Bertho, Bertolla, Micoud and Roy2011) and M918L-SE (variant reported by Panini et al., Reference Panini, Dradi, Marani, Butturini and Mazzoni2014) in this text. Important to say that in most insect species the kdr/super-kdr mutations are inherited as recessive characters, with limited or no expression of the resistant phenotype in heterozygotes (Davies et al., Reference Davies, Field, Usherwood and Williamson2007). On the contrary, M. persicae heterozygotes for L1014F, M918 T and M918L express a resistant phenotype to the pyrethroids tested (Eleftherianos et al., Reference Eleftherianos, Foster, Williamson and Denholm2008; Fontaine et al., Reference Fontaine, Caddoux, Brazier, Bertho, Bertolla, Micoud and Roy2011).
In Greece, M. persicae populations have been monitored for 14 years for kdr and super-kdr, as well as for 19 years for the carboxylesterace-based metabolic resistance mechanism (Anstead et al., Reference Anstead, Mallet and Denholm2007; Margaritopoulos et al., Reference Margaritopoulos, Skouras, Nikolaidou, Manolikaki, Maritsa, Tsamandani, Kanavaki, Bacandritsos, Zarpas and Tsitsipis2007b; Kati et al., Reference Kati, Mandrioli, Skouras, Malloch, Voudouris, Venturelli, Manicardi, Tsitsipis, Fenton and Margaritopoulos2014; Voudouris et al., Reference Voudouris, Kati, Sadikoglou, Williamson, Skouras, Dimotsiou, Georgiou, Fenton, Skavdis and Margaritopoulos2016). A total of 1087 and 416 aphids have been tested for L1014F and M918 T respectively, with PASA (Margaritopoulos et al., Reference Margaritopoulos, Skouras, Nikolaidou, Manolikaki, Maritsa, Tsamandani, Kanavaki, Bacandritsos, Zarpas and Tsitsipis2007b), PCR-RFLP (Voudouris et al., Reference Voudouris, Kati, Sadikoglou, Williamson, Skouras, Dimotsiou, Georgiou, Fenton, Skavdis and Margaritopoulos2016), or Taqman assays (Anstead et al., Reference Anstead, Williamson, Eleftherianos and Denholm2004, Reference Anstead, Mallet and Denholm2007). The two mutations have been found in the Greek populations since 2002. The L1014F mutation has been observed at very high frequencies over time, higher in peach than in tobacco (resistance allele frequencies: 35.9–78.9% and 18.3–64.3%, respectively, depending on the year). In studies on Greek populations for the presence of kdr mechanism in 1998–2000, a diagnostic dose of dichlorodiphenyltrichloroethane (DDT) was applied to 835 aphid clones (Cox et al., Reference Cox, Denholm, Devonshire, Simon, Dedryver, Rispe and Hullé2004) and only 4.9% of them possessed the mechanism, indicating a rapid increase since 2000 (fig. 2). The M918T mutation has been found at lower frequencies than L1014F. The resistant allele frequencies were higher in peach than in tobacco (2.0–48.1% and 0.0–19.2%, respectively). The frequency of the L1014F and M918T mutations in tobacco samples decreased in the last year of sampling (2015) compared to 2013 (L1014F: 50.0 vs. 18.3%, χ2 = 12.057, df = 1, P < 0.001, M918T: 9.5 vs. 0.0%, Fisher's exact, P = 0.012), a case worthwhile investigating further, which may be related to fitness-cost as the use of pyrethroids is reduced.
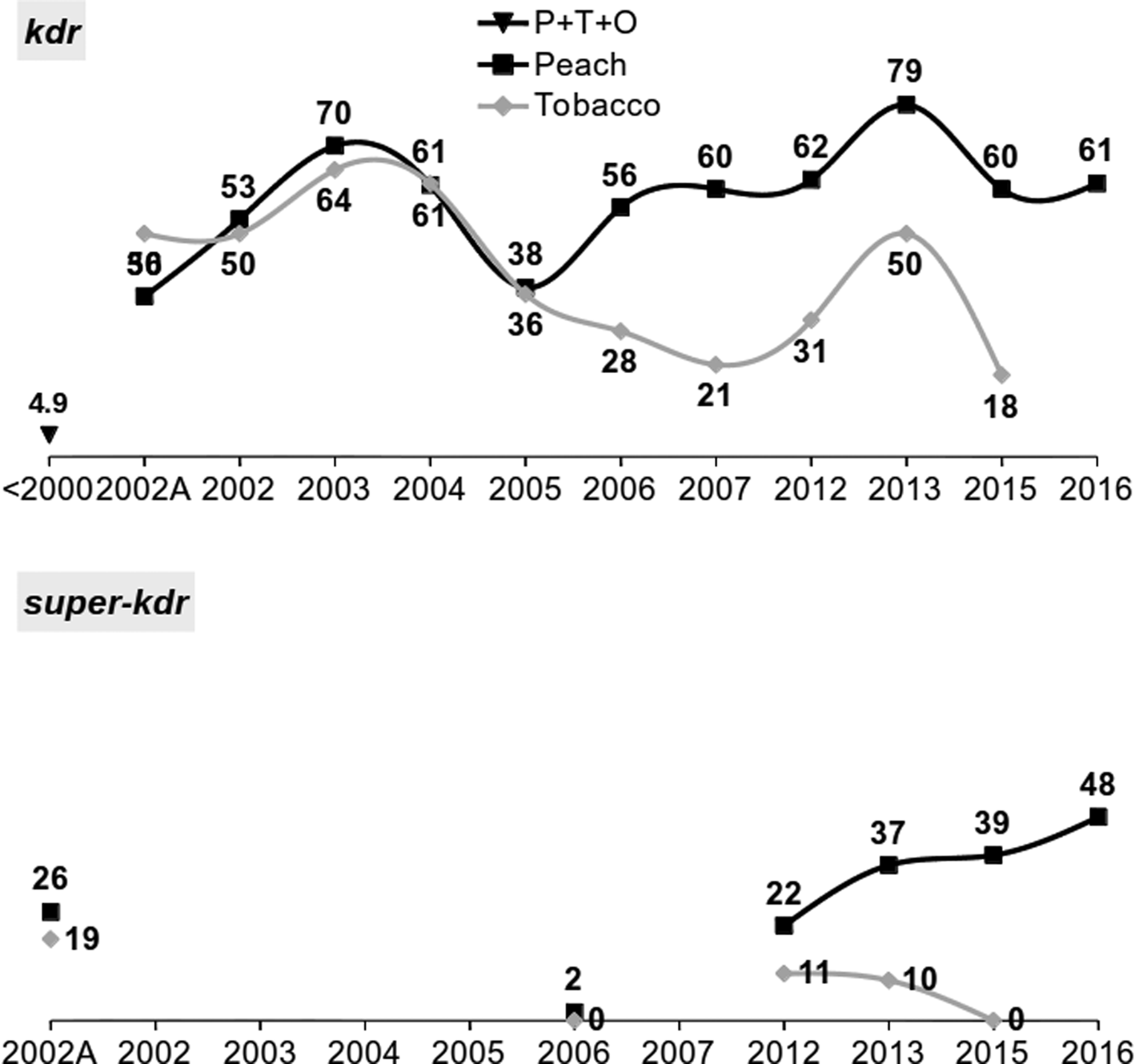
Figure 2. Frequency (%) of kdr (L1014F) and super-kdr (M981 T) resistance alleles. 2002–2016: 1087 and 416 aphids examined for kdr and super-kdr, respectively. 2002A: data from Anstead et al. (Reference Anstead, Mallet and Denholm2007). The frequency for 1998–2000 is an estimation because 835 clones were examined with a diagnostic dose of DDT (Cox et al., Reference Cox, Denholm, Devonshire, Simon, Dedryver, Rispe and Hullé2004).
