Introduction
Toxocara canis is an important gastrointestinal helminth found in the small intestine of dogs and foxes (Kassai, Reference Kassai1999; Schnieder et al., Reference Schnieder, Laabs and Welz2011). This helminth has a global distribution, which is investigated with necropsy and faecal examinations in the host. Humans are also paratenic hosts for T. canis. In humans, contamination starts with ingesting the embryonated eggs of T. canis by accident (which can be found in contaminated playgrounds and parks, in raw vegetables and fruits, in paratenic hosts, such as earthworms and rabbits, etc., and in cat or dog hair); then, the embryonated eggs enter the intestines (L3 release) and migrate into the organs (Kassai, Reference Kassai1999; Despommier, Reference Despommier2003; Smith et al., Reference Smith, Holland, Taylor, Magnaval, Schantz and Maizels2009; Lee et al., Reference Lee, Schantz, Kazacos, Montgomery and Bowman2010; Schnieder et al., Reference Schnieder, Laabs and Welz2011). According to the severity of the infection, toxocariasis is clinically classified as visceral larva migrans (VLM), neurological toxocariasis (NT), ocular larva migrans (OLM) or covert toxocariasis (Strube et al., Reference Strube, Heuer and Janecek2013). The diagnosis of VLM is difficult, and the differential diagnosis of the larval development stage is limited. Furthermore, since most cases are rather mild and transient, a case of VLM might be overlooked or misdiagnosed (Roberts & Janovy, Reference Roberts and Janovy2006).
Today, serological tests (e.g. TES-ELISA and TES-WB) are used in the routine diagnosis of toxocariasis in humans (Smith & Noordin, 2006; Li et al., Reference Li, Lin, Chen, Sani, Song and Zhu2007). The results of human toxocariasis seroprevalence studies show the following prevalence ratios: 5.1% in the US (Berrett et al., Reference Berrett, Erickson, Gale, Stone, Brown and Hedges2017); 50.6% in southern Brazil (Schoenardie et al., Reference Schoenardie, Scaini, Brod, Pepe, Villela, McBride, Borsuk and Berne2013) and 63.6% in north-eastern Brazil (Silva et al., Reference Silva, Amor and Santos2017); 38.33% in Argentina (Archelli et al., Reference Archelli, Santillan, Fonrouge, Céspedes, Burgos and Radman2014); 15.3% in western Slovakia (Boldis et al., Reference Boldis, Ondriska, Spitalska and Reiterova2015); 14.5% in Poland (Jarosz et al., Reference Jarosz, Mizgajska-Wiktor, Kirwan, Konarski, Rychlicki and Wawrzyniak2010); 7.7% in Egypt (Ei-Shazly et al., Reference Ei-Shazly, Abdel Baset, Kamal, Mohammed, Sakr and Hammad2013); 44.6% in Swaziland, southern Africa (Liao et al., Reference Liao, Sukati and D'lamini2010) and 59.9% in Gabon, central Africa (Lötsch et al., Reference Lötsch, Obermüller and Mischlinger2016); 19.27% in Shandong and Jilin Provinces, China (Cong et al., Reference Cong, Meng, You, Zhou, Dong, Dong, Wang, Qian and Zhu2015); and 2–45.9% in Turkey (Akyol et al., Reference Akyol, Bicerol, Ertug, Ertabaklar and Kıylıoglu2007; Kustimur et al., Reference Kustimur, Dogruman Al, Oguzulgen, Bakır, Maral, Turktas and Tuzun2007; Kaplan et al., Reference Kaplan, Kalkan, Kuk, Demırdag, Ozden and Kılıc2008).
However, these serological tests cannot distinguish which species of parasite (e.g. T. canis and T. cati) have caused this syndrome. In patients with multiparasitism, cross-reactions may be observed. For this reason, it is necessary to use molecular techniques to identify the aetiology and causative agents of human toxocariasis (Gasser et al., Reference Gasser, Zhu, Hu, Jacobs, Chilton, Holland and Smith2006; Smith & Noordin, 2006; Li et al., Reference Li, Lin, Chen, Sani, Song and Zhu2007).