Surveys were conducted for the presence of the two variants of the M918L mutation in Greece using a modified version of the super-kdr TaqMan assay (Anstead et al., Reference Anstead, Williamson, Eleftherianos and Denholm2004; Williamson, unpublished data). The surveys did not detect the M918L-SE in 15 clones from tobacco and peach collected in 2012–2013 (Voudouris et al., Reference Voudouris, Kati, Sadikoglou, Williamson, Skouras, Dimotsiou, Georgiou, Fenton, Skavdis and Margaritopoulos2016) and 55 clones from peach collected in 2016. On the contrary, the mutation M918L-WE was detected in peach clones in 2015 (N = 40) and 2016 (N = 81), at low frequencies (resistant allele frequencies: 3.0 and 7.1%, respectively). It is not known when the M918L-WE reached Greece as data are only available for the years 2015–2016. In UK, this mutation is common in field samples since 2011 (Foster et al., Reference Foster, Devine, Devonshire, van Emden and Harrington2017).
The metabolic resistance mechanism related to the over-production of carboxylesterases (accounting for 3% of the total proteins in a resistant aphid), conferring resistance to pyrethroids to a certain extent and strong resistance to organophosphates and mono-methyl carbamates, has been recorded at high frequencies on peach and tobacco for 19 years (1998–2013). In total 7797 aphids (from clones or field samples) were tested by a microplate assay for the total carboxylesteraces activity (Devonshire et al., Reference Devonshire, Devine and Moores1992). The assay discriminates the aphid phenotypes in four categories: S = sensitive, R1 = moderately resistant, R2 = highly and R3 = very highly resistant. Variations among years have been observed in the frequencies of resistant phenotypes (R2 + R3 categories), but in most cases they were over 60% and in many cases they reached 80–100%. The frequencies of susceptible (S phenotype) aphids were very low during all years (fig. 3)
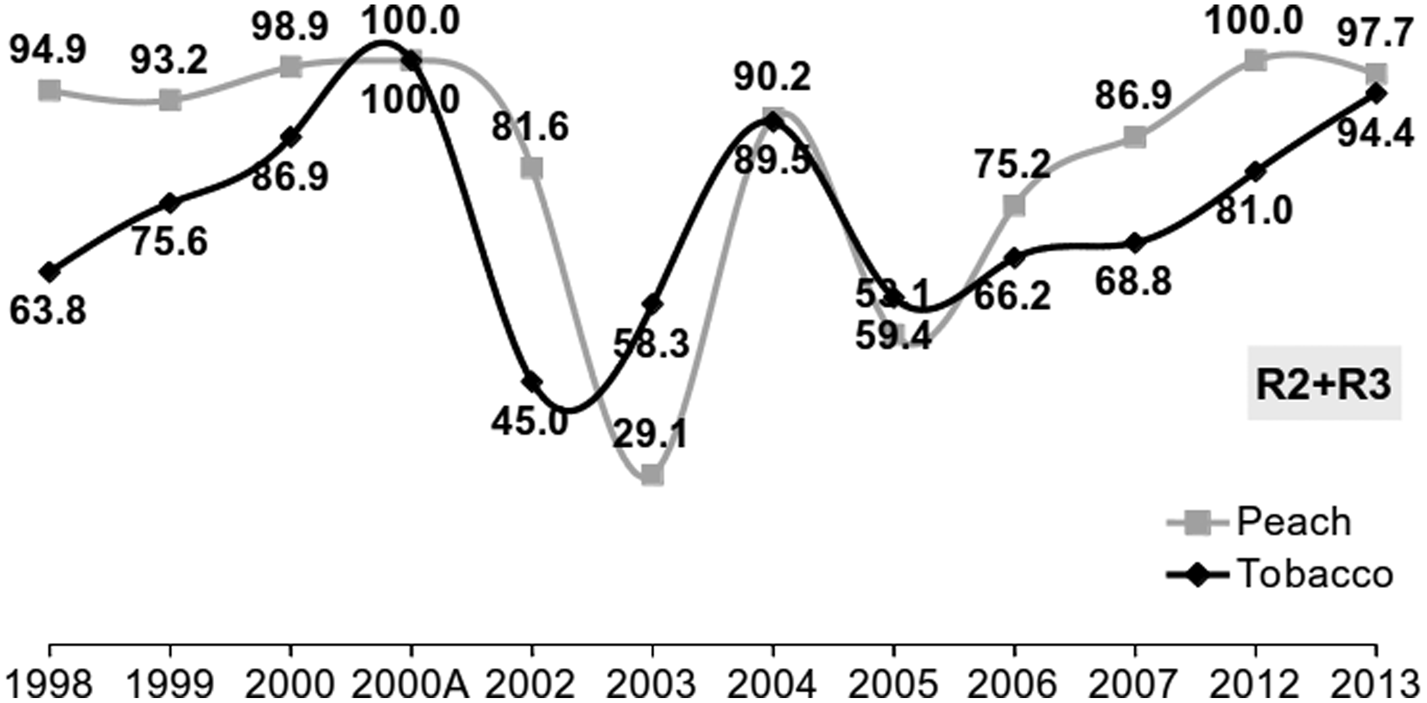
Figure 3. Frequency (%) of resistance Myzus persicae clones with carboxylesterase overproduction (R2 + R3 resistance categories). 1998–2000: data from Cox et al. (Reference Cox, Denholm, Devonshire, Simon, Dedryver, Rispe and Hullé2004); 2002A: data from Anstead et al. (Reference Anstead, Mallet and Denholm2007) (N = 7797).