Due to the promising molecular studies that have been carried out in this subject, the following study was devised to examine this topic further. VLM was created with embryonated T. canis eggs in BALB/c mice to achieve a diagnosis not only for the tissue most commonly affected by larval migration (the liver) but also for the limb muscles, lungs and brain. This study aimed to measure the sensitivity, specificity and accuracy of molecular diagnosis and determine the detection limit of the method. Another aim of the study was to compare molecular diagnosis with a conventional method: pepsin-HCl digestion.
Materials and methods
Sample collections
The ascarid samples were obtained from infected dogs and cats that were referred to parasitological examination at Ankara University, Faculty of Veterinary Medicine. Ascarids were collected after treatment with pyrantel pamoate (Kontil®, 5 mg/kg, peroral) in infected hosts and diagnosed morphologically. The adult T. canis females were used for infection of BALB/c and as a positive control for Polymerase chain reaction (PCR), while adult T. cati and T. leonina were used to measure the sensitivity of the PCR. The mature female T. canis were shredded into distilled water with a scalpel, and the eggs were removed. Next, 1% sodium hypochlorite was added to the suspension and stirred for 10 min on a magnetic stirrer. After 10 min of stirring, sodium hypochlorite was removed by repeated centrifugation (2000 rpm × 3 min; three times). Then, 0.5% formalin was added, and the suspension was transferred to Petri dishes and incubated at 26–27°C for 23 days. Egg-larval development was monitored with a microscope (daily control).
VLM syndrome model in BALB/c mice
The syndrome is tolerated, and larvae can survive notably well in the tissues of BALB/c mice. Some researchers have stated that BALB/c mice are the best animal model for toxocariasis studies (Akao, Reference Akao, Holland and Smith2006; Strube et al., Reference Strube, Heuer and Janecek2013); therefore, in the present study, BALB/c mice were used as an animal model.
Prior studies have shown that VLM may be induced by a wide range of embryonated T. canis eggs (6–5000) in mice (Sprent, Reference Sprent1955, Reference Sprent1958; Oshima, Reference Oshima1961; Sinha, Reference Sinha1966; Burren, Reference Burren1968, Reference Burren1971; Bisseru, Reference Bisseru1969; Abo-Shehada & Herbert, Reference Abo-Shehada and Herbert1984/1985; Ollero et al., Reference Ollero, Fenoy, Cuellar, Guillen and Aguila2008). In light of these studies, BALB/c were firstly infected with 2000 embryonated eggs as a pre-study. However, mass mortality was observed 2–3 days post-infection with this number of embryonated eggs. In the necropsy reports, the infection was identified as the cause of death. Based on this information, the infection dose was decreased to 1000 embryonated eggs.
On the 23rd day of incubation, the suspension was centrifuged three times at 2000 rpm for 2 min with 0.9% isotonic saline solution to remove formalin. Six- to eight-week-old female BALB/c mice were infected with 1000 embryonated T. canis eggs in 0.2 ml of 0.9% isotonic saline via intragastric lavage. Non-infected mice were given 0.2 ml of 0.9% isotonic saline solution.
The day upon which embryonated eggs were given to the BALB/c mice was accepted as ‘day 0’ of the infection. On the second, fourth, seventh and 14th days post-infection, six infected and three non-infected BALB/c mice were sacrificed (n = 36). The procedure was conducted by taking blood from the heart of each animal under xylazine–ketamine anaesthesia (xylazine: 5 mg/kg im; ketamine: 45 mg/kg im). The BALB/c mice were necropsied immediately.
This research was carried out with the approval of the Gazi University Animal Experiments Local Ethics Committee (approval number G.Ü.ET.10.038).
Pepsin-HCl digestion
The liver, lungs, brain, forelimb and hindlimb muscles were removed, and the weight of each organ was recorded. The organs were divided into two parts. One part of each organ was weighed and digested with pepsin-HCl, except for the brain tissue. Instead of using pepsin-HCl, brain samples were squashed and observed with a microscope (10×). The other parts of each organ were stored at −80°C for DNA isolation until they were used.
For digestion, the organs were finely cut, and pepsin-HCl was added. The tissue samples were incubated at 37°C for 2–3 days; next, larval presence/absence was detected, and larvae were counted.
DNA extraction
The 20-mg BALB/c mice tissue samples were homogenized with phosphate-buffered saline (PBS) in an ultrasonic homogenizer (HD2070, Bandelin, Germany). Subsequently, a commercial DNA purification kit was used (K0722, Thermo Scientific, Lithuania) according to the manufacturer's instructions. The amount of DNA was measured with a NanoDrop™ 2000 spectrophotometer (ND2000, Thermo Scientific, USA) and stored at −20°C until use.
The DNA isolation protocol of Sambrook et al. (Reference Sambrook, Fritschi and Maniatis1989) was applied for morphologically diagnosed adult T. canis, T. cati and T. leonina. The amount of DNA was measured and stored at −20°C.
The T. canis eggs were incubated in distilled water at 26–27°C for 23 days. During the incubation period, the eggs were checked and ventilated. On the 23rd day of incubation, the eggs were centrifuged with PBS four times for 5 min at 2000 rpm. In this experiment, 1, 2, 3, 4, 5, 6, 7, 8, 9 and 10 embryonated eggs were added to each of the ten 1.5-ml tubes. A DNA purification kit (K0722, Thermo Fisher Scientific, Lithuania) was used according to the manufacturer's instructions. The DNA samples were stored at −20°C until use.
PCR–RFLP technique
Forward (T. canis ATP-F1: GTTTGTTGTTTTGGGGGCTA) and reverse (T. canis ATP-R1: CCAAAGGACGAGAAACCTCA) primers were used to amplify a 217-bp region of the adenosine triphosphate (ATP) synthase subunit-6 (ATPase subunit 6) gene of T. canis. The primers were designed for this study. PCR was carried out in a 30-μl total volume mix containing 10× Taq buffer (1.25 ml, including (NH4)2SO4), 25 mmol/l of MgCl2, 10 mM of dNTP mix, 5 U/μl Taq DNA Polymerase (Fermentas, Waltham, MA, USA), molecular biology grade water (18 megohm-cm, Applichem, Darmstadt, Germany) and 10 pmol/μl primers. Ten microliters of template DNA was added to each reaction. Each sample was analysed (T1 thermocyler, Biometra, Germany) under the following conditions: 94°C for 5 min (initial denaturation) followed by 34 cycles at 94°C for 30 s (denaturation), 50°C for 1 min (annealing), 72°C for 1 min (extension) and 72°C for 10 min (final extension). Amplicons were detected on 3% agarose (TAE) gel with ethidium bromide staining. The gel was visualized under ultraviolet light with a gel-imaging system (Kodak Gel Logic 100, USA).
The positive amplicons were digested with BbsI restriction endonuclease (New England BioLabs Inc., UK; 5000 units/ml) at 37°C for 1 h. The fragments were detected on 3% agarose (TAE) gel with ethidium bromide staining and visualized with the gel-imaging system.
Statistical analysis
Descriptive statistics of the pepsin-HCl digestion and PCR results were obtained. The Mann–Whitney U-test was used for the PCR results (+/−) of the number of larvae detected in different tissue types. The Kruskal–Wallis test and Bonferroni correction test were performed to determine the differences in larval counts among tissues and the changes in larval counts during independent days of all necropsy. The frequency of occurrence was examined via a chi-square test. P-values <0.05 were considered to be statistically significant in the analysis of the results. Specificity, sensitivity and accuracy rates of the PCR results were calculated (SPSS 14.01-9869264).
Results
A total of 36 BALB/c mice were sacrificed on the second, fourth, seventh and 14th days post-infection. The results are shown in table 1.
Table 1. Results of pepsin-HCl digestion and PCR reactions during the necropsy days.