In conclusion, both primary and secondary mechanisms of pyrethroid resistance are recorded at high frequencies in Greek aphid populations, especially in peach. In 2015–2016, a variant of the super-kdr mutation was found, a matter requiring further investigation.
Carbamates and organophosphates
Di-methyl carbamates
Di-methyl carbamates, with main representatives pyrimicarb and triazamate, have been used successfully as aphicides in the past. Pirimicarb, in particular (currently licensed in Greece) due to its low toxicity to natural enemies (Unal and Jepson, Reference Unal and Jepson1991) has been a valuable tool within the framework of IPM, especially in countries with problems with high frequencies of the carboxylesterace overproduction metabolic resistance mechanism (Foster et al., Reference Foster, Harrington, Dewar, Denholm and Devonshire2002b; Bass et al., Reference Bass, Puinean, Zimmer, Denholm, Field, Foster, Gutbrod, Nauen, Slater and Williamson2014). However, a resistant mechanism was found in M. persicae populations in Greece in 1990. The mechanism, named MACE (Modified AChE), refers to the modification of acetylcholinesterase (AChE), the insecticide target (S431F mutation to the gene ace-1 of AChE). In biochemical assays, MACE reduced the sensitivity of AChE >100 times to pirimicarb (Moores et al., Reference Moores, Devine and Devonshire1994; Nabeshima et al., Reference Nabeshima, Kozaki, Tomita and Kono2003). This translates to resistance by 120 to >500 times to pirimicarb, according to the results of bioassays made on resistant clones of M. persicae (heterozygotes for the MACE mechanisms) (Moores et al., Reference Moores, Devine and Devonshire1994). Later, MACE was also found in Japan, South America, UK in 1995, having spread worldwide (Devonshire et al., Reference Devonshire, Field, Foster, Moores, Williamson and Blackman1998; Foster et al., Reference Foster, Harrington, Dewar, Denholm and Devonshire2002b; Anstead et al., Reference Anstead, Mallet and Denholm2007).
During 2004–2007, field samples from tobacco (N = 59) and peach (N = 39) were tested in dose response bioassays (FAO-dip test) with pirimicarb (Skouras, Reference Skouras2009). Also, the frequency of MACE in the two crops has been recorded for 21 years (1998–2013, 2015, 2016 and 2018), either by a biochemical (Cox et al., Reference Cox, Denholm, Devonshire, Simon, Dedryver, Rispe and Hullé2004; Anstead et al., Reference Anstead, Mallet and Denholm2007; Margaritopoulos et al., Reference Margaritopoulos, Skouras, Nikolaidou, Manolikaki, Maritsa, Tsamandani, Kanavaki, Bacandritsos, Zarpas and Tsitsipis2007b) or PCR-RFLP method (Voudouris et al., Reference Voudouris, Kati, Sadikoglou, Williamson, Skouras, Dimotsiou, Georgiou, Fenton, Skavdis and Margaritopoulos2016).
High RF values (20–122) were observed in 70.4% of the samples compared to low RF values (<10) in 11.2% of the samples. The results of the high resistance observed in many samples suggested problems in the control of the aphid, and/or in spraying failures (Skouras, Reference Skouras2009). The reanalysis of the data showed high frequencies of resistant samples (RF >15), in all years. In 2004, the frequency was 30.8% and it increased significantly in the following years (81.6, 97.1 and 100% in 2005, 2006 and 2007, respectively; Fisher's exact test, P < 0.001; pairwise comparisons were significant only between 2004 and the other 3 years, P < 0.003). In addition, the mean LC50 values of the total number of samples per year (Supplementary_table_S3) increased significantly year after year (Kruskal–Wallis H test, χ2 = 20.162, df = 3, P < 0.001; post-hoc test: Nemenyi test, P < 0.05 in most pairwise comparisons). In all years, higher mean LC50 values were recorded in tobacco than in peach. However, the difference was statistically different only in 2005 (Kruskal–Wallis H test, χ2 = 5.878, df = 1, P = 0.015). These differences, among crops and years, may be attributed to the different selection pressure (frequency of applications) exerted by pirimicarb on the pest populations resulting in increased resistance.
The MACE resistance mechanism was recorded mainly at high frequencies in samples from peach and tobacco over 21 years. In total, 8007 clones or field samples were tested. There was variation between years in the frequency of resistant phenotypes (pooled frequencies of homozygous and heterozygous aphids are presented, as biochemical assays do not discriminate between heterozygous and homozygous-resistant aphids), but mostly were recorded in frequencies >70% and in some cases they reached 89–100% (fig. 4).