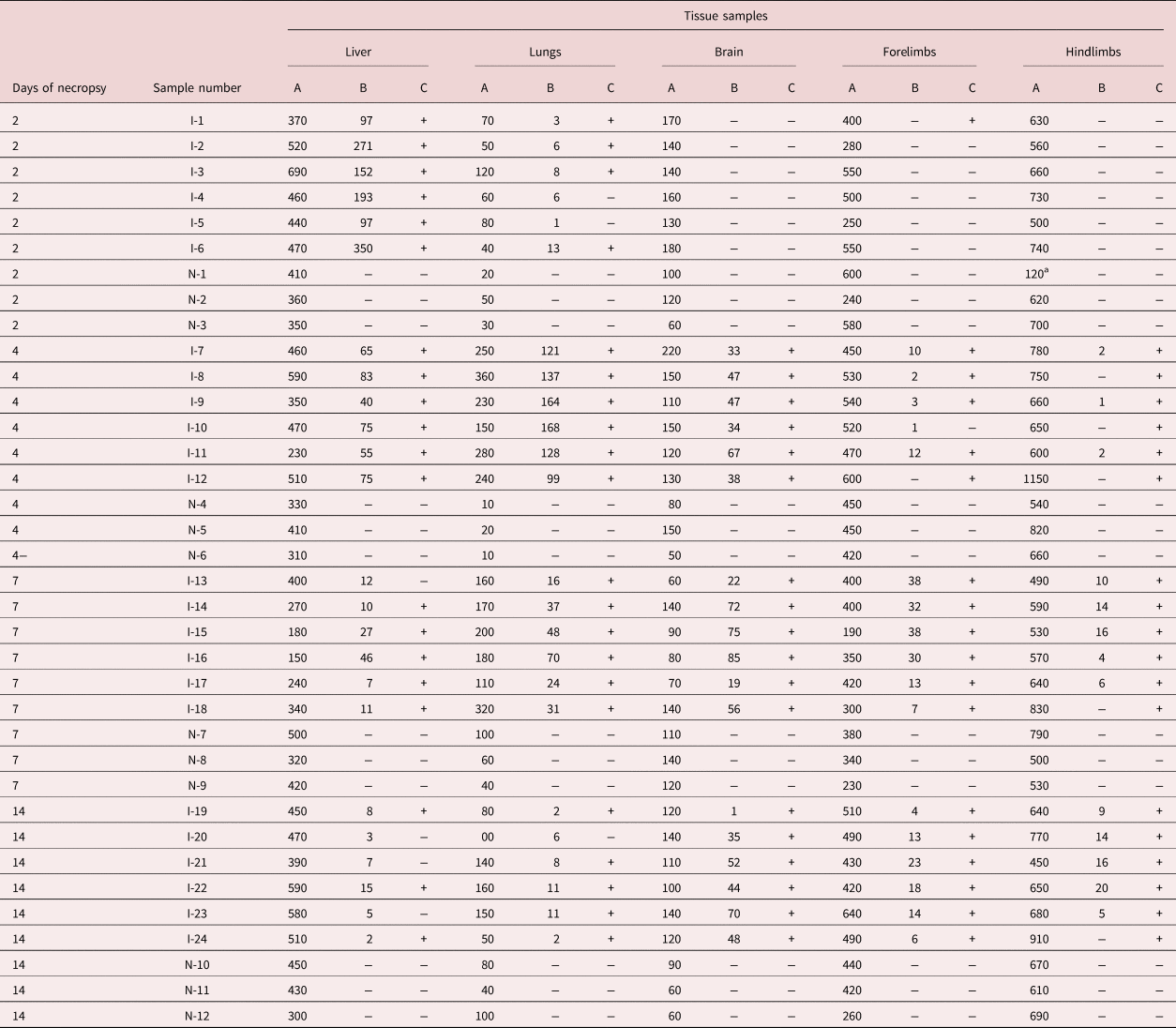
A, digested tissue (mg); B, number of larvae; C, PCR results; I, infected BALB/c; N, non-infected BALB/c; +, PCR positive; −, PCR negative.
a This tissue was weighted without bone.
Results of the VLM syndrome model
The presence/absence of larvae observed in infected BALB/c mice was consistent with the parasite biology. On the second day post-infection, larvae were found in the liver and lung tissues of all infected BALB/c groups; nonetheless, larvae were not found in the brain or the fore- and hindlimbs. However, the average number of these larvae gradually decreased each day due to larval migration (table 2).
Table 2. Number of larvae detected in different tissues and necropsy days in infected BALB/c groups.

** P < 0.01, ***P < 0.001.
abDifferent superscripts in the same column for each tissue represents statistically significant difference.
Larvae were detected in brain tissue beginning on the fourth day, and the highest average value was recorded on the seventh day post-infection.
The larvae began to appear in the fore- and hindlimb muscles on the fourth day post-infection. Samples I-12 in the forelimb and I-8, I-10, I-12, I-18 and I-24 in the hindlimb were negative on the fourth, seventh and 14th days of necropsy. The highest average larval count for forelimb muscles was detected on the seventh day post-infection (tables 1 and 2). Nonetheless, the average number of larvae in hindlimb tissue established a correlation, which increased daily. A difference was observed between the average larval number in the fore- and hindlimb muscles, with more larvae being observed in forelimb muscle tissue (table 2). No larvae were found in the non-infected BALB/c groups (table 1).
The descriptive statistics of the gram tissue are recorded in table 2. Larvae were encountered in the liver and lung of infected BALB/c mice on all days of necropsy. The highest median values in gram tissue were recorded on the second day of necropsy in the liver (341.0) and on the fourth day of necropsy in the lung (470.5). While larvae were not encountered in the brain or the fore- and hindlimb muscles on the second day of necropsy, the highest median values were recorded on the seventh day of necropsy.
In infected BALB/c mice, the number of larvae in the liver showed a similarity in the first two necropsy days but observed a significant decrease on the following days (P < 0.001). In the lungs, a significant increase in the number of larvae was observed between the second and fourth days, while a significant decrease was noted between the fourth and 14th days post-infection. A gradual increase was detected in the number of larvae in the brain tissues depending on the necropsy days. The larvae of the fore- and hindlimb muscles appeared to be observed on the same days, and the number of larvae increased on the seventh and 14th days of necropsy compared to the fourth day (table 2).
Results of PCR–RFLP
The new primers were reacted with cat, dog and human genomes, and no amplicons were observed. Negative results were also obtained for the T. cati and T. leonina samples.
The sensitivity of the PCR for T. canis eggs was determined. The PCR results for the samples taken on the relevant days of necropsy are given in table 1. As expected, the PCR results of all samples from the non-infected BALB/c groups were negative. For the PCR products, BbsI restriction endonuclease was cut from one position. Two DNA fragments were observed at 170 and 47 bp in size (fig. 1).