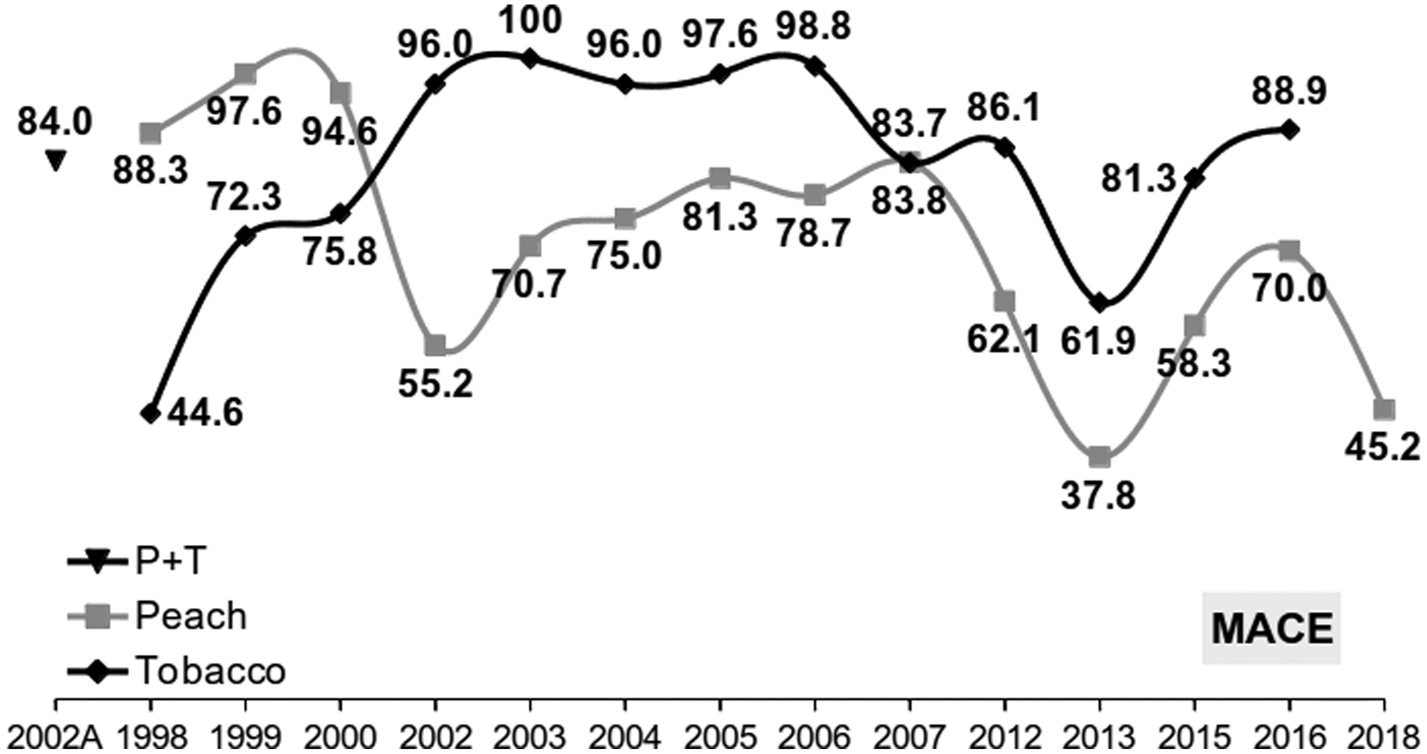
Figure 4. Frequency (%) of aphids with MACE (heterozygotes and resistant homozygotes combined because a biochemical assay was used for pre-2005 samples). 1998–2000: data from Cox et al. (Reference Cox, Denholm, Devonshire, Simon, Dedryver, Rispe and Hullé2004); 2002A: data from Anstead et al. (Reference Anstead, Mallet and Denholm2007) (N = 7676).
Pirimicarb has offered numerous solutions in the past, and it is important to continue monitoring the MACE mechanism in order to know if and to what extent it may be included in aphicide alternation programs.
Organophosphates and mono-methyl carbamates
Organophosphates have been used for aphid control since the 1940s. The first report for resistance to organophosphates was published in the 1950s in aphid populations from the USA (Anthon, Reference Anthon1955). The carboxylesterase-based metabolic mechanism conferring strong resistance to the two insecticide groups, making aphid control impossible, was first described in 1971 (Needham and Sawicki, Reference Needham and Sawicki1971). Since then, the mechanism has been thoroughly studied and the published data show worldwide distribution (Devonshire et al., Reference Devonshire, Field, Foster, Moores, Williamson and Blackman1998; Foster et al., Reference Foster, Harrington, Dewar, Denholm and Devonshire2002b; Anstead et al., Reference Anstead, Mallet and Denholm2007; Bass et al., Reference Bass, Puinean, Zimmer, Denholm, Field, Foster, Gutbrod, Nauen, Slater and Williamson2014).
The carboxylesterace overproduction is due to the amplification of E4 and FE4 structural genes. In resistant aphids, one or both genes -in fewer cases- are amplified (up to ~80 gene copies in resistant aphids). In Greek populations, this mechanism ranged at high levels over 19 years (see ‘Pyrethroid resistance mechanisms’). Molecular analysis of aphid clones collected in various regions of Greece, from peach and herbaceous hosts, in the years 2002–2007 and 2012 (Kati et al., Reference Kati, Mandrioli, Skouras, Malloch, Voudouris, Venturelli, Manicardi, Tsitsipis, Fenton and Margaritopoulos2014) showed that most of them (Ν = 1271) had the FE4 gene and only 3.5% of them the E4, always in combination with FE4. However, in the mid 1990s, E4 was recorded on its own in 20% of the total clones tested (Blackman et al., Reference Blackman, Spence, Field and Devonshire1999). The studies demonstrated that Greek populations are a classic example of evolution in the making (in a ‘perceptible time scale’), where insecticidal applications and cultivation practices, in conjunction with the genetic properties and biological characteristics of the aphid populations, play an important role.
Other aphicides
Pymetrozine
Pymetrozine has specific antifeedant activity on insects feeding in the plant phloem, such as aphids, whiteflies and leafhoppers. It causes irreversible inhibition of feeding, leading to the death of the insect within a few days. It affects the serotonin signaling pathway (neurotransmitter), thus preventing the function of the cibarial dilator muscle (responsible for the functioning of the insect mouthparts), which is innervated with the thoracic ganglion (Kaufmann et al., Reference Kaufmann, Schürmann, Yiallouros, Harrewijn and Kayser2004). The antifeedant action of pymetrozine significantly reduces the transmission of aphid-transmitted plant viruses (Harrewijn and Piron, Reference Harrewijn and Piron1994; Margaritopoulos et al., Reference Margaritopoulos, Tsamandani, Kanavaki, Katis and Tsitsipis2010). Also, it is a selective insecticide with low toxicity to natural enemies of M. persicae (Jansen et al., Reference Jansen, Defrance and Warnier2011).
Pymetrozine, due to its MoA (Group 9), is not affected by the resistance mechanisms already described for M. persicae. There exist few detailed studies on the resistance of pymetrozine worldwide, one with clones mainly from the UK (few from other countries), and one with Greek clones. Foster et al. (Reference Foster, Denholm and Thompson2002a) tested 21 clones with dose-response bioassays (leaf-dip method) and found low RF values (1.0–6.7). Margaritopoulos et al. (Reference Margaritopoulos, Tsamandani, Kanavaki, Katis and Tsitsipis2010) testing clones from peach (Ν = 15) and tobacco (Ν = 27), from various localities in Greece by the leaf-dip method, recording mortality in 4 and 7 days found low RF values (1.0–3.7 and 1.0–6.0 in clones from peach and tobacco, respectively), although there were differences between localities and crops. The highest mean LC50 value was recorded in aphids from tobacco in northern Greece (peach, central Greece: 2.403 ± 0.316 ppm; peach, northern Greece: 2.503 ± 0.238 ppm; tobacco central Greece: 2.719 ± 0.379 ppm; tobacco, northern Greece: 3.510 ± 0.283 ppm). These differences are attributed to more frequent use of pymetrozine, exerting selection pressure on aphid populations in tobacco in northern Greece.
After a decade, we examined with the same bioassay method 14 clones of M. persicae along with our standard susceptible (4106A) and the reference 5191A clone (resistant to various chemical classes of insecticides). The clones were collected from peach and tobacco in central and northern Greece in 2017–2018. The LC50 values of the field collected clones ranged from 2.149 to 4.744 ppm and so was the LC50 value of the susceptible clone 4106A. The reference clone 5191A showed the highest LC50 value (5.407 ppm). The results did not show any resistance, RF values being low, ranging from 1.1 to 2.2 (a field collected clone was used for RF calculations because it showed lower LC50 value than the 4106A clone). However, the LC50 value of nine clones was significantly higher than the most susceptible one (ratio test, P < 0.05) (table 2, Supplementary_table_S4).
The data collected so far show that M. persicae has not developed resistance to pymetrozine in Greece. This agrees with data collected in other countries. Pymetrozine could be used as an alternative to other aphicides, where alternation of different active ingredients is necessary to avoid and/or delay emergence of resistance problems. Due to its antifeedant properties, it could be also used in crops affected by non-persistent viruses. However, according to a recent EU decision, pymetrozine was withdrawn in 2019.
Spirotetramat
Spirotetramat is a relatively new insecticide used against aphids and other plant-sucking insects. It is active mainly against the premature instars of aphids, adversely affecting also female fertility. Its different MoA (Group 23) from other classic aphicides, and the low toxicity to beneficial are advantageous (Nauen et al., Reference Nauen, Reckmann, Thomzik and Thielert2008; Brück et al., Reference Brück, Elbert, Fischer, Krueger, Kühnhold, Klueken, Nauen, Niebes, Reckmann, Schnorbach, Steffens and van Waetermeulen2009; Wang et al., Reference Wang, Gong, Jin, Zhu and Wei2016).
It was registered recently in Greece, hence no resistance studies for M. persicae were available as yet, the first study conducted only recently (Voudouris et al., Reference Voudouris, Williamson, Skouras, Kati, Sahinoglou and Margaritopoulos2017). M. persicae clones from peach (Ν = 30) and tobacco (Ν = 30) were tested in dose response bioassays (leaf-dip test). The mean LC50 values (0.495–1.588 ppm) did not differ significantly among samples from different regions/hosts (Kruskal–Wallis H test, χ2 = 9.258, df = 5, P = 0.099). In addition, there was no resistance recorded with RF values 1.0–3.2, being comparable to an earlier study of three M. persicae clones tested with the same bioassay method (LC50: 0.27–0.69 ppm, RF: 1.0–2.6) (Elbert et al., Reference Elbert, Nauen and Salmon2008). The clones used were a susceptible lab clone and two from Japan and France, resistant to organophosphates, carbamates and pyrethroids. Up to date, spirotetramat has been an effective and valuable tool in aphid control programs, particularly in crops/areas with evidence of resistance to other insecticides.
It is to note, however, that there have been reports on resistance to tetronic acids (including spirotetramat) of other pests in various countries, i.e., mites (Tetranychus and Panonychus) and whiteflies (Trialeurodes vaporariorum Westwood (Hemiptera: Aleyrodidae)) (Van Pottelberge et al., Reference Van Pottelberge, Van Leeuwen, Khajehali and Tirry2009; Kramer and Nauen, Reference Kramer and Nauen2011; Karatolos et al., Reference Karatolos, Williamson, Denholm, Gorman, ffrench-Constant and Nauen2012). Recently, a metabolic-resistance mechanism to spirotetramat was reported for Α. gossypii. A lab strain from China, after selection with spirotetramat (579- and 15-fold resistance in adults and third instar nymphs, respectively) showed significantly increased transcriptional levels of the CYP6A2 P450 gene compared to the susceptible strain, suggesting its involvement in the spirotetramat resistance (Pan et al., Reference Pan, Yang, Gao, Peng, Bi, Xi, Xin, Zhu, Wu and Shang2015; Peng et al., Reference Peng, Pan, Yang, Gao, Xi, Wu, Huang, Zhu, Xin, Zhan and Shanget2016).
These reports suggest, possible resistance development to pests in crops under intense chemical control programs. M. persicae has the ability to detoxify xenobiotic molecules. A characteristic example is the gene CYP6CY3, metabolizing nicotine and detoxifying neonicotinoids (Puinean et al., Reference Puinean, Foster, Oliphant, Denholm, Field, Millar, Williamson and Bass2010; Bass et al., Reference Bass, Puinean, Zimmer, Denholm, Field, Foster, Gutbrod, Nauen, Slater and Williamson2014). Considering the biological characteristics of aphids (short generation time, high fecundity, high population increase rates, combination of sexual and parthenogenetic reproduction), and the relatively fast resistance evolution over perceptible timescales already reported in M. persicae (Fenton et al., Reference Fenton, Margaritopoulos, Malloch and Foster2010b; Kati et al., Reference Kati, Mandrioli, Skouras, Malloch, Voudouris, Venturelli, Manicardi, Tsitsipis, Fenton and Margaritopoulos2014), the selection of similar P450-based mechanisms, capable of detoxifying spirotetramat, is a possibility, especially in scenarios of intensive use of this compound. To ensure sustainable use of spirotetramat, strict guidelines for prevention and management of resistance is to be followed (i.e., reduction of applications, alternation of active ingredients with different MoA) (Elbert et al., Reference Elbert, Nauen and Salmon2008).