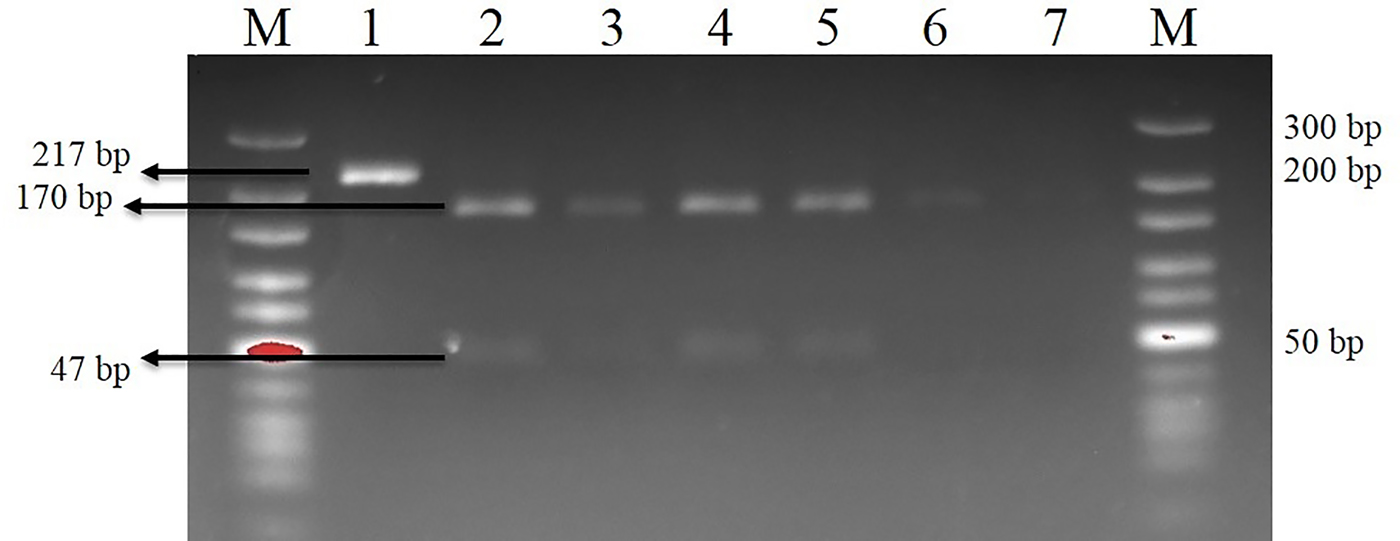
Fig. 1. Bbs-I reaction on the 14th day of necropsy (sample I-22). M, Marker (10–300 bp); 1, Toxocara canis positive control (uncut amplicon); 2, T. canis positive control (cut amplicon); 3, liver; 4, lungs; 5, brain; 6, forelimb muscles; 7, hindlimb muscles.
The difference between the PCR results and the digested amounts of larvae in all tissues was statistically significant (table 3). The frequency distribution of the PCR results for each tissue was examined. The highest sensitivity and accuracy were found in the lung. The ability to detect negative PCR results in all non-infected tissues in BALB/c mice was 100% (table 4).
Table 3. Descriptive statistics of PCR results on digested tissues.

*** P < 0.001.
Table 4. Frequency distribution for PCR results.
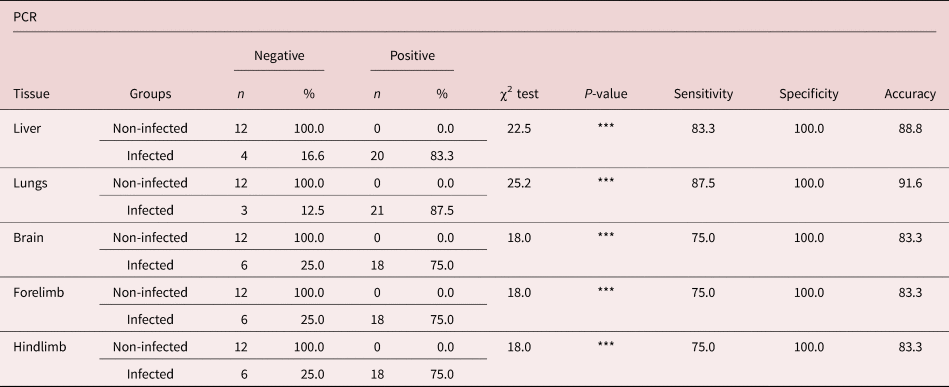
*** P < 0.001.
Discussion
Non-specific tests (blood count, total serum IgE levels, etc.), direct optical diagnosis and immunodiagnosis are used to detect VLM (Fillaux & Magnaval, Reference Fillaux and Magnaval2013). Due to the disadvantages of these tests, molecular techniques have been developed to diagnose VLM (de Moura et al., Reference de Moura, de Macedo, da Silva Terto, da Costa Avila, Leite, Scaini, Natália Berne Pinto, de Almeida, Strothmann, MarreiroVillela and Berne2018).