Flonicamid
Flonicamid belongs to the pyridinecarboxamide group and it is active against nymphs and adult aphids. It causes rapid (within 0.5 h post-treatment) and irreversible inhibition of feeding. The stylets of the aphids cannot penetrate plant tissues, leading to insect death within a few days, due to starvation. Flonicamid acts in the aphid nervous system, disrupting the function of chrodotonal stretch receptor organs, the target site not defined as yet. Although the insecticidal properties of flonicamid (antifeedant activity) resemble those of pymetrozine, the two insecticides have different MoA (Group 29 vs. Group 9) (Morita et al., Reference Morita, Ueda, Yoneda, Koyanagi and Haga2007, Reference Morita, Yoneda and Akiyoshi2014). In addition, flonicamid is a selective insecticide with null to low toxicity to beneficial arthropods (Jansen et al., Reference Jansen, Defrance and Warnier2011; Morita et al., Reference Morita, Yoneda and Akiyoshi2014), and due to its antifeedant activity it can be used against aphid-transmitted plant viruses. The target mechanism of flonicamid, being novel, it is not affected by the resistance mechanisms already described in M. persicae. There have not been any reports for resistance development to flonicamid by M. persicae or other aphid species.
Flonicamid has been used in Greece against M. persicae since 2010. We studied the effectiveness of this compound on 57 M. persicae clones collected from peach and tobacco in northern, central and southern Greece during 2017–2019 in dose response bioassays (leaf-dip method, mortality scored 7 days post-treatment; Voudouris et al., Reference Voudouris, Williamson, Skouras, Kati, Sahinoglou and Margaritopoulos2017) and compared with the susceptible clone 4106A and the reference clones 5191A, 5444B and FRC, resistant to various chemical classes of insecticides.
The lowest LC50 value (1.091 ppm) was observed in the susceptible clone 4106A. The LC50 values of the 57 field collected clones ranged from 1.101 to 6.212 ppm and those of the reference clones 5191A, 5444B and FRC were within 4.082–6.170 ppm. The RF values were low, ranging from 1.0 to 5.7, suggesting no resistance development. However, some qualitative differences were observed in regard to response to flonicamid among the examined clones. The LC50 of 53 field collected clones and of the three reference clones (5191A, 5444B and FRC) was significantly different (ratio test, P < 0.05) from that of the susceptible one (table 2, Supplementary_table_S5). Whether this variation is an indication for initiation of resistance development, especially under scenarios of intense selection pressure, remains to be seen.
The data collected so far, >50 clones collected from various regions of Greece from peach and tobacco, where aphid populations are under intense chemical control, suggest that Greek populations have not developed resistance to flonicamid, being an effective aphicide appropriate for IRM strategies, considering also its antifeedant property for use against the spread of non-persistent viruses. Monitoring for resistance is, however, important.
Synopsis-conclusions
The present article summarizes data of long term studies on the resistance of Greek M. persicae populations to insecticides of various chemical classes and MoA. The data analyzed and the extensive bibliography on this field, show that the aphid is a difficult to control pest, developing highly resistant populations, a challenge for the designers and applicants of IPM and IRM programs. To that also contributes, the seven resistance mechanisms described, the continuous selection pressure by insecticidal applications, as well as certain characteristics of the species' bio-ecology such as: (i) high genetic variability and adaptability, (ii) dispersal/migration between crops and localities, (iii) multiple generations per year, and, (iv) complex life cycle including holocyclic and functional parthenogenetic genotypes with interbreeding.
With the onset of resistance, in cases of high frequency of resistance alleles, its reversal is difficult, as demonstrated in the case of Plutella xylostella (L.) (Lepidoptera: Plutellidae) in Hawai (Sparks and Nauen, Reference Sparks and Nauen2015). A classic example is the populations of M. persicae in Greece (especially those from peach), where resistance mechanisms (carboxylesterases, MACE, kdr) range from moderate to very high levels for over 15 years. This differentiates Greek populations from those of Western Europe, where there has been a reduction of the MACE and carboxylesterace-based mechanisms and increase of the kdr, when the use of organophosphates and pirimicarb was reduced while the use of pyrethroids and neonicotinoids was increased. This reduction has been attributed to factors related to the differential selection pressure applied to the aphid populations in combination with the heavy fitness-cost of the carboxylesterase-based mechanism and its close association with MACE (Foster et al., Reference Foster, Denholm and Devonshire2000, Reference Foster, Harrington, Dewar, Denholm and Devonshire2002b; Field and Foster, Reference Field and Foster2002). The persistence of the aforementioned resistance mechanisms in Greece could be attributed to the following: (i) winters are milder and selection is expected to be weaker against the parthenogenetic-resistant genotypes (overwinter as nymphs or adults on winter hosts) that suffer from fitness cost (resistant genotypes have lower survival at winter temperatures than the susceptible ones) (Foster et al., Reference Foster, Harrington, Dewar, Denholm and Devonshire2002b; Fenton et al., Reference Fenton, Margaritopoulos, Malloch and Foster2010b), (ii) the sexual reproduction is extensive in Greece (Blackman et al., Reference Blackman, Malarky, Margaritopoulos and Tsitsipis2007), and holocyclic genotypes overwinter at the cold-tolerant diapause egg stage on peach, and, (iii) due to sexual reproduction, there take place genetic recombination and that selection could generate novel-resistant genotypes with competitive biological traits, counterbalancing any fitness costs, associated with insecticide resistance, as occurred in Scottish genotypes (Fenton et al., Reference Fenton, Kasprowicz, Malloch and Pickup2010a). These traits might then pass to long persistent functional parthenogens, as they co-exist with holocyclic genotypes, even within the same locality (Blackman et al., Reference Blackman, Malarky, Margaritopoulos and Tsitsipis2007), and there exists a degree of gene-flow between these two categories of genotypes (Vorburger et al., Reference Vorburger, Lancaster and Sunnucks2003; Margaritopoulos et al., Reference Margaritopoulos, Malarky, Tsitsipis and Blackman2007a; Kati et al., Reference Kati, Mandrioli, Skouras, Malloch, Voudouris, Venturelli, Manicardi, Tsitsipis, Fenton and Margaritopoulos2014).