In the current study, VLM was conducted in 36 female BALB/c mice with embryonated T. canis eggs, and mice were sacrificed on the second, fourth, seventh and 14th days post-infection. Detection of larvae in the liver, lung, fore- and hind limb muscle tissues was supported by pepsin-HCl digestion, and the brain samples were squashed and observed microscopically to determine the credibility of the study. The study aimed to diagnose larvae in these tissues using a molecular method.
The days of necropsy were determined considering the biology of the parasite. There are studies showing that larvae were observed in liver tissue within the first 24 h (Burren, Reference Burren1968; Bisseru, Reference Bisseru1969); in lung tissues within the first three days and on the sixth day at its highest level (Sinha, Reference Sinha1966; Burren, Reference Burren1968); in brain tissues after 2–3 days post-infection (Sprent, Reference Sprent1958; Sinha, Reference Sinha1966; Bisseru, Reference Bisseru1969; Burren, Reference Burren1968, Reference Burren1971; Ollero et al., Reference Ollero, Fenoy, Cuellar, Guillen and Aguila2008), and on the seventh day, as well as in brain tissue (Oshima, Reference Oshima1961; Abo-Shehada & Herbert, Reference Abo-Shehada and Herbert1984/1985). Larvae can be observed in muscle tissues on the first two days (Sprent, Reference Sprent1958; Burren, Reference Burren1968; Abo-Shehada & Herbert, Reference Abo-Shehada and Herbert1984/1985), and some researchers found larvae on the third to seventh days post-infection (Oshima, Reference Oshima1961; Abo-Shehada & Herbert, Reference Abo-Shehada and Herbert1984/1985). In our study, in keeping with the biology of the parasite, larvae were found on similar days post-infection as with the aforementioned studies (table 1).
Molecular diagnosis is commonly used. One of the most significant drawbacks of this method is the false negatives that are encountered depending on the DNA or RNA concentration obtained from the sample. Jacobs et al. (Reference Jacobs, Zhu, Gasser and Chilton1997) reported that for Tcan-NC2 primers, the lowest concentration of T. canis DNA detectable by PCR was on the order of 0.1–0.5 ng/μl−1. Li et al. (Reference Li, Lin, Chen, Sani, Song and Zhu2007) reported that in each PCR process diagnosed as T. canis, the smallest amount of T. canis DNA was 0.14 ng, which was less than a single nematode larva. Furthermore, referring to the related literature, Borecka et al. (Reference Borecka, Gawor, Niedworok and Sordyl2008) surmised that 0.8, 0.4, 0.09 and 0.09 larvae could be detected with PCR on the third, fifth, seventh and 14th days post-infection in the liver. However, in the present study, PCR was conducted by morula and/or embryonated eggs into portions of up to ten eggs consecutively, and amplicons were recorded as two or more eggs. The DNA were measured as 5.1–11.2 ng/μl.
Some studies were performed with different amounts of tissues to isolate DNA (Wang et al., Reference Wang, Shibata, Nguyen, Hayata, Nonaka, Maruyama and Yoshida2018). According to our study design, we used 20 mg of tissue and obtained positive PCR results. This finding might be related to the infection dose.
In previous studies, the primers were reacted with other ascaridoid species, and each specific primer had been negative in other parasite samples (Jacobs et al., Reference Jacobs, Zhu, Gasser and Chilton1997; Li et al., Reference Li, Lin, Chen, Sani, Song and Zhu2007). In our study, the suitability of the new primers was compared with that of T. cati and T. leonina, and no amplicons were detected. The negative results suggest that a specific diagnosis of T. canis can be achieved with current primer pairs.
When the methods used in the study were compared, the larvae were observed in four samples (I-13, I-20, I-21, I-23) of liver tissue and in three samples (I-4, I-5, I-20) of lungs on the second, seventh and 14th days post-infection with pepsin-HCI digestion, while the PCRs that belong to the samples were negative. Moreover, while the PCR was negative in sample I-10 in the forelimb on the fourth day of necropsy, larvae were observed in same tissue with digestion. These results indicate that the tissues contained no piece of the larva. Some researchers (Borecka et al., Reference Borecka, Gawor, Niedworok and Sordyl2008; de Moura et al., Reference de Moura, de Macedo, da Silva Terto, da Costa Avila, Leite, Scaini, Natália Berne Pinto, de Almeida, Strothmann, MarreiroVillela and Berne2018) have also reported similar data in their studies.