Sexual reproduction together with the high migration/dispersal rates of aphids favour the dispersal of the resistance genes, leading to difficulties in aphid control. In Greece, the aphid exhibits high levels of genetic variation, due to its ability to reproduce sexually (Blackman et al., Reference Blackman, Malarky, Margaritopoulos and Tsitsipis2007; Margaritopoulos et al., Reference Margaritopoulos, Malarky, Tsitsipis and Blackman2007a; Zepeda-Paulo et al., Reference Zepeda-Paulo, Simon, Ramírez, Fuentes-Contreras, Margaritopoulos, Wilson, Sorenson, Briones, Azevedo, Ohashi, Lacroix, Glais and Figueroa2010), and especially the peach-growing regions may be considered as ‘hot-spots’, where new genotypes with multiple resistance mechanisms are generated (Margaritopoulos et al., Reference Margaritopoulos, Skouras, Nikolaidou, Manolikaki, Maritsa, Tsamandani, Kanavaki, Bacandritsos, Zarpas and Tsitsipis2007b; Voudouris et al., Reference Voudouris, Kati, Sadikoglou, Williamson, Skouras, Dimotsiou, Georgiou, Fenton, Skavdis and Margaritopoulos2016), through sexual reproduction. The combinations of resistance genes may be conserved, at the parthenogenetic phase of the life cycle during the growing season, because of selection pressure from insecticides. These may pass to long persistent functional parthenogens, through gene-flow between these two types of genotypes. A hypothetical example of resistance development in M. persicae in regions where, the aphid invests in two life cycle strategies (cyclical parthenogenesis and anolocycly) is shown in fig. 5.
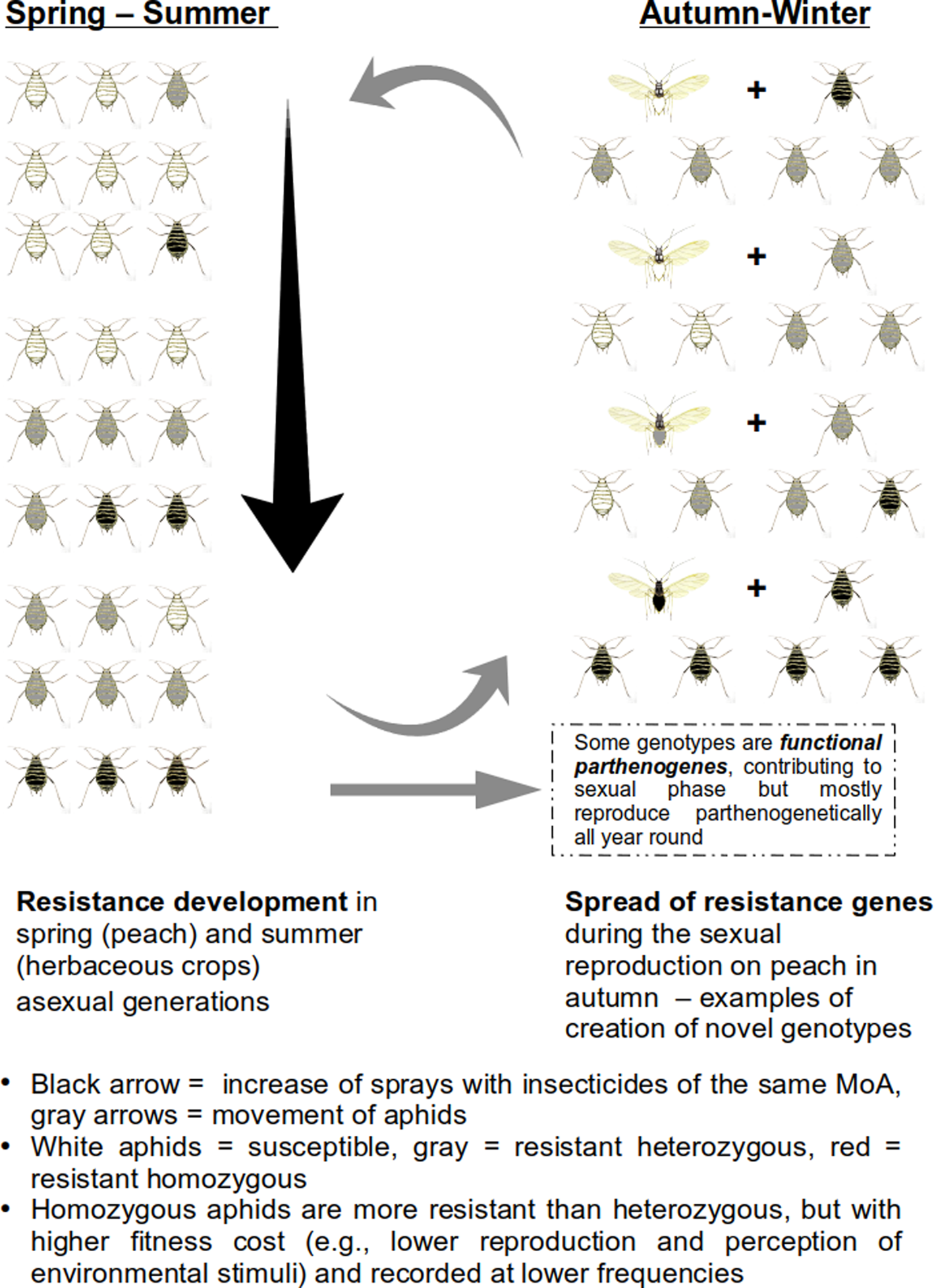
Figure 5. Scenario of resistance development in Myzus persicae.
A basic element of IRM is the alternation of insecticides with different MoA through the crop season (‘window’ approach). In Greece, the aphid shows high resistance to dimethyl-carbamate and pyrethroid aphicides. Given the tendency of the Greek aphid populations for neonicotinoid-resistance development, spirotetramat and flonicamid possess characteristics (different MoA, no resistance observed) making them suitable for cyclical alternation with neonicotinoids. It is worth noticing, that the efficacy of spirotetramat and flonicamid have been tested on a large number of aphid clones from various regions of Greece from peach and tobacco, and thus genotypes investing in different life cycle strategies were included. We are not aware of any other published study examining the resistance to flonicamid in a large number of aphid samples.
Another aphicide, that can take part in rotation programs is flupyradifurone (betunolide), registered in Greece in 2018. It has the same MoA (Group 4) with neonicotinoids, but it belongs to a different chemical class (4D and 4A respectively). We lack bioassay data for Greek populations given its recent registration. Nauen et al. (Reference Nauen, Jeschke, Velten, Beck, Ebbinghaus-Kintscher, Thielert, Wölfel, Haas, Kunz and Raupach2015) reported high insecticidal activity of flupyradifurone, against a strain of M. persicae with moderate (20-fold) resistance to imidacloprid, indicating a lack of cross-resistance between neonicotinoids and flupyradifurone. It is worth mentioning, however, that Bass et al. (Reference Bass, Denholm, Williamson and Nauen2015) reported high resistance (200-fold) in a clone from Spain, homogygous for the R81T mutation. The aphicide sulfoxaflor is another alternative, it has the same MoA with neonicotinoids, classified, however, to a different chemical group (4C). There is no data on Greek populations. However, the R81T mutation seems to influence its efficacy, although at a lower rate than neonicotinoids (Cutler et al., Reference Cutler, Slater, Edmunds, Maienfisch, Hall, Earley, Pitterna, Pal, Paul, Goodchild, Blacker, Hagmann and Crossthwaite2013; Bass et al., Reference Bass, Denholm, Williamson and Nauen2015). Furthermore, Wang et al. (Reference Wang, Gong, Chen, Su, Cao, Hoffmann and Wei2018) reported a 199-fold resistance in M. persicae, after selection in the laboratory for 45 generations. To some authors, sulfoxaflor is considered a ‘neonicotinoid’, in insecticide rotation programs that can be used as alternative to compounds such as flonicamid and spirotetramat, but not to neonicotinoids as they belong to the same MoA (Cutler et al., Reference Cutler, Slater, Edmunds, Maienfisch, Hall, Earley, Pitterna, Pal, Paul, Goodchild, Blacker, Hagmann and Crossthwaite2013).
Although available tools for aphid control and resistance management have been reduced, farmers in Greece still have an adequate number of aphicides (flonicamid, spirotetramat, flupyradifurone and sulfoxaflor), and with a prudent use, in rotation strategies, they (along with the remaining neonicotinoids) may maintain their effectiveness. A key factor, often ignored in the implementation of IPM/IRM strategies, in regions where M. persicae invests in sexual reproduction, is the migration from peach to herbaceous crops and vice versa, and the occurrence of several parthenogenetic generations in both crops. Plant protection practices applied in peach orchards affect populations in herbaceous crops and vice versa. The target should be the agroecosystem of peach–herbaceous crops, and special care should be given to the placement of each compound during the whole growing season (Spring to Autumn) and not to each crop individually, in order to avoid continuous selection of resistant genotypes (amplified with parthenogenetic reproduction), which leads to homogeneous-resistant populations. The situation is more complicated when protection against aphid-transmitted non-persistent viruses in herbaceous crops is concerned. In this case, flonicamid, could be a valuable tool.
Epilogue
M. persicae s.l. exhibits high genetic variation and adaptability, with evolutionary events related to insecticide resistance that have been recorded in perceptible timescales (Fenton et al., Reference Fenton, Margaritopoulos, Malloch and Foster2010b; Kati et al., Reference Kati, Mandrioli, Skouras, Malloch, Voudouris, Venturelli, Manicardi, Tsitsipis, Fenton and Margaritopoulos2014). It is difficult to control pest, due to fast development of resistance to almost all classes of insecticides.
The stakeholders involved in plant protection need to be alert for early onset of resistance (e.g., recent increase of resistance to neonicotinoids in Greece with concurrent spread of the R81T mutation) to design and implement timely effective control measures as soon as possible. Resistance is a time-persistent problem and continuous monitoring is fundamental in combination with resistance history, especially in crops under intense chemical control.
IRM should rely on ‘windows approaches’ with rotation of insecticides of different MoA, population monitoring, timely application and consideration of the pest's biology. An important aspect is the metabolic resistance that could jeopardize IRM strategies considering the broad substrate spectra of monooxygenases (Scott and Wen, Reference Scott and Wen2001) as it is known that novel active ingredients, never used before, showed reduced effectiveness soon after their registration (Sauphanor and Bouvier, Reference Sauphanor and Bouvier1995).
New knowledge emerges on the effect of various insecticides in the environment. Recent decisions on banning or restricting the use of certain products are issues to be considered in the design of IRM programs, which have to be dynamic and to foresee the implementation of alternative methods of control, for which there is an accumulation of data (Hance et al., Reference Hance, Kohandani-Tafresh, Munaut, van Emden and Harrington2017). Additionally, existing knowledge on the effect of insecticides on genes opens new horizons in understanding their role in the expression of resistance, that may eventually lead to its more essential management (Meng et al., Reference Meng, Chen and Zhang2019).
Supplementary material
The supplementary material for this article can be found at https://doi.org/10.1017/S0007485320000334.
Acknowledgements
The authors are grateful to various colleagues for assistance with the collection of aphids, bioassays and diagnostics. Especially, they are grateful to Martin Williamson (Rothamsted Research) for his help and collaboration during the last 15 years on various aspects of the research on insecticide resistance in Greek M. persicae populations. The research was supported by Greek national and European Union funds in the framework of various research projects such as ‘PYTHAGORAS II’ and ‘PENED 03’ ‘THALIS’ project 380264, ‘Supporting Postdoctoral Researchers’, ‘Research & Technology Development Innovation Projects’ (AgroETAK, MIS 453350), and project 96/T/18 ‘Management of the insect pests and viruses using ecologically compatible technologies’. We also thank K.&N. EFTHYMIADIS S.A. for partially funding of the monitoring of resistance in flonicamid and acetamiprid.