On the second day of necropsy, the PCR result was positive in forelimb sample I-1, and no larvae were observed in the digestion. On the same day, the results of the remaining forelimb muscle samples were negative for both methods (table 1). This finding, which was encountered on the second day of necropsy, shows that there was a piece of larval DNA on the tissue. It is possible to obtain similar findings, as some researchers (Sprent, Reference Sprent1958; Burren, Reference Burren1968; Abo-Shehada & Herbert, Reference Abo-Shehada and Herbert1984/1985) recorded that larvae were seen on the muscle tissue on the first and second days post-infection. In our study, the PCR results of five hindlimb muscle samples (I-8, I-10, I-12, I-18 and I-24) were positive on the fourth, seventh and 14th days post-infection, but no larvae were observed in their digested samples. The positive PCR results obtained suggest that the utilization of PCR in tissue samples is a suitable method for diagnosing VLM (T. canis).
In some VLM patients, the syndrome could be caused by the consumption of raw-uncooked organ/meat from paratenic hosts. Therefore, some studies were performed on this transmission by molecular diagnosis. The organs (liver, heart, lung, kidney, brain and intestine) of paratenic hosts were investigated with the digestion method and PCR technique. The results of both techniques revealed that PCR is more reliable and able to detect species of parasites (Zibaei et al., Reference Zibaei, Sadjjadi and Maraghi2017; Wang et al., Reference Wang, Shibata, Nguyen, Hayata, Nonaka, Maruyama and Yoshida2018). Although larvae could be observed by using the digestion method, this approach took a long time and required extra effort. It was also specified that a piece of larva could not be observed via this method. However, this approach would give positive PCR results, as it has a piece of Toxocara DNA (Ishiwata et al., Reference Ishiwata, Shinohara, Yagi, Horii, Tsuchiya and Nawa2004; Wang et al., Reference Wang, Shibata, Nguyen, Hayata, Nonaka, Maruyama and Yoshida2018).
PCR is a convenient procedure for epidemiological studies (detection of eggs to diagnose the source of environmental contamination, etc.) regarding Toxocara infection (Smith et al., Reference Smith, Holland, Taylor, Magnaval, Schantz and Maizels2009). Ascaridoid eggs in the soil samples that were distinguished as T. canis and T. cati can help to measure the prevalence of syndromes that could appear in humans (Borecka, Reference Borecka2004; Fogt-Wyrwas et al., Reference Fogt-Wyrwas, Jarosz and Mizgajska-Wiktor2007). The results of the present study have shown that Toxocara sp. eggs could also be identified as T. canis via this protocol. The current primer was also checked to prevent any reactivity that could occur with the cat, dog and human genomes; this step was also taken in similar molecular studies (Jacobs et al., Reference Jacobs, Zhu, Gasser and Chilton1997; Li et al., Reference Li, Lin, Chen, Sani, Song and Zhu2007). There were no amplified DNA in the dog, cat or human samples.
In conclusion, the present study shows that the designed primers can be reliable to determine the identity of Toxocara sp. eggs as T. canis in the faeces, paratenic host, sand and raw vegetables/fruits. Moreover, it is proposed that this method can also be applied in human tissues that were already diagnosed as VLM positive with histopathological examination in order to determine whether T. canis was the responsible ethiological factor.
Acknowledgement
This study was produced from a first author's PhD thesis, named ‘Toxocara canis ile deneysel enfekte farelerde visceral larva migrans in PCR ile Teşhisi (PCR detection of visceral larvae migrans in mice experimentally infected with Toxocara canis)', presented at Ankara University.
Financial support
This research received no specific grant from any funding agency, commercial or not-for-profit sectors.
Conflicts of interest
None.